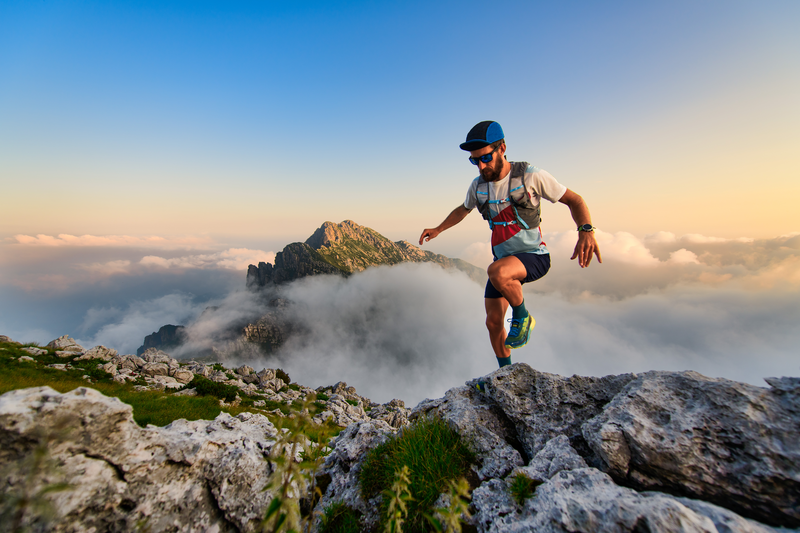
95% of researchers rate our articles as excellent or good
Learn more about the work of our research integrity team to safeguard the quality of each article we publish.
Find out more
ORIGINAL RESEARCH article
Front. Environ. Sci. , 14 June 2022
Sec. Soil Processes
Volume 10 - 2022 | https://doi.org/10.3389/fenvs.2022.872898
This article is part of the Research Topic Analysis, Fate and Transformation of Emerging Contaminants in Soil and Sediment View all 7 articles
Agricultural land soils have become a source and sink for microplastics. Due to the low recycling rate, long durability, and small size, microplastics pose a potential risk to soil fauna, which are critical for maintaining healthy soil. However, whether and how would microplastics affect soil biodiversity and ecological functioning is not well-understood. Soil nematodes are valuable indicators of the soil food web. In the present study, the abundance, diversity, community composition, maturity indices, soil food web indices, and metabolic footprints of soil nematodes in bulk soils of maize were utilized to indicate the potential impacts of polypropylene (PP) microplastic pollution on soil fauna using a soil-incubation experiment in a climate-controlled chamber with four concentration levels of microplastic pellets (0%, 0.5%, 1%, and 2%, w/w) added to loess soil collected from the Loess Plateau in China. Soil sampling was conducted at the fully ripe stage of maize. Twenty-nine genera of nematodes, including thirteen genera of plant-feeding nematodes, seven genera of bacterial-feeding nematodes, five genera of fungal-feeding nematodes, and four genera of omnivorous nematodes were recovered from soil samples. Microplastic concentration negatively affected the abundance, diversity (including genus richness, Margalef’s richness, Shannon–Wiener index, and Simpson’s dominance index), sigma maturity index (∑MI), structural index, and metabolic footprints. The abundances of plant parasites, bacterivores, fungivores, and omnivores in 2% soils were reduced by 90.16%, 76.06%, 82.35%, and 100%, respectively, in comparison with those of control. The major drivers of soil nematode communities in bulk soils of maize at a depth range of 0–20 cm were the soil pH, soil organic carbon content, C/N, and TP content. In conclusion, the addition of 200 μm-sized PP microplastic pellets negatively affected the soil nematode community and associated ecological functioning under greenhouse conditions.
The widespread use of plastic and inappropriate disposal of plastics disseminates plastic debris into the environment (Martínez-Campos et al., 2022). Microplastics (particle size <5 mm), originating from the degradation of plastics through weathering and other disintegration processes, have become emerging contamination in soils worldwide (Kaile Zhang et al., 2022; Kim et al., 2021; Lin et al., 2021). Agricultural soils are expected to be a source and sink of microplastics (Qi et al., 2018; Weithmann et al., 2018) due to the application of sewage sludge and wastewater, plastic mulching, littering, the input of tire wear from roads, and atmospheric deposition (Bläsing and Amelung, 2018; Hurley and Nizzetto, 2018; Liu et al., 2018; Büks and Kaupenjohann, 2020). Studies have documented that microplastics in soils can reduce rooting ability, suppress photosynthesis, and inhibit the growth of plants (Qi et al., 2018; Boots et al., 2019; de Souza Machado et al., 2019; Rillig et al., 2019; Wang et al., 2020; Yin et al., 2021; Huang et al., 2022) as well as change soil physicochemical properties and soil microbial community composition (de Souza Machado et al., 2018; Sun et al., 2022; Wang et al., 2022).
Microplastics are likely to affect soil fauna through several pathways. First, microplastics can easily be ingested by soil-living organisms, negatively affect growth, and disrupt the proliferation and function of decomposers (Okeke et al., 2022). Second, some microplastic types could release toxic plastic additives under certain soil environment conditions during their degradation (Zhang et al., 2020). Third, microplastics may serve as a vector of pathogenic bacteria (Martínez-Campos et al., 2022) and fungi as well as antibiotic-resistant bacteria (Parthasarathy et al., 2019). Furthermore, microplastics can be carriers of pesticides (Rodríguez-Seijo et al., 2018). Finally, microplastics represent fossil carbon, which is independent of photosynthesis and net primary production (Rillig and Lehmann, 2020). It is possible that microplastic inputs will affect soil carbon content and soil organisms closely correlated to the soil organic carbon (SOC) indirectly. Since microplastics can reduce habitat quality (Selonen et al., 2020), movement (Kim and An, 2019), and the potential prey (including plant roots, root exudates, litters, and soil microbes) of soil animals, it is likely to exert indirect effects on soil animals, which are critical for delivering multiple ecological functions and services. Although whelming studies have addressed the impacts of microplastic pollution on soil microbial community and the fitness of soil animals, only a few studies (e.g., Lin et al., 2021; Selonen et al., 2020) have examined the ecological risks of microplastic pollution on the diversity, community structure, and ecological functioning of soil animal, especially those that occupy several trophic groups.
Nematodes, the most abundant animals on Earth, fill all trophic levels in the soil food webs (van den Hoogen et al., 2019). Additionally, nematodes occupy a key position in the complex soil food webs (Moore et al., 2003), interact with other organisms, and play a more crucial role in ecological functioning in comparison with other soil animals (Forge et al., 2005). Previous studies also proposed that changes in one or several groups of soil organisms are likely to affect the abundance, diversity, and functioning of other groups in the food webs (Hedlund and Ӧhrn, 2000; Lenoir et al., 2006; Warde, 2006; Crowther et al., 2013; Lin et al., 2021). Furthermore, nematodes are responsive to environmental change and each sample provides high intrinsic information value. In addition, there are standard extraction, identification, and community analysis procedures for the nematode. Finally, a clear correlation between the structure and function has been demonstrated. Therefore, it has been widely used as an indicator of soil health. To date, the impacts of microplastic pollution on the nematode community have been evaluated in aquatic ecosystems (Wakkaf et al., 2020; Allouche et al., 2022; Rauchschwalbe et al., 2022; Stanković et al., 2022), while those in farmland ecosystems are scarce.
The major objectives of the present study are as follows: 1) whether and how microplastic concentration would affect the soil nematode community? 2) What are the drivers of variation in soil nematode assembly in bulk soils of maize? We hypothesized that microplastic addition would negatively affect soil nematodes since previous studies reported detrimental effects of microplastics on nematodes (Zhao et al., 2017; Mueller et al., 2020a; Lin et al., 2021; Schöpfer et al., 2020; Chen et al., 2021). As the ingestion of microplastics by nematodes depends on feeding strategy and buccal cavity size (Fueser et al., 2019; Mueller et al., 2020b), we further assumed that the impacts of microplastic addition on soil nematodes varied with trophic groups.
It has been reported that 50.51% of micro (meso)-plastics in farmland soils were polypropylene (PP) (Liu et al., 2018). Furthermore, about 82% of microplastics found in soils had sizes <250 µm (Büks and Kaupenjohann, 2020), and only PP microplastics with a particle size smaller than 250 μm decrease nematode offspring (Kim et al., 2020b). Therefore, we selected PP pellets with a particle size of about 200 μm, which were purchased from the Youngling-TECH company, Beijing, China. As described by Liu et al. (2017), the density of the PP microplastic is 0.91 g/cm3, and its melting temperature and brittle temperature are 164–170°C and −35°C, respectively. The experimental soil was collected from the farmland in Ansai County, Shaanxi Province, China. The soil type of this area is sandy soil developed from the Loess parent material, with a SOC concentration of 2.68 g/kg, total nitrogen (TN) concentration of 0.13 g/kg, Olsen phosphorus (TP) concentration of 0.47 g/kg, available phosphorus (AP) concentration of 6.27 mg/kg, ammonia nitrogen (NH4+-N) concentration of 4.93 mg/kg, nitrate-nitrogen (NO3-N) concentration of 18.25 mg/kg, and pH of 8.65 (H2O/soil at 1:2.5 weight/volume, w/v). Before use, the air-dried soil was sieved through a 5 mm steel sieve. The cultivar of maize was Huanong 887, which was provided by Yangling Seed Company.
This experiment was conducted in the climate-controlled chamber (AGC-Doo3N, Hangzhou, China) at the Institute of Soil and Water Conservation, Chinese Academy of Sciences, Yangling, Shaanxi province, China from July to October 2019. As reported, microplastic concentrations in soil samples collected from the industrial area were 300–67,500 mg/kg, that is 0.03%–6.7% (Fuller and Gautam, 2016). Meanwhile, a field survey reported that the concentration of plastic residues in Chinese Loess soil is 1% (w/w) (Chen, 2016). Taking the newly inputs of microplastics into soils as well as the great effort to reduce microplastic contamination into consideration, we set up the following four treatments: 1) CK, no microplastics added to the soil; 2) C1, 75 g of microplastics added to the soil (0.5%, w/w); 3) C2, 150 g of microplastics added to the soil (1%, w/w), and 4) C3, 300 g of microplastics added to the soil (2%, w/w). Each treatment had six replicates: 15 kg of soil and microplastics were thoroughly mixed before they were put into a PVC bucket with an upper diameter of 30 cm, a lower diameter of 22 cm, and a height of 30 cm. The pots were incubated in the light at 28°C (relative humidity of 80%, 300 m (photons) m−2 s−1). To avoid potential impacts of drought or water-logging stress on plant and soil biota, soil moisture was maintained at 65 ± 5% throughout the experiment. Crop diseases and insect pests were controlled according to field management measures in this region during the experiment.
During the fully ripe stage of maize, more accurately on October 22–24, 2019, the bulk soils from the topsoil layer (0–20 cm) of each pot were collected. All collected soil samples were sieved with 4 mm and mixed thoroughly, resulting in 24 composite samples. These samples were stored at 4°C before further processing.
Soil nematodes were extracted from 100 g of field-moist soil within 14 days after sampling. In brief, 100 g of soil were mixed with 200 ml of water in a 500 ml beaker and allowed to stand for 1 min. Then, the mixture was mixed well and sieved through 20/500-mesh stacked sieves to concentrate nematodes on a 500-mesh sieve. After replicating the same procedure three times, the materials on the 20-mesh sieve were discarded and those on the 500-mesh sieve were washed into a 100 ml centrifuge tube. Finally, nematodes were further separated from soils using the sucrose centrifugation method (Jenkins, 1964). The recovered nematodes were killed by heating at 60°C for 3 min and were preserved in 4% formalin solution before further analysis. The nematode individuals per sample were counted under a dissecting microscope, and the first 150 nematodes encountered in the sample were identified to the genus level where possible according to the external morphological features of the body shape, cuticle, head, papilla, and tail as well as the internal structures of the digestive system, reproductive system, excretory system and nervous system as described by Bongers (1994), Ahmad and Jairajpuri (2010) as well as Interactive Diagnostic Key to Plant Parasitic, Free-living, and Predaceous Nematodes (http://nematode.unl.edu/nemakey.htm) at a 400× or 1,000× magnification using an inverted compound microscope. If the individual recovered from a sample was less than 150, all specimens were identified. Nematode population was expressed as the individuals per 100 g of dry soil after being adjusted with soil moisture. Soil nematode genera were classified into bacterivores, fungivores, plant parasites, and omnivores–predators (Yeates et al., 1993) according to their feeding habits and assigned a c-p value (Bongers, 1990; Bongers and Bongers, 1998). The density of an individual trophic group was calculated.
Since soil biodiversity and community composition determine ecological functioning (Wagg et al., 2014), we examine the responses of soil nematode abundance, diversity, and composition to microplastic pollution first. Biodiversity indices, including taxa richness (S), Margalef’s richness (SR), Shannon–Wiener index (H′), Simpson’s index (λ), and Pielou’s evenness index (J′), were calculated based on the richness and relative abundance data. Subsequently, we compare the maturity indices (Bongers, 1990; Freckman and Ettema 1993), food web conditions (Ferris et al., 2001), and metabolic footprints (Ferris, 2010) across treatments. The maturity indices, food web conditions, and metabolic footprints were calculated by submitting the soil nematode array to NINJA (Nematode INdicator Joint Analysis) (Sieriebriennikov et al., 2014), provided at https://shiny.wur.nl/ninja.
The physicochemical properties of the soil, such as soil moisture, pH, SOC, TN, NH4+-N, NO3-N, TP, and AP, were determined using standard methods as described elsewhere. In brief, the soil moisture was calculated as the weight lost when baking a sample to a constant weight at 105 ± 2°C for 10 h, and the soil pH was estimated using an electronic pH meter fitted with a glass electrode (WTW pH 330, WTW, Weilheim, Germany) in soil: the water solution at a ratio of 1:2.5 (weight/volume, w/v), the SOC content was analyzed by the potassium-dichromate (K2Cr2O7) oxidation method (Walkley and Black, 1934), TN was determined by the Kjeldahl method (Boström et al., 1974), the NH4+-N content and NO3-N content were extracted with 2 M KCl and were measured using an AA3 continuous flow auto-analyzer (AutoAnalyzer 3-aa3, Bran+ Luebbe, Germany), the total P content of the soil was determined using colorimetric analysis after digestion with H2SO4 and HClO4 (Olsen et al., 1982; Wu et al., 2021) and the AP content was extracted with 0.5 M NaHCO3 at pH 8.5 and measured colorimetrically using a UV spectrophotometer and the ammonium molybdate method (Wu et al., 2021).
The effects of microplastic concentration on total nematode abundance, abundance per trophic group, nematode diversity indices, maturity indices, food web indices, and metabolic footprints of soil nematodes were analyzed using the linear mixed model with the dose as the fixed factor and pot as the random factor using the “nlme” package in R (R Core Development Team, 2018). Before analysis, the normality and the homogeneity of the residuals for data were examined by the Shapiro–Wilk test or by the Kolmogorov–Smirnov test in the “stats” package. If the data do not meet the assumption of the normality and the homogeneity of the variations, the effect of microplastic additions on a given variable was examined with the Kruskal–Wallis test, and differences across microplastics concentrations were checked and versioned by the “boxplerk”. Correlations between nematode community parameters and environmental factors were analyzed by the “cor.test” function. Furthermore, taxonomic community composition differences were examined by non-metric multidimensional scaling (NMDS) based on the Bray–Curtis distances. To show the reliability of the results, non-parametric multivariate statistical tests, including ANOSIM, Adonis, and MRPP, based on different algorithms were performed simultaneously to examine statistical significance using the “vegan” package. The major environmental factors shaping soil nematode community were selected with “envfit” function in the “vegan” package. In addition, the association between individual nematode abundance and microplastic contamination was examined with the “Indicspecies” package. Finally, the direct and indirect effects of microplastics on soil nematode community were analyzed using the “PLSPM” package. All the aforementioned analyses were performed with R software (version 4.1.2.; R foundation for statistical computing).
The abundance of total nematodes at a depth of 0–20 cm of bulk soil ranged from 20 to 108 nematode specimens/100 g dry soil. The abundance of total nematodes declined significantly with the increase in the microplastic concentration (Kruskal–Wallis test: χ2 = 12.76, p = 0.005; Figure 1A). The increase in the microplastic concentration exerted significant negative effects on the abundances of nematode trophic groups and free-living nematodes (Figures 1B,C,E,F), with exception of bacterivores (Kruskal–Wallis test: χ2 = 12.76, p = 0.05; Figure 1D). The correlation analysis suggested that nematode abundances were correlated to the SOC content, TP content, and C/N of soils (Supplementary Figure S1). Specifically, nematode abundances were positively correlated to the soil TP content, whereas negatively correlated to the SOC content and C/N. PLS modeling indicated that the direct impact of microplastics on nematode abundance is more significant than the indirect one (Supplementary Figure S3A).
FIGURE 1. Abundances (specimens/100 g dry soil) of total nematodes (A), plant-feeding nematodes (B), free-living nematodes (C), bacterial-feeding nematodes (D), fungal-feeding nematodes, (E) and ominous–carnivorous nematodes (F) at the depths of 0–20 cm soils exposed to varying magnitudes of microplastic pollution. The box-plot shows the min, lower quartile, median, mean, and upper quartile values, and the whiskers show the range of the variation. Different lowercase letters show a significant difference (p < 0.05) across treatments. CK: no microplastics added to the soil; C1:75 g of microplastics added to the soil (0.5%, w/w); C2: 150 g of microplastics added to the soil (1%, w/w), and C3: 300 g of microplastics added to the soil (2%, w/w).
Twenty-nine genera of nematodes including thirteen plant-feeding nematodes, seven bacterial-feeding nematodes, five fungal-feeding nematodes, and four omnivorous nematodes were recovered from the soil samples. The number of nematode genera ranged from six to nineteen and decreased significantly with the increase in the exposure dose of microplastics (Kruskal–Wallis test: χ2 = 16.35, df = 3, p < 0.001; Figures 2, 3A). Moreover, the addition of microplastics greatly impacted other diversity indices [including SR (Kruskal–Wallis test: χ2 = 12.55, df = 3, p < 0.001; Figure 3B), H' (one-way ANOVA: F = 11.00, df = 3, p = 0.0002; Figure 3C), and λ (Kruskal–Wallis test: χ2 = 12.89, df = 3, p < 0.005; Figure 3D)] of soil nematode community. However, the addition of microplastics did not affect the J′ of the soil nematode community (Kruskal–Wallis test: χ2 = 4.71, df = 3, p = 0.19; Figure 3E). Further analysis suggested that nematode community diversity was correlated to the SOC content, TP content, C/N, and C/P of soils (Supplementary Figure S2). The PLS path modeling confirms that PP concentrations affect soil nematode communities both directly and indirectly (Supplementary Figure S3B).
FIGURE 2. Venn diagram displaying the similarities in soil nematode genera exposed to varying magnitudes of microplastic pollution. CK: no microplastic added to the soil; C1:75 g of microplastics added to the soil (0.5%, w/w); C2: 150 g microplastics added to the soil (1%, w/w), and C3: 300 g of microplastics added to the soil (2%, w/w).
FIGURE 3. Diversity indices of soil nematode assemblages exposed to varying magnitudes of microplastic pollution. The box-plot shows the min, lower quartile, median, mean, and upper quartile values, and the whiskers show the range of the variation. Different lowercase letters show a significant difference (p < 0.05) across treatments. CK: no microplastics added to the soil; C1:75 g of microplastics added to the soil (0.5%, w/w); C2: 150 g of microplastics added to the soil (1%, w/w), and C3: 300 g of microplastics added to the soil (2%, w/w).
The NMDS plot shows that soil nematode assemblages across the examined microplastic application doses cannot be separated from each other (Figure 4, stress = 0.20, R = 0.45, and p < 0.01). Additionally, the results of ANOSIM, Adonis, and MRPP confirmed that the differences in soil nematode communities across examined microplastic application doses were significant (Table 1), indicating that the microplastic exposure dose exerted significant impacts on the nematode community composition. Indicator species analysis (Supplementary Table SA2) revealed that the Aporcelaimus, Longidorus, and Thonus were abundant in soils of control and the Plectus was more abundant in soils of C1 treatment. The Rotylenchus, Helicotylenchus, and Microdorylaimus were observed in soils of CK and C1 treatments, Scutellonema were more abundant in soils of C1 and C2 treatment, and a few Cephalobus were observed in soils exposed to microplastic pollution. The “Envfit” showed that the environmental factors shaping soil nematode communities in bulk soils of maize at a depth of 0–20 cm were the soil pH, SOC content, C/N, and TP content (Table 2).
FIGURE 4. Non-metric multidimensional scaling plot of soil nematode community assemblage exposed to varying magnitudes of microplastic pollution. CK: no microplastics added to the soil; C1:75 g of microplastics added to the soil (0.5%, w/w); C2: 150 g of microplastics added to the soil (1%, w/w), and C3: 300 g of microplastics added to the soil (2%, w/w).
TABLE 1. Significance tests of the impacts of microplastic concentration on the nematode community composition in top-layer soils with three non-parametric statistical analyses (n = 6).
TABLE 2. Environmental factors that are closely correlated to the ordination of NMDS based on the “envfit” analysis.
The examined microplastic concentration negatively affected ∑MI (Kruskal–Wallis test: χ2 = 14.946, df = 3, and p < 0.002) and SI (Kruskal–Wallis test: χ2 = 17.734, df = 3, and p < 0.0005) of the soil nematode community, whereas it did not affect PPI (Kruskal–Wallis test: χ2 = 0.576, df = 3, and p = 0.90) and EI of the soil nematode community (one-way ANOVA: F = 0.783, df = 3, and p = 0.517). Further analysis suggested the ∑MI and SI of the soil nematode community were positively correlated to the soil TP content, while they were negatively correlated to SOC content (Supplementary Figure S2).
The examined microplastic concentration negatively affected the composite footprint (Kruskal–Wallis test: χ2 = 16.72, df = 3, and p = 0.0008; Figure 5A), enrichment footprint (Kruskal–Wallis test: χ2 = 10.85, df = 3, and p = 0.01; Figure 5B), structure footprint (Kruskal–Wallis test: χ2 = 18.21, df = 3, and p = 0.0004; Figure 5C), herbivore (plant-feeding nematode) footprint (Kruskal–Wallis test: χ2 = 18.73, df = 3, and p = 0.0003; Figure 5D), bacterivore footprint (Kruskal–Wallis test: χ2 = 9.61, df = 3, and p = 0.02; Figure 5E), fungivore footprint (Kruskal–Wallis test: χ2 = 10.85, df = 3, and p = 0.01; Figure 5F), and omnivore footprint (Kruskal–Wallis test: χ2 = 18.35, df = 3, and p = 0.0004; Figure 5G). Further analysis suggested that nematode metabolic footprints were correlated to the SOC content, TP content, C/N and C/P of soils (Supplementary Figure S4).
FIGURE 5. Metabolic footprints of soil nematode assemblages exposed to varying magnitudes of microplastic pollution. The box-plot shows the min, lower quartile, median, mean, and upper quartile values, and the whiskers show the range of the variation. Different lowercase letters show a significant difference (p < 0.05) across treatments. CK: no microplastics added to the soil; C1:75 g of microplastics added to the soil (0.5%, w/w); C2: 150 g of microplastics added to the soil (1%, w/w), and C3: 300 g of microplastics added to the soil (2%, w/w).
The increasing microplastic contamination has been considered an emerging threat to biodiversity and ecosystem functioning (Boots et al., 2019). Since the fate of microplastics is closely correlated to the soil physicochemical properties and biota, investigating the potential toxicity of microplastics on soil organisms is of critical importance (He et al., 2018). However, the impacts of microplastic pollution on biodiversity and the functions of soil fauna in agricultural soils remain largely unknown. An earlier study has demonstrated that soil nematodes can serve as bioindicators of environmental pollution and especially MP pollution (Mueller et al., 2020a). The available studies focused on the impacts of microplastic on the fitness (including growth, reproduction, and survival) of a bacterivorous nematode, namely Caenorhabditis elegans (Zhao et al., 2017; Dong et al., 2018; Kim et al., 2019, 2020 a, 2020b; Lei et al., 2018a, 2018b; Qu et al., 2018; Mueller et al., 2020a, 2020b; Schöpfer et al., 2020; Shang et al., 2020), and the results showed that the inhibitory effects of microplastics on nematode are size- and composition-dependent (Kim et al., 2019, 2020a, 2020b; Lei et al., 2018a, 2018b). Therefore, there is a great need to thoroughly evaluate the potential risks of microplastics on soil nematode diversity and ecological functioning in soils. In the present study, we assessed the impacts of microplastic contamination on biodiversity and the ecological functioning of soil nematode communities during the maize growing season by manipulating PP concentration. Three pieces of evidence support that the 200 μm PP microplastics exert a concentration-dependent risk on soil nematode assemblage.
First, we observed that microplastic addition greatly reduced the abundances of total nematodes and trophic groups (with exception of bacterivores) (Figure 1). Our study combined with the previous research under field conditions (Lin et al., 2021) indicates that the impacts of microplastics on nematode abundance in soils are concentration-dependent and trophic group-specific. This fully supported our first hypothesis and partially supported our second hypothesis. As the density of plant parasites reduced due to the addition of microplastics, a likely reason would be the reduced root biomass of maize. An earlier study has reported that PLA reduced the biomass of Zea mays Linn (Wang et al., 2020). Regarding the neutral effect of microplastics’ addition on bacterivores abundance, one plausible explanation is that an indirect positive effect on bacterivorous nematode due to an increase in carbon input modulates the direct toxic effect of microplastics. We indeed observed a remarkable increase in the SOC with the microplastic addition treatments, but we also observed a negative correlation between the carbon quantity and quality (including SOC, C/N, and C/P) and nematode community parameters (Supplementary Figures S1–3). Although the correlations between SOC and nematode abundance are generally positive, a negative correlation was also reported (Su et al., 2012). Regarding the observed negative correlation between SOC and nematode metrics in the present study, we should be cautious because microplastic addition would disrupt accurate SOC determination based on the chemical oxidation method (Kim et al., 2021). The potential link between changes in the SOC content and nematode abundance resulting from microplastic addition should be investigated quantitatively in future studies using other SOC determination methods.
Additionally, microplastic pollution results in a decline in the nematode genera richness and diversity indices (Figure 2). Previous studies have demonstrated that a more soil diversity fosters more robust and complex soil food webs to control PPN through enhanced top-down regulation (Delgado-Baquerizo et al., 2017; Zhiqin Zhang et al., 2022) and also increases the likelihood that plants develop strong relationships with beneficial soil communities that could inhibit the attack of herbivores or pathogens (van der Heijden et al., 2008). We also observed lower MI and SI in soils with higher microplastic additions, indicating a disturbed soil nematode community. Our finding is in agreement with the proposal that disturbance generally leads to a lower MI (Bongers, 1990). In line with the similar findings that the effects of low-density polyethylene on soil nematode community (Lin et al., 2021) varied with microplastic concentrations, we observed striking nematode community assemblages across the examined microplastic concentrations (Figures 3, 4). The soil pH, SOC content, C/N, and TP content were the most important factors contributing to variation in the soil nematode community structure in bulk soils of maize (Table 2).
Finally, the addition of microplastics strongly impacted the metabolic footprints of nematodes (Figure 5). Nematode metabolic footprints are valuable indicators of ecosystem services provided by various functional groups of nematodes, indicating the biomass, metabolic activity, and magnitude of carbon and energy flow driven by different nematode groups in soil food webs (Ferris, 2010; Ferris et al., 2012). In the present study, the microplastic concentration reduced the herbivore footprint, bacterivore footprint, and fungivore footprint (Figure 5), indicating that microplastic addition greatly impacted the nutrient cycling and energy flow driven by soil nematodes. This corroborates an earlier report that microplastics have a potentially negative effect on soil biogeochemical cycles (Schöpfer et al., 2020).
The present study has some limitations, and improvements are greatly needed in the future. First, the observed effects of microplastics on the soil nematode community are based on one sampling event, and thus, more sampling events during the maize growing season may be needed to confirm our findings. Second, the present study only demonstrated the responses of the soil nematode community to approximately 200 μm-sized microplastic pellets in soils of lower fertility. Further associated experiments should take the effects of microplastic type, size and concentration, soil fertility, and plant species into consideration. Particularly, field experiments using realistic concentration and proportion of major microplastic types in agricultural soils are urgently needed. Third, the ecological risks of the microplastic mixture on soil nematode community should be included in our following studies. Furthermore, we should use metal or glass for testing soils to avoid potential transfer contamination in the future. Finally, further study should include more than two organisms to generate a deeper understanding of the ecological risks of microplastic pollution on soil biota. After all, the interactions among soil organisms play a crucial role in fundamental biogeochemical processes.
In conclusion, the addition of 200 μm-sized PP microplastic pellets negatively affected the soil nematode community and associated ecological functioning under greenhouse conditions. This study gives an insight into the status of soil nematodes and the magnitude of associated ecosystem services in agricultural ecosystems exposed to various magnitudes of microplastic pollution under greenhouse conditions. We observed lower abundance, diversity and metabolic footprint, simplified community structure, and poor soil food web conditions in soils with a higher microplastic concentration. Simultaneously, microplastic pollution-induced distinct soil nematode assemblages, soil pH, SOC content, C/N, and TP content account for the variations in nematode community structure in bulk soils of maize. Further work needs to address the combined effects of the microplastic type, size, and concentration on the soil nematode community across soil types under field conditions to gain an in-depth understanding of the impacts of microplastic containment on the soil nematode community structure and ecosystem functions.
The original contributions presented in the study are included in the article/Supplementary Material; Data are available from the corresponding author upon reasonable request.
BY, PL, WE, ZG, and SX contributed conception and design of the study. PL and WE took charge of the daily management of pot experiments and soil sampling. PL, WE, and ZG performed the soil physicochemical assay, SX conducted the data evaluation and statistics supported by PL, WE, and ZG. BY extracted and identified soil nematodes, conducted statistical analyses, and wrote the first draft of the manuscript. All authors contributed to manuscript revision, and approved the submitted version.
Financial support was jointly provided by an open research grant (A314021402-2006) from the State Key Laboratory of Soil Erosion and Dryland Farming on the Loess Plateau, Institute of Soil and Water Conservation, Chinese Academy of Sciences, and a Key Research and Development project (2021YFS0295) from Sichuan Sciences and Technology, and the Shaanxi Science fund for Distinguished Young Scholars (2021JC-50).
The authors declare that the research was conducted in the absence of any commercial or financial relationships that could be construed as a potential conflict of interest.
All claims expressed in this article are solely those of the authors and do not necessarily represent those of their affiliated organizations, the publisher, the editors, and the reviewers. Any product that may be evaluated in this article, or claim that may be made by its manufacturer, is not guaranteed or endorsed by the publisher.
The Supplementary Material for this article can be found online at: https://www.frontiersin.org/articles/10.3389/fenvs.2022.872898/full#supplementary-material
Ahmad, W., and Jairjpuri, M. S. (2010). “Mononchida: The Predaceous Nematodes inNematology Monographs and Perspectives 7,” in. Editor E. J. Brill Biodiversity of Soil Inhabiting Nematodes from Natural Habitat as Well as Agro-Ecosystem (Netherlands, 1–299.
Allouche, M., Ishak, S., Ben Ali, M., Hedfi, A., Almalki, M., Karachle, P. K., et al. (2022). Molecular Interactions of Polyvinyl Chloride Microplastics and Beta-Blockers (Diltiazem and Bisoprolol) and Their Effects on Marine Meiofauna: Combined In Vivo and Modeling Study. J. Hazard. Mater. 431, 128609. doi:10.1016/j.jhazmat.2022.128609
Bläsing, M., and Amelung, W. (2018). Plastics in Soil: Analytical Methods and Possible Sources. Sci. Total Environ. 612, 422–435. doi:10.1016/j.scitotenv.2017.08.086
Bongers, T., and Bongers, M. (1998). Functional Diversity of Nematodes. Appl. Soil Ecol. 10, 239–251. doi:10.1016/s0929-1393(98)00123-1
Bongers, T. (1990). The Maturity Index: an Ecological Measure of Environmental Disturbance Based on Nematode Species Composition. Oecologia 83, 14–19. doi:10.1007/bf00324627
Boots, B., Russell, C. W., and Green, D. S. (2019). Effects of Microplastics in Soil Ecosystems: above and below Ground. Environ. Sci. Technol. 53, 11496–11506. doi:10.1021/acs.est.9b03304
Boström, C., Cedergren, A., Johansson, G., and Pettersson, I. (1974). Rapid Coulometric Method for the Kjeldahl Determination of Nitrogen. Talanta 21, 1123–1127. doi:10.1016/0039-9140(74)80095-0
Büks, F., and Kaupenjohann, M. (2020). Global Concentrations of Microplastic in Soils–a Review. Soil Discuss. 6, 649–662. doi:10.5194/soil-2020-50
Chen, H., Hua, X., Li, H., Wang, C., Dang, Y., Ding, P., et al. (2021). Transgenerational Neurotoxicity of Polystyrene Microplastics Induced by Oxidative Stress in Caenorhabditis elegans. Chemosphere 272, 129642. doi:10.1016/j.chemosphere.2021.129642
Chen, H. (2016). Synergistic Effects of Microplastic and Glyphosate on Soil Microbial Activities in Chinese Loess Soil.
Crowther, T. W., Stanton, D. W. G., Thomas, S. M., A'Bear, A. D., Hiscox, J., Jones, T. H., et al. (2013). Top-down Control of Soil Fungal Community Composition by a Globally Distributed Keystone Consumer. Ecology 94, 2518–2528. doi:10.1890/13-0197.1
de Souza Machado, A. A., Kloas, W., Zarfl, C., Hempel, S., and Rillig, M. C. (2018). Microplastics as an Emerging Threat to Terrestrial Ecosystems. Glob. Change Biol. 24, 1405–1416. doi:10.1111/gcb.14020
de Souza Machado, A. A., Lau, C. W., Kloas, W., Bergmann, J., Bachelier, J. B., Faltin, E., et al. (2019). Microplastics Can Change Soil Properties and Affect Plant Performance. Environ. Sci. Technol. 53, 6044–6052. doi:10.1021/acs.est.9b01339
Delgado-Baquerizo, M., Powell, J. R., Hamonts, K., Reith, F., Mele, P., Brown, M. V., et al. (2017). Circular Linkages between Soil Biodiversity, Fertility and Plant Productivity Are Limited to Topsoil at the Continental Scale. New Phytol. 215, 1186–1196. doi:10.1111/nph.14634
Dong, S., Qu, M., Rui, Q., and Wang, D. (2018). Combinational Effect of Titanium Dioxide Nanoparticles and Nanopolystyrene Particles at Environmentally Relevant Concentrations on Nematode Caenorhabditis elegans. Ecotoxicol. Environ. Saf. 161, 444–450. doi:10.1016/j.ecoenv.2018.06.021
Ferris, H., Bongers, T., and de Goede, R. G. M. (2001). A Framework for Soil Food Web Diagnostics: Extension of the Nematode Faunal Analysis Concept. Appl. Soil Ecol. 18, 13–29. doi:10.1016/s0929-1393(01)00152-4
Ferris, H. (2010). Form and Function: Metabolic Footprints of Nematodes in the Soil Food Web. Eur. J. Soil Biol. 46, 97–104. doi:10.1016/j.ejsobi.2010.01.003
Ferris, H., Sánchez-Moreno, S., and Brennan, E. B. (2012). Structure, Functions and Interguild Relationships of the Soil Nematode Assemblage in Organic Vegetable Production. Appl. Soil Ecol. 61, 16–25. doi:10.1016/j.apsoil.2012.04.006
Forge, T. A., Bittman, S., and Kowalenko, C. G. (2005). Responses of Grassland Soil Nematodes and Protozoa to Multi-Year and Single-Year Applications of Dairy Manure Slurry and Fertilizer. Soil Biol. Biochem. 37, 1751–1762. doi:10.1016/j.soilbio.2004.11.013
Freckman, D. W., and Ettema, C. H. (1993). Assessing Nematode Communities in Agroecosystems of Varying Human Intervention. Agric. Ecosyst. Environ. 45, 239–261. doi:10.1016/0167-8809(93)90074-y
Fueser, H., Mueller, M.-T., Weiss, L., Höss, S., and Traunspurger, W. (2019). Ingestion of Microplastics by Nematodes Depends on Feeding Strategy and Buccal Cavity Size. Environ. Pollut. 255, 113227. doi:10.1016/j.envpol.2019.113227
Fuller, S., and Gautam, A. (2016). A Procedure for Measuring Microplastics Using Pressurized Fluid Extraction. Environ. Sci. Technol. 50, 5774–5780. doi:10.1021/acs.est.6b00816
He, D., Luo, Y., Lu, S., Liu, M., Song, Y., and Lei, L. (2018). Microplastics in Soils: Analytical Methods, Pollution Characteristics and Ecological Risks. TrAC Trends Anal. Chem. 109, 163–172. doi:10.1016/j.trac.2018.10.006
Hedlund, K., and Öhrn, M. S. (2000). Tritrophic Interactions in a Soil Community Enhance Decomposition Rates. Oikos 88, 585–591. doi:10.1034/j.1600-0706.2000.880315.x
Huang, D., Wang, X., Yin, L., Chen, S., Tao, J., Zhou, W., et al. (2022). Research Progress of Microplastics in Soil-Plant System: Ecological Effects and Potential Risks. Sci. Total Environ. 812, 151487. (in press). doi:10.1016/j.scitotenv.2021.151487
Hurley, R. R., and Nizzetto, L. (2018). Fate and Occurrence of Micro(nano)plastics in Soils: Knowledge Gaps and Possible Risks. Curr. Opin. Environ. Sci. Health 1, 6–11. doi:10.1016/j.coesh.2017.10.006
Jenkins, W. R. (1964). A Rapid Centrifugal-Flotation Technique for Separating Nematodes from Soil. Plant Dis. reptr. 48, 692.
Kaile Zhang, K., Schumacher, L., Maltais-Landry, G., Grabau, Z. J., George, S., Wright, D., et al. (2022). Integrating Perennial Bahiagrass into the Conventional Rotation of Cotton and Peanut Enhances Interactions between Microbial and Nematode Communities. Appl. Soil Ecol. 170, 104254. doi:10.1016/j.apsoil.2021.104254
Kim, H. M., Lee, D.-K., Long, N. P., Kwon, S. W., and Park, J. H. (2019). Uptake of Nanopolystyrene Particles Induces Distinct Metabolic Profiles and Toxic Effects in Caenorhabditis elegans. Environ. Pollut. 246, 578–586. doi:10.1016/j.envpol.2018.12.043
Kim, S. W., and An, Y.-J. (2019). Soil Microplastics Inhibit the Movement of Springtail Species. Environ. Int. 126, 699–706. doi:10.1016/j.envint.2019.02.067
Kim, S. W., Jeong, S.-W., and An, Y.-J. (2021). Microplastics Disrupt Accurate Soil Organic Carbon Measurement Based on Chemical Oxidation Method. Chemosphere 276, 130178. doi:10.1016/j.chemosphere.2021.130178
Kim, S. W., Kim, D., Jeong, S.-W., and An, Y.-J. (2020a). Size-dependent Effects of Polystyrene Plastic Particles on the Nematode Caenorhabditis elegans as Related to Soil Physicochemical Properties. Environ. Pollut. 258, 113740. doi:10.1016/j.envpol.2019.113740
Kim, S. W., Waldman, W. R., Kim, T.-Y., and Rillig, M. C. (2020b). Effects of Different Microplastics on Nematodes in the Soil Environment: Tracking the Extractable Additives Using an Ecotoxicological Approach. Environ. Sci. Technol. 54, 13868–13878. doi:10.1021/acs.est.0c04641
Lei, L., Liu, M., Song, Y., Lu, S., Hu, J., Cao, C., et al. (2018a). Polystyrene (Nano) Microplastics Cause Size-dependent Neurotoxicity, Oxidative Damages and Other Adverse Effects in Caenorhabditis elegans. Environ. Sci. Nano. 5. 2009–2020. doi:10.1039/c8en00412a
Lei, L., Wu, S., Lu, S., Liu, M., Song, Y., Fu, Z., et al. (2018b). Microplastic Particles Cause Intestinal Damage and Other Adverse Effects in Zebrafish Danio rerio and Nematode Caenorhabditis elegans. Sci. Total Environ. 619-620, 1–8. doi:10.1016/j.scitotenv.2017.11.103
Lenoir, L., Persson, T., Bengtsson, J., Wallander, H., and Wirén, A. (2006). Bottom-up or Top-Down Control in Forest Soil Microcosms? Effects of Soil Fauna on Fungal Biomass and C/N Mineralisation. Biol. Fertil. Soils 43, 281–294. doi:10.1007/s00374-006-0103-8
Lin, D., Yang, G., Dou, P., Qian, S., Zhao, L., Yang, Y., et al. (2021). Microplastics Negatively Affect Soil Fauna but Stimulate Microbial Activity: Insights from a Field-Based Microplastic Addition Experiment. Proc. Biol. Sci. 287, 20201268. doi:10.1098/rspb.2020.1268
Liu, H., Yang, X., Liu, G., Liang, C., Xue, S., Chen, H., et al. (2017). Response of Soil Dissolved Organic Matter to Microplastic Addition in Chinese Loess Soil. Chemosphere 185, 907–917. doi:10.1016/j.chemosphere.2017.07.064
Liu, M., Lu, S., Song, Y., Lei, L., Hu, J., Lv, W., et al. (2018). Microplastic and Mesoplastic Pollution in Farmland Soils in Suburbs of Shanghai, China. Environ. Pollut. 242, 855–862. doi:10.1016/j.envpol.2018.07.051
Martínez-Campos, S., Pissaridou, P., Drakou, K., Shammas, C., Andreou, K., González-Pleiter, M., et al. (2022). Evolution of Prokaryotic Colonisation of Greenhouse Plastics Discarded into the Environment. Ecotoxicol. Environ. Saf. 232, 113213. doi:10.1016/j.ecoenv.2022.113213
Moore, J. C., McCann, K., Setälä, H., and de Ruiter, P. C. (2003). Top-down Is Bottom-Up: Does Predation in the Rhizosphere Regulate Aboveground Dynamics? Ecology 84, 846–857. doi:10.1890/0012-9658(2003)084[0846:tibdpi]2.0.co;2
Mueller, M.-T., Fueser, H., Höss, S., and Traunspurger, W. (2020a). Species-specific Effects of Long-Term Microplastic Exposure on the Population Growth of Nematodes, with a Focus on Microplastic Ingestion. Ecol. Indic. 118, 106698. doi:10.1016/j.ecolind.2020.106698
Mueller, M.-T., Fueser, H., Trac, L. N., Mayer, P., Traunspurger, W., and Höss, S. (2020b). Surface-related Toxicity of Polystyrene Beads to Nematodes and the Role of Food Availability. Environ. Sci. Technol. 54, 1790–1798. doi:10.1021/acs.est.9b06583
Okeke, E. S., Okoye, C. O., Atakpa, E. O., Ita, R. E., Nyaruaba, R., Mgbechidinma, C. L., et al. (2022). Microplastics in Agroecosystems-Impacts on Ecosystem Functions and Food Chain. Resour. Conservation Recycl. 177, 105961. doi:10.1016/j.resconrec.2021.105961
Olsen, S., Sommers, L., and Page, A. (1982). Methods of Soil Analysis. Part 2. Chemical and Microbiological Properties of Phosphorus. ASA Monogr. 9, 403–430.
Parthasarathy, A., Tyler, A. C., Hoffman, M. J., Savka, M. A., and Hudson, A. O. (2019). Is Plastic Pollution in Aquatic and Terrestrial Environments a Driver for the Transmission of Pathogens and the Evolution of Antibiotic Resistance? Environ. Sci. Technol. 53, 1744–1745. doi:10.1021/acs.est.8b07287
Qi, Y., Yang, X., Pelaez, A. M., Huerta Lwanga, E., Beriot, N., Gertsen, H., et al. (2018). Macro- and Micro- Plastics in Soil-Plant System: Effects of Plastic Mulch Film Residues on Wheat (Triticum aestivum) growth(Triticum aestivum)growth. Sci. Total Environ. 645, 1048–1056. doi:10.1016/j.scitotenv.2018.07.229
Qu, M., Xu, K., Li, Y., Wong, G., and Wang, D. (2018). Using Acs-22 Mutant Caenorhabditis elegans to Detect the Toxicity of Nanopolystyrene Particles. Sci. Total Environ. 643, 119–126. doi:10.1016/j.scitotenv.2018.06.173
Rauchschwalbe, M.-T., Höss, S., Haegerbaeumer, A., and Traunspurger, W. (2022). Long-term Exposure of a Free-Living Freshwater Micro- and Meiobenthos Community to Microplastic Mixtures in Microcosms. Sci. Total Environ. 827, 154207. doi:10.1016/j.scitotenv.2022.154207
Rillig, M. C., Lehmann, A., de Souza Machado, A. A., and Yang, G. (2019). Microplastic Effects on Plants. New Phytol. 223, 1066–1070. doi:10.1111/nph.15794
Rillig, M. C., and Lehmann, A. (2020). Microplastic in Terrestrial Ecosystems. Science 368, 1430–1431. doi:10.1126/science.abb5979
Rillig, M. C. (2012). Microplastic in Terrestrial Ecosystems and the Soil? Environ. Sci. Technol. 46, 6453–6454. doi:10.1021/es302011r
Rodríguez-Seijo, A., Santos, B., da Silva, E. F., Cachada, A., and Pereira, R. (2018). Low-density Polyethylene Microplastics as a Source and Carriers of Agrochemicals to Soil and Earthworms. Environ. Chem. 16, 8–17.
Schöpfer, L., Menzel, R., Schnepf, U., Ruess, L., Marhan, S., Brümmer, F., et al. (2020). Microplastics Effects on Reproduction and Body Length of the Soil-Dwelling Nematode Caenorhabditis elegans. Front. Environ. Sci. 8, 41. doi:10.3389/fenvs.2020.00041
Selonen, S., Dolar, A., Jemec Kokalj, A., Skalar, T., Parramon Dolcet, L., Hurley, R., et al. (2020). Exploring the Impacts of Plastics in Soil - the Effects of Polyester Textile Fibers on Soil Invertebrates. Sci. Total Environ. 700, 134451. doi:10.1016/j.scitotenv.2019.134451
Shang, X., Lu, J., Feng, C., Ying, Y., He, Y., Fang, S., et al. (2020). Microplastic (1 and 5 μm) Exposure Disturbs Lifespan and Intestine Function in the Nematode Caenorhabditis elegans. Sci. Total Environ. Total Environ. 705, 135837. doi:10.1016/j.scitotenv.2019.135837
Sieriebriennikov, B., Ferris, H., and de Goede, R. G. M. (2014). NINJA: An Automated Calculation System for Nematode-Based Biological Monitoring. Eur. J. Soil Biol. 61, 90–93. doi:10.1016/j.ejsobi.2014.02.004
Stanković, J., Milošević, D., Jovanović, B., Savić-Zdravković, D., Petrović, A., Raković, M., et al. (2022). In Situ effects of a Microplastic Mixture on the Community Structure of Benthic Macroinvertebrates in a Freshwater Pond. Environ. Toxicol. Chem. 44, 888–895.doi:10.1002/etc.5119
Su, Y., Wang, X., Yang, R., Yang, X., and Liu, W. (2012). Soil Fertility, Salinity and Nematode Diversity Influenced by Tamarix Ramosissima in Different Habitats in an Arid Desert Oasis. Environ. Manage. 50, 226–236.doi:10.1007/s00267-012-9872-z
Sun, Y., Duan, C., Cao, N., Li, X., Li, X., Chen, Y., et al. (2022). Effects of Microplastics on Soil Microbiome: The Impacts of Polymer Type, Shape, and Concentration. Sci. Total Environ. 806, 150516. doi:10.1016/j.scitotenv.2021.150516
van den Hoogen, J., Geisen, S., Routh, D., Ferris, H., Traunspurger, W., Wardle, D. A., et al. (2019). Soil Nematode Abundance and Functional Group Composition at a Global Scale. Nature 572, 194–198. doi:10.1038/s41586-019-1418-6
van der Heijden, M. G. A., Bardgett, R. D., and van Straalen, N. M. (2008). The Unseen Majority: Soil Microbes as Drivers of Plant Diversity and Productivity in Terrestrial Ecosystems. Ecol. Lett. 11, 296–310. doi:10.1111/j.1461-0248.2007.01139.x
Wagg, C., Bender, S. F., Widmer, F., and van der Heijden, M. G. A. (2014). Soil Biodiversity and Soil Community Composition Determine Ecosystem Multifunctionality. Proc. Natl. Acad. Sci. U.S.A. 111, 5266–5270. doi:10.1073/pnas.1320054111
Wakkaf, T., Allouche, M., Harrath, A. H., Mansour, L., Alwasel, S., Mohamed Thameemul Ansari, K. G., et al. (2020). The Individual and Combined Effects of Cadmium, Polyvinyl Chloride (PVC) Microplastics and Their Polyalkylamines Modified Forms on Meiobenthic Features in a Microcosm. Environ. Pollut. 266, 115263. doi:10.1016/j.envpol.2020.115263
Walkley, A., and Black, I. A. (1934). An Examination of the Degtijareff Method for Determining Soil Organic Matter and a Proposed Modification of the Chromic Acid Titration Method. Soil Sci. 37, 29–38.doi:10.1097/00010694-193401000-00003
Wang, F., Zhang, X., Zhang, S., Zhang, S., and Sun, Y. (2020). Interactions of Microplastics and Cadmium on Plant Growth and Arbuscular Mycorrhizal Fungal Communities in an Agricultural Soil. Chemosphere 254, 126791. doi:10.1016/j.chemosphere.2020.126791
Wang, F., Wang, Q., Adams, C. A., Sun, Y., and Zhang, S. (2022). Effects of Microplastics on Soil Properties: Current Knowledge and Future Perspectives. J. Hazard. Mater. 424, 127531. doi:10.1016/j.jhazmat.2021.127531
Wardle, D. A. (2006). The Influence of Biotic Interactions on Soil Biodiversity. Ecol. Lett. 9, 870–886. doi:10.1111/j.1461-0248.2006.00931.x
Weithmann, N., Möller, J. N., Löder, M. G. J., Piehl, S., Laforsch, C., and Freitag, R. (2018). Organic Fertilizer as a Vehicle for the Entry of Microplastic into the Environment. Sci. Adv. 4, eaap8060. doi:10.1126/sciadv.aap8060
Wu, Y., Zhou, H., Chen, W., Zhang, Y., Wang, J., Liu, H., et al. (2021). Response of the Soil Food Web to Warming and Litter Removal in the Tibetan Plateau, China. Geoderma 401, 115318. doi:10.1016/j.geoderma.2021.115318
Yeates, G. W., Bongers, T., De Goede, R. G., Freckman, D. W., and Georgieva, S. S. (1993). Feeding Habits in Soil Nematode Families and Genera-An Outline for Soil Ecologists. J. Nematol. 25, 315–331. doi:10.1016/0038-0717(93)90089-t
Yin, L., Wen, X., Huang, D., Du, C., Deng, R., Zhou, Z., et al. (2021). Interactions between Microplastics/nanoplastics and Vascular Plants. Environ. Pollut. 290, 117999. doi:10.1016/j.envpol.2021.117999
Zhang, B., Yang, X., Chen, L., Chao, J., Teng, J., and Wang, Q. (2020). Microplastics in Soils: a Review of Possible Sources, Analytical Methods and Ecological Impacts. J. Chem. Technol. Biotechnol. 95, 2052–2068. doi:10.1002/jctb.6334
Zhao, L., Qu, M., Wong, G., and Wang, D. (2017). Transgenerational Toxicity of Nanopolystyrene Particles in the Range of μg L−1 in the Nematode Caenorhabditis elegans. Environ. Sci. Nano 4, 2356–2366. doi:10.1039/c7en00707h
Keywords: polypropylene (PP), microplastic concentration, soil nematode community, abundance, diversity, metabolic footprint
Citation: Yang B, Li P, Entemake W, Guo Z and Xue S (2022) Concentration-Dependent Impacts of Microplastics on Soil Nematode Community in Bulk Soils of Maize: Evidence From a Pot Experiment. Front. Environ. Sci. 10:872898. doi: 10.3389/fenvs.2022.872898
Received: 17 February 2022; Accepted: 29 April 2022;
Published: 14 June 2022.
Edited by:
Juying Li, Shenzhen University, ChinaReviewed by:
Andrés Rodríguez-Seijo, University of Porto, PortugalCopyright © 2022 Yang, Li, Entemake, Guo and Xue. This is an open-access article distributed under the terms of the Creative Commons Attribution License (CC BY). The use, distribution or reproduction in other forums is permitted, provided the original author(s) and the copyright owner(s) are credited and that the original publication in this journal is cited, in accordance with accepted academic practice. No use, distribution or reproduction is permitted which does not comply with these terms.
*Correspondence: Sha Xue, eHVlc2hhMTAwQDE2My5jb20=
Disclaimer: All claims expressed in this article are solely those of the authors and do not necessarily represent those of their affiliated organizations, or those of the publisher, the editors and the reviewers. Any product that may be evaluated in this article or claim that may be made by its manufacturer is not guaranteed or endorsed by the publisher.
Research integrity at Frontiers
Learn more about the work of our research integrity team to safeguard the quality of each article we publish.