- 1Belt and Road Initiative Institute for Chinese-European Studies (BRIICES), Guangdong University of Petrochemical Technology, Maoming, China
- 2Mining and Metallurgical Institute Named After O.A. Baikonurov, Satbayev University, Almaty, Kazakhstan
- 3Department of Mining Engineering and Education, Dnipro University of Technology, Dnipro, Ukraine
- 4Department of Cartography and Geoinformatics, Al Farabi Kazakh National University, Almaty, Kazakhstan
The purpose of this study was to develop a methodology for integrated monitoring of the rock mass state, which ensures the industrial and environmental safety of the Central Kazakhstan region. As a result of the research, a methodology for maintaining an integrated system of geodynamic monitoring has been developed. The results of predicting the physical–mechanical properties and stress–strain state of the rock mass are presented. Based on the data obtained, graphical–analytical dependences between the most important quantitative characteristics of the rock properties in the studied deposits are presented. The scientific novelty of the presented research lies in determining the pattern of changes in the structural peculiarities and strength properties of rocks with the depth of their occurrence, which makes it possible to predict the stress–strain state of the rock mass. The authors propose a new method for creating a geodynamic test site. The geodynamic test site created in the fields of Central Kazakhstan is a reliable basis for the organization of long-term monitoring for the earth’s surface deformation processes during large-scale development of the field, which improves the productivity and accuracy of observations. The results obtained can be used to improve the level of industrial safety at mines and minimize environmental risks caused by the subsoil development.
Introduction
Mining of mineral resources is considered an important issue for energy-saving and resource-saving sustainability (Golik et al., 2018). The energy sector of industry development needs more mineral resources for energy generation that is mined out from the earth’s subsoil. At the same time, the innovative development of mining technologies is also driven by the need for saturation of the long-term demand for raw materials (Zhironkin and Cehlár, 2021; Zhironkin and Szurgacz, 2021).
Large-scale mining operations conducted in highly stressed hard-rock mass are characterized by significant geomechanical and geodynamic processes associated with a change in the stress state, deformation, and displacement of mine rocks. These processes entail not only catastrophic technical-and-economic consequences but sometimes also lead to human losses. Technogenic earthquakes occurred in Germany, United States, Poland, and Czech Republic (Field, 2007; Burmin and Shumlianska, 2017; Adushkin, 2018; Gerasymenko et al., 2020; Sach et al., 2020; Amemoutou et al., 2021). In Russia, this problem is acute in the mines of the Verkhnekamensk potassic salt deposits and the Khibiny apatite-nepheline mines (Viktorov et al., 2005; Rebetskiy et al., 2017). All this is a direct consequence of the change in the geodynamic regime of the geological environment under the influence of large-scale mining operations (Pavlychenko and Kovalenko, 2013; Buzylo et al., 2018). Moreover, this is convincingly confirmed by the results of many years of scientific research into the Zhezkazgan natural–technical system, which is formed by mines, dressing mills with tailing dumps, and copper-smelting plants in Karaganda, Balkhash, Zhezkazgan, and Satpayev (Bekbergenov et al., 2020). The corresponding infrastructure in Central Kazakhstan is a powerful subject of anthropogenic impact on the environment, providing great opportunities for researching a wide range of environmental problems (Ryl’nikova et al., 2016; Mikhailova and Uzbekov, 2018a).
In recent years, objects with a complex geological structure and a large depth of occurrence have been increasingly included in the development and operation of ore deposits. This requires special conditions for mining and development of these objects. K.I. Satpayev (1962) wrote about this in his study “Zhezkazgan copper ore region and its mineral resources”, creating a metallogenic prediction map of Kazakhstan. And at present, the mineral resource base of Central Kazakhstan is expanding.
Rational development of mineral deposits presupposes the complete extraction of reserves while performing a minimum volume of overburden operations with geomechanical substantiation of the required degree of the rock mass stability (Stupnik et al., 2014; Babets et al., 2017; Abdiev et al., 2020; Zhanakova et al., 2020; Bazaluk et al., 2021). Masloboev et al. (2021) discussed the minimization of artificial impacts on the environment, and new technological solutions have been developed for mining enterprises. Waloski et al. (2021) indicate that from the point of view of the stability of underground structures, the most critical features of the rock mass are rock and rock mass strength as well as discontinuity surfaces. Moreover, the influence of cutting is considered an important issue in mining (Łukasz, 2020). The main initial information is the geological knowledge of the deposit (Aitkazinova et al., 2016; Petlovanyi et al., 2019), knowledge of the parameter values of the physical–mechanical and strength properties of rocks (Malanchuk et al., 2021), as well as the elements of the occurrence, structural-tectonic peculiarities of the rock mass, and fractures in rocks (Dychkovskyi et al., 2018; Lozynskyi et al., 2020). This makes it possible to determine the mass stress state in the mining process.
The geomechanical processes are studied at individual mines, and there is an experience in this direction. The dynamics of the mining complex development in Kazakhstan and in the world over the past century has led to a qualitatively new situation, when the “local” geomechanical fields induced by human technogenic activity are no longer small in comparison with the global geodynamic processes of the Earth’s tectonic activity (Begalinov et al., 2020a; Zeylik et al., 2021). Therefore, it is necessary to consider mines and pits as unique natural laboratories, where it is possible to study in detail the relationship between geomechanical and geodynamic processes using mining-geophysical and satellite geodetic methods.
In Kazakhstan, such studies, to a large extent, are conducted only during the development of oil and gas resources (Karatayev and Clarke, 2014), where both technogenic consequences and natural geodynamic peculiarities of the region are taken into account (Sarybayev et al., 2015; Moldabayev et al., 2019). At the same time, such studies on ore deposits have not been widely developed.
During large-scale and long-term mining of ore deposits, along with geomechanical deformation processes, geodynamic processes also develop (Mikhailova and Uzbekov, 2018b; Ursul and Ursul, 2018; Begalinov et al., 2020b). In this regard, the past decade has been marked by major achievements in the field of industrial and environmental safety, as evidenced by the increased number of publications on this topic (Mora and Keipi, 2006; Bondarenko et al., 2012; Oparin, 2015; Haiko et al., 2019). Special attention is paid to sustainable development because the environment is one of the essential state-level and international challenges (Rakhmangulov et al., 2017; Rozin, 2021).
The scope of application of modern mining requires an advanced study and constant monitoring of geomechanical processes occurring in the inner part of the earth, based on monitoring the state of the open-pit slopes, which provides a systematic approach to solving all components of the problems, comprehensive accounting, and analysis of all natural and technogenic factors (Dryzhenko et al., 2017; Cheskidov et al., 2019). Surface mining, in the broadest sense of the word monitoring, is the observation of the environment, presented as a dynamic, constantly changing system, and which is aimed at its control, study, prediction, and protection (Aitkazinova et al., 2014; Kyrgizbayeva et al., 2015; Nurpeissova et al., 2020). At the same time, sustainability of the open-pit mining and technical system is one of its key goals in the changing conditions of the external and internal environment (Rakhmangulov et al., 2021).
A prerequisite for the safe and environmentally sustainable development of surface mining is the improvement of the system for monitoring and observing the deformations of the open-pit slopes, slopes, and dumps using the modern methods of geodesy and high-precision deformation measurements (Malanchuk et al., 2020; Moshynskyi et al., 2020). The stability of the open-pit sides is characterized by the stability coefficient, the value of which shows the relative excess in the rock mass strength in comparison with the shearing stresses (Du and Xing, 2017). At the stage of open-pit design, in order to determine and substantiate the parameters of its slopes and benches, the stability coefficient is calculated taking into account the geological and hydrogeological conditions of the adjacent rock mass (Bitimbaev et al., 2017; Stupnik et al., 2019; Sobko et al., 2020). The choice of the optimal parameters of the slopes and local benches of open pits affects the safety of mining operations and the economic efficiency of the enterprise (Kharisov, 2018).
In general, minerals are mainly mined by the surface mining method, which accounts for up to 75% of the extracted mineral products from the subsoil, and this level will be maintained in the future (Cherniaiev, 2017; Ali Elbeblawi et al., 2021). At the same time, an increase in the depth, size, and production capacity of open pits can be traced to the tendency of their development (Dagdelen and Traore, 2018; Whittle et al., 2018; Babets et al., 2021). Under these conditions, the task of ensuring the stability of the open-pit slopes becomes especially urgent (Stacey et al., 2003). In this regard, it becomes necessary to conduct geomechanical monitoring and improve its geodetic observation methods to ensure the long-term stability of the open-pit slopes.
Modern methods of monitoring the geomechanical state of the open-pit slopes are very diverse (Fleurisson, 2012). Among the equipment most commonly used for monitoring the safety situation in open pits are laser scanning (Rysbekov et al., 2019), electronic total stations and Global Positioning System (GPS) technology (Kim et al., 2003), and big data (Krichevsky, 2016; Jiang et al., 2018). During geodetic monitoring of objects located in the industrial zone of the Maykain mine in Kazakhstan, such geodetic instruments as satellite technologies, electronic total stations, and leveling lasers have been successfully used (Krylova, 1978; Nurpeisova et al., 2016; Nurpeisova and Kurmanbaev, 2016).
The technical level of traditional geodetic observations during geomechanical monitoring does not always meet the requirements of mining enterprises. Since this requires a lot of working time and there is no possibility of promptly obtaining the necessary infomation about the deformation state of the rock mass. Therefore, the use of modern geodetic instruments in geomonitoring, such as electronic total stations, GPS technologies, and laser scanners, as well as the improvement of methods of working with them, are closely related to an increase in the level of innovative directions. All this confirms the importance of improving the methodology for conducting geomechanical monitoring using modern geodetic instruments as the basis for solving a scientific–technical problem.
Methods
Analysis of the state of the methodology for conducting geodetic observations on the territory of the developed field is primarily associated with the lack of effective methods for determining the values of deformations. This necessitates the improvement of the methodology for geodetic observations of rock deformations using modern instruments. Geodetic observations make it possible to reveal the mass deformations, which is essential for assessing the geomechanical situation in the area of field mining. But they do not provide a complete picture of deformation processes in the course of time. This can be performed only when using the proposed integrated methodology for conducting geomonitoring of the state of the near-side masses (Figure 1).
Based on the above, the purpose is set, the idea is substantiated and the structure of the integrated monitoring conducted in Central Kazakhstan is formulated.
According to the 1-block of the recommended methodology (Figure 1), the engineering-geological and mining-technical conditions of the giant Saryoba copper deposit located in Central Kazakhstan have been studied in detail. The parameters for studying the geology and tectonics in the area are the numerical data of surveying the deposit. The East Saryoba ore bodies are represented by stratified, rounded, and banded deposits with a very variable thickness and nonuniform distribution of useful components. The thickness of commercial ore bodies ranges from 0.1 to 20 m and averages 3–8 m for the East Saryoba deposit. The reserves of В + С1 + С2 categories have been explored and approved in quantities that make it possible to classify the ore field as a large industrial facility.
According to the 2-block of the recommended methodology (Figure 1), the structural peculiarities on the outcrops and the physical–mechanical properties of the rocks in the Saryoba deposit mass have been studied. The rock samples, including minerals, are taken for the purpose of laboratory determination of indicators of physical–mechanical properties that significantly influence the displacement process. In practice, the laboratory method is mainly used to study the physical–mechanical properties of rocks on samples made from cores from geological exploration wells (Figure 2A). In the presence of mine workings (trenches, pitholes, shafts, crossdrifts, etc.), samples are taken from rocks in the form of monoliths (Figure 2B). The parameters for studying the physical–mechanical properties and stress–strain state of the rock mass are depth of rock occurrence, compression strength, tension strength, density, adhesion, internal friction angle, and rock hardness according to Prof. M. M. Protodiakonov scale. It should be noted that the main part of the East Saryoba deposit reserves (up to 90%) is represented by flat-dipping and nearly level bedding with a dip angle of up to 25°. The East Saryoba deposit is mined using the pillar-and-panel system with the division of ore bodies into panels—mining units.
To compare the results obtained at the studied object of the Saryoba deposit with the data of other mines, a combined graph of the relationship of rock properties is compiled. Assessment and reliability of determining the dependencies are made according to the formulas of mathematical statistics:
where σ is the standard; x1 is the characteristic value;
If the condition Eq. 3 mv < V is satisfied, then the results can be considered reliable and the number of initial data for determining the statistical means is sufficient.
According to the 3-block of the recommended methodology (Figure 1), geodetic observation of the rock mass state is necessary. When performing geodetic observations of the mass state and determining the mining allotment boundaries, the spatial contours of the mineral deposit are taken into account: position of the site for construction, as well as operation of underground structures; boundaries of safe mining and blasting operations; zones of protection from the harmful effects of mining operations; zones of rock displacement; contours of protecting pillars under natural objects, buildings and structures; spacing out of the open-pit sides and cuts, as well as other factors influencing the state of the subsoil and the earth’s surface as a result of the process of geological exploration and the subsoil use. Mining of minerals is permissible only within the subsoil site boundaries provided for use in accordance with the standard procedure. To date, various methods and ways of controlling the rock mass stress–strain state have been developed. Among the main ones are unloading VNIMI method, core disking, etc. To determine the stress state of the rock masses in the Karatau deposit (1995–2000), the Akbakai deposit (2012–2015), the authors have studied the core materials of geological exploration wells and the disking method. When conducting the geotechnological studies of the mine rock stability with the issuance of recommendations for managing the rock pressure in the Akzhal mine of Nova Zinc LLP (2007–2018), a “Seismic-acoustic method for predicting the stress–strain state of a rock mass”, developed by the authors for determining the mass disturbance, is used in the Saryoba deposit (2020–2021).
The seismic-acoustic method for predicting the rock mass stress–strain state includes setting a sensor of seismic-acoustic signals emitted by natural sources in the rock mass, after which the parameters of these signals are determined. Seismic-acoustic signals are recorded by a sensor in wells drilled near mine workings at different horizons. This method is characterized by simplicity and speed of measurement with high reliability of results. The scheme of the seismic-acoustic method implementation for predicting the rock mass stress–strain state is presented in Figure 3.
The stress measurement scheme works according to the method of unloading the core at the end of the well (4). To do this, a special disk fixed on a rod is used to grind the end of the well and the strain gauge (1) is firmly pressed onto the end. The cable from the strain gauge is passed into a hollow drill rod and, with the help of a crown, is inserted into the annular slot (2). As a result, elastic expansion of the core (3) occurs, which is fixed by a measuring strain gauge in three directions, the results of which are shown in Table 2. After measuring the deformations, the core is broken off and is sent to the laboratory in a special package to determine the elastic characteristics of the rocks (Е and µ).
Result and Discussion
State of the Current Development of the Problem and Formulating a Research Task
In the ore field of the Saryoba deposit, 11 ore deposits are identified, in which 109 ore bodies are explored. The largest deposits are confined to the Taskuduk horizon. Their strike is north-eastern, the length is up to 3,200 m, the thickness is from 0.5 to 17 m, and the size of the dip is up to 1,400 m (Figure 4).
The geological structure of the area involves rocks of several geological systems—from the pre-Cambrian rocks to the Anthropocene. The thick (up to 3,000 m) Middle Paleozoic stratum of terrigenous and carbonate deposits transgressively overlies pre-Cambrian and Lower Paleozoic formations through basal conglomerates. The Zhezkazgan formation of deposits is assigned to the Upper Paleozoic, which includes from bottom to top:
A) Zhezkazgan ore-bearing stratum of argillo-arenaceous deposits, subdivided into the Taskuduk and Zhezkazgan formations with a total thickness of 650–680 m;
B) Zhidelisay formation, consisting of alternating layers of red argillites and sandstones with a total thickness of 300 m;
C) Red stratum, overlain uniformly by the Kengir formation, represented by interbedded layers of gray and dark gray marls and dolomitic limestones with a total thickness of 500 m.
The first two formations are ore-bearing, and the main attention in the area is paid to their study. Therefore, we have thoroughly studied the Taskuduk formation, which is currently being developed.
Pre-ore and post-ore disjunctive faults are present at the Saryoba deposit, which greatly complicates their detailed survey and development. In the studied area, the south-western part of the ore field (Figure 3) is complicated by high-order folds and faults, the largest of which is the Saryoba transpressional fault of submeridional strike. Its length is more than 10 km with a width of 250–400 m, the main plane dips to the west at an angle of 25–30°. The Saryoba transpressional fault divides the ore field into two almost equal parts. West Saryoba deposit is located in the western half, and East Saryoba is located in the eastern half.
Studying the Physical–Mechanical Properties and Stress–Strain State of the Mass
The results of testing the physical–mechanical properties of rocks from the Saryoba deposit are given in Table 1. It should be noted that the table includes only data on the average values of core samples from each horizon. Samples for studying the physical–mechanical properties of rocks to a depth of 100 m are taken from the outcropped benches of the open-pit sides, the rest are taken from the cores of drilled exploration and production wells.
In the studied area, the south-western part of the ore field (Figure 4) is complicated by high-order folds and faults, the largest of which is the Saryoba transpressional fault of submeridional strike. Its length is more than 10 km with a width of 250–400 m, the main plane dips to the west at an angle of 25–30°. The Saryoba transpressional fault divides the ore field into two almost equal parts. The West Saryoba deposit is located in the western half, and East Saryoba is located in the eastern half.
Analysis of the results of studying the physical–mechanical properties of rocks makes it possible to determine graphical-analytical dependences of changes in the strength properties of mass rocks with the depth of their occurrence and promptly make changes in the horizontally calculated stability of mine workings (Figure 5).
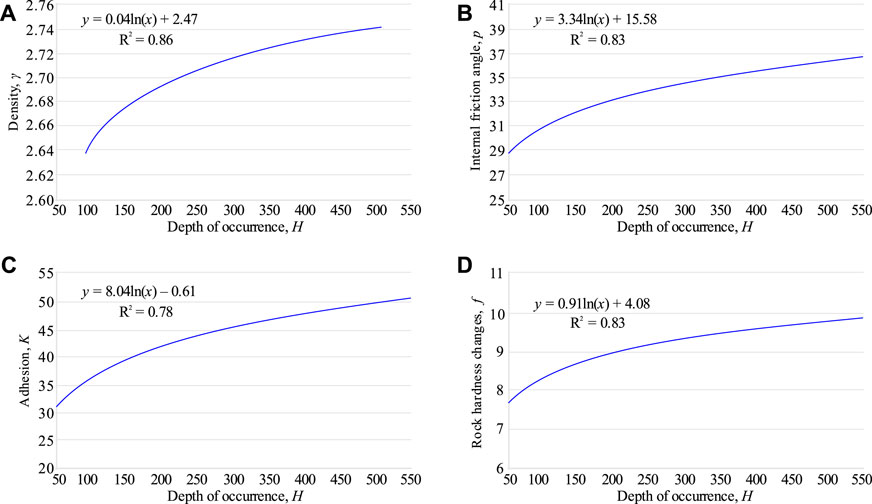
FIGURE 5. Dependence of the massive limestone strength properties with the depth of its occurrence (H): (A) density changes, g; (B) changes in the internal friction angle, р; (C) adhesion changes, K; (D) rock hardness changes, f.
Similar dependencies have also been obtained for a number of fields in Kazakhstan, namely for the Akzhal field, Akbakai, and Sayak deposits, etc. To find the general patterns of variability in the strength and structural properties of rocks, data from a number of deposits have also been generalized and graphical-analytical dependences have been determined between the average density, adhesion, compression, hardness of rocks, and the depth of their occurrence. The curves of changes in the properties of rocks are drawn according to the averaged group indicators for the depth intervals every 5 m. To compare the results obtained at the studied object with the data of other mines, a combined graph of the relationship of rock properties has been compiled (Figure 6).
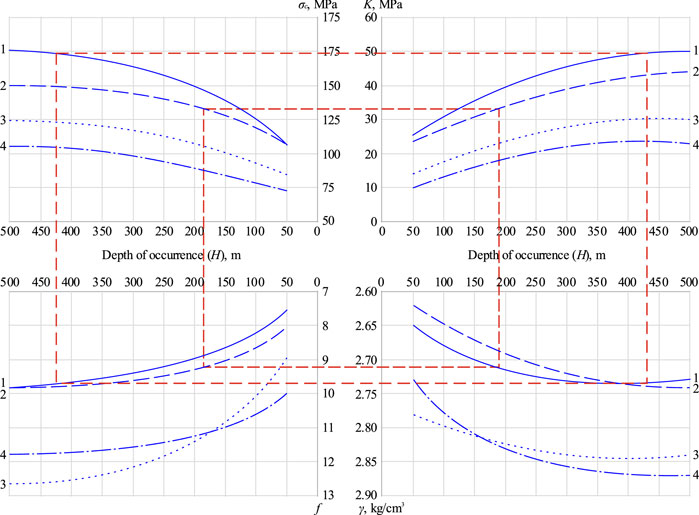
FIGURE 6. Dependences of the rock properties on changes in the depth of their occurrence: 1, Saryoba deposit; 2, Akzhal field, 3, Sayak deposit, 4, Akbakai deposit; σc, compression strength of rocks, MPa; K, adhesion, MPa; f, rock hardness; γ, density, kg/cm3.
The curves of changes in the rock properties are drawn according to averaged indicators in a depth every 50 m. Assessment and reliability of determining the dependencies are made according to the formulas of mathematical statistics. The deviation of the calculated curves from the empirical ones ranges from 5 to 8%, and most of the curves coincide with each other. The analysis of the data also shows that the strength properties of rocks change noticeably with the depth of their occurrence.
Based on the research performed, quantitative and qualitative characteristics of the rock properties in a number of deposits, including the Saryoba deposit, have been obtained. These characteristics will be used to solve a number of technical problems in open pits and underground mines, to assess the stability of benches and open-pit slopes, design pillars and limiting floor pillars, as well as to generalize the results of instrumental observations and understand the physical nature of the displacement process.
Studying the Physical–Mechanical Properties and Stress–Strain State of the Mass
The results of measuring the natural stresses in the Saryoba deposit according to the geodetic observation (3-block) are given in Table 2.
When analyzing Table 2 and the results of determining the natural stresses obtained for other mines, one cannot fail to notice the following peculiarities of the horizontal stresses distributed in the rock mass. Firstly, the horizontal stresses exceed vertical ones in terms of their values. Secondly, at equal depths from the daylight surface in hard rocks, horizontal stresses have higher values than in relatively weak rocks. Thirdly, the vertical stresses are on average close to the value of σН, regardless of the rock strength. Thus, all types of stresses change with depth, especially the sum of values of horizontal stresses (σx + σу) changes according to a certain pattern, and this is clearly seen from Figure 7.
According to Figure 7, the correlation between stress and depth is straight-line. The correlation coefficient of the relationship is R = 0.92. Accordingly, the correlation relationship can be determined by the following formula:
where Y is the sum of principal horizontal stresses, (σx+σу); x is the mining depth, Н; a and b are parameters of equations.or
If to focus on Formula (4), then normal stresses are also related to the bulk density of rocks. Then, Formula (5) has the following form:
where γ is average bulk density 2.8 t/m3; Н is the depth of the daylight surface, m.
The graphical-analytical dependences obtained as a result of the performed research make it possible to give a qualitative and quantitative assessment of changes in the properties of rocks. There is a stable correlation between the indicators. With some known indicators, it seems possible to determine others using graphs, thereby opening up the possibility of predicting the properties of rocks within the deposits. The initial data for this are the names of the rocks, their depth of occurrence, and individual characteristics, such as the specific density of rocks and others, the determination of which does not require labor-intensive laboratory studies.
Based on the research performed, quantitative and qualitative characteristics of the rock properties in a number of deposits, including the West Saryoba deposit, have been obtained. These characteristics will be used to solve a number of technical problems in open pits and underground mines, in assessing the stability of benches and open-pit sides, in calculating the pillars and limiting floor pillars, as well as to generalize the results of instrumental observations and understand the physical nature of the displacement process.
According to the 3-block of the recommended methodology, to conduct observations of the rock mass state, especially when prospecting a giant copper field, occupying a large area and consisting of several deposits, occurring in various deep horizons, it is necessary to create a high-precision geodetic substantiation. To provide a huge area, the classic option of creating geodetic networks in the fields is rather labor-intensive. It requires large financial costs for the organization and production of observations. In this regard, it is proposed to replace extended leveling lines with local geodetic constructions in the form of profile lines and check “clusters” of geodetic and leveling stations. The complex application of ground and space geodetic methods allows monitoring observations to cover the entire territory of the field, as well as to increase the efficiency of observations and reduce capital costs for their implementation.
Thus, during large-scale and long-term field development, along with geomechanical deformation processes, geodynamic processes can also develop. Therefore, when mining the deep-seated ore bodies, a geodynamic test site is created, where repeated geodetic observations are conducted (Nurpeisova et al., 2019; Nurpeisova et al., 2021).
Accordingly, for a differentiated study of geodynamic and geomechanical processes of mining the fields that occupy a large area and are intended for deep seams, it is proposed to create cluster arms at the field, consisting of base (reference), control (initial), and deformation geodetic and leveling stations. All the focal stations are located in accordance with ore veins and connected to the stations of the State Geodetic Network (Figure 8).
Instrumental observations have confirmed the labor intensity of field work, especially the transfer of a set of instruments (the instrument itself, a tripod, rods, etc.) from one station to another. In this regard, in order to install instruments and increase the speed of measuring operations, firstly, a permanent forced centering station (FCS) has been developed and patented, which is arranged at the control station during geomechanical monitoring. Then, the device is transferred to geodetic centers for setting new instruments and signals.
A schematic representation of the ground-based FCS construction is given in Figure 9, which shows a ground-based permanent geodetic station of forced centering of instruments, containing a support protruding above the ground (4) with a height of 1.25 m and buried to a depth of 2.25 m below the depth of soil freezing. On the upper surface, there is a center with the given coordinates (6) for setting the device. The support is made of a metal pipe (1) with a concreted lower part and filled with local soil material. At the upper pipe end, there is a metal platform (5) with a universal socket and a window for setting the device (3) and sighting mark (8). On the side surface of the metal pipe, at a distance of 1 m from the upper platform, a second metal platform is set for the high-elevation reference mark (7), and the lower end of the pipe is equipped with an anchor.
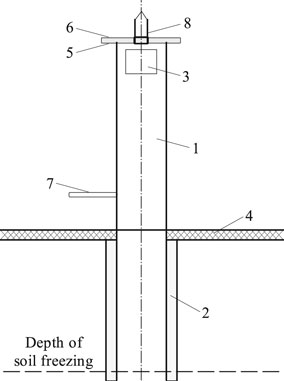
FIGURE 9. Ground-based FCS construction: 1, metal pipe; 2, concreted lower part; 3, window for setting the device; 4 is earth surface; 5, first metal platform; 6, station center; 7, second metal platform; 8, sighting mark.
The purpose of the invention is to improve the accuracy of centering, as well as to increase the efficiency of measurements in the absence of a tripod at the stations of setting and observation. The new device allows for quick and accurate centering, as well as eliminates the need for tripods.
Thus, on the basis of the performed GPS measurements, mine surveying services and observation stations of mines in Central Kazakhstan have been provided with control stations, the coordinates of which are determined with high accuracy. Observations of the absolute deformations of the sides at the studied objects have been conducted on the profile lines of the observation station with devices of a new generation.
The planned coordinates and preliminary heights of these stations have been determined by the satellite method using a geodetic satellite receiver Leica GS16 3.75G. The final heights of the reference marks have been determined by the method of geometric leveling of the II class using a Trimble Dini03 digital leveling laser and barcode rails. The coordinates of stations and reference marks of the geodynamic test site will be presented after their adjustment in a single system of coordinates and the Baltic height.
Conclusion
Based on the performed analysis in the field of studying geomechanical and geodynamic processes, as well as means of observing the deformations, an integrated methodology for conducting geomonitoring with the use of modern high-precision geodetic instruments and developed methods for their control has been developed and substantiated. The research results of geomechanical and geodynamic processes will allow for the strategic and operational management of mining operations to ensure maximum safety and economic efficiency. The practical use of the results is possible for Central Kazakhstan deposits. In the future, the research will be continued to study the possibility of applying the results obtained to other Zhilandinskaya Group deposits.
The scientific novelty of the presented research is in determining the pattern of changes in the structural peculiarities and strength properties of rocks with the depth of their occurrence, which makes it possible to predict the stress–strain state of the rock mass.
The geological, structural, and tectonic peculiarities of a giant copper deposit in Kazakhstan are presented. The possibility of predicting the physical–mechanical properties and stress–strain state of the rock mass has been substantiated. The results obtained have been compared with the results of similar works by other authors, and they make it possible to conclude that the obtained regression equations are quite reliable (correlation coefficient R = 0.92).
The modern approach to the formulation and implementation of observations of geodynamic and geomechanical processes in solid mineral deposits has been analyzed. A new “cluster” method for constructing geodetic observation systems at a geodynamic test site has been substantiated, which makes it possible to perform monitoring control coverage of mining operations, as well as to increase the efficiency of observations and reduce the costs of their implementation.
In order to set high-precision electronic and laser devices during the geomonitoring of the earth’s surface, a method has been developed for setting permanent forced centering stations, which make it possible to ensure the speed and accuracy of centering, as well as to exclude the use of tripods. It is proposed to conduct surveys using modern geodetic instruments, such as satellite technologies, and electronic and digital geodetic instruments.
Data Availability Statement
The original contributions presented in the study are included in the article/Supplementary Material, further inquiries can be directed to the corresponding authors.
Author Contributions
Conceptualization, KR, MN and GK; methodology, KR and GK; software, VL and TT; validation, KR and GK; formal analysis, VL and TT; investigation, MN and GK; resources, OB and VL; data curation, KR and MN; writing-original draft preparation, KR, MN and GK; writing-review and editing, KR, MN, GK and VL; visualization, OB, VL and TT; supervision, KR and MN; project administration, OB and KR; funding acquisition, OB. All authors have read and agreed to the published version of the manuscript.
Funding
This study was carried out as part of the project “Belt and Road Initiative Institute for Chinese-European studies (BRIICES)” and was funded by the Guangdong University of Petrochemical Technology.
Conflict of Interest
The authors declare that the research was conducted in the absence of any commercial or financial relationships that could be construed as a potential conflict of interest.
Publisher’s Note
All claims expressed in this article are solely those of the authors and do not necessarily represent those of their affiliated organizations, or those of the publisher, the editors and the reviewers. Any product that may be evaluated in this article, or claim that may be made by its manufacturer, is not guaranteed or endorsed by the publisher.
Acknowledgments
The authors express their gratitude to the reviewers for valuable recommendations that have been taken into account to significantly improve the quality of this paper. The research has been carried out in the framework of the implementation of a research project supported by the Science Committee of the Ministry of Education and Science of the Republic of Kazakhstan (grant No. AP08857097).
References
Abdiev, A., Mambetova, R., Abdiev, A., and Abdiev, S. (2020). Development of Methods for Assessing the Mine Workings Stability. E3s Web Conf. 201, 01040. doi:10.1051/e3sconf/202020101040
Adushkin, V. V. (2018). Technogenic Tectonic Seismicity in Kuzbass. Russ. Geology. Geophys. 59 (5), 571–583. doi:10.1016/j.rgg.2018.04.010
Aitkazinova, S. K., Nurpeisova, M. B., Kirgizbaeva, G. M., and Milev, I. (2014). Geomechanical Monitoring of the Massif of Rocks at the Combined Way of Development of fields. Int. Multidisciplinary Scientific GeoConference Surv. Geology. Mining Ecol. Manag. 2 (2), 79–292.
Aitkazinova, S., Soltabaeva, S., Kyrgizbaeva, G., Rysbekov, K., and Nurpeisova, M. (2016). Methodology of Assessment and Prediction of Critical Condition of Natural ‐ Technical Systems. Scientific Geoconference Surv. Geology. Mining Ecol. Manag. SGEM 2, 3–10. doi:10.5593/sgem2016/b22/s09.001
Ali Elbeblawi, M. M., Abdelhak Elsaghier, H. A., Mohamed Amin, M. T., and Elrawy Abdellah, W. R. (2021). Surface Mining Methods and Systems. Top. Mining, Metall. Mater. Eng., 289–333. doi:10.1007/978-981-16-3568-7_8
Amemoutou, A., Martínez‐Garzón, P., Kwiatek, G., Rubinstein, J. L., and Bohnhoff, M. (2021). Earthquake Source Mechanisms and Stress Field Variations Associated with Wastewater-Induced Seismicity in Southern Kansas, USA. J. Geophys. Res. Solid Earth 126 (7), e2020JB021625. doi:10.1029/2020jb021625
Babets, D. V., Sdvyzhkova, O. O., Larionov, M. H., and Tereshchuk, R. M. (2017). Estimation of Rock Mass Stability Based on Probability Approach and Rating Systems. Naukovyi Visnyk Natsionalnoho Hirnychoho Universytetu (2), 58–64.
Babets, Y., Terekhov, Y., Mormul, T., Melnikova, I., and Komirna, V. (2021). Environmental and Economic Assessment of the Possibilities to Increase the Land Preservation Level in Terms of Open-Pit Mining. E3s Web Conf. 280, 11002. doi:10.1051/e3sconf/202128011002
Bazaluk, O., Petlovanyi, M., Zubko, S., Lozynskyi, V., and Sai, K. (2021). Instability Assessment of Hanging Wall Rocks during Underground Mining of Iron Ores. Minerals 11 (8), 858. Available from: https://doi.org10.3390/min11080858. doi:10.3390/min11080858
Begalinov, A., Khomiakov, V., Serdaliyev, Y., Iskakov, Y., and Zhanbolatov, A. (2020). Formulation of Methods Reducing Landslide Phenomena and the Collapse of Career Slopes during Open-Pit Mining. E3s Web Conf. 168, 00006. doi:10.1051/e3sconf/202016800006
Begalinov, A., Almenov, T., Zhanakova, R., and Bektur, B. (2020). Analysis of the Stress Deformed State of Rocks Around the Haulage Roadway of the Beskempir Field (Kazakhstan). Min. Miner. Depos. 14 (3), 28–36. doi:10.33271/mining14.03.028
Bekbergenov, D., Jangulova, G., Kassymkanova, K.-K., and Bektur, B. (2020). Mine Technical System with Repeated Geotechnology within New Frames of Sustainable Development of Underground Mining of Caved Deposits of the Zhezkazgan Field. Geodesy and Cartography 46 (4), 182–187. doi:10.3846/gac.2020.10571
Bitimbaev, M. Z., Krupnik, L. A., Krupnik, L. A., Aben, E. K., and Aben, K. K. (2017). Adjustment of Backfill Composition for mineral Mining under Open Pit Bottom. Gornyi Zhurnal (2), 57–61. doi:10.17580/gzh.2017.02.10
Bondarenko, V., Symanovych, G., and Koval, O. (2012). The Mechanism of Over-coal Thin-Layered Massif Deformation of Weak Rocks in a Longwall. Geomechanical Process. During Underground Mining, 41–44. doi:10.1201/b13157-8
Burmin, V. Y., and Shumlianska, L. O. (2017). Spatial Distribution of Earthquake Hypocenters in the Crimea-Black Sea Region. J. Seismol 22 (2), 391–405. doi:10.1007/s10950-017-9712-y
Buzylo, V., Pavlychenko, A., Savelieva, T., and Borysovska, O. (2018). Ecological Aspects of Managing the Stressed-Deformed State of the Mountain Massif during the Development of Multiple Coal Layers. E3s Web Conf. 60 (60), 00013. doi:10.1051/e3sconf/20186000013
Cherniaiev, O. V. (2017). Systematization of the Hard Rock Non-metallic mineral Deposits for Improvement of Their Mining Technologies. Naukovyi Visnyk Natsionalnoho Hirnychoho Universytetu (5), 11–17.
Cheskidov, V., Kassymkanova, K.-K., Lipina, A., and Bornman, M. (2019). Modern Methods of Monitoring and Predicting the State of Slope Structures. E3s Web Conf. 105, 01001. doi:10.1051/e3sconf/201910501001
Dagdelen, K., and Traore, I. (2018). Open Pit Transition Depth Determination through Global Analysis of Open Pit and Underground Mine Production Scheduling. Adv. Appl. Strateg. Mine Plann., 287–296. doi:10.1007/978-3-319-69320-0_19
Dryzhenko, A., Moldabayev, S., Shustov, A., Adamchuk, A., and Sarybayev, N. (2017). Open Pit Mining Technology of Steeply Dipping mineral Occurences by Steeply Inclined Sublayers. Int. Multidisciplinary Scientific GeoConference Surv. Geology. Mining Ecol. Manag. SGEM 17 (13), 599–606. doi:10.5593/sgem2017/13/s03.076
Du, W., and Xing, Z.-g. (2017). Study on the Weight Coefficient Influence of Surface Water on the Stability of Open-Pit Dump. Comparative Analysis of 6 Degree Seismic Simulation. Vibroengineering Proced. 11 (11), 85–90. doi:10.21595/vp.2017.18535
Dychkovskyi, R., Vladyko, O., Vladyko, O., Maltsev, D., and Cáceres Cabana, E. (2018). Some Aspects of the Compatibility of mineral Mining Technologies. Rudarsko-geološko-naftni zbornik 33 (4), 73–82. doi:10.17794/rgn.2018.4.7
Field, E. H. (2007). Overview of the Working Group for the Development of Regional Earthquake Likelihood Models (RELM). Seismological Res. Lett. 78 (1), 7–16. doi:10.1785/gssrl.78.1.7
Fleurisson, J.-A. (2012). Slope Design and Implementation in Open Pit Mines: Geological and Geomechanical Approach. Proced. Eng. 46, 27–38. doi:10.1016/j.proeng.2012.09.442
Gerasymenko, O. O., Shumlyanska, L. O., Nadezhka, L. I., Pivovarov, S. P., Ganiev, O. Z., Ostapchuk, N. M., et al. (2020). Neural Network Modeling in the Problem of Localization Earthquake of Ukraine. Geofizicheskiy Zhurnal 42 (2), 86–98. doi:10.24028/gzh.0203-3100.v42i2.2020.201743
Golik, V. I., Kоmashchenko, V. I., Komashchenko, V. I., Morkun, V. S., Morkun, N. V., and Hryshchenko, S. M. (2018). Energy Saving in Mining Production. Sci. Innov. 14 (3), 29–39. doi:10.15407/scine14.03.029
Haiko, H., Saik, P., and Lozynskyi, V. (2019). The Philosophy of Mining: Historical Aspect and Future Prospect. Philos. Cosmology 22, 76–90. doi:10.29202/phil-cosm/22/6
Jiang, S., Lian, M., Lu, C., Gu, Q., Ruan, S., and Xie, X. (2018). Ensemble Prediction Algorithm of Anomaly Monitoring Based on Big Data Analysis Platform of Open-Pit Mine Slope. Complexity 2018, 1–13. doi:10.1155/2018/1048756
Karatayev, M., and Clarke, M. L. (2014). Current Energy Resources in Kazakhstan and the Future Potential of Renewables: A Review. Energ. Proced. 59, 97–104. doi:10.1016/j.egypro.2014.10.354
Kharisov, T. F. (2018). Problem of Assessment of the Safety Factor of the Open-Pit Sides. Problemy nedroispolzovaniya 3 (18), 108–118.
Kim, D., Langley, R. B., Bond, J., and Chrzanowski, A. (2003). Local Deformation Monitoring Using GPS in an Open Pit Mine: Initial Study. GPS Solutions 7 (3), 176–185. doi:10.1007/s10291-003-0075-1
Krichevsky, S. (2016). New Model of Evolution of Technologies and Prospects of Research with Using Big Data. Philos. cosmology (17), 118–135.
Krylova, L. Y. (1978). Exploration Significance of Iodine Distribution in the Bedrock and Weathering Crust of the Maykain deposit. Int. Geology. Rev. 20 (3), 357–361. doi:10.1080/00206817809471394
Kyrgizbayeva, G., Nurpeisov, M., and Sarybayev, O. (2015). The Monitoring of Earth Surface Displacements during the Subsoil Development. New Dev. Mining Eng. 2015, 161–167. doi:10.1201/b19901-30
Lozynskyi, V., Medianyk, V., Saik, P., Rysbekov, K., and Demydov, M. (2020). Multivariance Solutions for Designing New Levels of Coal Mines. Rudarsko-geološko-naftni zbornik 35 (2), 23–31. doi:10.17794/rgn.2020.2.3
Łukasz, B. (2020). Interpretation of the Results of Mechanical Rock Properties Testing with Respect to Mining Methods. Acta Montanistica Slovaca 25, 81–93. doi:10.46544/AMS.v25i1.8
Malanchuk, Z., Moshynskyi, V., Malanchuk, Y., Korniienko, V., and Koziar, M. (2020). Results of Research into the Content of Rare Earth Materials in Man-Made Phosphogypsum Deposits. Key Eng. Mater. 844 (844), 77–87. doi:10.4028/www.scientific.net/kem.844.77
Malanchuk, Z., Zaiets, V., Tyhonchuk, L., Moshchych, S., Gayabazar, G., and Dang, P. T. (2021). Research of the Properties of Quarry Tuff-Stone for Complex Processing. E3s Web Conf. 280 (280), 01003. doi:10.1051/e3sconf/202128001003
Masloboev, V., Makarov, D., Makarov, D., and Klyuchnikova, E. (2021). Sustainable Development of the Mining Complex of the Murmansk Region: Minimization of Man-Made Impacts on the Environment. Sustainable Dev. Mountain Territories 13 (2), 188–200. doi:10.21177/1998-4502-2021-13-2-188-200
Mikhailova, N. N., and Uzbekov, A. N. (2018). Tectonic and Technogenic Earthquakes in central Kazakhstan. News Natl. Acad. Sci. Republic Kazakhstan, Ser. Geology. Tech. Sci. 3 (429), 146–155.
Mikhailova, N. N., and Uzbekov, A. N. (2018). Tectonic and Technogenic Earthquakes in Central Kazakhstan. News Natl. Acad. Sci. Republic Kazakhstan (429), 137–145.
Moldabayev, S., Adamchuk, A., Sarybayev, N., and Shustov, A. (2019). Improvement of Open Cleaning-Up Schemes of Border Mineral Reserves. Mineral. Reserves. Int. Multidisciplinary Scientific GeoConference 19, 331–338. doi:10.5593/sgem2019/1.3/s03.042
Mora, S., and Keipi, K. (2006). Disaster Risk Management in Development Projects: Models and Checklists. Bull. Eng. Geol. Environ. 65 (2), 155–165. doi:10.1007/s10064-005-0022-1
Moshynskyi, V., Malanchuk, Z., Tsymbaliuk, V., Malanchuk, L., Zhomyruk, R., and Vasylchuk, O. (2020). Research into the Process of Storage and Recycling Technogenic Phosphogypsum Placers. Min. Miner. Depos. 14 (2), 95–102. doi:10.33271/mining14.02.095
Nurpeisova, M. B., and Kurmanbaev, O. S. (2016). Laws of Devolopment of Geomechanical Processes in the Rock Mass Maykain Mine. News Natl. Acad. Sci. Republic Kazakhstan, Ser. Geology. Tech. Sci. 6 (420), 109–115.
Nurpeisova, M. B., Sarybaiev, O. A., and Kurmanbaiev, O. S. (2016). Study of Regularity of Geomechanical Processes Development while Developing Deposits by the Combined Way. Naukovyi Visnyk Natsionalnoho Hirnychoho Universytetu (4), 30–36.
Nurpeisova, M., Donenbaeva, N., and Bek, A. (2019). Devepolment of Geodetic Methods of Monitoring of Open-Pit Sides Stability. Biosci. Biotechnol. Res. Commun., 67–78.
Nurpeisova, M. B., Umirbaeva, A. B., Fedorov, E. V., and Miletenko, N. A. (2021). Assessment of Deformed and Radiological State Territory Based on Integrated Monitoring. Eurasian Mining, 83–87. doi:10.17580/em.2021.01.17
Nurpeissova, M., Bekbassarov, S., Bek, A., Kyrgizbaeva, G., Turisbekov, S., and Ormanbekova, A. (2020). The Geodetic Monitoring of the Engineering Structures Stability Conditions. J. Eng. Appl. Sci. 12 (11), 9151–9163. doi:10.3923/jeasci.2017.9151.9163
Oparin, V. N. (2015). Sovremennye Dostizheniya Geotekhniki Dlya Postroeniya Monitoringovykh Sistem Geomekhaniko-Geodinamicheskoy Bezopasnosti Na Gornodobyvayushchikh Predpriyatiyakh. Fiziko-tekhnicheskie problemy razrabotki poleznykh iskopaemy (2), 6–15.
Pavlychenko, A., and Kovalenko, A. (2013). The Investigation of Rock Dumps Influence to the Levels of Heavy Metals Contamination of Soil. Annu. Scientific-Technical Collection, 237–238. doi:10.1201/b16354-43
Petlovanyi, M., Lozynskyi, V., Saik, P., Sai, K., and Sai, K. (2019). The Influence of Geology and Ore deposit Occurrence Conditions on Dilution Indicators of Extracted Reserves. Rudarsko-geološko-naftni zbornik 34 (1), 83–91. doi:10.17794/rgn.2019.1.8
Rakhmangulov, A., Burmistrov, K., and Osintsev, N. (2021). Sustainable Open Pit Mining and Technical Systems: Concept, Principles, and Indicators. Sustainability 13 (3), 1101. doi:10.3390/su13031101
Rakhmangulov, A., Sladkowski, A., Sladkowski, A., Osintsev, N., and Muravev, D. (2017). Green Logistics: Element of the Sustainable Development Concept. Part 1. Naše more 64 (3), 120–126. doi:10.17818/nm/2017/3.7
Rebetskiy, Yu. L., Sim, L. A., and Kozyrev, A. A. (2017). O Vozmozhnom Mekhanizme Generatsii Izbytochnogo Gorizontal'nogo Szhatiya Rudnykh Uzlov Kol'skogo Poluostrova (Khibiny, Lovozero, Kovdor). Geology. Ore Deposits 59 (4), 263–280. doi:10.7868/s0016777017040049
Rozin, V. (2021). From Engineering and Technological Process to Post-Cultural Technology. Fhi 15, 99–109. doi:10.29202/fhi/15/9
Ryl'nikova, M. V., Yun, A. B., Terent'yeva, I. V., and Esina, E. N. (2016). Vospolnenie Vybyvayushchikh Moshchnostey Rudnikov Na Stadii Dorabotki Balansovykh Zapasov Mestorozhdeniya-Uslovie Ekologicheski Sbalansirovannogo Razvitiya Zhezkazganskogo Regiona. Mine Surv. Bull. (5), 6–10. doi:10.3846/gac.2020.10571
Rysbekov, K., Huayang, D., Huayang, D., Kalybekov, T., Sandybekov, M., Idrissov, K., et al. (2019). Application Features of the Surface Laser Scanning Technology when Solving the Main Tasks of Surveying Support for Reclamation. Min. Miner. Depos. 13 (3), 40–48. doi:10.33271/mining13.03.040
Sach, V. J., Buchner, E., and Schmieder, M. (2020). Enigmatic Earthquake-Generated Large-Scale Clastic Dyke in the Biberach Area (SW Germany). Sediment. Geology. 398, 105571. doi:10.1016/j.sedgeo.2019.105571
Sarybayev, O., Nurpeisova, M., Kyrgizbayeva, G., and Toleyov, B. (2015). Rosk Mass Assessment for Man-Made Disaster Risk Management. New Dev. Mining Eng. 2015, 403–409. doi:10.1201/b19901-70
Satpaev, K. I. (1962). Osnovnye Rezul'taty Kompleksnogo Geologicheskogo Izucheniya I Voprosy Genezisa Dzhezkazgana. Geologiya rudnykh mestorozhdenii 3, 1–14.
Sobko, B., Lozhnikov, O., and Drebenshtedt, C. (2020). Investigation of the Influence of Flooded Bench Hydraulic Mining Parameters on Sludge Pond Formation in the Pit Residual Space. E3s Web Conf. 168, 00037. doi:10.1051/e3sconf/202016800037
Stacey, T. R., Xianbin, Y., Armstrong, R., and Keyter, G. (2003). New Slope Stability Considerations for Deep Open Pit Mines. J. South. Afr. Inst. Mining Metall. 103 (6), 373–389.
Stupnik, M., Kolosov, V., Kalinichenko, V., and Pismennyi, S. (2014). Physical Modeling of Waste Inclusions Stability during Mining of Complex Structured Deposits. Progressive Tech. Coal, Coalbed Methane, Ores Mining, 25–30. doi:10.1201/b17547
Stupnik, M., Kolosov, V., Pysmennyi, S., and Kostiantyn, K. (2019). Selective Mining of Complex Stuctured Ore Deposits by Open Stope Systems. E3s Web Conf. 123, 01007. doi:10.1051/e3sconf/201912301007
Ursul, A., and Ursul, T. (2018). Environmental Education for Sustainable Development. Fhi 9, 115–125. doi:10.29202/fhi/9/12
Viktorov, S. D., Iofis, M. A., and Odintsev, V. N. (2005). Razrushenie Gornykh Porod I Risk Tekhnogennykh Katastrof. Gornyi Zhurnal 4, 30–35.
Waloski, R., Korzeniowski, W., Bołoz, Ł., and Rączka, W. (2021). Identification of Rock Mass Critical Discontinuities while Borehole Drilling. Energies 14, 2748. doi:10.3390/en14102748
Whittle, D., Brazil, M., Grossman, P. A., Rubinstein, J. H., and Thomas, D. A. (2018). Combined Optimisation of an Open-Pit Mine Outline and the Transition Depth to Underground Mining. Eur. J. Oper. Res. 268 (2), 624–634. doi:10.1016/j.ejor.2018.02.005
Zeylik, B., Arshamov, Y., Baratov, R., and Bekbotayeva, A. (2021). New Technology for mineral Deposits Prediction to Identify Prospective Areas in the Zhezkazgan Ore Region. Min. Miner. Depos. 15 (2), 134–142. doi:10.33271/mining15.02.134
Zhanakova, R., Pankratenko, А., Almenov, Т., and Bektur, В. (2020). Rational Selection of the Form of Support for the Formation of Genetic Composition of Rocks in the Conditions of the Beskempir Field. NATIONAL ACADEMY SCIENCES REPUBLIC KAZAKHSTAN Inst.itute of Plant Biology and Biotechnology 1, 106–113. doi:10.32014/2020.2518-170x.13
Zhironkin, S., and Cehlár, M. (2021). Coal Mining Sustainable Development: Economics and Technological Outlook. Energies 14, 5029. doi:10.3390/en14165029
Keywords: deposits, fracturing, rocks, stress–strain state, mining operations, monitoring, mass stability, deposits
Citation: Bazaluk O, Rysbekov K, Nurpeisova M, Lozynskyi V, Kyrgizbayeva G and Turumbetov T (2022) Integrated Monitoring for the Rock Mass State During Large-Scale Subsoil Development. Front. Environ. Sci. 10:852591. doi: 10.3389/fenvs.2022.852591
Received: 11 January 2022; Accepted: 14 March 2022;
Published: 26 April 2022.
Edited by:
Biswajeet Pradhan, University of Technology Sydney, AustraliaReviewed by:
Jianwei Cheng, China University of Mining and Technology, ChinaIzabela Jonek-Kowalska, Silesian University of Technology, Poland
Copyright © 2022 Bazaluk, Rysbekov, Nurpeisova, Lozynskyi, Kyrgizbayeva and Turumbetov. This is an open-access article distributed under the terms of the Creative Commons Attribution License (CC BY). The use, distribution or reproduction in other forums is permitted, provided the original author(s) and the copyright owner(s) are credited and that the original publication in this journal is cited, in accordance with accepted academic practice. No use, distribution or reproduction is permitted which does not comply with these terms.
*Correspondence: Vasyl Lozynskyi, bHZnLm5tdUBnbWFpbC5jb20=; Guldana Kyrgizbayeva, Zy5reXJnaXpiYXlldmFAc2F0YmF5ZXYudW5pdmVyc2l0eQ==