- 1Department of Land Improvement, Environmental Development and Spatial Management, Faculty of Environmental and Mechanical Engineering, Poznań University of Life Sciences, Poznań, Poland
- 2Department of Soil Science and Land Reclamation, Faculty of Environmental and Mechanical Engineering, Poznań University of Life Sciences, Poznań, Poland
- 3Department of Mathematical and Statistical Methods, Faculty of Agronomy, Horticulture and Bioengineering, Poznań University of Life Sciences, Poznań, Poland
River-lake systems in Central Europe represent the majority of surface water system forms. In these systems lakes play an important role in river water quality. Published reports on the quality of surface waters in Europe indicate progressive deterioration of their quality, resulting mainly from increasing eutrophication. This study analyzed the content of two biogenic elements—nitrogen and phosphorus—and their mineral forms in the Głuszynka river, representative for the river-lake systems of Central Europe. The research was conducted in the hydrological years 2016–2018. The ecological status of the Głuszynka river, due to the “poor” status of both biological elements and physicochemical elements (content of phosphorus and nitrogen compounds), was classified as “poor.” In the period analyzed an increase in the content of nitrogen compounds was recorded in the hydrological year 2018. However, during the growing period a significant decrease in the content of total and nitrate nitrogen was observed, which was related to the activity of primary producers. For phosphorus compounds a slight increase of their content was observed during this period. This was associated with high tourist and recreational pressure on the analyzed system. Analyzing the spatial variability of biogenic compounds it was observed that along the course of the river the content of nitrite and nitrate nitrogen as well as total nitrogen increased at successive sampling points. An opposite trend of change along the river course was observed for phosphorus compounds (content of P-PO4 and total phosphate decreased by 14 and 15.9%, respectively). Statistical analyses carried out highlighted the relationship between water quality and land use in the direct catchments of lakes included in the river-lake network. Arable land was associated with higher the content of orthophosphorus phosphate, grassland total nitrogen, nitrite and nitrate nitrogen, while urbanization was strongly associated with ammonium nitrogen.
Introduction
Surface water resources around the world are under constant pressure. Rapidly growing populations leading to intensification of agricultural production and also human economic activities, increasing urbanization and climate change negatively affect water quality. Despite the implementation of the objectives of the Water Framework Directive 2000/60/EC of the European Parliament and of the Council of 23 October 2000 establishing a framework for community action in the field of water policy (OJ EU.L.00.327.1), the ecological status of surface waters in the European Union is still unsatisfactory. Detailed identification of pollution sources in catchment areas, their transfer routes to surface waters, and phenomena occurring in aquatic ecosystems are crucial for water protection. Failure to protect and improve water quality can lead to serious consequences in terms of the provisions of the Water Framework Directive as well as social consequences. Flowing waters as well as standing waters (natural and artificial reservoirs) that are polluted become useless to residents for both consumption and recreation. Therefore, in order to achieve at least their good ecological and chemical status, it is necessary to develop a water management plan and protect them from pollution such as surface runoff into watercourses. By consciously exploiting the relationships between catchment area processes, ecosystem capacity, resilience, and the ability to respond flexibly to threats can be increased.
The intensification of eutrophication processes in surface waters, especially in reservoirs, observed in recent years is related to many environmental factors. This process is caused by increased loads of phosphorus (P) and nitrogen (N) entering surface waters (Saunders and Kalff, 2001; Elser et al., 2007; Chislock et al., 2013; Li and Yao, 2015; Tan et al., 2017; Kuss et al., 2020; Matej-Lukowicz et al., 2020; Tao et al., 2021).
The main sources of phosphorus in surface waters are both raw and treated sewage, and stormwater entering the water body (Nausch et al., 2017; Stackpoole et al., 2019; Pietrzak et al., 2020). Because of its limited migratory capacity due to its bioaccumulation, sorption, and low solubility, phosphorous enters surface waters from surface runoff in smaller amounts than nitrogen ( Kanclerz et al., 2015; Wiatrowska and Komisarek, 2015). In areas intensively fertilized with phosphorus, this element may enter waters in larger quantities with surface runoff, but it migrates mostly in the form of adsorbed solid phase particles, and to a lesser extent in ionic form (Grabinska et al., 2004; Pokojska and Bednarek, 2021). This phenomenon applies in particular to sandy soils with low phosphorus retention capacity used for agricultural purposes. Due to the nature of lithogenic materials, such soils are common for the area of Poland (Kondracki, 2002).
Nitrogen compounds, on the other hand, enter surface waters mainly with surface runoff from agricultural areas and also with wastewater, or as precipitation (Dojlido 1995; Allan 2004; Lossow et al., 2006; Causse et al., 2015; Burzyńska 2019; Kuss et al., 2020). Between 1961 and 2000, there was a significant increase in global nitrate fertiliser use (by about 600%), which had a major impact on surface water quality (Revenga et al., 2000).
According to the European Environment Agency, surface water pollution in the European Union is mainly related to agricultural pressures, and due to significant spatial differences related to climate, soil type and chemistry, geological conditions, topography and diverse agricultural activities, the negative impact of nutrients on surface waters varies across the EU (Vagstad et al., 2004; Kristenen, 2012). Recent research by the European Environment Agency (EEA) shows that measures to reduce emissions of nitrogen and phosphorus compounds have led to improvements in surface water quality. According to the Water Framework Directive, European Union Member States should aim to achieve at least good water status, but only 44% of surface waters in Europe achieve good or very good ecological status (Bruyninckx, 2020). According to the EEA report, Belgium, Hungary and Denmark had the lowest surface water quality compared to other European countries. Poland ranks slightly better in this classification, as nearly 50% of the surface waters studied were classified as surface waters with good or very good ecological status (Kristensen et al., 2018).
One of the main parameters affecting surface water quality is the way in which the catchment area is developed and used (Ye et al., 2013; Potasznik and Szymczyk, 2015; Yang et al., 2016; Kuriata-Potasznik et al., 2018; Djodjic et al., 2021). Reservoirs and rivers located in lowland areas, whose catchment areas are intensively used for agriculture, are particularly exposed to the eutrophication process. At the same time, these areas are dominated by river-lake systems (Saunders and Kalff, 2001; Foley et al., 2005; Dabrowska, 2008; Woli et al., 2008; Varanka and Luoto, 2012; Bajkiewicz-Grabowska et al., 2020). According to Kuriata-Potasznik (2018), in these systems, rivers act as transporters of nutrients while lakes serve as temporary retention sites. The processes of nutrient deposition in the bottom sediments of lakes depend on the physicochemical conditions in the water body. At the same time, the assimilation of nutrients by primary producers occurs in lakes during the growing period, which consequently reduces the content of these elements in surface waters (Saunders and Kalff, 2001; Sun et al., 2018; Uuemaa et al., 2018). Depending on the processes occurring in lakes, different trends of water quality changes were observed in the rivers below them. Thus, in river-lake systems, both improvement and deterioration of water quality parameters are observed depending on the element considered as well as changes in the activity of primary producers. A large role in shaping the chemistry of river waters is played by bottom sediments, which on the one hand can accumulate nutrients, temporarily excluding them from the biological cycle, or release them into the water (Hillbricht-Ilkowska, 1999; Glińska-Lewczuk, 2006; Sobczyńska-Wójcik and Rafałowska, 2011; Kuriata-Potasznik et al., 2020). Previous work dealing with water quality in river-lake systems has focused primarily on analyzing the total content of various elements, mainly biogenic. Unfortunately, there are no data on the behavior of individual chemical forms of biogens, especially nitrogen, which determine their bioavailability and thus the quality of aquatic ecosystems.
The study analyzed the temporal and spatial variability of phosphorus and nitrogen and their mineral forms in the waters of the river-lake system of the Głuszynka river. The results of the study were related to the land use of the catchment area, in order to make an attempt to indicate the main causes of water quality deterioration in the analyzed catchment area. For this purpose, the following hypotheses were formulated: 1) The quality of surface waters depends primarily on the way the catchment is used. The study assumes that agricultural and urbanized areas with incomplete sewage management will contribute to an increased content of nutrients, especially nitrogen and phosphorus, as opposed to forest areas, where the content of these elements in water should decrease. 2) Taking into account the biogeochemistry of nitrogen, it was assumed that both in the case of the content of total nitrogen and mineral forms of nitrogen, an increase in their content would be observed along the course of the river. On the other hand, in the case of phosphorus, which is easily precipitated, its concentration will decrease along with the flow through reservoirs with a longer water residence times (longer than 1 year). 3) The dynamics of changes in nitrogen content and its forms will be influenced by the activity of primary producers. For phosphorus, due to the possible supply from bottom sediments, such an effect will not be observed. To test these hypotheses, we analyzed data collected from 7 sampling points located along the Głuszynka river course for the period of hydrological years 2016–2018 (Figure 1).
Methods and Materials
Study Area
The catchment area of the Głuszynka river is 134.72 km2 and it is situated in central-western Poland. According to the physico-geographical division of Poland it is located in the south of the Baltic Lakelands (Kondracki, 2002). The study area is located within the range of the Weichselian glaciation.
According to Corine Land Cover 2018, 60% of the catchment area is dominated by agricultural land. The largest part of the area is non-irrigated arable land coded 211. Anthropogenic land constitutes 10.41 km2 (7.87% of the catchment area) with a predominance of discontinuous urban fabric (code 112). In turn, forests and semi-natural ecosystems constitute an area of 32.56 km2 (24.63%) (Figure 2). The attractive location and natural values of the Kórnik-Zaniemyska Gutter make the lakes particularly interesting for tourists, which may be an additional burden for the catchment area (Rosińska and Gołdyn 2018).
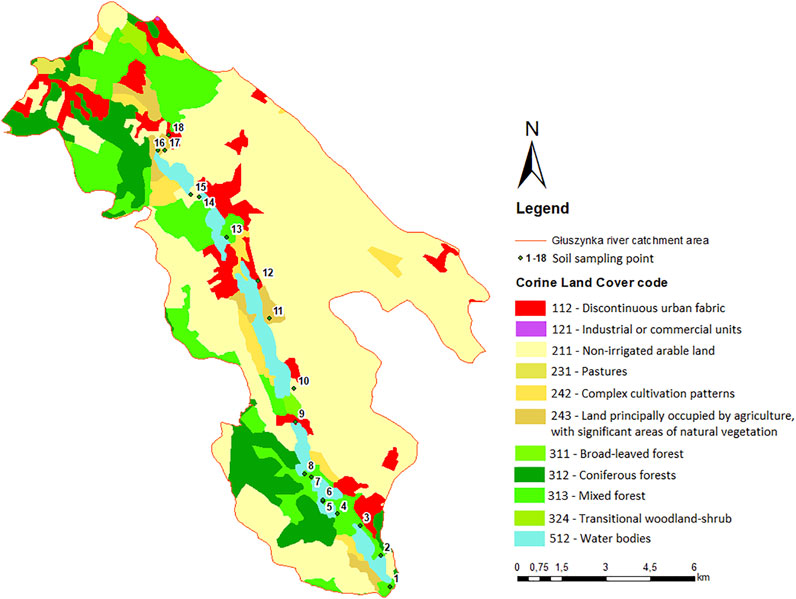
FIGURE 2. Structure of land use of the Głuszynka river catchment area (source: CLC 2018).
The 24.4 km long river-lake system of the Głuszynka river consists of eight lakes in the Kórnik-Zaniemyśl Gutter connected by short sections of river, where the ratio of the length of the river sections to the total length of the river sections flowing through the lakes is 0.62. The source of the Głuszynka river is considered to be Lake Raczyńskie. The river then flows through the following lakes: Łękno, Jeziory Małe, Jeziory Wielkie, Bnińskie, Kórnickie, Skrzynki Duże and Skrzynki Małe (Figure 1). The largest lake of this river-lake system is Lake Bnińskie (0.225 km2).
Taking into account the land use structure of the immediate catchment areas of the lakes of the studied system, two groups of catchments were distinguished in the study: agricultural (with the percentage of agricultural land from 58.8 to 81.7%) and forest-agricultural (with the share of forests and semi-natural ecosystems from 35.2 to 71.4%) (Table 1). This division of the immediate catchments was performed using the agglomeration method in the Statistica 13.3 program. (TIBCO Software Inc., United States).
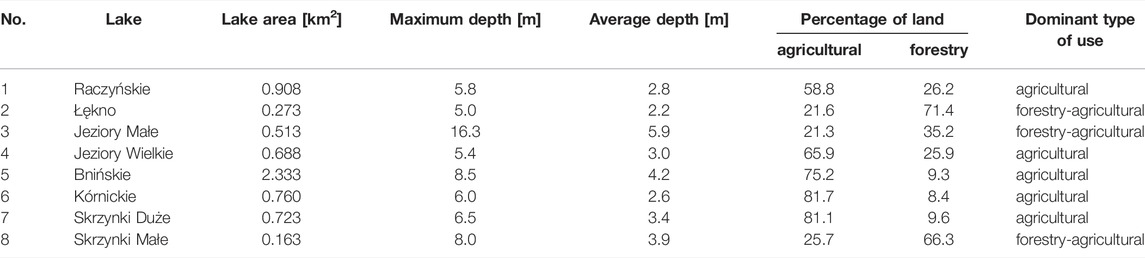
TABLE 1. Basic characteristics of lakes of the Kórnik-Zaniemyska Gutter (source: Choiński 2006, own research).
The catchment area is mainly covered with Luvisols. The parent materials of these soils are glacial till and loamy sand. The analysed catchment area consists mostly of coarse-textured soils. It is dominated by fine loamy sand (Supplementary Table S1). These are easily permeable formations with low retention capacity, and in the case of intensive fertilization of these soils, there is a real risk of rapid transport of nutrients to surface and groundwaters.
Cartographic Works
The cartographic works involved an analysis of the structure of the catchment area according to Corine Land Cover 2018 and a morphometric analysis of the lakes of the Kórnik-Zaniemyska Gutter. In terms of the land use structure of the immediate catchment area of the lakes, two groups of catchment areas were distinguished: agricultural (agricultural areas from 58.8 to 81.7%) and forest-agricultural (forest areas from 35.2 to 71.4%). All spatial analyses were performed using ArcMap 10.7.1.
The meteorological data were provided by the Institute of Meteorology and Water Management—National Research Institute, for the period from 1990 to 2019. The classification of precipitation condition of individual years studied was performed according to the methodology of Kaczorowska (1962). In this classification, the precipitation was calculated on the basis of the percentage of precipitation in a given year over the multi-year average (at least 30 years) (WMO 2009). The thermal condition classification was performed according to the method developed by Lorenc (2000). Characteristics such as average temperature, average value for the multi-year period and standard deviation for the multi-year period were used in the thermal classification.
The assessment of the ecological status of surface waters was made on the basis of the Regulation of the Minister of Infrastructure of 25th June, 2021 (Journal of Laws 2021, item 1475, 2021) on the basis of the evaluation of biological elements obtained from the Inspectorate of Environmental Protection (GIOŚ, 2018) and physicochemical elements.
Field Works
The samples of surface water for the laboratory analyses were collected from 7 sampling points (sp) located on the Głuszynka river and they were marked with the alphanumeric code (G1-G7) (Figure 1), at a frequency of once per month during hydrological years in the period 2016 to 2018. The water samples were collected in 1 L polyethylene bottles which were transported afterwards at the temperature of 4°C and analyzed in the laboratory within 48 h of their collection.
In the profiles located on the river, measurements of the water flow velocity were carried out using a Valeport hydrometric mill, and the water flow intensity in the river was calculated using the Harlacher calculation method (Byczkowski, 1996). The water exchange in lakes was determined on the basis of the capacity of the lake basin and the average river water flows in the sections between the lakes (above and below the lake).
Taking into account the predominantly agricultural nature of the catchment area, the abundance of available phosphorus in the soils was also analyzed. For this purpose, 18 composite soil samples were collected from the lakeshore of the studied river-lake system (Figure 2). The samples were collected based on a cross-shaped plan with an arm length of 1 meter, in duplicate from the surface horizon (0–20 cm).
Laboratory Work
The following parameters were determined in the collected samples of surface water: total nitrogen, ammonium nitrogen, nitrite nitrogen, nitrate nitrogen, total phosphorus and orthophosphate phosphorous (V). The measurements were done in accordance with the current standard. The analyses were performed in duplicate and the data were presented as averaged values. The content of total nitrogen was determined by Koroleff’s method according to the standard BS EN ISO 11905-1:1998 (Water quality. Determination of nitrogen) with measurement uncertainty 0.15 mg N dm−3. Mineral forms of nitrogen were determined spectrophotometrically using EPA method 354.1 (Nitrite by Spectrophotometry) with measurement uncertainty 0.02 mg N-NO3 dm−3 and with measurement uncertainty 0.0083 mg N-NO2 dm−3, but EPA method 350.1 (Determination of ammonia nitrogen by semi-automated colorimetry) for N-NH4 with measurement uncertainty 0.01 mg N-NH4 dm−3.
The concentration of orthophosphate phosphorous (V). and total phosphorus was determined according to the method EN ISO 6878, (2004). The content was measured using ammonium molybdate and ascorbic acid at 712 nm on a JENWAY instrument (7315 Spectrophotometer). However, total phosphorus content was determined after mineralization with ammonium persulfate. Measurement uncertainty for orthophosphate phosphorous (V) and for total phosphorous was 0.044 mg dm−3.
The soil texture was determined by Casagrande’s areometric method, modified by Prószyński according to PN-R-040032 standard (1998). The fine particles (smaller than ∅< 0.05 mm) were determined with the sedimentation method and in the case of the larger ones (0.05–2.00 mm) the sieve method was applied. The soil texture samples were classified according to the standards of the United States Department of Agriculture. The content of available phosphorus was determined using the Egner method (Mocek et al., 2006). The analyses were performed in duplicate and the results are presented as averaged values. For this study, an internal reference sample was used to check the accuracy of the performed analyses.
Other parameters were also determined during field and laboratory work: dissolved oxygen, temperature, EC, pH, BOD5, SO42−, Cl, H-CO3− and metal cations Na+, K+, Mg2+, Ca2+ (data not shown).
Statistical Analyses
Data in the study are presented as average values, in the case of water analysis results n = 9 and for soil samples n = 2. Both the spatial and temporal variability of selected parameters of surface water quality were analyzed. Due to the clear seasonal variability of parameters in the analysis of temporal variability, the sampling dates were divided into two groups: the growing period and the non-vegetative period. Months were separated into particular periods on the basis of the analysis of average daily air temperatures for individual months. The threshold temperature ≥ 5°C was adopted for a growing period. (Tomczyk and Szyga-Pluta, 2016).
In order to identify environmental factors influencing the content of nitrogen and phosphorus and their mineral forms in surface waters, principal component analysis (PCA) was used. This statistical analysis was performed using the Statistica 13.3 program (TIBCO Software Inc., United States). The PCA results were presented as biplots (Morrison, 1990; Falniowski, 2003). Parametric statistical change point analysis was used to determine the critical point of change in the content of the analysed parameters of surface water quality along the river course (Cobb, 1978; Killick and Eckley, 2014). This analysis was performed using the R program (R Development Core Team 2019).
Results
Characteristics of the Catchment Area
The catchment area of the Głuszynka river is characterized by the lowest annual rainfall in Poland. The average annual rainfall recorded in the years 1989–2018 was 571 mm, and the average annual air temperature in these years was 9.3°C. The hydrological years 2016–2018 in which the research was conducted were classified on the basis of precipitation as wet (2016), very wet (2017) and normal (2018), in which the sums of annual rainfall accounted for 125.99, 126.83 and 106.14% of the average multi-year precipitation, respectively (Supplementary Figures S1, S2). During the research period, the occurrence of torrential rains was recorded on 14th July 2016 (64.3 mm), in July 2017 (150.6 mm) and on 2nd June 2018 (99.3 mm), which resulted in an increase in the water flow in the river. Thermally, the hydrological years 2016 and 2018 were classified as warm, while 2017 was classified as normal.
The average of the mean annual flows of the Głuszynka river in the G7 water gauge profile in 2016–2018 was (SSQ) 0.39 m3 s−1, and the extreme flows ranged from the lowest annual low flow (NNQ), 0.047 m3 s−1 (12.07.2016), noted for G6, located between the lakes Skrzynki Duże and Skrzynki Małe to the highest annual high flow (WWQ), 2.18 m3 s−1 (02.02.2018). The lowest average flow in this profile was recorded in 2016 (NSQ = 0.23 m3 s−1) and the highest in 2018 (WSQ = 0.55 m3 s−1). The average annual unit outflow in 2016–2018 was 2.89 dm3 s– 1 km–2, and the impact on the river outflow ratio was 0.13. The lakes Łękno and Jeziory Małe are connected by a concrete culvert, so no sampling point was designated between these lakes.
The water residence times in the lakes of the Kórnik-Zaniemyska Gutter was diverse : Raczyńskie Lake 14 months, Lake Łękno 78 months, Lake Jeziory Małe 18 months, Lake Jeziory Wielkie 37 months, Lake Bnińskie 7 months, Lake Kórnickie 65 months, Lake Skrzynki Duże 9 months and Lake Skrzynki Małe 16 months.
Spatial Variability of the Content of Nitrogen and Phosphorus and Their Forms
The quality of surface waters, in accordance with the provisions of the Water Directive, is assessed on the basis of biological elements supported by physicochemical elements. This article focuses more on the temporal and spatial variability of nutrient content in the Głuszynka river, observed in the hydrological years 2016–2018. Taking into account the fact that the availability of nutrients significantly influences the growth of primary producers, the study tried to determine how the use of the catchment area and the rate of water change in water reservoirs affect their content in waters. However, the final assessment of the Głuszynka river waters presented in the paper takes into account both factors required by the Water Directive.
The content of total nitrogen in the Głuszynka river during the hydrological years 2016–2018, ranged from 0.87 mg N dm−3–9.32 mg N dm−3. The highest mean content of total nitrogen was observed at sp G6 (3.87 mg N dm−3) and the lowest at sp G1 (2.31 mg N dm−3) (Table 2). For mineral forms, a slightly different pattern of changes in the average content in individual sampling points was observed. Only the form with the highest oxidation state showed similar changes with the course of the river. The highest mean content of nitrate nitrogen was observed at point G5 (2.43 mg N-NO3 dm−3), and the lowest at point G1 (1.21 mg N-NO3 dm−3). In the case of nitrite nitrogen, the values of this nitrogen speciation ranged from 0.06 to 0.44 mg N-NO2 dm−3. The lowest mean content of this form of nitrogen was recorded at point G1 (0.08 mg N-NO2 dm−3), below Lake Raczyńskie, while the highest mean content of nitrite nitrogen was observed below Lake Skrzynki Duże (G6), 0.14 mg N-NO2 dm−3. The highest mean content of ammonium nitrogen was recorded at G2 (0.130 mg N-NH4 dm−3), and the lowest at G4 (0.102 mg N-NH4 dm−3) (Figures 3A–D). With nitrite and nitrate nitrogen as well as total nitrogen, a tendency of an increase in the content of these pools of nitrogen was observed along with the course of the river. The average content of nitrite nitrogen increased by 50% (G1—0.08 to G7—0.12 mg dm−3), total nitrogen by 67% (G1—2.31 to G7—3.86 mg dm−3) and nitrate nitrogen by 100% (G1 –1.21 to G7—2.42 mg dm−3). In the case of total nitrogen, nitrite and nitrate forms, a significant increase in the value from sp G4 below Lake Bnińskie was observed (Figures 3A–C). The increase in the mean content of these parameters from sp G1 to G4 was 44.55, 25.85 and 68.12% for total nitrogen, nitrite and nitrate nitrogen, respectively. The parametric statistical change point analysis performed confirmed that a significant increase in the content of nitrate nitrogen and total nitrogen occurred between G4 and G5 sites, and for nitrite nitrogen between G3 and G4 sites (Figures 4A–C). The above-mentioned change points were assigned on the basis of statistically significant changes in the values of these parameters.
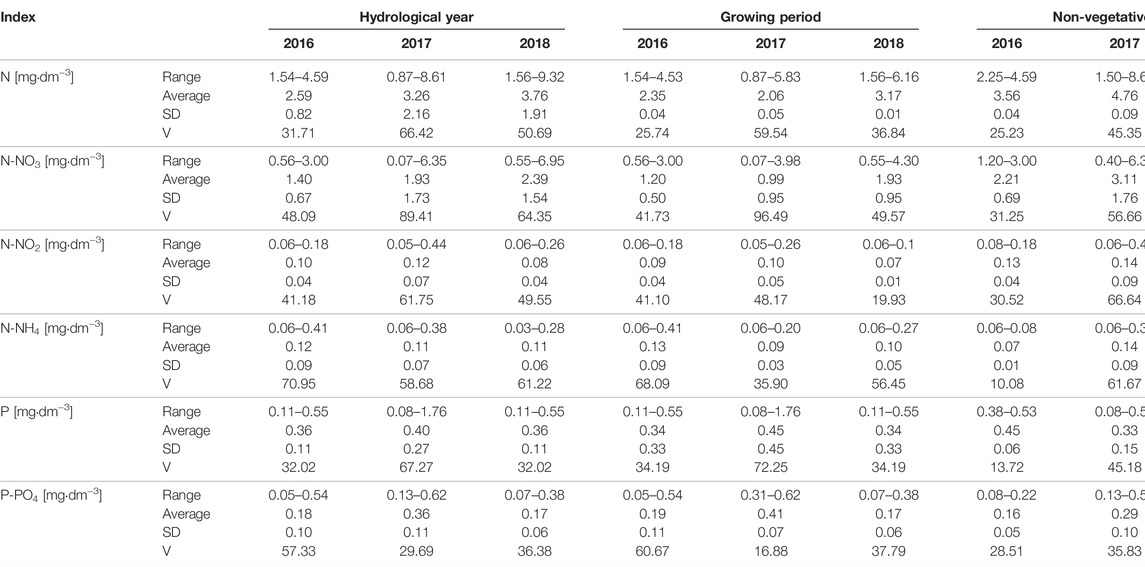
TABLE 2. Water quality indicators of the Głuszynka river during the hydrological years 2016–2018, broken down into growing and non-vegetative period.
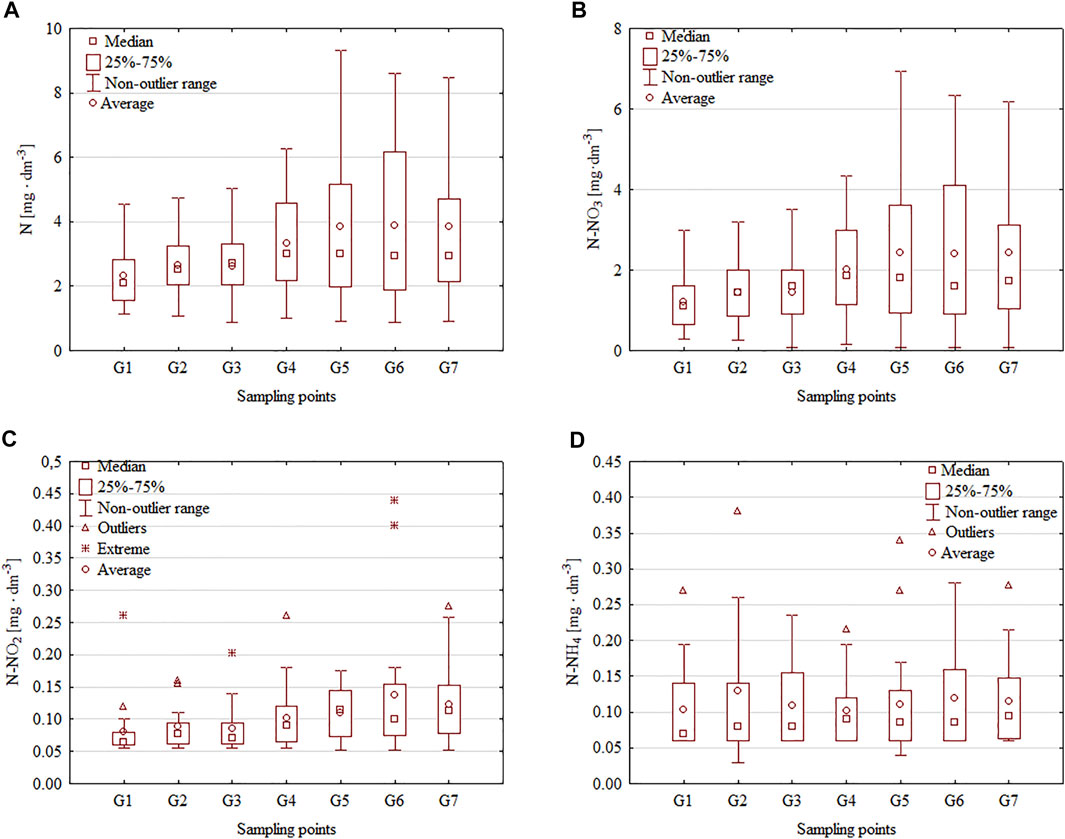
FIGURE 3. Spatial variability of: (A) total nitrogen; (B) nitrite nitrogen; (C) nitrate nitrogen; (D) ammonium nitrogen in the waters of the Głuszynka river in the hydrological years 2016–2018.
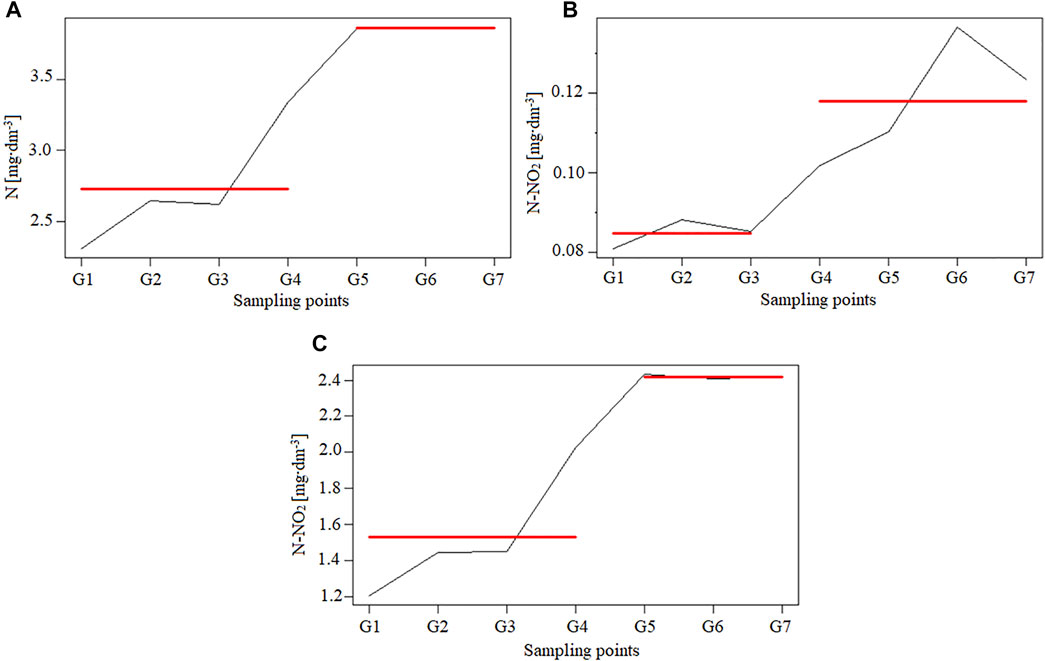
FIGURE 4. Average contents of (A) total nitrogen; (B) nitrite nitrogen; (C) nitrate nitrogen; in the waters of the Głuszynka river in the hydrological years 2016–2018, with the inflection point indicated.
The second element influencing the eutrophication process is phosphorus. Two forms of this element were analysed in this study: total phosphorus and orthophosphate phosphorus. The content of total phosphorus in the river waters during the studied years ranged from 0.08 to 1.76 mg P dm−3. The lowest mean value of this parameter was observed at sp G5 (0.32 mg P dm−3), and the highest mean value was recorded at sp G1 (0.44 mg P dm−3), below Lake Raczyńskie. High contents of orthophosphate phosphorus were observed in the Głuszynka river, ranging from 0.05 mg P-PO4 dm−3 to 0.62 mg P-PO4 dm−3. The lowest mean value of this parameter was recorded at point G6 (0.246 mg P-PO4 dm−3), and the highest mean value was recorded at point G1 (0.288 mg P-PO4 dm−3). Along the course of the river the quality of water improved with respect to the abovementioned indices. For orthophosphate phosphorus a 14% reduction was observed between sampling points G1 and G7 (G1—0.29 to G7—0.25 mg dm−3) and for total phosphorus a 15.9% reduction was observed (G1—0.44 to G7—0.37 mg dm−3) (Figures 5A,B). In the case of total phosphorus, the analysis of parametric statistical change point analysis showed no statistically significant change in the mean values of this indicator. However, it was detected in the case of orthophosphates. A statistically significant change in the content of this anion occurred between G3 and G4 sites (Figure 6). In the lower sections of the river, lower mean values were observed in the case of orthophosphate phosphorus (V).
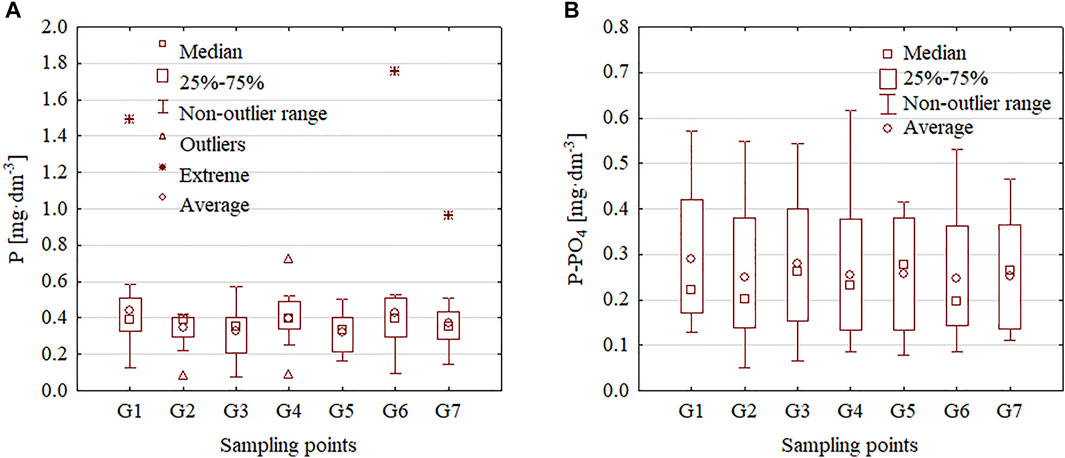
FIGURE 5. Spatial variability of the content of (A) orthophosphate phosphorous; (B) total phosphorus in the waters of the Głuszynka river during the hydrological years 2016–2018.
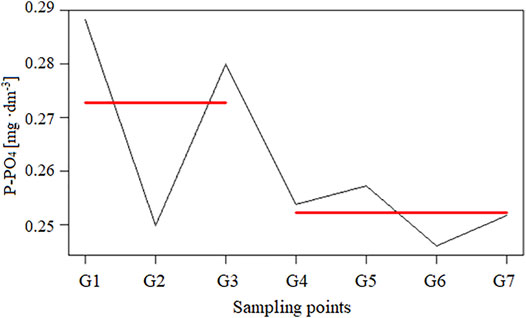
FIGURE 6. Average content of orthophosphate phosphorous in the waters of the Głuszynka river during hydrological years 2016–2018, with the inflection point indicated. Temporal variability of the content of nitrogen and phosphorus and their forms.
The content of total nitrogen showed high variability (CV = 58.11%) during the research period. The maximum content of total nitrogen was observed at sp G5 (9.32 mg N dm−3, April 2018), located below Lake Kórnickie (Table 2). Analyzing the annual mean content of total nitrogen, an increasing trend was observed for this parameter. The highest content of this element, 3.76 mg N dm−3, was observed in the hydrological 2018 years and was higher than the average annual content of this parameter by 44.9% compared to the hydrological year 2016. The highest variability of this parameter was observed in the hydrological year 2017 (CV = 66.42%). Also, the concentration of mineral forms of nitrogen (N-NO3, N-NO2 and N-NH4) showed high variability (CV = 78.57, 58.70 and 63.06%, respectively) in the analysed period. The highest content of N-NO3 was recorded at sp G5 (6.95 N-NO3 dm−3, April 2018). In the case of this form of mineral nitrogen (N-NO3), an increasing trend of its content was observed in successive research years. In the last analysed hydrological year, the content of this indicator was higher by 70.7% higher compared to the hydrological year 2016. However, the highest value of the coefficient of variation, 89.4%, was observed in the hydrological year 2017. For the nitrate nitrogen form (III), the highest value was recorded at sp G6 (0.44 mg N-NO2 dm−3, March 2017), while for ammonium nitrogen, it was recorded in May 2016, at sp G2 (0.41 mg N-NH4 dm−3). The content of these forms of mineral nitrogen (N-NO2 and N-NH4) did not show a visible trend of changes in the successive research years. However, as for the previous forms of nitrogen, the highest variability of N-NO2 was recorded in 2017 (CV = 61.75%). For the ammonium form only, higher variability was recorded in 2016 (70.9%).
The analyzed forms of phosphorus showed different variability. Also for total phosphorus, the highest variability of the content was observed in the hydrological year 2017, which was 67.3%. It was also the year in which the highest values for this parameter were recorded (0.08–1.76 mgP dm−3). In comparison to nitrogen, no significant changes were observed between the analyzed years for total phosphorus. A slightly different pattern was observed for orthophosphate phosphorous, for which the highest variability in content was observed in 2016 (CV = 57.3%), although much higher concentrations of this form of phosphorus were recorded in 2017 (Table 2).
One hypothesis put forward in the study assumes a significant influence of primary producers on the content of total nitrogen and its mineral forms in river waters. The results obtained for total nitrogen and nitrate nitrogen in the growing period were statistically significantly lower than for the non-vegetation period (p = 0.05) (Figure 7). In the growing period, the average content of nitrate nitrogen was 135% lower than in the non-vegetation period, and for total nitrogen 92% lower. However, for other mineral forms of nitrogen (N-NO2 and N-NH4), no statistically significant differences were observed between the separate periods. The analysis of the temporal variability of the content of total phosphorus and orthophosphate phosphorus confirmed that there were no significant differences between these periods. However, for orthophosphate phosphorous (V), higher concentrations of this ion were recorded during the growing period, especially in the hydrological year 2017, by 41%.
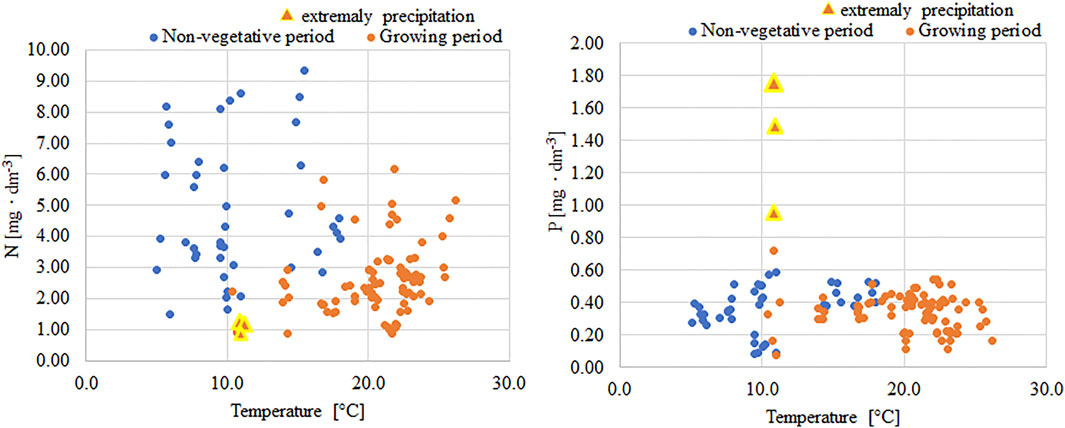
FIGURE 7. Total nitrogen and phosphorous concentration vs. air temperature during hydrological years 2016–2018.
The biogenic conditions in the river were characterized in this study based on the content of nitrogen and total phosphorus and their mineral forms. According to the Regulation of the Minister of Infrastructure, these parameters classified the ecological status of the waters of the Głuszynka river as below “good,” with the exception of ammonium nitrogen content, which met the threshold value for first class surface water quality (≤0.12 mg dm−3). The assessment of biological elements on the basis of phytoplankton, chlorophyll a, phytobenthos and macrophyte indexes conducted for the lakes of the Kórnik-Zaniemyska Gutter by WIOŚ in Poznań (2011–2018) classified waters of these lakes as “poor.” Taking into account the information on biological parameters of the analyzed river-lake system, the ecological status of the waters of the Głuszynka river were classified as “poor.”
Discussion
In this study, an attempt was made to determine the variability of phosphorus and nitrogen content and their mineral forms in the waters of the river-lake system of the Głuszynka river, both in temporal and spatial terms. The results of the river water quality analysis obtained were compared to the mode of catchment management and the activity of primary producers.
In the discussed river-lake system, trends in the analyzed parameters with the course of the river were observed. In the case of total nitrogen and its mineral forms, an increase in their content was observed in successive sampling points. On the other hand, for total phosphorus and orthophosphate phosphorus, a decrease in the values of those parameters was noted along with the course of the river. The results obtained indicate the heterogeneous role of lakes in this system. In the case of phosphorus, lake basins probably accumulated phosphorus and thus the quality of waters flowing out of them improved. As regards nitrogen, the more mobile element, the inflow of its additional loads to lakes, including adjacent agricultural areas and tourist activity, resulted in deterioration of surface water along with the course of the river. Similar results were obtained by Kuriata-Potasznik (2018), who noted that lakes of river-lake systems can play an accumulation or transport role, depending on the morphometry and the size of loads introduced into the waters.
The ecological status of the waters of the Głuszynka river, due to the poor condition of both biological elements and physicochemical elements (the content of phosphorus and nitrogen compounds), was classified as “poor.” The only exception was ammonium nitrogen, on the basis of which the water of the river studied was classified as “good” (except for two measurement dates). The values of the coefficient of variation indicate high temporal variability of the parameters studied, which may result from the changing meteorological conditions, the activity of primary producers and the variable magnitude of anthropogenic pressure over time. This may explain the highest values of the coefficients of variation observed for 2017. It was a very wet year with an incident of torrential rain (July 2017), which caused a significant inflow of nutrients to the waters of the Głuszynka river (Iital et al., 2014; Øygarden et al., 2014; Nausch et al., 2017; Matej-Lukowicz et al., 2020) (Supplementary Figure S1).
The highest contents of total nitrogen and its mineral forms were observed in spring, which could indicate the transport of pollutants from nearby arable lands from drainage water, surface runoff, and groundwater. Similar results were obtained by Kneis et al. (2006), who observed the highest nitrogen content in early spring and a reduction of this parameter in the following months. Also Bhat et al. (2014) analyzing the river-lake system of the Skuhang river, observed a similar trend of temporal changes in relation to nitrite and nitrate nitrogen. The lower content of total nitrogen observed in the waters of the Głuszynka river during the growing period (on average by 48.85%) was probably related to the activity of primary producers and intensive photosynthesis. A similar trend was also observed for nitrate nitrogen, while in the case of ammonium nitrogen and nitrite nitrogen no differences were found between the growing and non-vegetative periods. This could be explained by the bioavailability (Haynes and Goh, 1978; Lewi, 1986; Kobus, 1996) of particular mineral forms of nitrogen. Only nitrate nitrogen and ammonium nitrogen could be assimilated by primary producers. Hence, no differences were observed between these periods for the nitrite form. In the analysed period the waters of the Głuszynka river were characterised by conditions favourable to nitrification processes, which led to rapid oxidation of ammonium to nitrate forms, which could result in a lack of observed variation between these periods (Van Loon and Duffy, 2007). The analyzed area was classified as especially vulnerable to agricultural nitrate pollution. The variability of nitrogen content in surface water may also have been influenced by groundwater. Rosińska and Gołdyn (2018), analyzing the specific conditions of one of the lakes of the Kórnik-Zaniemyska Gutter, reported that the external load reached the lake mainly with the gradual and delayed inflow of groundwater from soils polluted by sewage from leaky septic tanks in the past.
For both total phosphorus and orthophosphate phosphorus, no statistically significant differences were recorded in their contents between the analyzed periods. During the growing period, higher concentrations of total phosphorus were observed at four sampling points (G1, G4, G6 and G7), which could be related to the transport of this nutrient with surface runoff. Determination of available phosphorus content in soils along with soil texture conducted for soil samples collected from shores and banks supports this assumption. Soils of the analysed river-lake system were characterized mostly by loamy sand texture or coarser, which are easily permeable formations with low retention capacity. In combination with a high and a very high content of available phosphorus in these soils (6.74–20.95 mg P100g−1), favourable conditions for fast transport of phosphorus to surface waters were created. Municipal wastewater from the catchment area of the analysed river is directed to the wastewater treatment plants in town: Jeziory Małe, Borówiec and Poznań. Due to the lack of a chemical treatment stage, the treated wastewater discharged into the river is characterized by a high phosphorus load. According to the report made available by the treatment plants, the treated wastewater discharged into the analysed river contains up to 15 mg N dm−3 for total nitrogen and up to 2 mg P dm−3 for phosphorus. At the same time, the lack of clear seasonal differences in the phosphorus content in waters could also be related to the inflow of phosphorus loads from tourist and recreational activities, which in turn compensated for phosphorus assimilated by primary producers. According to Rosińska and Gołdyn (2018), approximately 8,000 people in total use the bathing areas in the summer.
In addition, a PCA analysis between lake catchment land use and mean nutrient contents in water was performed in this study. This analysis explained 80.4% of the variability of the data. The first factor, which accounted 58.16% of data variability, was mainly related to the values of positively correlated indices such as: total nitrogen, nitrite and nitrate nitrogen. These indicators were negatively correlated with the content of total phosphorus. The above-mentioned indicators were mainly related to the agricultural land cover, which according to CLC2018 were designated as 231 (pastures) and311 (broad-leaved forest). The second factor, accounting for 22.24% of the variability in the data, was associated with ammonium nitrogen content in water, the content of which was negatively correlated with orthophosphate phosphate (V) content (Figures 8A,B). Ammonium nitrogen content was associated with coniferous forest areas (312) and urbanized areas (112) (CLC, 2018; Supplementary Figure S2).
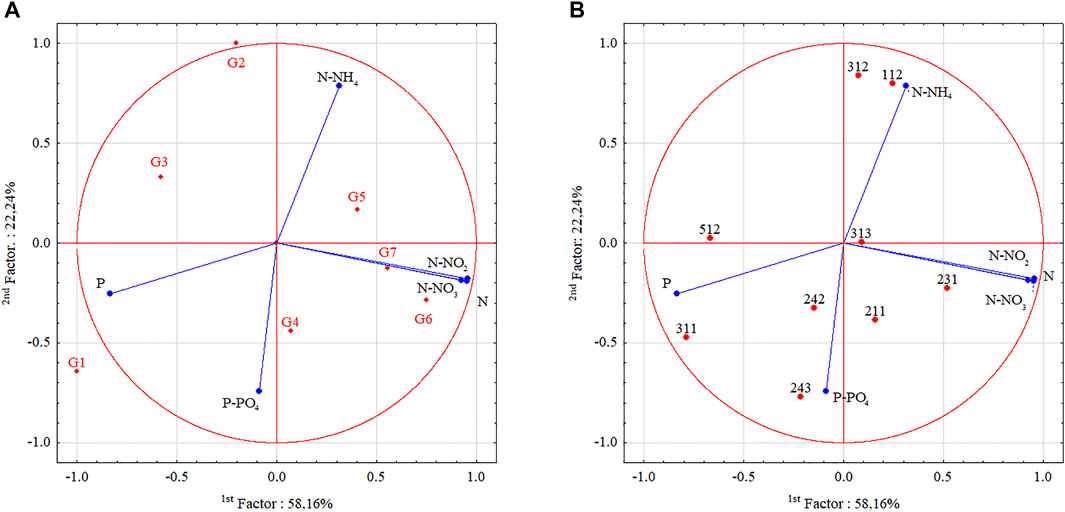
FIGURE 8. Analysis of the main components of the physicochemical parameters of the Głuszynka river water in relation to: (A) use of the catchment area of the Kórnik-Zaniemyska Gutter; (B) measurement points of the Głuszynka river downstream of the lakes (112—Discontinuous urban fabric; 121—Industrial or commercial units; 211—Non-irrigated arable land; 231—Pastures; 242—Complex cultivation patterns; 243—Land principally occupied by agriculture, with significant areas of natural vegetation; 311—Broad-leaved forest; 312—Coniferous forests; 313—Mixed forest; 324—Transitional woodland-shrub; 512—Water bodies).
The PCA analysis showed that the agricultural areas had the greatest impact on the increase in total nitrogen, N-NO3 and N-NO2 values. This was confirmed by the analysis of variability of these parameters. The greatest changes in the content of total nitrogen and nitrate nitrogen were observed at the G5, G6 and G7 sampling points, which are located below the lakes with a typically agricultural character of the catchment area. A significant change in the values of these parameters was confirmed by the analysis of the parametric statistical change point, which indicated that the inflection point was at sp G4, below Lake Bnińskie. It is the largest lake of this system with a typically agricultural catchment, which probably contributed to higher inflows of this element to surface waters. Also other lakes in the studied system were characterized by a significant share of agricultural land in the catchment area. It is likely that fertiliser materials used in agriculture increased the amount of mobile nitrogen forms, resulting in significantly higher nitrogen concentration in further sections of the river. Taking into account the fact that nitrogen is not subject to significant abiotic sorption processes and does not tend to precipitate as inorganic salts, the increased amounts of this element flowing from the catchment areas resulted in an increase in its concentration in the river waters. Tong et al. (2019) in their study on the variability of total nitrogen and phosphorus in water bodies highlighted the association of parameter variability with the location of the water bodies and anthropogenic factors. Similar conclusions were reached by Sobolewski et al. (2014), who reported that as the proportion of land used for agriculture increased, surface water quality deteriorated. Also, studies conducted by Burzyńska (2016) and Burzyńska (2019) indicate a significant influence of agricultural areas on the quality of surface waters. The worst quality of surface water was observed for samples collected from the vicinity of arable land and agricultural buildings. Similarly, Lossow et al. (2006), in a study on the Marózka river-lake system, flowing through Maróz lake, in the catchment area of which arable land constitutes 60%, also observed deterioration in the quality of water in terms of nitrogen. The total nitrogen content below the reservoir was 6% higher. A study conducted on the Skuhang river (Bhata et al., 2014) also identified agricultural activities, including excessive and incompetent use of fertilizers and pesticides along with unregulated water and sewage management, as the main causes of poor quality of surface water.
Many studies conducted on river-lake systems have detected an increase in the content of ammonium nitrogen along with the course of the river. Kuriata-Potasznik (2018) in the Symsarna river-lake system observed an increase in ammonium nitrogen by 44.1%, which was explained by the occurrence of the denitrification process. On the other hand, the research conducted by Burzyńska (2016) on the Raszynka river showed an increase in the content of ammonium nitrogen along with the course of the river, and the relationship between the intensity of rural and suburban development and municipal sewage discharges into the river was identified as the cause. No statistically significant changes (p ≤ 0.05) in the content of ammonium nitrogen along the river course were observed in the studied system. The analyzed catchment of the Głuszynka river is located in a suburban area, where the percentage of the catchment area with sewers does not exceed 61%. However, despite the high presence of urbanized areas in individual lake catchments (Bnińskie, Kórnickie, Skrzynki Duże lakes), it did not significantly affect the quality of the waters.
A completely different trend of total nitrogen changes with the river course was reported by Andersen (1994), Cook et al. (2010) and Wang et al. (2020). For the Danish Guedeny river-lake system, which has a typically agricultural catchment (78% arable land), a 46% reduction in total nitrogen was observed, mainly due to the denitrification process in lakes (Andersen 1994). Cook et al. (2010) ) in a study on the Australian river-lake system of the Murray river, observed a reduction of total nitrogen with the course of the river by 7%. They associated this observation with processes occurring in the basin of Alexandrina and Albert, which are part of the river-lake system. Wang et al. (2020) found a 58% reduction in total nitrogen in the Yuxi river in China as a result of water flow through Chaochu lake. In the river-lake system of the Głuszynka river, the total nitrogen content probably did not decrease with the course of the river as there were unfavourable conditions for the denitrification process. In the analysed river-lake system seven lakes are non-stratified reservoirs characterised by year-round overturn. As a result, the oxygen content was too high for the denitrification process to have a significant effect on the total nitrogen content in the surface waters of the studied river-lake system.
The waters of the analysed river-lake system were characterised by high contents of both total phosphorus (up to 1.76 mg dm−3) and orthophosphate phosphorous (0.05–0.62 mg dm−3), which classified them into an ecological status below “good.” The highest content of total phosphorus was observed at sp G6, located downstream of Skrzynki Duże lake with a large share of agricultural land in the catchment area, and at sp G1, located downstream of holiday resorts and numerous summer cottages around Lake Raczyńskie. High contents of this nutrient in the river-lake system of the Głuszynka river could have been caused by sewage discharges from nearby farms and surface run-off from nearby arable lands and recreational loads on the catchment area. The analysis of available phosphorus content in soils of the lakeshore supports these assumptions. In the majority of soils the abundance of this element was high or very high, which, combined with the coarse texture of these soils, creates favourable conditions for its transport (leaching) to surface waters (Supplementary Table S1) (Wiatrowska and Komisarek, 2015). During the study, the highest total phosphorus contents in the river waters were observed in months with the highest rainfall totals exceeding 150 mm (Janicka 2020). The river-lake system studied is located in a sewered area, but in the catchment boundary, there are still many leaking cesspits (underground sealed tanks) (The Local Data Bank, 2020).
Analyzing the spatial variability of the content of total phosphorus and orthophosphate phosphorous along with the river flow through the lakes, there was a decrease of P by 15.9% and P-PO4 by 14%. However, in the case of sampling points G4 and G6, due to the agricultural nature of the catchment area of the Bnińskie and Skrzynki Duże lakes, increases in the total phosphorus value were observed at these points. The obtained results contradicted the hypothesis that with a longer residence time of the water in the lake the content of phosphorus and its forms in the water body would decrease as a result of the sorption and precipitation processes. It is possible that these results were strongly influenced by the catchment land usage. In the case of the two lakes with the longest water exchange period (Bnińskie (G4) and Skrzynki Duże (G6)), the proportion of agricultural land exceeded 75% of the catchment area. In the case of orthophosphate phosphorus, higher values were observed at sp G3, below Lake Jeziory Wielkie, which could have been caused by the inflow of treated sewage from the municipal sewage treatment plant. The positive impact of the lakes on the water quality of the Głuszynka river in terms of the P-PO4 content is confirmed by the parametric statistical change point analysis. The results of this analysis indicate significantly lower mean values of the orthophosphate phosphorus below sp G3. Similar results were obtained by Potasznik et al. (2014) in the Symsarna river catchment area, which confirmed the positive impact of lakes on the river quality in terms of total phosphorus content. As a result of the river flowing through lakes, the content of this element decreased by nearly 28%. At the same time, they observed that a decrease in the content of orthophosphate phosphorous occurred only during the growing period, when it was taken up by primary producers. Similar conclusions were reached by Sobczyńska-Wójcik and Rafałowska (2011), who conducted research on the water quality of the river-lake system of the Sętal river, where they observed a 36% reduction in total phosphorus content in the river. Lossow et al. (2006) analyzing the river-lake systems of the Marózka and Łyna rivers, also detected a beneficial effect of the lakes on river water quality, where the phosphorus load on the river was reduced by 10.1%. Similarly, Cook et al. (2010) in a study on the Australian river-lake system of the Murray river and Wang et al. (2020) on the Yuxi river observed the phenomenon of total phosphorus retention in lake waters, which resulted in a 48% reduction in the content of this element in river waters. The phenomenon of reduction of phosphorus content in river-lake systems after water flow through lakes may be related, according to Kanclerz et al. (2015) and Janicka (2020), to the precipitation of insoluble forms of phosphate salts. As suggested by Kanclerz et al. (2015) and Janicka (2020), accumulated phosphorus compounds in bottom sediments may be a secondary source of river water pollution under conditions favourable for P release from bottom sediments (low P-PO4 concentrations).
The PCA analysis, taking into account the catchment use of the lakes and the content of analyzed nutrient forms, confirmed previous assumptions about the significant role of catchment management on surface water quality. The projection of sampling points at the Głuszynka river (Figures 8A,B) indicated an influence of agricultural land (211, 242, 243) on the content of orthophosphate phosphorous and deciduous forests (311) on total phosphorus. The higher contents of total and P-PO4 were strongly related to two sampling points G1 and G4. Sp G1, located downstream of Lake Raczyńskie, with a typically agricultural character of the catchment and a high tourist load, was most strongly related to the content of total phosphorus in the water, whereas sampling point G4 located below the Lake Bnińskie, with a typically agricultural catchment area was mostly related to orthophosphate phosphorus.
Conclusion
The water quality of river-lake systems is the result of several factors, including the structure of the catchment use, the seasonality of anthropopressure factors (e.g., agricultural activity and recreation) and the activity of living organisms. The ecological status of the waters of the Głuszynka river were classified as “poor,” due to a high load of nutrients from the catchment area as arable land reaches the shoreline of the lakes, strong tourist and recreational pressure, and incomplete sewerage in communities. The statistical analyses carried out showed that catchment use affects the amount of nutrients. Arable land contributed to an increase in the content of orthophosphate phosphorus, while grassland contributed to total nitrogen, nitrate and nitrite nitrogen. The urbanized areas were responsible for inflows of ammonium nitrogen. The content of nitrogen and its mineral forms in the Głuszynka surface waters were also related to both the period of the year and the agricultural works carried out at that time. The highest values of these parameters were observed in the spring, before the intensive period of plant growth. Along with the intensification of the photosynthesis process, the content of nitrogen and its compounds in surface waters decreased. Only the content of orthophosphate phosphorus increased during the growing period, which could be related to intensive recreational use of the lakes of the analyzed system. Analyzing the influence of the lakes on the water quality of the Głuszynka river, their negative influence was observed in terms of total nitrogen and its mineral forms, which increased along the course of the river. However, in the case of total phosphorus and orthophosphate phosphorus a reduction in the values of these indicators was observed, which was probably related to the process of accumulation of phosphorus compounds in bottom sediments that took place in reservoirs. The parametric statistical change point analysis carried out showed that sp G4, located below Lake Bnińskie, was a critical point for the water quality of the Głuszynka river.
The quality of surface waters depends on many factors, but in the case of cascade river-lake systems the situation becomes even more complicated due to the possible influence of bottom sediments on the chemistry of flowing waters. Therefore, the possibility of improving surface water quality, as required by the Water Framework Directive, in such systems requires further research that additionally takes into account the chemistry of lake bottom sediments
Data Availability Statement
The original contributions presented in the study are included in the article/Supplementary Material, further inquiries can be directed to the corresponding author.
Author Contributions
EJ, JK and KW conceived and designed this study. EJ performed the field trip, sample analysis and data analysis. EJ drafted the original manuscript. JK and KW provided comments. JK and KW provided the very constructive suggestion and revision. AB helped with the statistical analysis.
Funding
The publication was co-financed/financed within the framework of Ministry of Science and Higher Education programme as “Regional Initiative Excellence” in years 2019–2022, Project No. 005/RID/2018/19.
Conflict of Interest
The authors declare that the research was conducted in the absence of any commercial or financial relationships that could be construed as a potential conflict of interest.
Publisher’s Note
All claims expressed in this article are solely those of the authors and do not necessarily represent those of their affiliated organizations, or those of the publisher, the editors, and the reviewers. Any product that may be evaluated in this article, or any claim that may be made by its manufacturer, is not guaranteed or endorsed by the publisher.
Acknowledgments
I would like to thank the Department of Land Improvement, Environmental Development and Spatial Management for the opportunity to conduct the research.
Supplementary Material
The Supplementary Material for this article can be found online at: https://www.frontiersin.org/articles/10.3389/fenvs.2022.874754/full#supplementary-material
References
Allan, J. D. (2004). Landscapes and Riverscapes: the Influence of Land Use on Stream Ecosystems. Annu. Rev. Ecol. Evol. Syst. 35, 257–284. doi:10.1146/annurev.ecolsys.35.120202.3000000
Andersen, J. M. (1994). Water Quality Management in the River Gudenaa, a Danish lake-stream-estuary System. Hydrobiologia 275-276 (1), 499–507. doi:10.1007/BF00026738
Bajkiewicz-Grabowska, E., Golus, W., Markowski, M., and Kwidzińska, M. (2020). The Role of Lakes in Shaping the Runoff of lakeland Rivers. Polish River Basins and Lakes–I 86. 175–187. doi:10.1007/978-3-030-12123-5_9
Bhat, S. A., Meraj, G., Yaseen, S., and Pandit, A. K. (2014). Statistical Assessment of Water Quality Parameters for Pollution Source Identification in Sukhnag Stream: an Inflow Stream of lake Wular (Ramsar Site), Kashmir Himalaya. J. Ecosyst. 2014, 1–18. doi:10.1155/2014/898054
Bruyninckx, H. (2020). Towards Zero Pollution in Europe. EEA. Copenhagen, Luxemburg. Copenhagen: Publications Office of the European Union. Available at: https://www.eea.europa.eu/signals/signals-2020-towards-zero-pollution (Accessed December 15, 2021).
Burzyńska, I. (2016). Evaluation of Selected Physical and Chemical Indicators in Raszynka River. Woda-Środowisko-Obszary Wiejskie. 16, 23–34.
Burzyńska, I. (2019). Monitoring of Selected Fertilizer Nutrients in Surface Waters and Soils of Agricultural Land in the River valley in Central Poland. J. Water land Dev. 43, 41–48. doi:10.2478/jwld-2019-0061
Causse, J., BaurèS, E., Méry, Y., Jung, A.-V., and Thomas, O. (2015). Variability of N export in Water: a Review. Crit. Rev. Environ. Sci. Techn. 45 (20), 2245–2281. doi:10.1080/10643389.2015.1010432
Chislock, M. F., Doster, E., Zitomer, R. A., and Wilson, A. E. (2013). Eutrophication: Causes, Consequences, and Controls in Aquatic Ecosystems. Nat. Edu. Knowledge 4, 10.
Choiński, A. (2006). “Catalog of Polish Lakes,” in Polish: Katalog Jezior Polski. Poland (Wydawnictwo Naukowe UAM Poznań).
CLC (2018). CORINE Land CoverEuropean Topic Centre on Urban, Land and Soil Systems. Wien: Publications of European Commision. Technical GuidesService Contract No 3436/R0-Copernicus/EEA.56665. Ausrtia. 2017 https://land.copernicus.eu/pan-european/corine-land-cover.
Cobb, G. W. (1978). The Problem of the Nile: Conditional Solution to a Changepoint Problem. Biometrika 65, 243–251. doi:10.1093/biomet/65.2.243
Cook, P. L. M., Aldridge, K. T., Lamontagne, S., and Brookes, J. D. (2010). Retention of Nitrogen, Phosphorus and Silicon in a Large Semi-arid Riverine lake System. Biogeochemistry 99, 49–63. doi:10.1007/s10533-009-9389-6
Dąbrowska, J. (2008). Evaluation of the Content of Nitrogen and Phosphorus Compounds in the Waters of Trzemna River. Infrastructure Ecol. Rural Areas 7, 57.
Djodjic, F., Bieroza, M., and Bergström, L. (2021). Land Use, Geology and Soil Properties Control Nutrient Concentrations in Headwater Streams. Sci. Total Environ. 772, 145108. doi:10.1016/j.scitotenv.2021.145108
Dojlido, J. R. (1995). “Chemistry of Surface Waters,” in Polish: Chemia Wód Powierzchniowych. Poland. Wydawnictwo Ekonomia I Środowisko (Białystok.
Elser, J. J., Bracken, M. E. S., Cleland, E. E., Gruner, D. S., Harpole, W. S., Hillebrand, H., et al. (2007). Global Analysis of Nitrogen and Phosphorus Limitation of Primary Producers in Freshwater, marine and Terrestrial Ecosystems. Ecol. Lett. 10, 1135–1142. doi:10.1111/j.1461-0248.2007.01113.x
EN ISO 6878 (2004). Water Quality — Determination of Phosphorus — Ammonium Molybdate Spectrometric Method.
Falniowski, A. (2003). “The Numerical Methods in Taxonomy,” in Polish: Metody Numeryczne W Taksonomii. Poland. Wydawnictwo Uniwersytetu Jagiellońskiego (Cracow.
Foley, J. A., DeFries, R., Asner, G. P., Barford, C., Bonan, G., Carpenter, S. R., et al. (2005). Global Consequences of Land Use. Science 309, 570–574. doi:10.1126/science.1111772
GIOŚ (2018). The State of the Environment in Poland. In Polish: Stan Środowiska W Polsce. Raport 2018Available at: https://www.gios.gov.pl/pl/stan-srodowiska/raporty-o-stanie-srodowiska (Accessed October 26, 2021).
Glińska-Lewczuk, K. (2006). Effect of Land Use and Lake Presence on Chemical Diversity of the Łyna River System. Polish J. Environ. Stud. 15, 259–269. doi:10.1007/s10661-016-5638-z
Grabinska, B., Koc, J., and Glinska-Lewczuk, K. (2004). “Impact of Land Use on the Water hazard by Phosphorus Compounds in the River Narew basin,” in Fertilisers and Fertilization In Polish (Puławy: Nawozy i Nawożenie), 6, 178.
Haynes, R. J., and Goh, K. M. (1978). Ammonium and Nitrate Nutrition of Plants. Biol. Rev. 53, 465–510. doi:10.1111/j.1469-185X.1978.tb00862.x
Hillbricht-Ilkowska, A. (1999). “Shallow Lakes in lowland River Systems: Role in Transport and Transformations of Nutrients and in Biological Diversity,” in Shallow Lakes’ (Dordrecht: Springer), 98143, 349–358. doi:10.1007/978-94-017-2986-4_39
Iital, A., Klõga, M., Pihlak, M., Pachel, K., Zahharov, A., and Loigu, E. (2014). Nitrogen Content and Trends in Agricultural Catchments in Estonia. Agric. Ecosyst. Environ. 198, 44–53. doi:10.1016/j.agee.2014.03.010
Janicka, E. (2020). The Role of Lakes in Shaping Water Quality in the River-lake System of the Kórnicko-Zaniemyska Gully. PhD Thesis. Poznań: University of Life Sciences Poznan. Available at: http://www1.up.poznan.pl/wisgp/wp-content/uploads/2020/10/Autoreferat-pr.-dokt.-E.-Janicka.pdf (Accessed December 5, 2021).
Journal of Laws 2021 item 1475 (2020). Rozporządzenie Ministra Infrastruktury Z Dnia 25 Czerwca 2021 R. W Sprawie Klasyfikacji Stanu Ekologicznego, Potencjału Ekologicznego I Stanu Chemicznego Oraz Sposobu Klasyfikacji Stanu Jednolitych Części Wód Powierzchniowych, a Także Środowiskowych Norm Jakości Dla Substancji Priorytetowych. (in polish)Available at: https://isap.sejm.gov.pl/isap.nsf/DocDetails.xsp?id=WDU20210001475 (Accessed December 5, 2021).
Kaczorowska, Z. (1962). “Precipitation in Poland in Long-Period Averages. Poland,” in Geographical Studies. Wydawnictwa Geologiczne (Warsaw: Polska Akademia Nauk).
Kanclerz, J., Wiatrowska, K., and Adamska, A. (2015). Phosphorous Concentration in Surface Water of Gorzuchowskie lake Catchment. Polish J. Agron. 22, 10
Killick, R., and Eckley, I. A. (2014). Changepoint: AnRPackage for Changepoint Analysis. J. Stat. Soft. 58 (3), 1–19. doi:10.18637/jss.v058.i03
Kneis, D., Knoesche, R., and Bronstert, A. (2006). Analysis and Simulation of Nutrient Retention and Management for a lowland River-lake System. Hydrol. Earth Syst. Sci. 10, 575–588. doi:10.5194/hess-10-575-2006
Kobus, J. (1996). “Role of Microorganisms in Nitrogen Transformation in Soil. In Polish: Rola Mikroorganizmów W Przemianach Azotu,” in Proceedings of Agricultural Sciences. In Polish: Zeszyty Problemowe Postępów Nauk Rolniczych, 440, 151–173.
Kristenen, P. (2012). European Waters – Assessment of Status and Pressures. Copenhagen, Luxemburg: Office for Official Publ. of the European UnionPublications Office of the European Union. doi:10.2800/63266
Kristensen, P., Whalley, C., Zal, F. N. N., and Christiansen, T. (2018). European Waters Assessment of Status and Pressures 2018. EEA Report. Available at: https://www.eea.europa.eu/publications/state-of-water (Accessed October 20, 2021).
Kuriata-Potasznik, A., Szymczyk, S., and Pilejczyk, D. (2018). Effect of Bottom Sediments on the Nutrient and Metal Concentration in Macrophytes of River-lake Systems. Ann. Limnol. - Int. J. Lim. 54, 1. doi:10.1051/limn/2017028
Kuriata-Potasznik, A., Szymczyk, S., and Skwierawski, A. (2020). Influence of Cascading River-Lake Systems on the Dynamics of Nutrient Circulation in Catchment Areas. Water 12, 1144. doi:10.3390/w12041144
Kuriata-Potasznik, A. (2018). The Functioning of a Water Body within a Fluvio-Lacustrine System as an Effect of Excessive Nitrogen Loading-The Case of Lake Symsar and its Drainage Area (Northeastern Poland). Water 10, 1163. doi:10.3390/w10091163
Kuss, J., Nausch, G., Engelke, C., Weber, M. V., Lutterbeck, H., Naumann, M., et al. (2020). Changes of Nutrient Concentrations in the Western Baltic Sea in the Transition between Inner Coastal Waters and the central Basins: Time Series from 1995 to 2016 with Source Analysis. Front. Earth Sci. 8, 106. doi:10.3389/feart.2020.00106
Li, Y., and Yao, J. (2015). Estimation of Transport Trajectory and Residence Time in Large River-Lake Systems: Application to Poyang Lake (China) Using a Combined Model Approach. Water 7, 5203–5223. doi:10.3390/w7105203
Lorenc, H. (2000). In Polish: Studia Nad 220-letnią (1779-1998) Serią Temperatury Powietrza W Warszawie Oraz Ocena Jej Wiekowych Tendencji. Poland: IMiGW.
Lossow, K., Gawrońska, H., Łopata, M., and Teodorowicz, M. (2006). Role of Lakes in Phosphorus and Nitrogen Transfer in the River-lake System of the Marózka and the Upper Łyna Rivers. Limnological Rev. 6, 171.
Matej-Lukowicz, K., Wojciechowska, E., Nawrot, N., and Dzierzbicka-Głowacka, L. A. (2020). Seasonal Contributions of Nutrients from Small Urban and Agricultural Watersheds in Northern Poland. PeerJ 8, e8381. doi:10.7717/peerj.8381
Mocek, A., Drzymała, S., and Maszner, P. (2006). Genesis, Analysis and Classification of Soils. Poland. Wydawnictwo Akademii Rolniczej imAugusta Cieszkowskiego. Poznań.
Morrison, D. F. (1990). “Multivariate Statistical Analysis,” in Polish: Wielowymiarowa Analiza Statystyczna. Poland (Warsaw: PWN).
Nausch, M., Woelk, J., Kahle, P., Nausch, G., Leipe, T., and Lennartz, B. (2017). Phosphorus Fractions in Discharges from Artificially Drained lowland Catchments (Warnow River, Baltic Sea). Agric. Water Manage. 187, 77–87. doi:10.1016/j.agwat.2017.03.006
Øygarden, L., Deelstra, J., Lagzdins, A., Bechmann, M., Greipsland, I., Kyllmar, K., et al. (2014). Climate Change and the Potential Effects on Runoff and Nitrogen Losses in the Nordic-Baltic Region. Agric. Ecosyst. Environ. 198, 114–126. doi:10.1016/j.agee.2014.06.025
Pietrzak, S., Pazikowska-Sapota, G., Dembska, G., Dzierzbicka-Glowacka, L. A., Juszkowska, D., Majewska, Z., et al. (2020). Risk of Phosphorus Losses in Surface Runoff from Agricultural Land in the Baltic Commune of Puck in the Light of Assessment Performed on the Basis of DPS Indicator. PeerJ 8, e8396. doi:10.7717/peerj.8396
PN-R-040032 Standard (1998). Soils and mineral Formations. Sampling and Determination of Granulometric Composition. Warszawa. Polish: PKN.
Pokojska, U., and Bednarek, R. (2021). “Landscape Geochemistry,” in In Polish: Geochemia Krajobrazu. Poland (Toruń: Wydawnictwo Naukowe UMK).
Potasznik, A., and Szymczyk, S. (2015). Magnesium and Calcium Concentrations in the Surface Water and Bottom Deposits of a River-lake System. J. Elementology 20 (3), 788. doi:10.5601/jelem.2015.20.1.78810.5601/jelem.2014.19.4.788
Potasznik, A., Szymczyk, S., Sidoruk, M., and Świtajska, I. J. (2014). Role of Lake Symsar in the Reduction of Phosphorus Concentration in Surface Runoff from Agricultural Lands. J. Water land Develop. 20, 39–44. doi:10.2478/jwld-2014-0006
Revenga, C., Brunner, J., Henninger, N., Kassem, K., and Payne, R. (2000). Freshwater Systems. Washington: DC: World Resources Institute.
Rosińska, J., and Gołdyn, R. (2018). Response of Vegetation to Growing Recreational Pressure in the Shallow Raczyńskie Lake. Knowl. Manag. Aquat. Ecosyst. 419, 1. doi:10.1051/kmae/2017050
Saunders, D. L., and Kalff, J. (2001). Nitrogen Retention in Wetlands, Lakes and Rivers. Hydrobiologia 443 (1), 205–212. doi:10.1023/A:1017506914063
Sobczyńska-Wójcik, K., and Rafałowska, M. (2011). Assessment of Restored Water Bodies in a River-Lake System Based on Phosphorus Concentrations. Ecol. Chem. Eng. A. 18, 1441.
Sobolewski, W., Borowiak, D., Borowiak, M., and Skowron, R. (2014). “The Database of Polish Lakes and its Use in Limnological Research,” in Polish: Baza Danych Jezior Polski I Jej Wykorzystanie W Badaniach Limnologicznych. Poland (Lublin: Maria Curie-Skłodowska University. Faculty of Earth Sciences and Spatial ManagementPicador Komunikacja Graficzna).
Stackpoole, S. M., Stets, E. G., and Sprague, L. A. (2019). Variable Impacts of Contemporary versus Legacy Agricultural Phosphorus on US River Water Quality. Proc. Natl. Acad. Sci. U.S.A. 116, 20562–20567. doi:10.1073/pnas.1903226116
Sun, X., Bernard-Jannin, L., Grusson, Y., Sauvage, S., Arnold, J., Srinivasan, R., et al. (2018). Using SWAT-LUD Model to Estimate the Influence of Water Exchange and Shallow Aquifer Denitrification on Water and Nitrate Flux. Water 10, 528. doi:10.3390/w10040528
Tan, X., Zhang, Q., Burford, M. A., Sheldon, F., and Bunn, S. E. (2017). Benthic Diatom Based Indices for Water Quality Assessment in Two Subtropical Streams. Front. Microbiol. 8, 601. doi:10.3389/fmicb.2017.00601
Tao, W., Niu, L., Dong, Y., Fu, T., and Lou, Q. (2021). Nutrient Pollution and its Dynamic Source-Sink Pattern in the Pearl River Estuary (South China). Front. Mar. Sci. 8, 13907. doi:10.3389/fmars.2021.713907
The Local Data Bank (2020). Database: The Local Data Bank, Central Statistical Office in Warszawa. Available at: https://bdl.stat.gov.pl/BDL/dane/teryt/jednostka# (Accessed December 5, 2021).
Tomczyk, A. M., and Szyga-Pluta, K. (2016). Growing Seasons in Poland in the Period 1971-2010. Prz. Geogr. 88, 75–86. doi:10.7163/PrzG.2016.1.4
Tong, Y., Li, J., Qi, M., Zhang, X., Wang, M., Liu, X., et al. (2019). Impacts of Water Residence Time on Nitrogen Budget of Lakes and Reservoirs. Sci. Total Environ. 646, 75–83. doi:10.1016/j.scitotenv.2018.07.255
Uuemaa, E., Palliser, C., Hughes, A., and Tanner, C. (2018). Effectiveness of a Natural Headwater Wetland for Reducing Agricultural Nitrogen Loads. Water 10, 287. doi:10.3390/w10030287
Vagstad, N., Stålnacke, P., Andersen, H.-E., Deelstra, J., Jansons, V., Kyllmar, K., et al. (2004). Regional Variations in Diffuse Nitrogen Losses from Agriculture in the Nordic and Baltic Regions. Hydrol. Earth Syst. Sci. 8, 651–662. doi:10.5194/hess-8-651-2004
Van Loon, G. W., and Duffy, S. J. (2007). “Environmental Chemistry,” in In Polish: Chemia Środowiskowa, Poland. Wyd (Warsaw: Naukowe PWN).
Varanka, S., and Luoto, M. (2012). Environmental Determinants of Water Quality in Boreal Rivers Based on Partitioning Methods. River Res. Applic. 28, 1034–1046. doi:10.1002/rra.1502
Wang, Y., Kong, X., Peng, Z., Zhang, H., Liu, G., Hu, W., et al. (2020). Retention of Nitrogen and Phosphorus in Lake Chaohu, China: Implications for Eutrophication Management. Environ. Sci. Pollut. Res. 27, 41488–41502. doi:10.1007/s11356-020-10024-7
Wiatrowska, K., and Komisarek, J. (2015). Phosphorous Sorption in Soil Catena of Luvisols and Pheozems of the Poznań Lakeland. Polish J. Agron. 22, 25.
WMO (2009). Guidelines on Analysis of Extremes in a Changing Climate in Support of Informed Decisions for Adaptation. Geneva: World Meteorological Organization. WCDMP-No. 72 Available at: https://www.ecad.eu/documents/WCDMP_72_TD_1500_en_1.pdf# (Accessed October 25, 2018).
Woli, K. P., Hayakawa, A., Kuramochi, K., and Hatano, R. (2008). Assessment of River Water Quality during Snowmelt and Base Flow Periods in Two Catchment Areas with Different Land Use. Environ. Monit. Assess. 137, 251–260. doi:10.1007/s10661-007-9757-4
Yang, G., Zhang, Q., Wan, R., Lai, X., Jiang, X., Li, L., et al. (2016). Lake Hydrology, Water Quality and Ecology Impacts of Altered River-lake Interactions: Advances in Research on the Middle Yangtze River. Hydrol. Res. 47, 1–7. doi:10.2166/nh.2016.003
Keywords: river-lake system, nitrogen, phosphorus, water framework directive, eutrophication, river-lake interconnection, hydro-environmental
Citation: Janicka E, Kanclerz J, Wiatrowska K and Budka A (2022) Variability of Nitrogen and Phosphorus Content and Their Forms in Waters of a River-Lake System. Front. Environ. Sci. 10:874754. doi: 10.3389/fenvs.2022.874754
Received: 12 February 2022; Accepted: 28 March 2022;
Published: 21 April 2022.
Edited by:
Jiangyu Dai, Nanjing Hydraulic Research Institute, ChinaCopyright © 2022 Janicka, Kanclerz, Wiatrowska and Budka. This is an open-access article distributed under the terms of the Creative Commons Attribution License (CC BY). The use, distribution or reproduction in other forums is permitted, provided the original author(s) and the copyright owner(s) are credited and that the original publication in this journal is cited, in accordance with accepted academic practice. No use, distribution or reproduction is permitted which does not comply with these terms.
*Correspondence: Ewelina Janicka, ewelina.janicka@up.poznan.pl