- 1College of Economics and Management, Hefei University, Hefei, China
- 2PetroChina (Tianjin) International Petroleum Exploration & Development Technology Co, Ltd, Tianjin, China
- 3Atmospheric Sciences Research Center, University at Albany, State University of New York, Albany, NY, United States
- 4Jiangsu Key Laboratory of Atmospheric Environment Monitoring and Pollution Control, School of Environmental Science and Engineering, Nanjing University of Information Science and Technology, Nanjing, China
- 5School of Biology, Food and Environment, Hefei University, Hefei, China
The technological innovation of horizontal drilling and high-volume hydraulic fracturing has promoted the development of unconventional natural gas (UNG) production worldwide, and hence has aroused public concern about the air pollution it may bring about. In this study, we have provided (1) an overview of the study on air pollutants from UNG emissions in the USA, focusing on both the air pollutant characterization and their related observation technologies/platforms; and (2) the potential air quality measurements of UNG development emerging in China. This study will provide useful information for Chinese environmental researchers and the local governments to deal with related air quality issues.
Introduction
Nature gas is accounting for more percentage of the world’s energy supply from 20% in 2000 to 23% in 2019 (https://www.iea.org), due to its relatively less environmental impact and more economic advantages compared to oil and coal (Finkel et al., 2013). To seek “energy independence,” unconventional natural gas (hereafter referred to as UNG, which originates from shale, sandstone, etc.) production has been promoted extensively in the USA during recent years at the major basins containing shale gas (i.e., Marcellus Shale Play at Appalachian Basin; Fayetteville Shale Play at Arkoma Basin; Barnett Shale Play at Ft. Worth Basin, etc. Figure 1), benefiting from the significant technological innovation of the horizontal drilling and the high-volume hydraulic fracturing techniques (Finkel et al., 2013; Wang and Krupnick, 2013; Moore et al., 2014; Allshouse et al., 2017; Helmig, 2020). From 2007 to 2019, the annual UNG production increased from about 560 × 108 m3 to 7900 × 108 m3 with an enhancement factor of about 14 (http://www.eia.gov/naturalgas/). However, the surge of UNG development is accompanied by a significant increase in air pollutants emissions (McKenzie et al., 2012, Shonkoff et al., 2014; Allen, 2016; Kort et al., 2016), including carbon monoxide (CO), nitrogen oxides (NOx), volatile organic compounds (VOCs, Gilman et al., 2013; Bunch et al., 2014; Helmig, 2020; Pétron, et al., 2014), primary particulate matter (PM), and their related secondary productions (i.e., ozone and secondary organic aerosols (SOA), Kemball-Cook et al., 2010; Pacsi et al., 2015; Lee et al., 2015; Liggio et al., 2016; Cheadle et al., 2017; Pozzer et al., 2020). Public concern about the air pollutants has risen with the growth of the UNG development, which has expanded into urban residential areas and has promoted related scientific studies (Pacsi et al., 2015; Adgate et al., 2014; Field et a., 2014; Vinciguerra et al., 2015; McMullin et al., 2018; McKenzie et al., 2019).
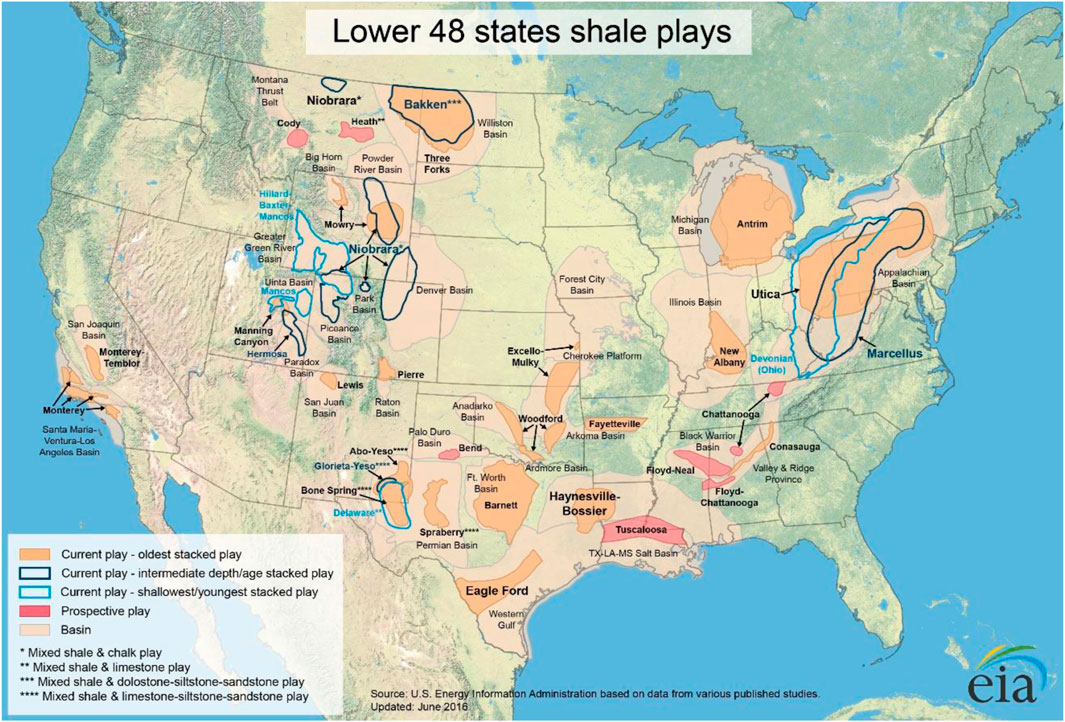
FIGURE 1. The U.S. lower 48 states shale oil and natural gas maps [source: U.S. Energy Information Administration (June 2016)].
China has the most abundant shale gas resources with a total amount of 31 × 1012 m3, which is about 1.5 times of global shale gas reserves of the United States (https://www.eia.gov/analysis/studies/worldshalegas/). Although UNG production has quickly picked up in China, the annual UNG production of the USA is still about 40 times that of China in 2020 (7900×108 vs 200 × 108 m3 (Sun et al., 2021). Under the most recent Chinese government energy policy, UNG production is expected to increase continually and reach 2200 × 108 m3 in 2040 (Zou et al., 2017; IEA, 2020). With the surge of UNG production, the UNG wells will inevitably expand into human communities, especially in the Sichuan Basin (Ma and Xie, 2018; He et al., 2020; Nie et al., 2020, Figure 2) which is also a population cluster with two megacities-Chongqing and Chengdu, and the subsequent air quality issues could occur. Taking the fact into consideration, we organized this study to summarize the main air pollution issues detected during the USA UNG developments and their related measurement technologies, which can provide useful guidance for Chinese environmental researchers and the local governments to better understand the air pollution-related to UNG developments.
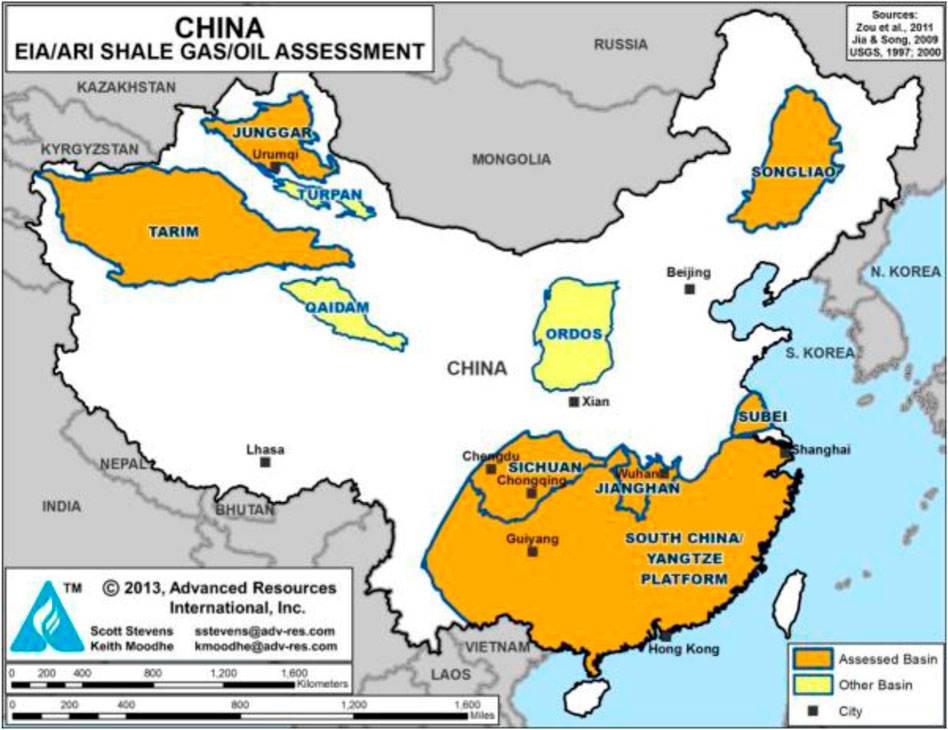
FIGURE 2. China’s most prospective shale gas basins [Source: U.S. Energy Information Administration (September 2015)].
Air pollutants from UNG production in the USA
The air pollutants caused by UNG production are mainly focused on VOCs species [including methane and Non-methane volatile organic compounds (NMVOCs)] and their related secondary productions (including Ozone and SOA) due to their clear effect on the radiative forcing (methane) and on the human health (NMVOCs, Ozone and SOA).
Methane
Methane (CH4) is the second-most anthropogenic greenhouse gas (after CO2) for its effect on radiative forcing, with significant emissions from UNG operations (Helmig, 2020). Methane emissions from UNG operations were shown to be about 23 times greater than from conventional ones, due to the larger size and higher production rate of UNG (Omara et al., 2016). The reportedly estimated annual CH4 emissions from UNG production of the main shale regions were about 158 Gg from Denver-Julesburg Basin in 2015 (Peischl et al., 2018), 270 Gg from Bakken Shale Play at Williston Basin in 2014 (Peischl et al., 2015), 131 Gg from Marcellus Shale Play at Appalachian Basin in 2013 (Peischl et al., 2015), 342 Gg from the Fayetteville Shale Play at Arkoma Basin (Peischl et al., 2015), 525 Gg from the Barnett at Ft. Worth Basin in 2013 (Karion et al., 2015), and 700 Gg from the Haynesville Shale Play at Texas-Louisiana Salt Basin (Peischl et al., 2015). The locations of each region are shown in Figure 1. Besides the CH4 emissions from the gathering/processing plants and well pads during UNG production, CH4 could also be emitted from the gathering pipeline, which was used to transport gases to a processing facility or a transmission line (Zimmerle et al., 2017).
Non-methane volatile organic compounds
NMVOCs from UNG production contain hazardous compounds (benzene, toluene, ethylbenzene, and xylenes (BTEX)) and other compounds (Shonkoff et al., 2014; Bolden et al., 2015), which act as precursors to O3 and secondary organic aerosol (SOA) (Liggio et al., 2016; McDuffie et al., 2016). Enhanced NMVOCs mixing ratios near the UNG wells have been observed with variable VOCs emission rates under different well operations (namely, drilling, fracking, coiled tubing, and flowback) (Hecobian et al., 2019). The concentration of hazardous BTEX is reported to be as high as 500 ppb downwind of oil and gas wastewater disposal facilities, which highlights the importance of monitoring BTEX concentrations during UNG operations (Helmig, 2020).
O3 and PM2.5
O3 and PM2.5 are classified as two air pollutants on the EPA list, whose high concentration levels can cause serious harm to the human health, respiratory system in particular (Anenberg et al., 2010). The elevated level of VOCs and NOx from the UNG emissions are precursors to O3 and SOA (Liggio et al., 2016; McDuffie et al., 2016). A number of studies have linked UNG production to nearby O3 exceedances, even in winter (Edwards et al., 2014). The studies at the Denver basin showed about 20 ppb of O3 enhancement (Benedict et al., 2019) and about 38% of SOA (Bahreini et al., 2018) being associated with VOCs and NOx emitted from UNG production.
Prospected air quality measurements in China
The measured air pollutants from the US shale plays based on the previous studies will be useful for narrowing the Chinese environmental researchers’ focus and comparing the different emission rates of the air pollutants due to the different types of the shale basins comparing China and the United States. The measurements used in the USA also highlighted the necessity of the integration of multiple platforms for the air pollutants, including the (1) site observation platform, (2) mobile lab, drone, and airplane observation platform, and (3) satellite observation platform, for a better understanding the air pollutants emissions, while, the platforms and the related instruments mentioned below will quickly help the environmental researchers build their suitable platforms.
Site observation platform
Large-sized temporarily fixed observation sites, generally modified from mobile shelters (EPA, 2017), are most commonly used, which could support numerous monitors with a stable power supply for long-term measurements, such as (1) the commercial on-site instruments for O3, NO/NO2, PM2.5 (e.g., 2B Model 205 O3 Analyzer, Thermo Model 42C NO/NO2/NOx Analyzer, GRIMM EDM 180 FEM PM2.5 Monitor), (2) the mass spectrometry technology-based instruments for VOCs species [e.g., IONICON Proton-transfer-reaction mass spectrometry (PTR-MS), Thermo thermal desorption gas chromatography-mass spectrometry (TD-GC-MS)] and aerosol species [e.g., Aerodyne Aerosol mass spectrometry (AMS)], (3) the laser technology-based instruments for VOCs species [e.g., Aerodyne quantum cascade lasers (QCLs)], (4) the LiDARs for O3/PM2.5 vertical profiles, (5) VOCs canister collection system for further high-sensitivity VOC species analysis, and (6) the meteorological sensors (e.g. temperature, RH, wind speed, wind direction, solar radiance, precipitation). In addition to the larger temporarily fixed sites, temporary smaller sites with low-energy and low-cost sensors have also been established and widely used (https://www.epa.gov/air-sensor-toolbox/evaluation-emerging-air-sensor-performance), which could compensate for the shortcomings of the larger fixed sites (e.g., lack of mobility, high cost to deploy quick-response situations or wide range). When equipped with a photoionization detector (PID) trigger system (Hecobian et al., 2019), the temporary smaller site can capture the high VOC plume using small canisters, which could provide useful information for the VOCs identification from UNG emissions.
Mobile lab, drone, and airplane observation platform
The mobile lab, generally converted from a van or a truck, can carry the above instruments to conduct on-road measurements using a multi-battery system or power generated from the engine (Boanini et al., 2021). The mobile lab could be used to capture the transport of air pollutant plumes, estimate the emission flux based on circle-route measurements, observe the spatial concentration distribution of air pollutants, act as a fixed site at some high concentration spots, etc (Mohr et al., 2011; von der Weiden-Reinmüller et al., 2014; Huang et al., 2020; Zhang J et al., 2020). However, given the terrain or the UNG factory regulations, the mobile lab could miss some areas. In this case, an unmanned aerial vehicle (UAV) carrying light-weight sensors could be used for such region observations and also for concentration vertical profile measurements (McKinney et al., 2019). The airplane platform could be used for large-scale observation, but the number of flights or aircraft could be limited due to the high cost.
Satellite observation platform
Satellite observations could provide temporary and spatial concentration variation of some species, such as NOx, CH4, O3, etc, which could be used to evaluate the influence of UNG emissions on a large scale (Jacob et al., 2016; Goldberg et al., 2019; Varon et al., 2019). Combining the model simulation using existing emission inventories and comparing it with the current satellite observations, the current species emission rate could be estimated (Zhang Y et al., 2020), which would be very useful for updating the existing emission inventories.
Conclusion
Benefiting from the horizontal drilling and hydraulic fracturing techniques innovation, the USA has experienced the boom of the UNG development and reached its goal of “energy independence.” However, UNG development in China is still in its infancy and has a great potential for rapid growth, which will inevitably result in air pollution, as has been seen in the Denver Basin. These issues should be given top priority due to the clean air action from the current Chinese government. The studies on the air quality in the USA for the UNG-related air pollutant emissions provide solid knowledge, either on the air pollutants characterization or their related observation technologies/platforms, which can provide very useful guidance for Chinese environmental researchers and the local governments to deal with the air quality issues caused by the further surging of UNG production.
Author contributions
Conceptualization, formal analysis, TF and JZ; investigation, resources, TF, XZ, SQ, and JF; writing—original draft preparation, TF; writing—review and editing, XZ, SQ, and JZ. All authors have read and agreed to the published version of the manuscript.
Funding
This research was funded by a domestic visiting program for outstanding young people in Colleges, grant number “gxgnfx2020027”.
Conflict of interest
Author XZ is employed by PetroChina (Tianjin) International Petroleum Exploration &Development Technology Co., Ltd.
The remaining authors declare that the research was conducted in the absence of any commercial or financial relationships that could be construed as a potential conflict of interest.
Publisher’s note
All claims expressed in this article are solely those of the authors and do not necessarily represent those of their affiliated organizations, or those of the publisher, the editors and the reviewers. Any product that may be evaluated in this article, or claim that may be made by its manufacturer, is not guaranteed or endorsed by the publisher.
References
Allen, D. T. (2016). Emissions from oil and gas operations in the United States and their air quality implications. J. Air Waste Manag. Assoc. 66, 549–575. doi:10.1080/10962247.2016.1171263
Allshouse, W. B., Adgate, J. L., Blair, B. D., and McKenzie, L. M. (2017). Spatiotemporal industrial activity model for estimating the intensity of oil and gas operations in Colorado. Environ. Sci. Technol. 51 (17), 10243–10250. doi:10.1021/acs.est.7b02084
Anenberg, S. C., Horowitz, L. W., Tong, D. Q., and West, J. J. (2010). An estimate of the global burden of anthropogenic ozone and fine particulate matter on premature human mortality using atmospheric modeling. Environ. Health Perspect. 118 (9), 1189–1195. doi:10.1289/ehp.0901220
Bahreini, R., Ahmadov, R., McKeen, S. A., Vu, K. T., Dingle, J. H., Apel, E. C., et al. (2018). Sources and characteristics of summertime organic aerosol in the Colorado front range: Perspective from measurements and WRF-chem modeling. Atmos. Chem. Phys. 18, 8293–8312. doi:10.5194/acp-18-8293-2018
Benedict, K. B., Zhou, Y., Sive, B. C., Prenni, A. J., Gebhart, K. A., Fischer, E. V., et al. (2019). Volatile organic compounds and ozone in rocky mountain national park during FRAPPE. Atmos. Chem. Phys. 19, 499–521. doi:10.5194/acp-19-499-2019
Boanini, C., Mecca, D., Pognant, F., Bo, M., and Clerico, M. (2021). Integrated mobile laboratory for air pollution assessment: Literature review and cc-TrAIRer design. Atmosphere 12 (8), 1004. doi:10.3390/atmos12081004
Bolden, A. L., Kwiatkowski, C. F., and Colborn, T. (2015). New look at BTEX: Are ambient levels a problem? Environ. Sci. Technol. 49 (9), 5261–5276. doi:10.1021/es505316f
Bunch, A. G., Perry, C. S., Abraham, L., Wikoff, D. S., Tachovsky, J. A., Hixon, J. G., et al. (2014). Evaluation of impact of shale gas operations in the Barnett Shale region on volatile organic compounds in air and potential human health risks. Sci. Total Environ. 468−469, 832–842. doi:10.1016/j.scitotenv.2013.08.080
Cheadle, L. C., Oltmans, S. J., Pétron, G., Schnell, R. C., Mattson, E. J., Herndon, S. C., et al. (2017). Surface ozone in the Colorado northern Front Range and the influence of oil and gas development during FRAPPE/DISCOVER-AQ in summer 2014. Elem. Sci. Anth 5, 61. doi:10.1525/elementa.254
Edwards, P. M., Brown, S. S., Roberts, J. M., Ahmadov, R., Banta, R. M., deGouw, J. A., et al. (2014). High winter ozone pollution from carbonyl photolysis in an oil and gas basin. Nature 514, 351–354. doi:10.1038/nature13767
EPA (2017). Quality assurance handbook for air pollution measurement systems, volume II, ambient air quality monitoring program. Available at: https://www3.epa.gov/ttnamti1/files/ambient/pm25/qa/Final%20Handbook%20Document%201_17.pdf.
Finkel, M., Hays, J., and Law, A. (2013). The shale gas boom and the need for rational policy. Am. J. Public Health 103 (7), 1161–1163. doi:10.2105/AJPH.2013.301285
Gilman, J. B., Lerner, B. M., Kuster, W. C., and de Gouw, J. A. (2013). Source signature of volatile organic compounds from oil and natural gas operations in northeastern Colorado. Environ. Sci. Technol. 47 (3), 1297–1305. doi:10.1021/es304119a
Goldberg, D. L., Lu, Z., Streets, D. G., de Foy, B., Griffin, D., McLinden, C. A., et al. (2019). Enhanced capabilities of TROPOMI NO2: Estimating NOx from north American cities and power plants. Environ. Sci. Technol. 53, 12594–12601. doi:10.1021/acs.est.9b04488
He, Z. L., Nie, H. K., Hu, D., Jiang, T. X., Wang, R. Y., Zhang, Y., et al. (2020). Geological problems in the effective development of deep shale gas: A case study of upper ordovician wufeng-lower silurian longmaxi formations in Sichuan Basin and its PeripheryHighly elevated atmospheric levels of volatile organic compounds in the Utah basin, Utah. Acta Pet. sin.environ. Sci. Technol. 4148 (4), 3794707–3914715. doi:10.7623/syxb202004001Helmig10.1021/es405046r
Hecobian, A., Clements, A. L., Shonkwiler, K. B., Zhou, Y., MacDonald, L. P., Hilliard, N., et al. (2019). Air toxics and other volatile organic compound emissions from unconventional oil and gas development. Environ. Sci. Technol. Lett. 6 (12), 720–726. doi:10.1021/acs.estlett.9b00591
Helmig, D. (2020). Air quality impacts from oil and natural gas development in Colorado. Elem. Sci. Anth 8, 4. doi:10.1525/elementa.398
Huang, Y., Li, A., Wagner, T., Wang, Y., Hu, Z., Xie, P., et al. (2020). The quantification of NOx and SO2 point source emission flux errors of mobile differential optical absorption spectroscopy on the basis of the Gaussian dispersion model: A simulation study. Atmos. Meas. Tech. 13, 6025–6051. doi:10.5194/amt-13-6025-2020
IEA (2020). World energy outlook 2020. Paris: IEA. Available at: https://www.iea.org/reports/world-energy-outlook-2020.
Jacob, D. J., Turner, A. J., Maasakkers, J. D., Sheng, J., Sun, K., Liu, X., et al. (2016). Satellite observations of atmospheric methane and their value for quantifying methane emissions. Atmos. Chem. Phys. 16, 14371–14396. doi:10.5194/acp-16-14371-2016
Karion, A., Sweeney, C., Kort, E. A., Shepson, P. B., Brewer, A., Cambaliza, M. O. L., et al. (2015). Aircraft-based estimate of total methane emissions from the Barnett Shale Region. Environ. Sci. Technol. 49 (13), 8124–8131. doi:10.1021/acs.est.5b00217
Kemball-Cook, S., Bar-Ilan, A., Grant, J., Parker, L., Jung, J. G., Santamaria, W., et al. (2010). Ozone impacts of natural gas development in the Haynesville Shale. Environ. Sci. Technol. 44 (24), 9357–9363. doi:10.1021/es1021137
Lee, L., Wooldridge, P. J., deGouw, J., Brown, S. S., Bates, T. S., Quinn, P. K., et al. (2015). Particulate organic nitrates observed in an oil and natural gas production region during wintertime. Atmos. Chem. Phys. 15, 9313–9325. doi:10.5194/acp-15-9313-2015
Liggio, J., Li, S. M., Hayden, K., Taha, Y. M., Stroud, C., Darlington, A., et al. (2016). Oil sands operations as a large source of secondary organic aerosols. Nature 534, 91–94. doi:10.1038/nature17646
Ma, X. H., and Xie, J. (2018). The progress and prospects of shale gas exploration and development in southern Sichuan Basin, SW China. Petroleum Explor. Dev. 45 (1), 172–182. doi:10.1016/S1876-3804(18)30018-1
McDuffie, E. E., Edwards, P. M., Gilman, J. B., Lerner, B. M., Dube, W. P., Trainer, M., et al. (2016). Influence of oil and gas emissions on summertime ozone in the Colorado Northern Front Range. J. Geophys. Res. Atmos. 121, 8712–8729. doi:10.1002/2016JD025265
McKenzie, L. M., Crooks, J., Peel, J. L., Biafr, B. D., Brindley, S., Allshouse, W. B., et al. (2019). Relationships between indicators of cardiovascular disease and intensity of oil and natural gas activity in Northeastern Colorado. Environ. Res. 170, 56–64. doi:10.1016/j.envres.2018.12.004
McKenzie, L. M., Witter, R. Z., Newman, L. S., and Adgate, J. L. (2012). Human health risk assessment of air emissions from development of unconventional natural gas resources. Sci. Total Environ. 424, 79–87. doi:10.1016/j.scitotenv.2012.02.018
McKinney, K. A., Wang, D., Ye, J., de Fouchier, J. B., Guimarães, P. C., Batista, C. E., et al. (2019). A sampler for atmospheric volatile organic compounds by copter unmanned aerial vehicles. Atmos. Meas. Tech. 12, 3123–3135. doi:10.5194/amt-12-3123-2019
Moore, C., Zielinska, B., Pétron, G., and Jackson, R. B. (2014). Air impacts of increased natural gas acquisition, processing, and use: A critical review. Environ. Sci. Technol. 48 (15), 8349–8359. doi:10.1021/es4053472
Nie, H. K., Li, D. H., Liu, G. X., Lu, Z. Y., Hu, W., Wang, R. Y., et al. (2020). An overview of the geology and production of the fuling shale gas Field, Sichuan Basin, China. Energy Geosci. 1 (3−4), 147–164. doi:10.1016/j.engeos.2020.06.005
Omara, M., Sullivan, M. R., Li, X., Subramanian, R., Robinson, A. L., Presto, A. A., et al. (2016). Methane emissions from conventional and unconventional natural gas production sites in the Marcellus shale basin. Environ. Sci. Technol. 50 (4), 2099–2107. doi:10.1021/acs.est.5b05503
Pacsi, A. P., Kimura, Y., McGaughey, G., McDonald-Buller, E. C., and Allen, D. T. (2015). Regional ozone impacts of increased natural gas use in the Texas power sector and development in the eagle ford shale. Environ. Sci. Technol. 49, 3966–3973. doi:10.1021/es5055012
Peischl, J., Eilerman, S. J., Neuman, J. A., Aikin, K. C., deGouw, J., Gilman, J. B., et al. (2018). Quantifying methane and ethane emissions to the atmosphere from Central and Western U.S. oil and natural gas production regions. J. Geophys. Res. Atmos. 123, 7725–7740. doi:10.1029/2018JD028622
Peischl, J., Ryerson, T. B., Aikin, K. C., de Gouw, J. A., Gilman, J. B., Holloway, J. S., et al. (2015). Quantifying atmospheric methane emissions from the Haynesville, Fayetteville, and northeastern Marcellus shale gas production regions. J. Geophys. Res. Atmos. 120, 2119–2139. doi:10.1002/2014JD022697
Pétron, G., Karion, A., Sweeney, C., Miller, B. R., Montzka, S. A., Frost, G. J., et al. (2014). A new look at methane and nonmethane hydrocarbon emissions from oil and natural gas operations in the Colorado Denver-Julesburg Basin. J. Geophys. Res. Atmos. 119 (11), 6836–6852. doi:10.1002/2013JD021272
Pozzer, A., Schultz, M. G., and Helmig, D. (2020). Impact of U.S. Oil and natural gas emission increases on surface ozone is most pronounced in the central United States. Environ. Sci. Technol. 54 (19), 12423–12433. doi:10.1021/acs.est.9b06983
Shonkoff, S. B., Hays, J., and Finkel, M, L. (2014). Environmental public health dimensions of shale and tight gas development. Environ. Health Perspect. 122, 787–795. doi:10.1289/ehp.1307866
Sun, C., Nie, H., Dang, W., Chen, Q., Zhang, G., Li, W., et al. (2021). Shale gas exploration and development in China: Current status, geological challenges, and future directions. Energy fuels. 35 (8), 6359–6379. doi:10.1021/acs.energyfuels.0c04131
Varon, D. J., McKeever, J., Jervis, D., Maasakkers, J. D., Pandey, S., Houweling, S., et al. (2019). Satellite discovery of anomalously large methane point sources from oil/gas production. Geophys. Res. Lett. 46, 13507–13516. doi:10.1029/2019GL083798
von der Weiden-Reinmüller, S. L., Drewnick, F., Crippa, M., Prévôt, A. S. H., Meleux, F., Baltensperger, U., et al. (2014). Application of mobile aerosol and trace gas measurements for the investigation of megacity air pollution emissions: The paris metropolitan area. Atmos. Meas. Tech. 7, 279–299. doi:10.5194/amt-7-279-2014
Wang, Z., and Krupnick, A. A. (2013). Retrospective review of shale gas development in the United States: What led to the boom? Resour. Future, DP13–12.
Zhang J, J., Ninneman, M., Joseph, E., Schwab, M. J., Shrestha, B., Schwab, J. J., et al. (2020). Mobile laboratory measurements of high surface ozone levels and spatial heterogeneity during LISTOS 2018: Evidence for sea‐breeze influence. J. Geophys. Res. Atmos. 125 (11). doi:10.1029/2019JD031961
Zhang Y, Y., Gautam, R., Pandey, S., Omara, M., Maasakkers, J. D., Sadavarte, P., et al. (2020). Quantifying methane emissions from the largest oil-producing basin in the United States from space. Sci. Adv. 6, eaaz5120. eaaz5120. doi:10.1126/sciadv.aaz5120
Zimmerle, D. J., Pickering, C. K., Bell, C. S., Heath, G. A., Nummedal, D., and Pétron, G. (2017). Gathering pipeline methane emissions in Fayetteville shale pipelines and scoping guidelines for future pipeline measurement campaigns. Elem. Sci. Anthropocene 5, 70. doi:10.1525/elementa.258
Keywords: unconventional natural gas development, air pollution, air quality measurements, China, United States
Citation: Fang T, Zhang X, Zhang J, Wang J and Sun Q (2022) The prospected air quality measurements for further unconventional natural gas developments in China based on the United States experience. Front. Environ. Sci. 10:864818. doi: 10.3389/fenvs.2022.864818
Received: 28 January 2022; Accepted: 29 June 2022;
Published: 19 July 2022.
Edited by:
Lang Liu, Northwestern Polytechnical University, ChinaReviewed by:
Eduardo Landulfo, Instituto de Pesquisas Energéticas e Nucleares (IPEN), BrazilCopyright © 2022 Fang, Zhang, Zhang, Wang and Sun. This is an open-access article distributed under the terms of the Creative Commons Attribution License (CC BY). The use, distribution or reproduction in other forums is permitted, provided the original author(s) and the copyright owner(s) are credited and that the original publication in this journal is cited, in accordance with accepted academic practice. No use, distribution or reproduction is permitted which does not comply with these terms.
*Correspondence: Jie Zhang, jzhang35@albany.edu; Qianqian Sun, sqqufhefei@163.com