- 1Department of Animal Biology, Plant Biology and Ecology, and University Research Institute on Olive Groves and Olive Oils, University of Jaén, Jaén, Spain
- 2Department of Geology, University of Jaén, Jaén, Spain
In Southern Spain, olive trees have traditionally been cultivated in marginal areas with relatively shallow and bare soils under rainfed conditions, resulting in heavy soil losses and soil degradation. The implementation of temporary spontaneous cover crops in the inter-rows of olive groves, has proven to be a suitable diversification strategy to reduce soil erosion but it can also contribute to climate change mitigation and the boosting of internal nutrient recycling. However, information on the contribution of cover crops to atmospheric CO2 fixation and on nutrient retention in olive groves is scarce, which is a major drawback when it comes to modelling on larger spatial scales. In this study, we aimed to assess the potential effects of temporary spontaneous cover crops in olive groves on CO2 fixation and nutrient retention. The aerial biomass of cover crops (0.25m2 frames) and contents of carbon and nitrogen (CNHS analyser), phosphorus and potassium (IPC-MS) were analyzed in 46 commercial olive groves with different tree densities and cover crop layouts; the whole farm (WCC), the whole farm except the area below the tree canopy (CCC) or in bands of a given width in the inter-row area (BCC). Cover crops of 56% of the olive groves were under BCC whereas only 17% were under WCC. The annual net primary production of cover crops under WCC (1,707.4 kg DM ha−1 y−1) was significantly higher than that of CCC (769.5 kg DM ha−1 y−1) and with intermediate values for BCC (1,186.4 kg DM ha−1 y−1). Similarly, the annual rate of C-CO2 fixation in the annual net primary production of olive groves with WCC (642.1 kg C ha−1 y−1) was 1.35 and 2.1 times higher than the olive groves with BCC and CCC, respectively. On average, 19.5 kg N ha−1 y−1, 2.48 kg P ha−1 y−1 and 24.30 kg K ha−1 y−1 was accumulated in the biomass of the cover crops. This study demonstrates that cover crops contribute significantly to CO2 reduction and the retention of significant amounts of tree-unused nutrients. In addition, the higher the area covered by cover crops, the higher the contribution to these ecosystem services.
1 Introduction
Olea europaea L. is the most important perennial crop grown in the Mediterranean basin. It occupies about 10 million ha and it shapes the socio-economic and cultural life of many villages (Loumou and Giourga, 2003). Furthermore, olive orchards configure the rural natural landscapes of many areas in Spain, Italy, Portugal and Greece. Andalusia, the southernmost region of Spain, is the largest producer of olive oil in the world, and about 47% of its arable land is devoted to olive groves (MAPA, 2020a). Therefore, current and subsequent changes in management practices have a great impact on climate change mitigation and adaption, biodiversity and provision of ecosystem services on a regional scale (López-Vicente et al., 2021).
In the pre-industrial period, olive-groves often fulfilled multiple functions in rural communities, but they gradually shifted to intensive systems to maximize commercial profitability (Infante-Amate and de Molina, 2013). This entailed several changes in management from 1970 onwards, such as an increase in the tree density, the widespread application of synthetic agrochemicals and an intensive use of tillage, irrigation and fossil fuels (Beaufoy, 2001). In recent decades, olive farming has also become a paradigmatic example of territorial expansion and concentration with a clear trend towards monoculture (Ortega et al., 2020). This has in turn resulted in simplified landscapes with a relatively low capacity for providing ecosystem services other than the production of olives fruit.
Olive cultivation is strongly associated with soil and water losses by erosion and runoff mainly due to: 1) its location on marginal soils and steeply sloping soils (Gómez et al., 2003), 2) the relatively intensive and short rainfall episodes during autumn and late winter, characteristics of Mediterranean climate (Morugán-Coronado et al., 2020), 3) the sparse ground cover provided by the tree canopy (Fraga et al., 2021), 4) the soil management based on the systematic removal of the unwanted herbaceous vegetation to avoid competition for water, often by tillage (Gómez, 2017). As a result, erosion has been a historical source of environmental degradation in olive groves, which has been exacerbated by the intensification process (Vanwalleghem et al., 2011). Soil erosion not only means the loss of the inorganic fraction of the soil, but also the loss of soil organic matter, which is an important reservoir of organic carbon, nutrients and valuable soil biota (Zuazo and Pleguezuelo, 2008). Therefore, soil erosion is a great threat to the economical sustainability of the olive grove, since it impacts negatively on the soil fertility (Liu et al., 2021).
In order to mitigate soil losses by erosion, the regional authorities of Spain implemented a regulation that enforced the establishment of temporary herbaceous covers of a minimum of 1 m width in the inter-row area of olive groves with a mean slope greater than 15% (MAGRAMA, 2014). Moreover, it is likely that these conditions will be changed to a minimum of 2 m width on a mean slope higher than 10% in the new eco-schemes of the CAP 2023—2027 (European Commission, 2021).
Cover crops are a recognized agricultural diversification tool (Rosa-Schleich et al., 2019). In woody crops orchards, they usually consist of a native or seeded herbaceous cover intercropped in the inter-rows, which is mowed or removed at a given time to avoid competition with the trees for water and nutrients (Junta de Andalucía, 2007; Francaviglia and Vicente-Vicente, 2021). It has been demonstrated that cover crops provide a wide variety of soil ecosystem services such as reduction of soil and water loss by erosion and runoff (Sastre et al., 2017; Gómez et al., 2018; Repullo-Ruibérriz de Torres et al., 2018). They have also been shown to increase the stock of soil organic carbon (Almagro and Martínez-Mena, 2014; López-Vicente et al., 2021). When implemented between the harvest and the sowing of annual crops, cover crops have demonstrated to decrease nutrient leaching (Abdalla et al., 2019). In perennial woody crops, such as olive groves, temporary spontaneous cover crops (TSCC) reduced the nutrient losses during rainfall events by reducing runoff yield and soil loss (Rodríguez-Lizana et al., 2007; Gómez et al., 2009). Some studies have suggested that cover crops might retain nutrients when the nutrient demand of the tree is low (García-Ruiz et al., 2011) and then release them through mineralization when the olive tree is active (Gómez-Muñoz et al., 2014). Furthermore, cover crops may also reduce the downward movement of nitrate and potassium, retrieve N, P and K from superficial and deep soil layers, and fix atmospheric N2 if the TSCC community includes a significant proportion of legumes. Therefore, one of the roles of TSCC in olive orchards is to boost internal nutrient recycling by transforming inorganic nutrients into organic nutrients embedded in the TSCC biomass. However, there is little information on the nutrient retention capacity of cover crops in tree crops and olive groves (Gómez-Muñoz et al., 2014; Rodríguez-Liazana et al., 2020; Repullo-Ruibérriz de Torres et al., 2021a). Most of the studies on the effects of cover crops on olive groves usually focus on particular species to be used as seeded cover crops, although they often include spontaneous cover crops for comparative purposes. On the other hand, these studies are not based on extensive sampling, but are performed on a single or few experimental plots. This is a major drawback in extrapolating the results on to a larger spatial extent (Gómez, 2017), which is necessary to model the beneficial effects of diversification in olive groves on climate change mitigation and nutrient retention.
Boosted by cross-compliance measures attached to agrarian subsidies and by scientific dissemination, the implementation of cover crops is becoming popular in olive-groves in Andalusia (Junta de Andalucía, 2015b). According to the last national survey on soil management (MAPA, 2020b), 37% of the olive grove areas in the region are managed by cover crops, of which about 99% are temporary spontaneous cover crops. However, this survey does not provide any information on the percentage of soil covered by cover crops, different cover crop design strategies, the number of months taken to maintain the cover crops, the control methods or the amount of biomass produced. Recent studies have used GIS and remote sensing methodologies to estimate the degree of soil cover (Peña-Barragán et al., 2004; Cruz-Ramírez et al., 2012; Lima-Cueto et al., 2019) or the biomass production of temporary spontaneous cover crops through satellite or aerial images (Blázquez et al., 2021). Although these methodologies are suitable to estimate the covered area, they are still not sufficiently precise to estimate the biomass.
The capacity of temporary spontaneous cover crops as a diversification strategy to provide ecosystem services is ultimately determined by the fraction of soil covered (Zuazo and Pleguezuelo, 2008; Laflen et al., 1985; Unger et al., 1991; Sastre et al., 2017) and the biomass produced (Finney et al., 2016; Gómez, 2017). For instance, Gómez et al. (2009) found that by applying the RUSLE model in olive groves, the higher the area covered by TSCC (WCC and CCC), the lower the soil erosion. Therefore, proper characterization of the temporary spontaneous cover crops in many real and representative olive groves of Andalusia needs to be accomplished. We hypothesize that TSCC might significantly reinforce the role of olive groves in mitigating climate change and in retaining nutrients within the orchard, and that the magnitude of these will differ according to the TSCC layout models. This study aims to assess the aboveground net primary production, the nutrient retention potential and the carbon fixation of TSSC in 46 commercial olive groves of Andalusia.
2 Materials and Methods
2.1 Olive Groves Selection
46 olive groves with spontaneous cover crops before 2015 were randomly selected using Google Earth Pro images from the provinces of Jaén, Córdoba, Granada and Seville (Figure 1; Supplementary Table S1). These are the areas with the highest olive oil production in Andalusia, accounting for 72% of the Spanish and 27% of the total world production (Vilar and Pereira, 2018; MAPA, 2020a).
Sampling was carried out on the selected olive groves from mid-March to mid-April 2021. In each olive grove, the canopy area of five trees was measured using Google Earth Pro and the total canopy area per hectare was obtained from the mean tree canopy area and the tree density. Sampled olive groves included 30 traditional (<150 trees per hectare), 13 intensive (150—400 trees per hectare), and three super-intensive orchards (>400 trees per hectare), with a tree canopy area ranging between 534.9 and 4,833.5 m2 ha−1 (Supplementary Table S1). The annual rainfall during 2021 and over the previous 10 years (2011–2020) was obtained from the closest weather stations (Agroclimatic Information Network of Andalusia, RIA). The 10-years (2010–2020) mean annual rainfall ranged between 332 and 615 mm, whereas that of 2021 was on average, 25% lower (between 204–459) than that (RIA, 2021).
The soil types of the selected plots were obtained from the REDIAM WMS Soil Map of Andalusia (Junta de Andalucía, 2012), prepared by the Ministry of the Environment using as reference base the Landsat-TM satellite orthoimage. The soils appear in cartographic units characterized by associations grouped at the second order level of the FAO classification criteria (FAO-UNESCO, 1974) and the Soil Map of the European Union (CEC, 1985).
The main soil types in the selected olive groves are calcareous regosols and calcic cambisols with lithosols, calcareous fluvisols and rendsins (37% of the olive groves), calcium cambisols with calcareous regosols (13%) and calcareous regosols and calcium cambisols with calcium luvisols and calcareous fluvisols (11%).
2.2 Sampling of Temporary Spontaneous Cover Crop and Aboveground Net Primary Production Estimates
The aboveground biomass was estimated by the Ravindranath and Ostwald harvest method (2008). Olive groves were visited between mid-March 2021 to the end of April 2021 and the aboveground biomass of the temporary spontaneous cover crops was harvested a few days before the farmers controlled them. Mid-March to Mid-April is the recommended period to control the cover crop to avoid competition for water in Andalusia, especially in rainfed olive groves (Junta de Andalucía, 2007). In each of the olive groves, five 0.5 m × 0.5 m frames were randomly set in the area covered by temporary spontaneous vegetation and the aboveground biomass was manually cut to ground level with grass shears and stored in plastic bags (Gómez-Muñoz et al., 2014). In each of the olive groves where the spontaneous cover crops occupied a strip in the inter-row tree area, the width of at least five strips was recorded.
Samples were dried in an oven at 50°C for 4 days. After being dried, the harvested biomass was weighed. The harvested aboveground biomass was assumed to be the aboveground net annual primary production as it was produced between April of 2020 to mid-April 2021, when sampling was carried out.
The area covered by temporary spontaneous cover crops (TSCC) in 1 ha was estimated by photointerpretation of Google Earth Pro images depending on the cover crop layout. For olives groves where the whole ground was covered by the TSCC (hereinafter referred to as WCC), an occupation of 100% of the area was assumed. For olives groves where the cover crop was absent under the tree canopy but occupied all the inter-tree rows (hereinafter referred to as CCC), the cleared area under the tree canopy of five trees was measured using Google Earth Pro and the mean was extrapolated to 1 ha by considering the tree density. This cleared area was subtracted from 1 ha to calculate the area covered by the TSCC. When the TSCC of the olive groves occupied a strip of the inter-tree row area (hereinafter referred to as BCC) of a given width, the number of inter-tree rows in 100 m and the mean width area were taken into account to calculate the area in 1 ha occupied by temporary cover crops.
The annual aboveground net primary production (kg DM ha−1 y−1) was calculated by taking into account the harvested biomass in the frames (g DM in 0.25 m2) and the area covered by the cover crop in 1 ha.
2.3 Carbon, Nitrogen, Phosphorus and Potassium Analyses
Once the biomass was weighed, an aliquot of between 20 and 100 g was completely ground to powder with a hammer mill with a 1 mm sieve. Carbon and nitrogen were analyzed in a CHN elemental analyzer (Leco TruSpect Micro). In addition, an aliquot of the milled biomass was subjected to perchloric-nitric (3:5 v/v) digestion and the total phosphorus (P) and potassium (K) were analyzed in an ICP-MS mass spectrometer (Agilent 7900). The contents of total carbon, nitrogen, phosphorus and potassium of the aboveground biomass of the temporary spontaneous cover crops were expressed as percentages on dry weight basis.
The accumulation of carbon, nitrogen, phosphorus and potassium in the TSCC (kg element ha−1 y−1) was calculated by taking into account the harvested biomass in the frames (g DM in 0.25 m2), the area covered by the cover crop and the C, N, P and K contents of the aerial biomass.
The atmospheric CO2 captured annually (kg CO2 ha−1 y−1) by the TSCC was calculated from the C accumulated in the aerial biomass (kg C ha−1 y−1), using a molecular weight ratio (1.0 g carbon = 3.66 g CO2) and by assuming that the C taken up by plants comes exclusively from the atmospheric CO2.
2.4 Statistical Analysis
Statistical analyses were performed using the statistical software STATISTICA v.10 (StatSoft Inc.). The effects of the typologies of the TSCC layout (WCC, CCC, and BCC) and the categories of tree densities (traditional, intensive and superintensive) on the studied variables were tested using the non-parametric Kruskal-Wallis test (p < 0.05) and differences between factor groups were tested by pairs with the Mann-Whitney U test (p < 0.05).
3 Results
The statistical results of Kruskal Wallis and Mann-Whitney tests are presented in the Supplementary Tables S2, S3, S4, and S5.
3.1 Spontaneous Cover Crop Layout and Area Covered
Three main cover crop layouts were identified. In eight of the 46 olive farms, TSCC covered the whole olive grove (WCC), whereas in 12, TSCC was absent in the area under tree canopy (CCC). Finally, in 26 of the olive groves, the TSCC was distributed in strips of a given width in the inter-rows area (BCC). The mean width of the strips with TSCC was 3.2 m. Besides that, 69 % and 100% of the olive groves under intensive and super-intensive tree densities, respectively had a TSCC layout of BCC. The mean tree densities of the olive groves managed with WCC, CCC and BCC were 167, 93, and 260 trees per hectare, respectively.
Overall, the area outside the tree canopy projection averaged 7,365.8 m2 ha−1 and there were no significant differences between TSCC layouts or tree densities (Figure 2).
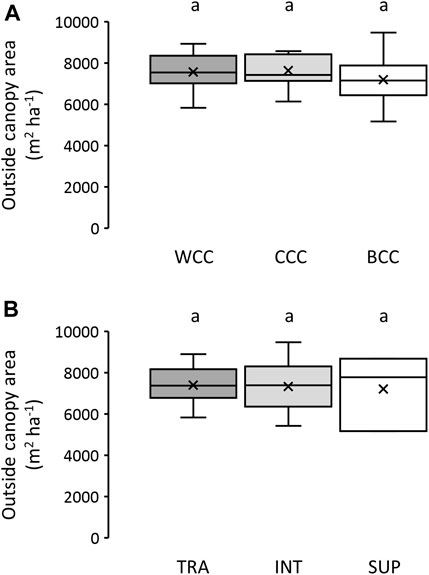
FIGURE 2. Box and whiskers plot of the inter-row area of the olive groves for each of the cover crop layouts (A) and tree density categories (B). Horizontal black line, x-shaped cross, box borders and whiskers stand for the median, the mean, quartiles and outliers. Different letters over the box-plot indicate significant differences (p < 0.05). WCC, CCC and BCC stand for olives groves where TSCC occupied the whole area, was absent under tree canopy or only occupied a strip of the inter-row area, respectively. TRA, INT and SUP stand for olive grove with a traditional, intensive and superintensive tree density.
On average, the area covered by TSCC was 5,615.8 m2 ha−1 with significant differences among the TSCC layouts (Figure 3). Under CCC, the mean area covered was 6,820.9 m2 ha−1 which was significantly higher than the 3,710.6 m2 ha−1 covered in BCC olive groves (Figure 3). No significant differences were found in the area covered by the TSCC among olive groves with different categories of tree densities, which was not unexpected as BCC was the predominant TSCC layout for the three categories of tree densities.
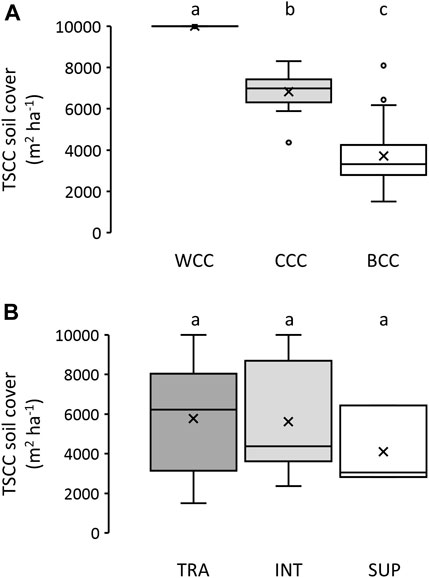
FIGURE 3. Box and whiskers plot of soil covered by TSCC for each of the cover crop layout (A) and tree density (B). Horizontal black line, x-shaped cross, box borders and whiskers stand for the median, the mean, quartiles and outliers. Different letters over the box-plot indicate significant differences (p < 0.05). WCC, CCC and BCC stand for olives groves where TSCC occupied the whole area, was absent under tree canopy or only occupied a strip of the inter-row area, respectively. TRA, INT and SUP stand for olive grove with a traditional, intensive and superintensive tree density.
3.2 Aboveground Net Primary Production of TSCC
Supplementary Figure S1 shows the frequency distribution of the aboveground net primary production (ANPP) per m2 of the TSCC. On average, for the whole set of olive groves, the ANPP in the covered area averaged 200.5 g DM m−2 y−1 with a very high variability (min 20.3 g DM m−2 y−1; max 666.6 g DM m−2 y−1). No significant differences among TSCC layouts were found (Figure 4), although differences were found due to tree densities. The ANPP in super-intensive olive groves (436.1 g DM m−2 y−1) was significantly higher than that in the traditional olive groves (193.3 g DM m−2 y−1).
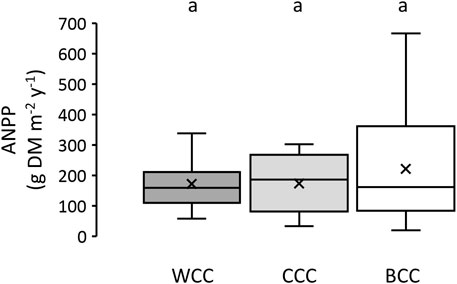
FIGURE 4. Box and whiskers plot of ANPP for each of the cover crop layout. Horizontal black line, x-shaped cross, box borders and whiskers stand for the median, the mean, quartiles and outliers. Different letters over the box-plot indicate significant differences (p < 0.05). WCC, CCC and BCC stand for olives groves where TSCC occupied the whole area, was absent under tree canopy or only occupied a strip of the inter-row area, respectively.
Differences in the ANPP per ha were found between TSCC layouts. The average ANPP per ha in TSCC under WCC (1,707.4 kg DM ha−1 y−1 on average) and under CCC (1,186.4 kg DM ha−1 y−1) were both significantly higher (122 % and 54% higher, respectively) than that of BCC (769.5 kg DM ha−1 y−1) (Figure 5). Despite the average ANPP for CCC (1,186.4 kg DM ha−1 y−1) being 30.5% lower than that of WCC, no significant differences were found (Figure 5). ANPP per ha showed no difference between tree densities.
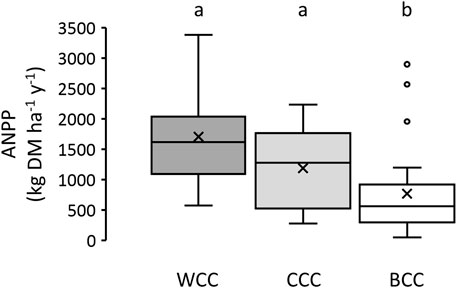
FIGURE 5. Box and whiskers plot of ANPP for each of the cover crop layout. Horizontal black line, x-shaped cross, box borders and whiskers stand for the median, the mean, quartiles and outliers. Different letters over the box-plot indicate significant differences (p < 0.05). WCC, CCC and BCC stand for olives groves where TSCC occupied the whole area, was absent under tree canopy or only occupied a strip of the inter-row area, respectively.
3.3 Carbon Fixation and Nutrient Retention
The carbon content of the aboveground biomass of the TSCC averaged 40.4% with a very low coefficient of variation (4.4%) (Supplementary Figure S2). No differences were found between TSCC layout types.
The amount of C fixed by TSCC on the whole set of olive groves averaged 419.4 kg C ha−1 y−1 (or 1,537.8 kg CO2 ha−1 y−1) (Figure 6). As for the ANPP, the fixed carbon of the TSCC under WCC (704.9 kg C ha−1 y−1 or 2,584.6 kg CO2 ha−1 y−1) and under CCC (475.3 kg C ha−1 y−1 or 1,742.8 kg CO2 ha−1 y−1) were significantly higher than that under BCC (305.7 kg C ha−1 y−1 or 1,120.9 kg CO2 ha−1 y−1). No differences were found between CCC and WCC (Figure 6).
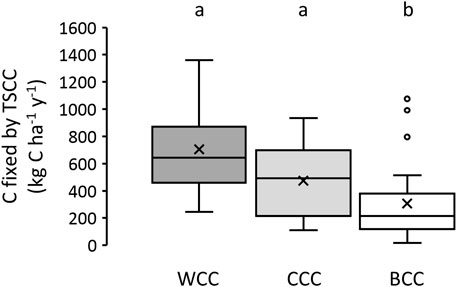
FIGURE 6. Box and whiskers plot of carbon content of TSCC for each of the cover crop layout. Horizontal black line, x-shaped cross, box borders and whiskers stand for the median, the mean, quartiles and outliers. Different letters over the box-plot indicate significant differences (p < 0.05). WCC, CCC and BCC stand for olives groves where TSCC occupied the whole area, was absent under tree canopy or only occupied a strip of the inter-row area, respectively.
For the whole set of olive groves, the nitrogen content of the aboveground biomass of the TSCC was 1.96% with a relatively high variability (coefficient of variation of 47.5%) (Supplementary Figure S2). As expected, differences between tree densities or TSCC layouts were not significant but averages were higher for BCC (2.13%) than for WCC and CCC (1.71 % and 1.76%, respectively).
The amount of N in the aboveground biomass of TSCC averaged 19.5 kg N ha−1 y−1 but there were olive groves with values as low as 1.7 kg N ha−1 y−1 and as high as 98.0 kg N ha−1 y−1. There were no significant differences among tree densities. However, results were significantly higher for WCC (31.1 kg N ha−1 y−1) than for BCC (16.0 kg N ha−1 y−1). No differences were found between CCC (20.3 kg N ha−1 y−1) and the other treatments (Figure 7). Phosphorus content of the aboveground biomass of TSCC of the 46 olive groves averaged 0.24% but values as low as 0.05% and as high as 0.61% were found (Supplementary Figure S2). In this case, there were no significant differences between TSCC layouts or tree densities. Annual P accumulated in the aboveground biomass of TSCC averaged 2.48 kg P ha−1 y−1 with a high variability (89% coefficient of variation). Annual P accumulation in the WCC, CCC and BCC olive groves averaged 3.60, 2.89 and 1.95 kg P ha−1 y−1, but differences were not significant (Figure 8).
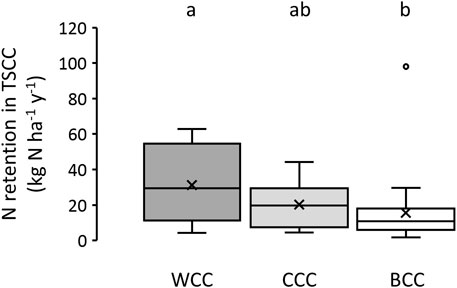
FIGURE 7. Box and whiskers plot of nitrogen retention in the TSCC for each of the cover crop layouts. Horizontal black line, x-shaped cross, box borders and whiskers stand for the median, the mean, quartiles and outliers. Different letters over the box-plot indicate significant differences (p < 0.05). WCC, CCC and BCC stand for olives groves where TSCC occupied the whole area, was absent under tree canopy or only occupied a strip of the inter-row area, respectively.
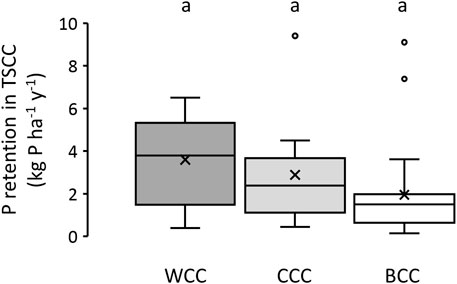
FIGURE 8. Box and whiskers plot of phosphorus retention in TSCC for each of the cover crop layouts. Horizontal black line, x-shaped cross, box borders and whiskers stand for the median, the mean, quartiles and outliers. Different letters over the box-plot indicate significant differences (p < 0.05). WCC, CCC and BCC stand for olives groves where TSCC occupied the whole area, was absent under tree canopy or only occupied a strip of the inter-row area, respectively.
The average potassium content of the dry biomass of the aboveground TSCC was 2.28% with a coefficient of variation of 38% (Supplementary Figure S2). No significant differences in the K content of the TSCC biomass was found due to TSCC layouts or tree densities. The annual rate of K accumulation in the aboveground biomass of TSCC was 24.3 kg K ha−1 y−1. Values for WCC (42.4 kg K ha−1 y−1) were 76.6 % and 123.2% higher than those of CCC (24.0 kg K ha−1 y−1) and BCC (19.0 kg K ha−1 y−1) olive groves, respectively, but the differences were not significant (Figure 9).
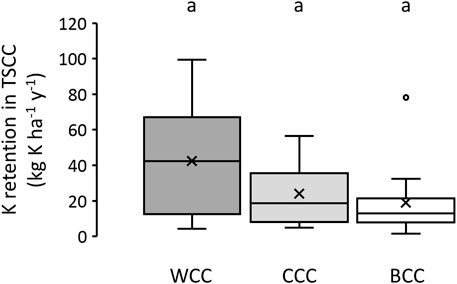
FIGURE 9. Box and whiskers plot of potassium retention in TSCC for each of the cover crop layouts. Horizontal black line, x-shaped cross, box borders and whiskers stand for the median, the mean, quartiles and outliers. Different letters over the box-plot indicate significant differences (p < 0.05). WCC, CCC and BCC stand for olives groves where TSCC occupied the whole area, was absent under tree canopy or only occupied a strip of the inter-row area, respectively.
Mean C/N, C/P, N/P and N/K ratios of the aboveground biomass of the TSCC averaged 26.5, 210.2, 8.6 and 0.9, respectively (Figure 10). Mean C/N and C/P ratios tended to be higher under WCC and lower under BCC with intermediate values for CCC, although differences were not significant in any of the ratios. No significant differences were found between tree densities except for the N/P ratio. In this case, the biomass of the TSCC showed significantly higher values of the N/P ratio in super-intensive olive groves (12.2 on average) than in traditional (8.0 on average) and intensive olive groves (9.0 on average).
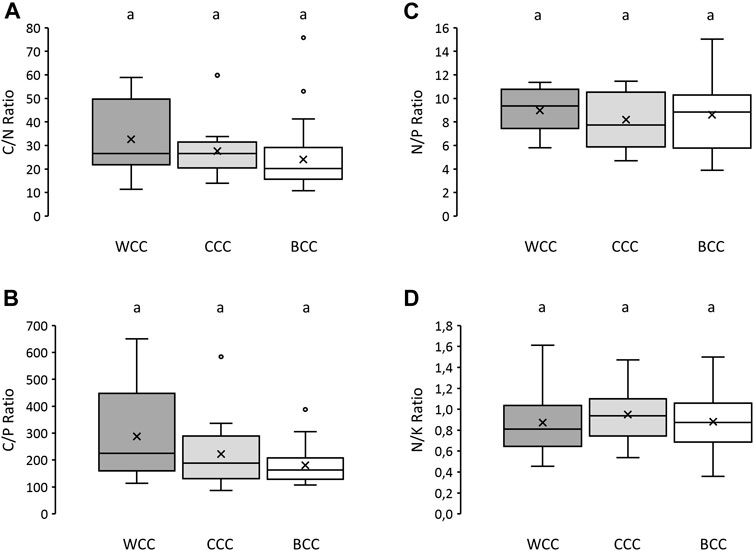
FIGURE 10. Box and whiskers plots of C/N (A), C/P (B), N/P (C) and N/K (D) ratios of the of TSCC for each of the cover crop layouts. Horizontal black line, x-shaped cross, box borders and whiskers stand for the median, the mean, quartiles and outliers. Different letters over the box-plot indicate significant differences (p < 0.05). WCC, CCC and BCC stand for olives groves where TSCC occupied the whole area, was absent under tree canopy or only occupied a strip of the inter-row area, respectively.
4 Discussion
4.1 TSCC Layout and Soil Cover
TSCC in most of the olive groves of this study followed the BCC layout, and mainly in olive groves with a tree density higher than 150 trees ha−1. This TSCC layout is the result of controlling the spontaneous vegetation under the tree rows by mechanical or chemical means. The strip width of the studied olive groves averaged 3.2 m, and was well above the 1 m width imposed by the normative, suggesting that the minimum width could be increased, at least for olive groves with a high mean slope.
In 26% of the olive groves, TSCC showed a CCC layout, mainly to facilitate olive fruit harvest and to avoid direct water and nutrient competition with the trees. Under this TSCC layout, typical of olive groves with relatively low tree density, cover crops are controlled manually and on a tree-by-tree basis mainly by using pre and post-emergency backpack herbicide sprayers or a brush cutter. Lastly, the number of olive groves in which TSCC showed a WCC layout was low and was typical of low tree density olive groves or those with higher tree densities but with irrigation.
4.2 Aboveground Net Primary Production of Temporary Spontaneous Cover Crops
Variability of the aboveground net primary productivity (ANPP) of TSCC (kg DM m2 y−1) was as high as one order of magnitude. Spatial variability of ANPP among olive groves was not unexpected as it is driven by a network of interrelated factors which include differences in: 1) pedoclimatic and landscape conditions (Taguas et al., 2017), 2) size and diversity of the seedbank, 3) current and previous management of soil and TSCC (Gómez, 2017; Vicente-Vicente, 2017), 4) main dominant species of the TSCC communities (Cruz-Ramírez et al., 2012), and 5) herbivory pressure level (Guerrero-Casado et al., 2015). Together with the variability amongst the olive groves, the intra-farm variability, expressed as a coefficient of variation, averaged 42% for the whole set of olive groves.
In addition to the high spatial variability found in this study, a relatively high inter-annual variability in the level of soil covered and ANPP are also a feature of the TSCC. For instance, in two different 3-year studies, the inter-annual variability of ANPP per hectare was about 3-fold (Repullo-Ruibérriz de Torres et al., 2012) and 20-fold (Castro et al., 2008), and mainly driven by the high inter-annual variability in precipitation which characterizes Mediterranean regions. Temporal variability of the degree of soil covered by TSCC has also been proved (Taguas et al., 2017).
The mean annual ANPP of TSCC of 1,041 kg DM ha−1 y−1 from our study is within the range of 0.65—2.53 Mg DM ha−1 y−1 obtained by Vicente-Vicente (2017) in 10 olive groves with mature TSCC. Similarly, the mean for BCC (769.5 kg DM ha−1 y−1) and WCC (1,707.4 kg DM ha−1 y−1) are consistent with results obtained by Blázquez-Carrasco et al. (2021) in three and four olive groves managed with strip and full-cover TSCC layout, respectively. Other field studies performed on only one olive grove reported generally higher figures. For instance, Repullo-Ruibérriz de Torres et al. (2012), in an olive orchard covered with spontaneous vegetation in Córdoba (Spain), found an ANPP of 2.1–6.7 Mg DM ha−1 y−1 during a study period of three agricultural years, and Rodríguez-Lizana et al. (2018) found an even wider range: 0.18—9.56 Mg DM ha−1 y−1.
The mean olive fruit production of Andalusia olive groves in the period between 2014–2019 was of about 3,558 kg fresh weight ha−1 y−1 (Junta de Andalucía, 2014; 2015a, 2016, 2017, 2018, 2019), or 1,423 kg DM ha−1 y−1 considering 40% of dry matter in olives (Zeleke et al., 2012). Therefore, the mean dry biomass production of olive groves with cover crops could increase the productivity by 73%, taking into account only the olive fruit production. If the 1.66 million hectares devoted to olive groves in Andalusia (Junta de Andalucía, 2019) allowed the development of TSCC, about 1.73 million tons of dry biomass could be produced, a figure which should be higher if the belowground biomass of the TSCC had been taken into account. This estimate, which has many uncertainties attached to it, highlights the significance of the implementation of this technically and economically viable diversification practice on many ecosystem services, at least on a regional scale.
The amount of dry biomass produced by the TSCC is a crucial factor in the C sequestration (Peregrina et al., 2014), soil properties and nutrient dynamics (Ovalle et al., 2007; Repullo-Ruibérriz de Torres et al., 2021b). The area covered and the biomass produced by TSCC in BCC olive groves were significantly smaller than that of WCC and CCC, despite the area outside the tree canopy which is potentially covered by TSCC in olive groves being very similar. Therefore, there is a significant potential to increase the ANPP of olive groves under BCC. In spite of the BCC layout strategy fulfilling the requisite to acquire the economic subsidy, narrow strips could significantly limit the potential role of TSCC in providing and boosting agroecosystem services.
4.3 Carbon Fixation by Temporary Spontaneous Cover Crops
The content of carbon in the aboveground biomass of the TSCC showed little variability. It was independent of the tree densities, cover crop layouts and pedoclimatic conditions, and the values were similar to those from other studies (Gómez-Muñoz et al., 2014; Rodríguez-Lizana et al., 2020).
On average, for the 46 olive oil orchards, the organic carbon input to the soil in 1 year through the aboveground cover crop residues was 0.42 Mg C ha−1. This average is within the range of 0.2–0.7 Mg C ha−1y−1 estimated by several researchers (Freibauer et al., 2004; Hutchinson et al., 2007) as the potential for C sequestration under scenarios of crop residue application. However, the extent to which the input of organic carbon derived from plant cover increases the soil C stock of olive orchards will ultimately depend on the decomposition rate of that organic carbon. The decomposition rate depends on many factors including plant biomass quality (e.g., C-to-N ratio and lignin and polyphenol contents), edaphic and environmental conditions and aboveground plant residue management (e.g., cover crop control method and residue displacement) (Kumar and Goh, 2000). Gómez-Muñoz et al. (2014) found that the mean percentage of the aboveground TSCC, with a C: N ratio of 30.3, remaining after 1 year of incubation on top of the soil in field conditions using the litterbag approach was 29.1%, whereas Repullo-Ruibérriz de Torres et al. (2021b), found that 55% of the carbon from TSCC remains on the field after a 1-year period. By applying the most conservative percentage (29.1%) to the mean of 0.42 Mg C ha−1 y−1, about 0.122 Mg C ha−1 y−1 of TSCC-derived organic carbon would remain in the soil after 1 year, or 202,520 Mg C (or 741,223.2 Mg of CO2) if the entire 1.66 M ha cultivated with olive groves in Andalusia permitted the development of TSCC. By 2030, the regional authorities have a commitment to reduce the annual CO2 emissions of 2005 (62,070,000 Mg CO2) by 41%, which means that by 2030, annual CO2 emissions should have reduced by 25,448,700 Mg CO2. The yearly amount of CO2 fixed by the TSCC as organic carbon, after discounting that emitted as CO2 during the TSCC residue decomposition, highlights the enormous contribution of TSCC in olive groves and other orchard type crops to climate change mitigation (Kaye and Quemada, 2019).
There is a lot of evidence regarding the increase in the stocks of soil organic carbon (SOC) due to the presence of TSCC in olive groves. For instance, in four out of the five paired TSCC versus non-TSCC comparisons, between 9.0 and 16.1 more Mg C ha−1 was stored in the top 15 cm of the soils of the TSCC olive groves after many years (>8 years) with the same management (Vicente-Vicente et al., 2017). These values were similar to the 8.4–15.0 Mg C ha−1 more SOC storage in the top 15 cm of an olive oil orchard under TSCC compared to a TSCC-free plot (Castro et al., 2008). In a short period of 3 years, different seeded cover crops, including TSCC, fixed on average 1.5 Mg C ha−1 of organic carbon (Repullo-Ruibérriz de Torres et al., 2012).
However, the increased SOC stock in soils under the cover crops treatment might not only be due to the annual inputs of organic carbon from the plant residues, but also due to a decrease in SOC losses through soil erosion (Gómez et al., 2009). In addition, the diversity of wild spontaneous plant cover might have an important impact on SOC accrual by improving the ability of soil microbial communities to rapidly process plant residues and protect them into aggregates, and by also introducing a greater diversity of organic carbon compounds into the soil, some of which may be more resistant to decomposition (Tiemann et al., 2015).
4.4 Role of Temporary Spontaneous Cover Crops in Nutrient Retention
The 19.6 kg N ha−1 y−1 that on average was, mainly, taken up from the soil by the aboveground biomass of TSCC and transformed into organic N might positively impact the N cycling of olive groves. Annual herbaceous vegetation of TSCC is likely efficient in the acquisition of nutrients when they are provided in pulses, such as peaks in soil organic matter mineralization during early spring when soil water content and soil temperature are optimal for organic matter decomposition. The highest annual growth rates, and thus nutrient uptake, of TSCC or seeded cover crops in olive groves of Mediterranean areas are usually found from mid-February to late April (Ordoñez-Fernández et al., 2018), when optimal soil water levels and daily temperatures coincide. Rainfall during late winter-early spring accounts for a relatively high proportion (about 24%) of the annual rainfall in Andalusia (RIA, 2021), and therefore the N taken up by TSCC might play an important role in reducing N losses by leaching in the olive groves. In a meta-analysis of 106 studies of 372 sites covering different countries, climatic zones and management, Abdalla et al. (2019) demonstrated that cover crops significantly decreased N leaching, although site-specific studies on olive groves are needed.
The extent to which the N uptake by the TSCC can compete with the tree demand for N, depends mainly on the magnitude and timing of the tree nutrients uptake. There are several indications that suggest that TSCC do not compete significantly with olive trees for nutrients. Typically, fertilization in olive trees is applied to the soil below the tree canopy to increase nutrient use efficiency. Therefore, it is expected that little of the soil available nutrients of the inter-row are used by trees. On the other hand, the highest tree demand for N and P takes place in late April–June and in October (Cadahía, 2005; García-Ruiz et al., 2011), several weeks after the highest TSCC demands for nutrients. Furthermore, the N balances in two olive groves of Fernández-Escobar et al. (2012) revealed that the usual N application rate of 1 kg N tree−1 y−1 (as urea), split in two applications in the early spring, resulted in a very positive N balance. This, hence, highlighted that the rate of N fertilizer application was much higher than that demanded by the tree, and therefore cover crops are expected to have little competition for N.
On the other hand, it is rather likely that the main sources of available N and P taken up by TSCC are the N and P which are mineralized from the soil organic matter. Fernández-Escobar et al. (2012) estimated that 44.8 and 69.9 kg N ha−1 y−1 were available during the decomposition of soil organic matter in two olive groves, whereas Gómez-Muñoz et al. (2015) found values between 80 and 220 kg N ha−1 y−1 for the top 20 cm of soil in two olive groves with soil organic matter levels of 3%. These values are more than double the mean nitrogen taken up by TSCC in this study. Lastly, the main indications that TSCC do not compete with the olive trees for nutrients come from the assessment of the olive fruit production and olive oil quality in mature olive groves with and without cover crops. Zuazo et al. (2020) demonstrated during four consecutive years, that the olive yield did not differ significantly between olive groves without cover crops and comparable olive groves with TSCC or seeded cover crops layout in strips or in the entire orchard. In this regard, Sastre et al. (2016) found in an olive plantation of the drought-resistant cultivar Cornicabra that cover crops had not reduced fruit or oil yield, neither in heavy nor in low yield years. However, in a very high density olive grove (Gucci et al., 2012) and in an intensive young olive tree grove (Caruso et al., 2011), a significant decrease in the fruit yield under cover crops has been reported.
The fact that TSCC in 50% of the studied olive groves had a C/N ratio lower than 22.2 indicates that in general, TSCC grow up with little N limitation. Xu et al. (2020) found in a meta-analysis with 2236 paired observations from 123 published studies to investigate the responses of foliar C/N ratio to experimental N addition that the foliar C:N ratio declined with N availability, and Rodríguez et al. (2013) found that the aboveground biomass of the spontaneous cover crops in unfertilized and fertilized (60 kg N ha−1y−1) soils of the inter-row area of an olive grove in Portugal had a C/N ratio of 52.1 and 25.9, respectively. The relatively low C:N ratio of the aboveground biomass of the TSCC of our study indicates that the environmental conditions were suitable for plant growth and N was not limited, and therefore plants compete more for light (and thus C), exhibiting low C/N ratios (Zhang et al., 2019). According to Trinsoutrot et al. (2000), C/N ratios above 24, a value similar to that of our study, imply a net N immobilization. This could slightly affect the availability of N for olive trees.
TSCC in 75% of the examined olive groves had a N/P ratio lower than 10.6. There are many factors responsible for a given N/P ratio. Optimal N/P ratios depend on species, growth rate, plant age and plant parts. At vegetation level, N/P ratios <10 often correspond to a growth lacking P limitation (Güsewell, 2004), as shown by short-term fertilization experiments. The generalized low C/N and N/P ratios of the aboveground biomass of the TSCC for most of the olive groves of this study suggest no N or P limitation during their growth.
In summary, there are numerous indications that the 19.6 kg N ha−1 y−1, 2.5 kg P ha−1 y−1 and 24.3 kg K ha−1 y−1 accumulated on average in the aboveground biomass of the TSCC of the 46 olive groves in this study, show that the TSCC do not compete significantly with the olive trees for nutrients, but might instead play an important role in reducing the N, P and K that are prone to be lost by leaching, soil erosion and runoff. The rapid growth rate typical of the herbaceous vegetation of the TSCC permits the interception and storage of highly mobile nutrients in its biomass that will then be progressively released at rates which are more in accordance to those required for acquisition by olive trees, contributing to more efficient nutrient cycling.
Assuming that annually, 19.6 kg N ha−1, 2.5 kg P ha−1 and 24.3 kg K ha−1 are retained in the olive groves due to the TSCC, and TSCC are maintained in the 1.6 million hectares of olive groves of Andalusia, then about 29,400, 3,750 and 36,450 Mg of N, P and K could be saved, avoiding further environmental and economic costs. For instance, this amount of N is the equivalent of 29 million euros in N fertilizers (assuming an average price of 1 € kg−1 N). This estimate, which has many uncertainties, highlights the significance of the implementation of this technically and economically viable diversification practice on nutrient retention at a regional scale. In addition, the N taken up by the TSCC might help to alleviate the nutritional problems in olive-growing as a result of the overuse of N fertilizers, as has been reported for other fruit crops (Weibaum et al., 1992).
The extent to which TSCC have a significant role in retaining nutrients within the olive groves greatly depends on the area covered, since the annual rates of N, P and K taken up by TSCC growing in strips were almost half of that taken up in olive groves with TSCC growing in the entire area.
5 Conclusion
The variability of the aboveground net annual primary production of temporary spontaneous cover crops was as high as one order of magnitude. Hence, to model the beneficial effects of diversification in olive groves on climate change mitigation and nutrient retention on large spatial scales, extensive sampling that presents a high variability should be undertaken, as has been done in this study.
The 1.53 Mg of CO2 ha−1 y−1 that was fixed annually on average by the aboveground biomass of temporary spontaneous cover crops could contribute significantly to the regional commitments of CO2 reduction if all the areas devoted to olive groves implement this diversification strategy. Through the amounts of N, P and K accumulated into the aboveground biomass, the temporary spontaneous cover crops were also an adequate strategy in reducing the nutrient loss prone to occur in the olive orchards and in boosting the soil-plant internal nutrient loop, thus enhancing the nutrient retention within the olive groves.
This study proves that temporary spontaneous cover crops in olive orchards are a sound diversification strategy with the potential to contribute significantly to the increase in productivity in olive groves, to climate change mitigation and to nutrient retention. The level of contribution was highly dependent on the cover crop layout model and was lower when cover crops were distributed in strips in the inter-rows area. Therefore, by increasing the area that the inter-row covered, the potential contribution of temporary spontaneous cover crops could be enhanced further.
Data Availability Statement
The raw data supporting the conclusions of this article will be made available by the authors, without undue reservation.
Author Contributions
Conceptualization, RG-R; Sampling and analyses, MT-C, PD, JH, and JC; formal analysis and visualization: PD; writing—original draft preparation, MT-C, PD, and RG-R; writing—review and editing, MT-C, JC, PD, and RG-R; funding acquisition, RGR. All authors have read and agreed to the published version of the manuscript.
Funding
This research was funded by the PRIMA-H2020 project SUSTAINOLIVE (grant no 1811) and co-supported by the European Union via FEDER funds, “FEDER de Andalucía 2014–2020”.
Conflict of Interest
The authors declare that the research was conducted in the absence of any commercial or financial relationships that could be construed as a potential conflict of interest.
Publisher’s Note
All claims expressed in this article are solely those of the authors and do not necessarily represent those of their affiliated organizations, or those of the publisher, the editors and the reviewers. Any product that may be evaluated in this article, or claim that may be made by its manufacturer, is not guaranteed or endorsed by the publisher.
Acknowledgments
The authors would like to thank Gustavo Ruiz Cátedra and Jose Manuel Ramírez Pardo for their work in processing the samples. The main authors, MT-C and PD, are grateful for the opportunity to benefit from a pre-doctoral contract of university professor in training (FPU scholarship), granted by the Spanish Ministry of Universities.
Supplementary Material
The Supplementary Material for this article can be found online at: https://www.frontiersin.org/articles/10.3389/fenvs.2022.868410/full#supplementary-material
Abbreviations
ANPP, annual net primary production; BCC, TSCC in bands of a given width in the inter-row area; CCC, TSCC in the whole farm except the area below the tree canopy; DM, dry matter; SOC, soil organic carbon; TSCC, temporary spontaneous cover crops; WCC, TSCC in the whole farm.
References
Abdalla, M., Hastings, A., Cheng, K., Yue, Q., Chadwick, D., Espenberg, M., et al. (2019). A Critical Review of the Impacts of Cover Crops on Nitrogen Leaching, Net Greenhouse Gas Balance and Crop Productivity. Glob. Change Biol. 25, 2530–2543. doi:10.1111/gcb.14644
Almagro, M., and Martínez-Mena, M. (2014). Litter Decomposition Rates of Green Manure as Affected by Soil Erosion, Transport and Deposition Processes, and the Implications for the Soil Carbon Balance of a Rainfed Olive Grove under a Dry Mediterranean Climate. Agric. Ecosyst. Environ. 196, 167–177. doi:10.1016/J.AGEE.2014.06
Beaufoy, G. (2001). EU Policies for Olive Farming. Unsustainable on All Counts. Brussels: WWF/Bird Life International.
Blázquez-Carrasco, A., Gómez-Giráldez, P., Castro-Rodríguez, J., Colombo, S., Carpintero, M. P., and González-Dugo, M. P. (2021). Estimation of Olive Groves Cover Crops Net Primary Productivity Using Remote Sensing Data. Proc. SPIE 11856Remote Sens. Agric. Ecosyst. Hydrology XXIII, 118560S. doi:10.1117/12.2601181
Cadahía, C. (2005). Fertirrigación. Cultivos Hortícolas, Frutales Y Ornamentales. España: MundiPrensa.
Caruso, G., Gucci, R., and Sifola, M. I. (2011). Soil Management Affects Yield Components of Young Olive Trees under Deficit Irrigation. Acta Hortic. 924, 219–223. doi:10.17660/ActaHortic.2011.924.27
Castro, J., Fernández-Ondoño, E., Rodríguez, C., Lallena, A. M., Sierra, M., and Aguilar, J. (2008). Effects of Different Olive-Grove Management Systems on the Organic Carbon and Nitrogen Content of the Soil in Jaén (Spain). Soil Tillage Res. 98 (1), 56–67. doi:10.1016/j.still.2007.10.002
Commission of European Communities (CEC), (1985). Soil Map of the European Communities. Luxemburg: Office for Official Publications of the European Communities. [Map]. 1:1,000,000.
Cruz-Ramírez, M., Hervás-Martínez, C., Jurado-Expósito, M., and López-Granados, F. (2012). A Multi-Objective Neural Network Based Method for Cover Crop Identification from Remote Sensed Data. Expert Syst. Appl. 39 (11), 10038–10048. doi:10.1016/j.eswa.2012.02.046
de Andalucía, J. (2015b). Análisis de las plantaciones de olivar en Andalucía: Año 2015. Encuesta sobre Superficies y Rendimientos de Cultivos en España (ESYRCE). Avabilableat: https://www.juntadeandalucia.es/organismos/agriculturaganaderiapescaydesarrollosostenible/servicios/estudios-informes/detalle/129619.html (Accessed November 20, 2021).
de Andalucía, J. (2014). Anuario de estadísticas agrarias y pesqueras año 2014. Avabilableat: https://www.juntadeandalucia.es/organismos/agriculturaganaderiapescaydesarrollosostenible/servicios/estadistica-cartografia/anuarios.html (Accessed November 20, 2021).
de Andalucía, J. (2015a). Anuario de estadísticas agrarias y pesqueras año 2015. Avabilableat: https://www.juntadeandalucia.es/organismos/agriculturaganaderiapescaydesarrollosostenible/servicios/estadistica-cartografia/anuarios.html (Accessed November 20, 2021).
de Andalucía, J. (2016). Anuario de estadísticas agrarias y pesqueras año 2016. Avabilableat: https://www.juntadeandalucia.es/organismos/agriculturaganaderiapescaydesarrollosostenible/servicios/estadistica-cartografia/anuarios.html (Accessed November 20, 2021).
de Andalucía, J. (2017). Anuario de estadísticas agrarias y pesqueras año 2017. Avabilableat: https://www.juntadeandalucia.es/organismos/agriculturaganaderiapescaydesarrollosostenible/servicios/estadistica-cartografia/anuarios.html (Accessed November 20, 2021).
de Andalucía, J. (2018). Anuario de estadísticas agrarias y pesqueras año 2018. Avabilableat: https://www.juntadeandalucia.es/organismos/agriculturaganaderiapescaydesarrollosostenible/servicios/estadistica-cartografia/anuarios.html (Accessed November 20, 2021).
de Andalucía, J. (2007). Cubiertas vegetales en olivar. Consejería de Agricultura y Pesca. Avabilableat: https://www.juntadeandalucia.es/sites/default/files/2020-04/1337161293CUBIERTAS_VEGETALES_9-07_mod_por_JMD.pdf (Accessed November 20, 2021).
Durán Zuazo, V. H., and Rodríguez Pleguezuelo, C. R. (2008). Soil-erosion and Runoff Prevention by Plant Covers. A Review. A Rev. Agron. sustain. Dev. 28, 65–86. doi:10.1051/agro:2007062
European Commission (2021). List of Potential Agricultural Practices that Eco-Schemes Could Support. Avabilableat: https://ec.europa.eu/info/news/commission-publishes-list-potential-eco-schemes-2021-jan-14_en#moreinfo (Accessed November 20, 2021).
Fernández-Escobar, R., García-Novelo, J. M., Molina-Soria, C., Parra, M. A., and Parra, M. A. (2012). An Approach to Nitrogen Balance in Olive Orchards. Sci. Hortic. 135, 219–226. doi:10.1016/j.scienta.2011.11.036
Finney, D. M., White, C. M., and Kaye, J. P. (2016). Biomass Production and Carbon/Nitrogen Ratio Influence Ecosystem Services from Cover Crop Mixtures. Agron. J. 108 (1), 39–52. doi:10.2134/agronj15.0182
Fraga, H., Moriondo, M., Leolini, L., and Santos, J. A. (2021). Mediterranean Olive Orchards under Climate Change: A Review of Future Impacts and Adaptation Strategies. Agronomy 11, 56. doi:10.3390/agronomy11010056
Francaviglia, R., and Vicente-Vicente, J. L. (2021). “Cover Cropping,” in Food and Agriculture Organization of the United Nations, Recarbonizing Global Soils: A Technical Manual of Recommended Sustainable Soil Management - Cropland, Grassland, Integrated Systems and Farming Approaches - Practices Overview (Rome, IT: FAO), 3, 2–13.
Freibauer, A., Rounsevell, M. D. A., Smith, P., and Verhagen, J. (2004). Carbon Sequestration in the Agricultural Soils of Europe. Geoderma 122, 1–23. doi:10.1016/j.geoderma.2004.01.021
García-Ruiz, R., Gómez–Muñoz, B., Carreira, J. A., and Hinojosa, B. (2011). “La fertilización en el olivar ecológico,” in El Olivar Ecológico. Editor J. de Andalucía, 95–131.
Gómez, J. A., Battany, M., Renschler, C. S., and Fereres, E. (2003). Evaluating the Impact of Soil Management on Soil Loss in Olive Orchards. Soil use manag. 19, 127–134. doi:10.1111/j.1475-2743.2003.tb00292.x10.1079/sum2002179
Gómez, J. A., Campos, M., Guzmán, G., Castillo-Llanque, F., Vanwalleghem, T., Lora, Á., et al. (2018). Soil Erosion Control, Plant Diversity, and Arthropod Communities under Heterogeneous Cover Crops in an Olive Orchard. Environ. Sci. Pollut. Res. 25, 977–989. doi:10.1007/s11356-016-8339-9
Gómez, J. A., Guzmán, M. G., Giráldez, J. V., and Fereres, E. (2009). The Influence of Cover Crops and Tillage on Water and Sediment Yield, and on Nutrient, and Organic Matter Losses in an Olive Orchard on a Sandy Loam Soil. Soil Tillage Res. 106, 137–144. doi:10.1016/j.still.2009.04.008
Gómez, J. A. (2017). Sustainability Using Cover Crops in Mediterranean Tree Crops, Olives and Vines - Challenges and Current Knowledge. HunGeoBull 66, 13–28. doi:10.15201/hungeobull.66.1.2
Gómez-Muñoz, B., Hatch, D. J., Bol, R., and García-Ruiz, R. (2014). Nutrient Dynamics during Decomposition of the Residues from a Sown Legume or Ruderal Plant Cover in an Olive Oil Orchard. Agric. Ecosyst. Environ. 184, 115–123. doi:10.1016/j.agee.2013.11.020
Gómez-Muñoz, B., Hinojosa, M. B., and García-Ruiz, R. (2015). In Situ net N Mineralisation and Nitrification under Organic and Conventionally Managed Olive Oil Orchards. Nutr. Cycl. Agroecosyst 101 (2), 223–239. doi:10.1007/s10705-015-9672-y
Gucci, R., Caruso, G., Bertolla, C., Urbani, S., Taticchi, A., Esposto, S., et al. (2012). Changes of Soil Properties and Tree Performance Induced by Soil Management in a High-Density Olive Orchard. Eur. J. Agron. 41, 18–27. doi:10.1016/j.eja.2012.03.002
Guerrero-Casado, J., Carpio, A. J., Prada, L. M., and Tortosa, F. S. (2015). The Role of Rabbit Density and the Diversity of Weeds in the Development of Cover Crops in Olive Groves. Span. Span. J. Agric. Res. 13 (3), e03SC01. doi:10.5424/sjar/2015133-7022
Güsewell, S. (2004). N : P Ratios in Terrestrial Plants: Variation and Functional Significance. New Phytol. 164 (2), 243–266. doi:10.1111/j.1469-8137.2004.01192.x
Hutchinson, J. J., Campbell, C. A., and Desjardins, R. L. (2007). Some Perspectives on Carbon Sequestration in Agriculture. Agric. For. Meteorology 142, 288–302. doi:10.1016/j.agrformet.2006.03.030
Infante-Amate, J., and de Molina, M. G. (2013). The Socio-Ecological Transition on a Crop Scale: The Case of Olive Orchards in Southern Spain (1750-2000). Hum. Ecol. 41 (6), 961–969. doi:10.1007/s10745-013-9618-4
Junta de Andalucía (2012). Red de Información Ambiental de Andalucía. WMS Mapa de Suelos de Andalucía. [Map]. 1:400,000. Sevilla, Spain: Consejería de Medio Ambiente y Ordenación del Territorio.
Junta de Andalucía (2019). Anuario de estadísticas agrarias y pesqueras año 2019. Avabilable at: https://www.juntadeandalucia.es/organismos/agriculturaganaderiapescaydesarrollosostenible/servicios/estadistica-cartografia/anuarios.html (Accessed November 20, 2021).
Kumar, K., and Goh, K. M. (2000). Biological Nitrogen Fixation, Accumulation of Soil Nitrogen and Nitrogen Balance for White Clover (Trifolium Repens L.) and Field Pea (Pisum Sativum L.) Grown for Seed. Field Crops Res. 68 (1), 49–59. doi:10.1016/S0378-4290(00)00109-X
Laflen, J. M., Foster, G. R., and Onstad, C. A. (1985). “Simulation of Individual-Storm Soil Loss for Modeling the Impact of Soil Erosion on Crop Productivity,” in Soil Erosion and Conservation. Editors S. A. El-Swaify, W. C. Moldenhauer, and A. Lo (Ankeny,IL: Soil Cons. Soc. Am), 285–295.
Lima-Cueto, F. J., Blanco-Sepúlveda, R., Gómez-Moreno, M. L., and Galacho-Jiménez, F. B. (2019). Using Vegetation Indices and a UAV Imaging Platform to Quantify the Density of Vegetation Ground Cover in Olive Groves (Olea Europaea L.) in Southern Spain. Remote Sens. 11 (21), 2564. doi:10.3390/rs11212564
Liu, R., Thomas, B. W., Shi, X., Zhang, X., Wang, Z., and Zhang, Y. (2021). Effects of Ground Cover Management on Improving Water and Soil Conservation in Tree Crop Systems: A Meta-Analysis. Catena 199, 105085. doi:10.1016/j.catena.2020.105085
López-Vicente, M., Gómez, J. A., Guzmán, G., Calero, J., and García-Ruiz, R. (2021). The Role of Cover Crops in the Loss of Protected and Non-protected Soil Organic Carbon Fractions Due to Water Erosion in a Mediterranean Olive Grove. Soil Tillage Res. 213, 105119–119. doi:10.1016/j.still.2021.105119
Loumou, A., and Giourga, C. (2003). Olive Groves: “The Life and Identity of the Mediterranean”. Agric. Hum. Values 20, 87–95. doi:10.1023/A:1022444005336
MAGRAMA (Ministerio de Agricultura, Alimentación y Medio Ambiente) (2014). Real Decreto 1078/2014, de 19 de Diciembre, por el que se Establecen las Normas de la Condicionalidad que Deben Cumplir los Beneficiarios que Reciban Pagos Directos, Determinadas Primas Anuales de Desarrollo Rural, o Pagos en Virtud de Determinados Programas de Apoyo al Sector VitivinícolaBOE 307: 103644–103849. Avabilableat: https://www.boe.es/eli/es/rd/2014/12/19/1078 (Accessed November 20, 2021).
MAPA (Ministerio de Agricultura, Pesca y Alimentación) (2020a). Encuesta sobre Superficies y Rendimientos de Cultivos. 2020. Avabilableat: https://www.mapa.gob.es/es/estadistica/temas/estadisticas-agrarias/totalespanayccaa2020_tcm30-553610.pdf (Accessed November 20, 2021).
MAPA (Ministerio de Agricultura, Pesca y Alimentación) (2020b). Encuesta sobre Superficies y Rendimientos de Cultivos. 2020. Análisis de las Técnicas de Mantenimiento del Suelo y de los Métodos de Siembra en España. Avabilableat: https://www.mapa.gob.es/es/estadistica/temas/estadisticas-agrarias/cubiertas2020_tcm30-526244.pdf (Accessed November 20, 2021).
Morugán-Coronado, A., Linares, C., Gómez-López, M. D., Faz, Á., and Zornoza, R. (2020). The Impact of Intercropping, Tillage and Fertilizer Type on Soil and Crop Yield in Fruit Orchards under Mediterranean Conditions: A Meta-Analysis of Field Studies. Agric. Syst. 178, 102736. doi:10.1016/j.agsy.2019.102736
Ordóñez-Fernández, R., Repullo-Ruibérriz de Torres, M. A., Márquez-García, J., Moreno-García, M., Carbonell-Bojollo, R. M., and Carbonell-Bojollo, R. M. (2018). Legumes Used as Cover Crops to Reduce Fertilisation Problems Improving Soil Nitrate in an Organic Orchard. Eur. J. Agron. 95, 1–13. doi:10.1016/j.eja.2018.02.001
Ortega, M., Pascual, S., Elena-Rosselló, R., and Rescia, A. J. (2020). Land-use and Spatial Resilience Changes in the Spanish Olive Socio-Ecological Landscape. Appl. Geogr. 117, 102171. doi:10.1016/j.apgeog.2020.102171
Ovalle, C., del Pozo, A., Lavín, A., and Hirzel, J. (2007). Cover Crops in Vineyards: Performance of Annual Forage Legume Mixtures and Effects on Soil Fertility. Agric. Técnica 67, 384–392. doi:10.4067/s0365-28072007000400006
Peña-Barragán, J. M., Jurado-Expósito, M., López-Granados, F., Atenciano, S., Sánchez-de la Orden, M., Garcı́a-Ferrer, A., et al. (2004). Assessing Land-Use in Olive Groves from Aerial Photographs. Agric. Ecosyst. Environ. 103, 117–122. doi:10.1016/j.agee.2003.10.014
Peregrina, F., Pérez-Álvarez, E. P., and García-Escudero, E. (2014). The Short Term Influence of Aboveground Biomass Cover Crops on C Sequestration and β-glucosidase in a Vineyard Ground under Semiarid Conditions. Span. J. Agric. Res. 12 (4), 1000–1007. doi:10.5424/sjar/2014124-5818
Ravindranath, N. H., and Ostwald, M. (2008). Carbon Inventory Methods. Handbook for Greenhouse Gas Inventory, Carbon Mitigation and Roundwood Production Projects. Dordrecht: Springer. doi:10.1007/978-1-4020-6547-7
Repullo-Ruibérriz de Torres, M. A., Carbonell-Bojollo, R., Alcántara-Braña, C., Rodríguez-Lizana, A., and Ordóñez-Fernández, R. (2012). Carbon Sequestration Potential of Residues of Different Types of Cover Crops in Olive Groves under Mediterranean Climate. Span. J. Agric. Res. 10 (3), 649–661. doi:10.5424/sjar/2012103-562-11
Repullo-Ruibérriz de Torres, M. A., Carbonell-Bojollo, R. M., Moreno-García, M., Ordóñez-Fernández, R., and Rodríguez-Lizana, A. (2021a). Soil Organic Matter and Nutrient Improvement through Cover Crops in a Mediterranean Olive Orchard. Soil Tillage Res. 210, 104977–977. doi:10.1016/j.still.2021.104977
Repullo-Ruibérriz de Torres, M. A., Moreno-García, M., Ordóñez-Fernández, R., Rodríguez-Lizana, A., Cárceles Rodríguez, B., García-Tejero, I. F., et al. (2021b). Cover Crop Contributions to Improve the Soil Nitrogen and Carbon Sequestration in Almond Orchards (SW Spain). Agronomy 11 (2), 387. doi:10.3390/agronomy11020387
Repullo-Ruibérriz de Torres, M. A., Ordóñez-Fernández, R., Giráldez, J. V., Márquez-García, J., Laguna, A., and Carbonell-Bojollo, R. (2018). Efficiency of Four Different Seeded Plants and Native Vegetation as Cover Crops in the Control of Soil and Carbon Losses by Water Erosion in Olive Orchards. Land Degrad. Dev. 29, 2278–2290. doi:10.1002/ldr.3023
RIA (Red de información agroclimática de Andalucía) (2021). Precipitación [Data File]. Avabilableat: https://www.juntadeandalucia.es/agriculturaypesca/ifapa/riaweb/web/inicio_estaciones (Accessed November 20, 2021).
Rodrigues, M. Â., Correia, C. M., Claro, A. M., Ferreira, I. Q., Barbosa, J. C., Moutinho-Pereira, J. M., et al. (2013). Soil Nitrogen Availability in Olive Orchards after Mulching Legume Cover Crop Residues. Sci. Hortic. 158, 45–51. doi:10.1016/j.scienta.2013.04.035
Rodríguez-Lizana, A., Ordóñez, R., Espejo-Pérez, A. J., and González, P. (2007). Plant Cover and Control of Diffuse Pollution from P in Olive Groves. Water Air Soil Pollut. 181, 17–34. doi:10.1007/s11270-006-9273-0
Rodríguez-Lizana, A., Repullo-Ruibérriz de Torres, M. A., Carbonell-Bojollo, R., Alcántara, C., and Ordóñez-Fernández, R. (2018). Brachypodium Distachyon, Sinapis Alba, and Controlled Spontaneous Vegetation as Groundcovers: Soil Protection and Modeling Decomposition. Agric. Ecosyst. Environ. 265, 62–72. doi:10.1016/j.agee.2018.05.012
Rodríguez-Lizana, A., Repullo-Ruibérriz de Torres, M. Á., Carbonell-Bojollo, R., Moreno-García, M., and Ordóñez-Fernández, R. (2020). Study of C, N, P and K Release from Residues of Newly Proposed Cover Crops in a Spanish Olive Grove. Agronomy 10, 1041. doi:10.3390/agronomy10071041
Rosa-Schleich, J., Loos, J., Mußhoff, O., and Tscharntke, T. (2019). Ecological-economic Trade-Offs of Diversified Farming Systems - A Review. Ecol. Econ. 160, 251–263. doi:10.1016/J.ECOLECON.2019.03.002
Sastre, B., Barbero-Sierra, C., Bienes, R., Marques, M. J., and García-Díaz, A. (2017). Soil Loss in an Olive Grove in Central Spain under Cover Crops and Tillage Treatments, and Farmer Perceptions. J. Soils Sediments. 17, 873–888. doi:10.1007/s11368-016-1589-9
Sastre, B., Pérez-Jiménez, M. Á., Bienes, R., García-Díaz, A., and de Lorenzo, C. (2016). The Effect of Soil Management on Olive Yield and VOO Quality in a Rainfed Olive Grove of Central Spain. J. Chem. 2016, 1–15. doi:10.1155/2016/4974609
Taguas, E. V., Vanderlinden, K., Pedrera-Parrilla, A., Giráldez, J. V., and Gómez, J. A. (2017). Spatial and Temporal Variability of Spontaneous Grass Cover and its Influence on Sediment Losses in an Extensive Olive Orchard Catchment. Catena 157, 58–66. doi:10.1016/j.catena.2017.05.017
Tiemann, L. K., Grandy, A. S., Atkinson, E. E., Marin-Spiotta, E., and McDaniel, M. D. (2015). Crop Rotational Diversity Enhances Belowground Communities and Functions in an Agroecosystem. Ecol. Lett. 18, 761–771. doi:10.1111/ele.12453
Trinsoutrot, I., Recous, S., Bentz, B., Linères, M., Chèneby, D., and Nicolardot, B. (2000). Biochemical Quality of Crop Residues and Carbon and Nitrogen Mineralization Kinetics under Nonlimiting Nitrogen Conditions. Soil Sci. Soc. Am. J. 64, 918–926. doi:10.2136/sssaj2000.643918x
Unger, P. W., Stewart, B. A., Parr, J. F., and Singh, R. P. (1991). Crop Residue Management and Tillage Methods for Conserving Soil and Water in Semi-arid Regions. Soil Tillage Res. 20, 219–240. doi:10.1016/0167-1987(91)90041-U
Vanwalleghem, T., Amate, J. I., de Molina, M. G., Fernández, D. S., and Gómez, J. A. (2011). Quantifying the Effect of Historical Soil Management on Soil Erosion Rates in Mediterranean Olive Orchards. Agric. Ecosyst. Environ. 142, 341–351. doi:10.1016/j.agee.2011.06.003
Vicente-Vicente, J. L. (2017). Soil Organic Carbon Sequestration in Olive Groves of Andalusia: Effect of the Managements on Soil Organic Carbon Dynamics. Jaén, Spain: University of Jaén. [PhD Thesis]. [Jaén (SP)].
Vilar, J., and Pereira, J. E. (2018). La Olivicultura Internacional. Difusión Histórica, Análisis Estratégico Y Visión Descriptiva. Jaén, Spain: Fundación Caja Rural de Jaén.
Xu, S., Sardans, J., Zhang, J., and Peñuelas, J. (2020). Variations in Foliar Carbon:nitrogen and Nitrogen:phosphorus Ratios under Global Change: a Meta-Analysis of Experimental Field Studies. Sci. Rep. 10, 12156. doi:10.1038/s41598-020-68487-0
Zeleke, K., Mailer, R., Eberbach, P., and Wünsche, J. (2012). Oil Content and Fruit Quality of Nine Olive (Olea Europaea L.) Varieties Affected by Irrigation and Harvest Times. N. Z. J. Crop Hortic. Sci. 40 (4), 241–252. doi:10.1080/01140671.2012.662159
Zhang, J., He, N., Liu, C., Xu, L., Chen, Z., Li, Y., et al. (2020). Variation and Evolution of C:N Ratio Among Different Organs Enable Plants to Adapt to N-Limited Environments. Glob. Change Biol. 26 (4), 2534–2543. doi:10.1111/gcb.14973
Keywords: Olive orchards, Cover crops, CO2 fixation, nutrient retention, Diversification
Citation: Torrús-Castillo M, Domouso P, Herrera-Rodríguez JM, Calero J and García-Ruiz R (2022) Aboveground Carbon Fixation and Nutrient Retention in Temporary Spontaneous Cover Crops in Olive Groves of Andalusia. Front. Environ. Sci. 10:868410. doi: 10.3389/fenvs.2022.868410
Received: 03 February 2022; Accepted: 04 May 2022;
Published: 01 June 2022.
Edited by:
María Almagro, Center for Edaphology and Applied Biology of Segura, (CSIC), SpainReviewed by:
Carmen Segura, Rothamsted Research, United KingdomAsma Jebari, Basque centre for climate change, Spain
Copyright © 2022 Torrús-Castillo, Domouso, Herrera-Rodríguez, Calero and García-Ruiz. This is an open-access article distributed under the terms of the Creative Commons Attribution License (CC BY). The use, distribution or reproduction in other forums is permitted, provided the original author(s) and the copyright owner(s) are credited and that the original publication in this journal is cited, in accordance with accepted academic practice. No use, distribution or reproduction is permitted which does not comply with these terms.
*Correspondence: Roberto García-Ruiz, cmdhcmNpYUB1amFlbi5lcw==
†These authors have contributed equally to this work