- Department of Biology, University of Padova, Padova, Italy
Although the presence of pharmaceutical and personal care products in aquatic ecosystems is well documented, little information is available about their sublethal effects, on aquatic invertebrates. From an ecotoxicological point of view, the use of in vitro approaches has been recommended as a tool to assess adverse effects and to understand the mechanisms of action of chemicals at the cellular level. In the present in vitro study, the effects of Amoxicillin (AMX), Trimethoprim (TMP) and Ciprofloxacin (CIP) (1 µg/L, each) were tested alone and—for the first time—as a mixture (MIX) on haemocytes of the clam Ruditapes philippinarum. After the exposure, a battery of cellular parameters was evaluated, such as haemocyte viability, lysosomal membrane stability, superoxide anion production, acid phosphatase activity, the frequency of micronuclei and chromosomal aberrations. The results demonstrated that AMX, TMP, CIP and MIX affected lysosomal membrane stability, as well as superoxide anion and acid phosphatase production, and promoted chromosomal aberrations. This study highlighted that Manila clam haemocytes are a sensitive cell model to assess the effects of exposure to pharmaceutical products on non-target species. Our study demonstrated that the effects of pharmaceutical mixtures on marine species should be experimentally evaluated because they are not predictable from single exposures as the compounds can interact in different ways on the various biological endpoints considered.
1 Introduction
Pharmaceuticals and personal care products (PPCPs) are a wide group of chemicals. These bioactive compounds are used in both medicine and body personal care and the occurrence of PPCPs in aquatic environments has garnered great attention over the past 30 years (Schumock et al., 2014; Yang et al., 2017). PPCPs are classified as emerging contaminants due to their frequent detection in various water bodies at concentrations ranging from ng/L to µg/L (Dai et al., 2015; Yang et al., 2017; Zhang et al., 2020). Despite their high transformation rates, PPCPs are considered pseudo-persistent contaminants, since their degradation is balanced by a constant release into aquatic environments (Bottoni and Caroli, 2018).
Pharmaceuticals are divided in many classes, such as antibiotics, anti-inflammatory, analgesics, hormones, and anti-hypertensives (Zhang et al., 2017). They are discharged by many sources, largely represented by domestic wastewaters, improper manufacturer disposal and hospitals; however, they are mainly released by agriculture and aquaculture wastewaters (Yang et al., 2017; Bottoni and Caroli, 2018). In the environment, usually low but stable concentrations of pharmaceuticals, suggest prevailing chronic rather than acute effects (Fent et al., 2006; Zhang et al., 2017; Srain et al., 2021).
Antibiotics are one of the most widely used group of pharmaceutical compounds: for instance, in the United States the antibiotic usage was 17.900 tons in 2011/2012, while in China about 162.000 tons had been used in 2013 (Zhang et al., 2015). Moreover, the Organization for Economic Cooperation and Development (OECD) predicted a dramatic increase of antibiotic consumption in all livestock systems rising by 67% by 2030, with China and the United States as main contributors (Van Boeckel et al., 2015). Moreover, antibiotics are not only used as medical compounds, but also as agents to accelerate growth in livestock (Zhao et al., 2021). In this regard, the Food and Drug Administration of United States estimated that almost 80 percent of the total antibiotics was consumed for farm animals, with about 83% of antibiotic usage for prophylaxis or growth promotion (McEwen and Fedorka-Cray, 2002). Furthermore, half of the annual antibiotic production in China went into the animal industry with a total amount of 105,000 tons (Collignon and Voss, 2015). Antibiotics are found in water bodies near livestock and aquaculture sites, and most of the antibiotics provided are not completely metabolized and are directly excreted (Sweetman, 2005; Sarmah et al., 2006; Ju et al., 2019). In addition, the data from 76 countries, indicate that the total global antibiotic consumption rate grew by 39% between 2000 and 2015, up to 42.3 billion defined daily doses (DDDs) (Klein et al., 2018). This growth is caused by both world’s population increase and its food demand (Van Boeckel et al., 2015; Klein et al., 2018). Indeed, the global antibiotic consumption for livestock has been estimated at 63,200 tons in 2010, probably more than all human consumption (Van Boeckel et al., 2015; Pulicharla et al., 2017). Furthermore, increased demand for fishery products has promoted the intensification of aquaculture production, which in turn has led to an increasing usage of antibiotics for both prevention and treatment of bacterial diseases (Cañada-Cañada et al., 2009; De la Casa-Resino et al., 2021). In the European Union, Italy has one of the highest antibiotic consumption rates, with approximately 21.4 DDD/1,000 inhabitants die in 2018 (AIFA, 2019).
The antibiotics used in this study were Amoxicillin (AMX), Trimethoprim (TMP) and Ciprofloxacin (CIP). AMX is one of the most worldwide used β-lactams antibiotic, in both human and veterinary medicine for the treatment of gastrointestinal and system infections (Matozzo et al., 2016; De Franco et al., 2017). In 2000, AMX was the most-sold antibiotic compound in United Kingdom veterinary (Sarmah et al., 2006), while in 2007, AMX was the most-consumed antibiotic in France (Sabuncu et al., 2009). Leung et al. (2012) demonstrated that in sewage treatment plants in Hong Kong the AMX concentrations were 261 + 3 ng/L and 66
TMP is a synthetic sulphonamide that inhibits the dihydrofolate reductase; due to its anti-inflammatory, antioxidant, and neuroprotective properties, it is widely used in both human and veterinary medicine to treat gastrointestinal and respiratory tract infections (Sweetman, 2005; Gust et al., 2012). TMP is usually present in wastewater, at concentrations from tens to hundreds of ng/L (Carvalho and Santos, 2016). TMP concentration ranged from 124
CIP is a fluoroquinolone that inhibits the gyrase B. In both human and veterinary medicine, it is the most used fluoroquinolone in eighteen European countries (Ferech et al., 2006; Gust et al., 2012; Papich, 2017). CIP is hardly biodegraded (Carabineiro et al., 2012), and in Italian rivers, CIP was observed at a concentration up to 60,1 ng/L (Grobin et al., 2022). Furthermore, in 2019, Zhang et al. (2020) observed, that CIP level was higher in the wet season (3.453 ± 0.979 ng/L) than in the dry season (3.091 ± 0.824 ng/L) in the Yinma River in Northeast China. CIP was also observed at a mean value of 83 ng/L in summer and 3,120 ng/L in winter in hospital effluents located in Turkey (Aydin et al., 2019). Furthermore, Kostich et al. (2014) demonstrated that CIP concentration was around 67 ng/L in effluents from wastewater treatment plants across the United States. Nevertheless, Feitosa-Felizzola and Chiron (2009) detected ciprofloxacin at maximum concentration of 9.7 μg L− 1 in Arc River (France). In Gościcina and Reda Rivers (Poland) CIP was found at 2.7 μg L− 1 (Wagil et al., 2014), while in the Spanish River Llobregat reported at 2 μg/L (Lòpez-Roldán et al., 2010). In coastal waters up to 109 ng/L of ciprofloxacin were recorded in coastal waters (Lu et al., 2018) and up to 6.4 ng/L in seawater (Čelić et al., 2019). Lastly, ciprofloxacin concentrations of 1.56–7.14 ng/L were detected in the estuary of Tejo River (Portugal) (Reis Santos et al., 2018).
Considering the widespread presence of antibiotics in aquatic ecosystems, it is crucial to assess the potential toxic effects of AMX, TMP, CIP, and antibiotics in general, to non-target aquatic organisms, such as marine invertebrates, whose chronic exposure to these compounds can cause detrimental effects (Kostich et al., 2014; Matozzo, 2014; Fabbri, 2015; Yang et al., 2017; Zhang et al., 2020). The aim of the present study was to evaluate the effects of the three antibiotics, both alone and as a mixture, on in vitro-exposed haemocytes of the Manila clam Ruditapes philippinarum, one of the major fishery and aquaculture species in the world, widely used in ecotoxicological studies (Matozzo and Marin, 2010; Liu et al., 2011). Haemocytes are the main immune effectors in clams, responsible for both cellular and humoral defense. They are also involved in many physiological processes, such as tissue repair and shell production, and are known to be susceptible to environmental modifications and toxicant exposure, acting as sentry cells. (Donaghy et al., 2009). Evidence of morphological and functional alterations in haemocytes can represent a first tool to screen toxicity and mode of action of environmental contaminants and their mixtures.
2 Materials and Methods
2.1 Acclimatization of R. philippinarum
Clams of 3.5–3.8 cm shell length were collected from licensed areas for bivalve culture in the southern basin of the Venice Lagoon (Italy). Animals were acclimatized for 7 days in a 50 L aquarium filled with seawater (salinity of 35 and temperature of 17°C ± 0.5°C) under constant aeration with sandy bottom (10–15 cm thick). Seawater was renewed every 2 days and the clams were fed with a microalgae mixture (Tetraselmis chuii and Phaeodactylum tricornutum).
2.2 In vitro Assays
Several assays, each repeated twice, were sequentially performed on clam haemocytes. For each assay, a pool of hemolymph from six clams was used and five experimental conditions were assessed in duplicate: control in filtered sea water (FSW), AMX, TMP, CIP and antibiotic mixture (MIX). The antibiotics were tested at an environmentally realistic concentration of 1 µg/L in FSW, both alone and as a mixture. Haemolymph was drawn from the anterior adductor muscle with a 1-ml plastic syringe and pooled adding 0.5 ml of 0.38% sodium citrate in 0.45 µm FSW (pH of 7.5) to prevent clotting. The pool was centrifuged at 780 g for 10 min at 4°C. Afterwards, the pellet was carefully resuspended in FSW. Briefly, 60 µL of haemocyte suspension were placed in culture chambers made by a Teflon ring (15 mm internal diameter and 1 mm thick) smeared with petroleum jelly, glued to a siliconized glass slide, and covered with a coverslip. Chambers were kept upside down for 30 min at room temperature to allow haemocytes to adhere to coverslips (Ballarin et al., 1994). Then, the FSW was removed and replaced with an equal volume of FSW (control) or antibiotic solutions and the exposure lasted 60 min. Afterwards, the solutions were removed, and the cells were processed as described below. At least 100 cells per slide were observed under a light microscope (LM) (Leica DMLB) at 1,000X.
2.2.1 Haemocyte Viability Assay
Treated and untreated haemocytes were stained with 60 µL of Trypan blue (0.25% in FSW) for 5 min. The in vivo count of alive and dead cells (stained in blue) was performed under LM. Haemocyte viability was reported as the percentage of alive cells.
2.2.2 Haemocyte Morphology
After the exposure, haemocytes were fixed for 30 min at 4°C (1% glutaraldehyde and 1% sucrose in FSW), washed in phosphate-buffered saline (PBS: 1.37 M NaCl, 0.03 M KCl, 0.015 M KH2PO4, 0.065 M Na2HPO4, pH 7.2) for 10 min, stained for 10 min in 10% Giemsa solution, washed in distilled water, and mounted on glass slides with Aquovitrex (Carlo Erba, Milan, Italy). The slides were then observed under the LM at 1,000X. The percentage of stained haemocytes with a round shape was then estimated.
2.2.3 Neutral Red Retention Assay
Neutral Red (NR) retention assay allows to evaluate the lysosome membrane stability. Briefly, after the removal of the treatment solution, 60 µL of NR solution (0.0008%) were added and haemocytes were incubated for 10 min at room temperature to allow the uptake of the staining solution (Lowe, 1995). Then, haemocytes were observed in vivo under LM at 1,000X. One hundred cells per slide were observed. The results were expressed as percentage of damaged haemocytes (reddish pink cytoplasm).
2.2.4 Hydrolytic Enzymes
After the exposure, the haemocytes were fixed as described above and incubated in specific reaction mixture.
- β-glucuronidase: fixed haemocytes were washed for 10 min in 0.1 M sodium acetate buffer (pH 5.2) and incubated for 2 h at 37°C with a mixture containing 4 mg naphthol AS-BI β-glucuronide (Sigma) dissolved in 250 µL dimethylformamide (DMF), 400 µL solution A [0.4 g pararosaniline (Fluka), 2 ml HCl 37%, 8 ml distilled water], 400 µL solution B (4% NaNO2 in distilled water) and 20 ml of 0.1 M sodium acetate buffer (pH 5.2) (Hayashi et al., 1964). Haemocytes were then washed for 10 min in sodium acetate buffer and mounted using Aquovitrex. Positive haemocytes were stained red.
- Acid phosphatase: after the fixation, haemocytes were washed for 10 min in 0.1 M sodium acetate buffer, pH 5.2, and then incubated for 1 h at 37°C in a mixture like that described before, but containing 10 mg naphthol AS-BI phosphate (Sigma) previously dissolved in 400 µL DMF as substrate (Cima et al., 2000). Positive haemocytes were stained red.
- Alkaline phosphatase: fixed haemocytes were washed with Tris/HCl (pH 9.0) for 10 min. Then, they were incubated for 2 h at 37°C with a mixture containing 0.05 g of naphthol AS-BI phosphate in 2 ml of DMFA, 2 ml of solution A, 2 ml of solution B and raised up to 100 ml by adding TRIS/HCl (Burstone, 1962) After a second wash, the coverslips were mounted using Aquovitrex. Results were expressed as percentage of positive (violet) cells.
- 5′ Nucleotidase: after exposure, the coverslips were washed with Tris/maleate buffer for 10 min and incubated for 2 h at 37°C with the reaction mixture (0.04 g of adenosine-5′-monophosphate dissolved in 44 ml of distilled water, 40 ml of Tris/maleate buffer, 6 ml of 2% lead nitrate and 10 ml of 2.5% magnesium sulfate). After the incubation, the coverslips were washed with 0.2% ammonium sulphide for 2 min and then with distilled water for 5 min before being mounted (Wachstein and Meisel, 1957). The results were expressed as percentage of positive cells (stained black).
- Peroxidase: after exposure, the coverslips were washed with PBS buffer for 10 min and then incubated for 2 h at 37°C with the reaction mixture (0.05 g of 3–3’ diaminobenzidine tetrahydrochloride in 100 ml of distilled water with 50 µL of 30% hydrogen peroxide) (Graham and Karnovsky, 1966). The coverslips were washed with distilled water for 10 min and mounted using Aquovitrex. The results were expressed as percentage of positive cells (stained brown).
2.2.5 Intracellular Superoxide Anion
Intracellular superoxide anion was detected using the method of Song and Hsieh (1994). After the exposure, 60 µL of the antibiotic solutions were removed and replaced by 60 µL of nitro blue tetrazolium chloride (NBT) 0.2% solution, and incubated for 1 h at room temperature, fixed as described above and washed for 10 min in PBS. The reaction product (formazan) was solubilized by adding a solution containing 120 μL 2 M KOH and 140 μL DMSO for 30 min. The slides were then mounted and the results were expressed as percentage of positive (blue) cells.
2.2.6 Micronucleus Assay (MN)
Micronucleus test, which allows the detection of genotoxic damage, was performed according to the method of Pavlica et al. (2000). After 1 h of exposure of haemocytes to antibiotics in Eppendorf tubes, 150 µL of haemolymph were placed on a microscopy slide and left for 15 min in a humidified chamber at room temperature to allow haemocyte adhesion. The haemocytes were then fixed with a glutaraldehyde solution (1% in FSW) for 5 min. The slides were washed with phosphate-buffered saline (PBS), stained with Hoechst dye 33,258 solution (1 µg/ml) for 5 min, rewashed with PBS, mounted in glycerol-McIlvaine buffer (1:1), covered with a coverslip and kept in the dark at 4°C until examination. The slides were observed under an Olympus CX31 fluorescent microscope, equipped with a submerged lens at 1,000X magnification. Two hundred nuclei were counted for each slide. Micronuclei and chromosome aberrations (e.g., multipolar, or multinucleated cells, eight-shaped nuclei) were identified according to Kirsch-Volders et al. (2000) and Pavlica et al. (2000), and the results were expressed as the MN frequency and aberration frequency (‰).
2.2.7 Spectrophotometric Assays
NR uptake assay was performed according to the procedure reported in previous studies (Cajaraville et al., 1996; Matozzo et al., 2002). The assay evaluates the capability of haemocytes to carry out endocytosis. In detail, 500 µL of pooled haemolymph was centrifuged at 780 g for 10 min and haemocytes were resuspended in an equal volume of FSW (control) or antibiotic solutions. After the exposure, haemocytes were centrifuged at 780 g for 10 min, resuspended in an equal volume of NR dye solution (0.0008%) in FSW, and incubated at room temperature for 30 min. Again, they were centrifuged at 780 g for 10 min, re-suspended in distilled water, and centrifuged at 12,000 g for 5 min at 4°C. The supernatant (haemocyte lysate) was collected and the absorbance was recorded using a Beckman 730 spectrophotometer at 550 nm. The results were expressed as optical density per ml of haemolymph (OD550/ml haemolymph).
As for phenoloxidase (PO) activity, after the exposure to antibiotics, haemocytes were centrifuged at 3,000 g for 10 min at room temperature. After the centrifugation, the supernatant was removed, and cells were resuspended in PBS. Then, in a new tube 100 µL of sample in PBS with 900 µL of l-DOPA were added and incubated at 37°C for 30 min. The supernatant was collected and the absorbance was recorded using a Beckman 730 spectrophotometer at 490 nm. The results were expressed as optical density per U of phenoloxidase/mg of protein.
2.3 Statistical Analysis
The ANOVA assumptions (normal distribution and homogeneity of the variance) were assessed. The STATISTICA 13.4 software package (StaSoft, Tulsa, OK) was used to perform the one-way ANOVA and the LSD post-hoc test. Data are reported as mean ± standard error (SE, n = 4). Asterisks denote significant differences with respect to controls: *p < 0.05, **p < 0.01, ***p < 0.001.
3 Results
Based on the Trypan Blue assay, the tested concentrations of the 3 antibiotics and their mixture did not significantly affect haemocyte viability (ANOVA: F = 1.19, p = 0.354) (Figure 1A, Supplementary Figures S1A,S1B).
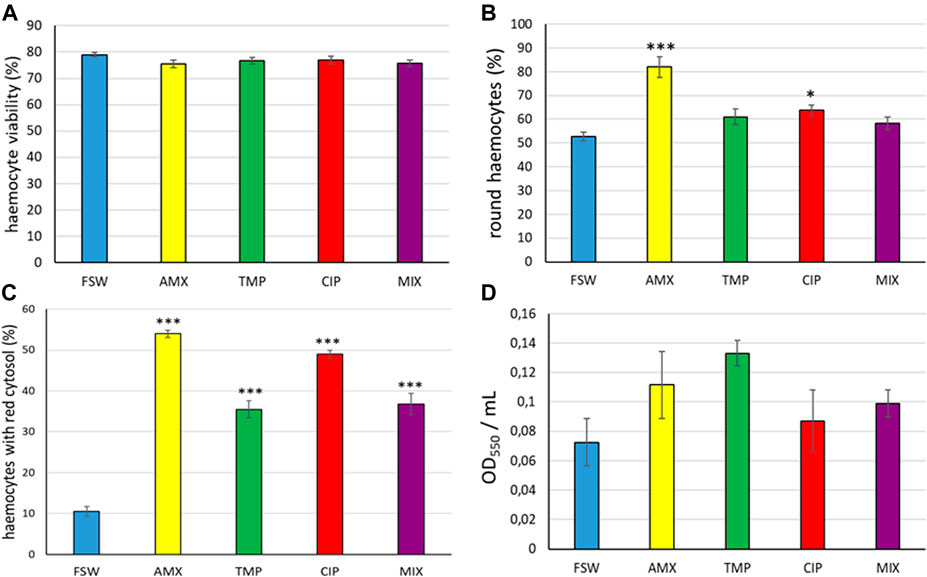
FIGURE 1. Haemocytes of R. philippinarum exposed to AMX, TMP, CIP and MIX. (A) Percentage of viable haemocytes. (B) Percentage of round-shaped haemocytes stained with Giemsa. (C) Percentage of haemocytes showing Neutral Red loss into the cytoplasm. (D) Neutral Red uptake by haemocytes. Values are means +SD, n = 4. Asterisks denote significant differences with respect to controls (FSW): *p < 0.05, **p < 0.01, ***p < 0.001.
Conversely, the treatment significantly affected the percentage of round cells (ANOVA: F = 13.45, p = 0.000). In detail, the LSD post-hoc test revealed a significant increase of the percentage of round-shaped cells after exposure to AMX (p < 0.001) and CIP (p < 0.05) (Figure 1B, Supplementary Figure S1C).
In addition, the NR retention assay highlighted that the three antibiotics and their mixture significantly affected the lysosomal membrane stability of haemocytes (ANOVA: F = 99.92, p = 0.000). In all treatments, the LSD post-hoc test revealed a significant (p < 0.001) increase in the percentage of haemocytes showing dye loss from lysosomes into cytosol when compared with the control (Figure 1C, Supplementary Figure S1D). However, based on the spectrophotometric NR uptake assay, in vitro exposure to the 3 antibiotics and their mixture did not significantly affect (ANOVA: F = 1.93, p = 0.156) the endocytotic capability of haemocytes (Figure 1D).
As for intracellular superoxide anion, the exposure to antibiotics significantly increased the intracellular production of O2− in haemocytes (ANOVA: F = 25.13, p = 0.000). In pairwise comparisons, the percentage of positive cells significantly increased in cells treated with AMX, CIP and MIX (p < 0.001) and in TMP (p < 0.01), with respect to the control (Figure 2A, Supplementary Figure S1E).
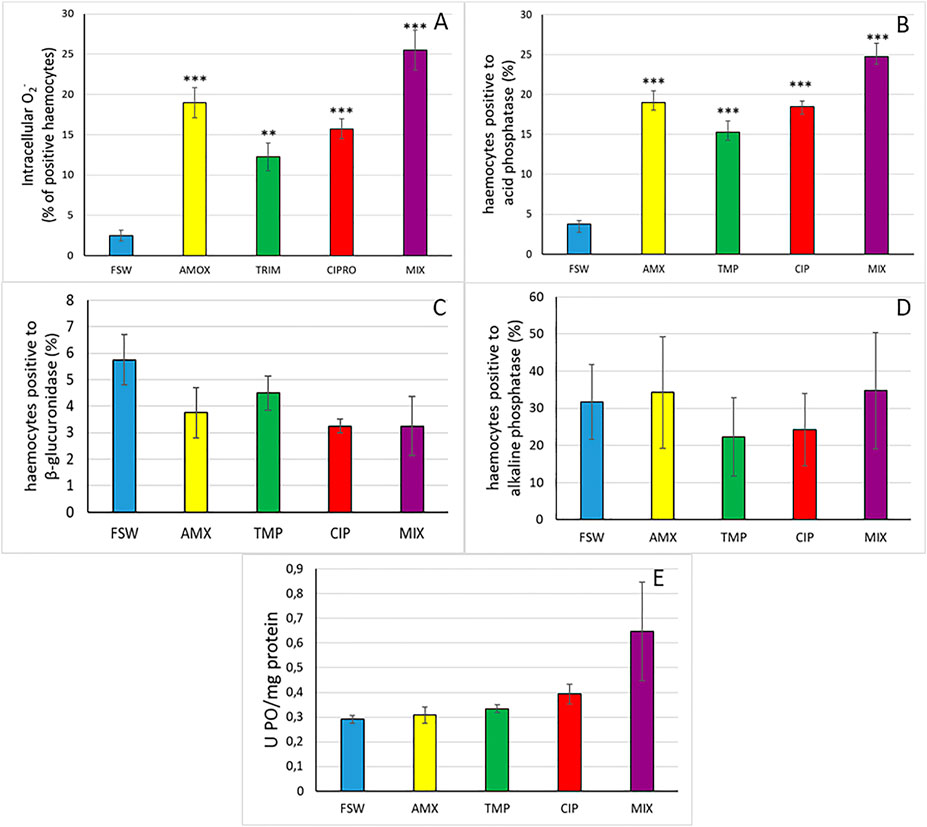
FIGURE 2. (A) Percentage of haemocytes with blue precipitate of formazan, denoting O2− production. (B) Percentage of haemocytes positive to acid phosphatase activity. (C) Percentage of haemocytes positive to β-glucuronidase activity. (D) Percentage of haemocytes positive to alkaline phosphatase activity. (E) Phenoloxydase activity in haemocytes. Asterisks denote significant differences with respect to controls (FSW): *p < 0.05, **p < 0.01, ***p < 0.001.
The percentage of haemocytes positive to acid phosphatase was significantly affected by treatment (ANOVA: F = 39.80, p < 0.001). In particular, the percentage of cells with red-orange granules showed a significant increase (p < 0.001) in all treatments, compared to control (Figure 2B, Supplementary Figure S1F).
On the contrary, β-glucuronidase (ANOVA: F = 1.58, p = 0.228) (Figure 2C, Supplementary Figure S1G), alkaline phosphatase (ANOVA: F = 0.21, p = 0.924) (Figure 2D, Supplementary Figure S1H) and phenoloxydase (ANOVA: F = 2.50, p = 0.086) activities were not influenced by the treatments (Figure 2E). Similarly, 5’ nucleotidase and peroxidase remained unchanged after the exposure (ANOVA: F = 0.46, p = 0.758 and ANOVA: F = 0.57, p = 0.687, respectively) (Figures 3A,B, Supplementary Figures S1I,S1J).
The micronucleus assay revealed that antibiotics did not significantly increase the MN frequency (ANOVA: F = 2.50, p = 0.086) (Figures 4A, 5). However, we observed a significant effect of treatment on the chromosomal aberration frequency (ANOVA: F = 10.72, p < 0.001) (Figures 4B, 5), with a significant increase in the frequency of aberrations at all treatments (p < 0.001 for AMX and TMP treatments, p < 0.01 for CIP and MIX, respectively).
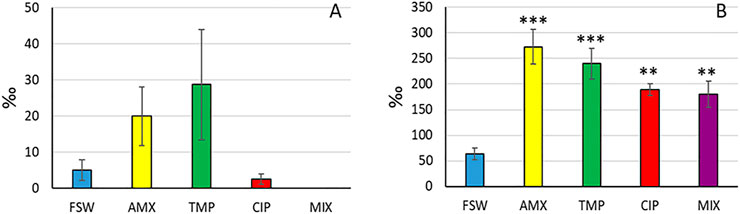
FIGURE 4. Frequency of micronuclei (A) and chromosomal aberrations (B) in haemocytes. Asterisks denote significant differences with respect to controls (FSW): *p < 0.05, **p < 0.01, ***p < 0.001.
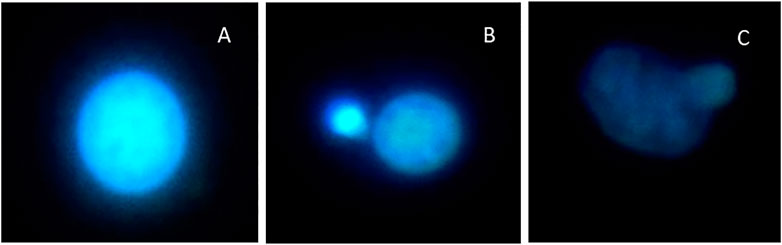
FIGURE 5. Chromosomal aberrations and micronuclei in R. philippinarum haemocytes following exposure to antibiotics and their mixture. (A) Normal nucleus. (B) Micronucleus. (C) Multipolar cell.
4 Discussion
In the present study, clams’ haemocytes were exposed in vitro to assess the potential toxicity of three antibiotics, such as Amoxicillin, Trimethoprim, Ciprofloxacin, and a mixture of all of them. Although the presence of AMX, TMP, CIP is continuously reported in aquatic environments, in-depth knowledge of potential toxicity of these pharmaceuticals, alone or as a mixture, to non-target aquatic invertebrates, such as bivalves, is scarce.
Based on the results of Trypan Blue assay, all the tested concentrations of the antibiotics were sublethal. However, all the antibiotics and their mixture strongly affected the lysosomal membrane stability of haemocytes. Similarly to our results, Binelli et al. (2009a) observed a significant destabilization of lysosomes with a significant decrease in NR retention time in in vitro exposed haemocytes of Dreissena polymorpha to TMP. In the same species, after an in vivo exposure to TMP the lysosomal membrane stability was reduced in a dose- and time-dependent manner (Binelli et al.,2009b). However, we did not observe detrimental effects on endocytotic capability of haemocytes assessed by the NR uptake assay.
The antibiotics tested in our study increased the proportion of round-shaped cells, probably as a consequence of detrimental effects on the cytoskeleton as previously observed in haemocytes in vitro exposed to PFAS (Fabrello et al., 2021). In a previous study, Matozzo et al. (2015) observed that the haemocyte volume was significantly lower in clams exposed for 1 day to 600 ng/L TMP and significantly higher in those exposed for 3 days to 900 ng/L TMP compared to the controls. In that study, haemocyte proliferation increased significantly in clams exposed for 1 and 3 days to 900 ng/L TMP and in those exposed for 7 days to 300, 600 and 900 ng/L TMP compared with control clams.
Alterations of enzyme activities were observed in haemocytes of bivalves exposed to various contaminants, such as glyphosate (Matozzo et al., 2018; Matozzo et al., 2019) and C6O4—a substitute of perfluorooctanoic acid— (Fabrello et al., 2021). Hydrolytic enzymes play an important role in immune and oxidative stress defense, acting against both pathogens and ROS production following contaminant exposure. In this study, both intracellular superoxide anion and acid phosphatase assays showed an increase in the percentage of positive haemocytes in all treatments, when compared to control. In particular, the percentage of positive cells to intracellular superoxide anion increased by 5–12 folds with respect to control. In addition, the same pattern of variation was observed among treatments in the two cytochemical assays, with the lowest values in TMP-treated cells and the highest in the mixture-treated cells. Like in our study, TMP was shown to alter enzyme activities in previous studies. For example, TMP increased lactate dehydrogenase activity (indicative of cell damage) in clam hemolymph after 3 and 7 days of exposure to 300, 600 and 900 ng/L TMP, compared to controls (Matozzo et al., 2015). TMP also decreased the lysozyme activity in the haemocyte lysate (HL) of clams exposed for 1 day to 600 ng/L TMP and in those exposed for 3 days to 600 and 900 ng/L TMP. In addition, TMP was shown to highly promote the phagocytosis of in vitro treated haemocytes from Elliptio complanata (Gagné et al., 2006). Acid phosphatase increased by 3–5 fold in our experiment after exposure to antibiotics. On the contrary, the percentage of cells positive to the other two hydrolytic enzymes, alkaline phosphatase and β-glucuronidase, was not altered.
Previous studies demonstrated that antibiotics alter the antioxidant system and induce oxidative stress. In Cyprinus carpio, Elizalde-Velazquez, et al. (2017) observed that AMX and amoxicilloic acid induced oxidative stress and oxidative damage in several organs of animals exposed to the antibiotic (10 ng/L, 10 µg/L, 10 mg/L). Similar results were obtained by González-González et al. (2021) in zebrafish embryos o exposed at 0.039 µg/L of AMX. Moreover, in haemocytes of the freshwater bivalve Elliptio complanata, TMP increased the intracellular ROS level at all the tested concentrations (0.2, 1, 5 and 25 times the recorded effluent concentration of 50 ng/L) and at the highest CIP concentration (2.5 µg/L) (Gust et al., 2012). Interestingly, in the same study, the mixture of six antibiotics (including TMP and CIP), also increased the ROS intracellular levels at the 5x and 25x tested concentrations. An increase of the immunocapacity and immunoefficiency of haemocytes was observed after TMP, CIP, and mixture exposure (Gust et al., 2012). Oxidative damage was observed after exposure of Danio rerio embryos at high concentrations of paracetamol, CIP, and their mixture, resulting in alterations of zebrafish embryonic development (Rosas-Ramírez et al., 2022). Moreover, in the fish Cirrhinus mrigala exposed to environmentally realistic CIP concentrations (1 μg/L and 1.5 μg/L) there was an increase of the superoxide dismutase activity (SOD) and lipid peroxidation in gills, liver, and kidney, whereas catalase activity (CAT) decreased in gill and liver, but significantly increased in kidney. Moreover, CIP promoted a series of histological anomalies in gills, liver, and kidney tissues (Ramesh et al., 2021). Indeed, antibiotics can also induce malformations affecting the normal development as demonstrated by González-González et al. (2021) in zebrafish embryos exposed to AMX.
The Micronucleus test is one of the most-used biomarkers of genotoxicity in aquatic organisms (Bolognesi and Fenech, 2012), in both laboratory and field conditions (Crupkin et al., 2021; Musrri et al., 2021) (Figure 4A). In this study, there was no significant increase of micronucleus frequency in R. philippinarum haemocytes following exposure to the antibiotics tested. Conversely, MN frequency was found significantly increased in haemocytes from both clams (R. philippinarum) and mussels (M. galloprovincialis) exposed in vivo to AMX (Matozzo et al., 2016). However, in that study the tested concentrations were 1,000 and 4,000 higher than ours. In the blood cells of Cyprinus carpio, AMX induced DNA damage and cytotoxic effects with a significant increase of micronucleus frequency and DNA fragmentation at environmentally realistic concentrations of 0.039 µg/L and 1.67 µg/L (Orozco-Hernández et al., 2019). Furthermore, in an in vitro study, Lacaze et al. (2015) observed that TMP was genotoxic at 200 µg/L and immunotoxic at 20 mg/L on haemocytes of Mytilus edulis. Parolini et al. (2013) observed an increase in the percentage of apoptotic haemocytes of Dreissena polymorpha after 72 and 96 h of exposure to 1 nM (corresponding to 290 ng/L) of TMP. However, the NR retention time did not change and a slight but not significant increase in the micronuclei percentage was found. Interestingly, we observed an increase in the frequency of chromosomal aberrations after all treatments (Figure 4B), with AMX- and TMP-treated haemocytes showing the highest values. This result suggested that more evident genotoxic damage could be revealed by longer haemocyte exposure time, as recently observed in clam haemocytes exposed in vitro the new PFAS C6O4 (Fabrello et al., 2021). A significant increase in genetic damage index was detected in Daphnia magna starting from 0.013 mg/L of ciprofloxacin (Nunes et al., 2017).
Lastly, Trombini et al. (2021) evaluated the toxicity effects of mixtures of ciprofloxacin, flumequine and ibuprofen at low and high concentrations (10 and 100 μg/L) on the crayfish Procambarus clarkii over 21 days of exposure. Alterations were observed in activity of antioxidant and biotransformation enzymes, as well as in proteins involved in carbohydrate metabolism and immune response. However, the effects of the single pharmaceuticals were not assessed in that study (Trombini et al., 2021).
5 Conclusion
In this study, we tested the hypothesis that AMX, TMP, CIP and the mixture affect functional characteristics of haemocytes of a non-target species, R. philippinarum, an ecologically and commercially relevant bivalve species. Overall, results highlighted increased oxidative stress in cells exposed to the antibiotics. The increased intracellular production of superoxide anion probably resulted in damage to lysosomal membranes and increased chromosomal aberrations, even though cell viability did not change. The exposure to the antibiotic mixture did not always result in an additive effect, when compared with the single antibiotics. Indeed, the most detrimental effects on lysosomal membrane stability, cell shape and aberration frequency were observed in AMX treated cells. This suggested potential antagonistic interactions among antibiotics in the mixture. In conclusion, efforts should be addressed at evaluating and comparing effects of both individual compounds and their mixtures, in order to highlight a more realistic risk to aquatic organisms, with particular attention to marine coastal species which have been scarcely considered in previous studies.
Data Availability Statement
The raw data supporting the conclusion of this article will be made available by the authors, without undue reservation.
Author Contributions
MM and VM designed the study. GN, JF, DA, and MC performed the experiments. JF and VM conducted the data analysis. All authors participated in the initial draft of the manuscript. MM supervised the study.
Conflict of Interest
The authors declare that the research was conducted in the absence of any commercial or financial relationships that could be construed as a potential conflict of interest.
Publisher’s Note
All claims expressed in this article are solely those of the authors and do not necessarily represent those of their affiliated organizations, or those of the publisher, the editors and the reviewers. Any product that may be evaluated in this article, or claim that may be made by its manufacturer, is not guaranteed or endorsed by the publisher.
Supplementary Material
The Supplementary Material for this article can be found online at: https://www.frontiersin.org/articles/10.3389/fenvs.2022.845213/full#supplementary-material
References
AIFA (2019). Osservatorio Nazionale sull'Impiego dei Medicinali. L'uso degli antibiotici in Italia. Rapporto Nazionale 2018. Roma, Italy: Agenzia Italiana Del Farmaco.
Alygizakis, N. A., Gago-Ferrero, P., Borova, V. L., Pavlidou, A., Hatzianestis, I., and Thomaidis, N. S. (2016). Occurrence and Spatial Distribution of 158 Pharmaceuticals, Drugs of Abuse and Related Metabolites in Offshore Seawater. Sci. Total Environ. 541, 1097–1105. doi:10.1016/j.scitotenv.2015.09.145
Andreozzi, R., Caprio, V., Ciniglia, C., de Champdoré, M., Lo Giudice, R., Marotta, R., et al. (2004). Antibiotics in the Environment: Occurrence in Italian STPs, Fate, and Preliminary Assessment on Algal Toxicity of Amoxicillin. Environ. Sci. Technol. 38, 6832–6838. doi:10.1021/es049509a
Aydin, E., and Talinli, I. (2013). Analysis, Occurrence and Fate of Commonly Used Pharmaceuticals and Hormones in the Buyukcekmece Watershed, Turkey. Chemosphere 90, 2004–2012. doi:10.1016/j.chemosphere.2012.10.074
Aydin, S., Aydin, M. E., Ulvi, A., and Kilic, H. (2019). Antibiotics in Hospital Effluents: Occurrence, Contribution to Urban Wastewater, Removal in a Wastewater Treatment Plant, and Environmental Risk Assessment. Environ. Sci. Pollut. Res. 26, 544–558. doi:10.1007/s11356-018-3563-0
Ballarin, L., Cima, F., and Sabbadin, A. (1994). Phagocytosis in the Colonial Ascidian Botryllus Schlosseri. Develop. Comp. Immunol. 18, 467–481. doi:10.1016/S0145-305X(06)80002-9
Binelli, A., Cogni, D., Parolini, M., Riva, C., and Provini, A. (2009a). Cytotoxic and Genotoxic Effects of In Vitro Exposure to Triclosan and Trimethoprim on Zebra Mussel (Dreissena polymorpha) Hemocytes. Comp. Biochem. Physiol. C: Toxicol. Pharmacol. 150, 50–56. doi:10.1016/j.cbpc.2009.02.005
Binelli, A., Parolini, M., Cogni, D., Pedriali, A., and Provini, A. (2009b). A Multi-Biomarker Assessment of the Impact of the Antibacterial Trimethoprim on the Non-target Organism Zebra Mussel (Dreissena polymorpha). Comp. Biochem. Physiol. Part C: Toxicol. Pharmacol. 150, 329–336. doi:10.1016/j.cbpc.2009.05.011
Bolognesi, C., and Fenech, M. (2012). Mussel Micronucleus Cytome Assay. Nat. Protoc. 7, 1125–1137. doi:10.1038/nprot.2012.043
Borecka, M., Białk-Bielińska, A., Siedlewicz, G., Kornowska, K., Kumirska, J., Stepnowski, P., et al. (2013). A New Approach for the Estimation of Expanded Uncertainty of Results of an Analytical Method Developed for Determining Antibiotics in Seawater Using Solid-phase Extraction Disks and Liquid Chromatography Coupled with Tandem Mass Spectrometry Technique. J. Chromatogr. A 1304, 138–146. doi:10.1016/j.chroma.2013.07.018
Bottoni, P., and Caroli, S. (2018). Presence of Residues and Metabolites of Pharmaceuticals in Environmental Compartments, Food Commodities and Workplaces: A Review Spanning the Three-Year Period 2014-2016. Microchemical J. 136, 2–24. doi:10.1016/j.microc.2017.06.016
Cajaraville, M. P., Olabarrieta, I., and Marigomez, I. (1996). In VitroActivities in Mussel Hemocytes as Biomarkers of Environmental Quality: A Case Study in the Abra Estuary (Biscay Bay). Ecotoxicol. Environ. Saf. 35, 253–260. doi:10.1006/eesa.1996.0108
Cañada-Cañada, F., Arancibia, J. A., Escandar, G. M., Ibañez, G. A., Espinosa Mansilla, A., Muñoz de la Peña, A., et al. (2009). Second-order Multivariate Calibration Procedures Applied to High-Performance Liquid Chromatography Coupled to Fast-Scanning Fluorescence Detection for the Determination of Fluoroquinolones. J. Chromatogr. A 1216, 4868–4876. doi:10.1016/j.chroma.2009.04.033
Carabineiro, S. A. C., Thavorn-Amornsri, T., Pereira, M. F. R., Serp, P., and Figueiredo, J. L. (2012). Comparison between Activated Carbon, Carbon Xerogel and Carbon Nanotubes for the Adsorption of the Antibiotic Ciprofloxacin. Catal. Today 186, 29–34. doi:10.1016/j.cattod.2011.08.020
Carvalho, I. T., and Santos, L. (2016). Antibiotics in the Aquatic Environments: A Review of the European Scenario. Environ. Int. 94, 736–757. doi:10.1016/j.envint.2016.06.025
Čelić, M., Gros, M., Farré, M., Barceló, D., and Petrović, M. (2019). Pharmaceuticals as Chemical Markers of Wastewater Contamination in the Vulnerable Area of the Ebro Delta (Spain). Sci. Total Environ. 652, 952–963. doi:10.1016/j.scitotenv.2018.10.290
Cima, F., Matozzo, V., Marin, M. G., and Ballarin, L. (2000). Haemocytes of the Clam Tapes philippinarum (Adams & Reeve, 1850): Morphofunctional Characterisation. Fish Shellfish Immunol. 10, 677–693. doi:10.1006/fsim.2000.0282
Collignon, P., and Voss, A. (2015). China, what Antibiotics and what Volumes Are Used in Food Production Animals? Antimicrob. Resist. Infect. Control. 4, 16. doi:10.1186/s13756-015-0056-5
Crupkin, A. C., Fulvi, A. B., Iturburu, F. G., Medici, S., Mendieta, J., Panzeri, A. M., et al. (2021). Evaluation of Hematological Parameters, Oxidative Stress and DNA Damage in the Cichlid Australoheros Facetus Exposed to the Fungicide Azoxystrobin. Ecotoxicol. Environ. Saf. 207, 111286–286. doi:10.1016/j.ecoenv.2020.111286
Dai, G., Wang, B., Huang, J., Dong, R., Deng, S., and Yu, G. (2015). Occurrence and Source Apportionment of Pharmaceuticals and Personal Care Products in the Beiyun River of Beijing, China. Chemosphere 119, 1033–1039. doi:10.1016/j.chemosphere.2014.08.056
De Franco, M. A. E., De Carvalho, C. B., Bonetto, M. M., Soares, R. d. P., and Féris, L. A. (2017). Removal of Amoxicillin from Water by Adsorption onto Activated Carbon in Batch Process and Fixed Bed Column: Kinetics, Isotherms, Experimental Design and Breakthrough Curves Modelling. J. Clean. Prod. 161, 947–956. doi:10.1016/j.jclepro.2017.05.197
De la Casa-Resino, I., Empl, M. T., Villa, S., Kolar, B., Fabrega, J., Lillicrap, A. D., et al. (2021). Environmental Risk Assessment of Veterinary Medicinal Products Intended for Use in Aquaculture in Europe: the Need for Developing a Harmonised Approach. Environ. Sci. Eur. 33, 84. doi:10.1186/s12302-021-00509-8
Donaghy, L., Lambert, C., Choi, K.-S., and Soudant, P. (2009). Hemocytes of the Carpet Shell Clam (Ruditapes Decussatus) and the Manila Clam (Ruditapes Philippinarum): Current Knowledge and Future Prospects. Aquaculture 297, 10–24. doi:10.1016/j.aquaculture.2009.09.003
Elizalde-Velázquez, A., Martínez-Rodríguez, H., Galar-Martínez, M., Dublán-García, O., Islas-Flores, H., Rodríguez-Flores, J., et al. (2017). Effect of Amoxicillin Exposure on Brain, Gill, Liver, and Kidney of Common Carp (Cyprinus carpio ): The Role of Amoxicilloic Acid. Environ. Toxicol. 32, 1102–1120. doi:10.1002/tox.22307
Fabbri, E. (2015). Pharmaceuticals in the Environment: Expected and Unexpected Effects on Aquatic Fauna. Ann. N.Y. Acad. Sci. 1340, 20–28. doi:10.1111/nyas.12605
Fabrello, J., Targhetta, F., Ciscato, M., Asnicar, D., Bernardini, I., Milan, M., et al. (2021). First Evidence of In Vitro Effects of C6O4-A Substitute of PFOA-On Haemocytes of the Clam Ruditapes Philippinarum. Toxics 9 (8), 191. doi:10.3390/toxics9080191
Feitosa-Felizzola, J., and Chiron, S. (2009). Occurrence and Distribution of Selected Antibiotics in a Small Mediterranean Stream (Arc River, Southern France). J. Hydrol. 364, 50–57. doi:10.1016/j.jhydrol.2008.10.006
Fent, K., Weston, A., and Caminada, D. (2006). Ecotoxicology of Human Pharmaceuticals. Aquat. Toxicol. 76, 122–159. doi:10.1016/j.aquatox.2005.09.009
Ferech, M., Coenen, S., Malhotra-Kumar, S., Dvorakova, K., Hendrickx, E., Suetens, C., et al. (2006). European Surveillance of Antimicrobial Consumption (ESAC): Outpatient Quinolone Use in Europe. J. Antimicrob. Chemother. 58, 423–427. doi:10.1093/jac/dkl183
Gagné, F., Blaise, C., Fournier, M., and Hansen, P. D. (2006). Effects of Selected Pharmaceutical Products on Phagocytic Activity in Elliptio Complanata Mussels. Comp. Biochem. Physiol. Part C: Toxicol. Pharmacol. 143, 179–186. doi:10.1016/j.cbpc.2006.01.008
González-González, E. D., Gómez-Oliván, L. M., Islas-Flores, H., and Galar-Martínez, M. (2021). Developmental Effects of Amoxicillin at Environmentally Relevant Concentration Using Zebrafish Embryotoxicity Test (ZET). Water Air Soil Pollut. 232, 196. doi:10.1007/s11270-021-05148-6
Graham, R. C., and Karnovsky, M. J. (1966). Thf Early Stages of Absorption of Injected Horseradish Peroxidase in the Proximal Tubules of Mouse Kidney: Ultrastructural Cytochemistry by a New Technique. J. Histochem. Cytochem. 14, 291–302. doi:10.1177/14.4.291
Grobin, A., Roškar, R., and Trontelj, J. (2022). Multi-parameter Risk Assessment of Forty-One Selected Substances with Endocrine Disruptive Properties in Surface Waters Worldwide. Chemosphere 287, 132195. doi:10.1016/j.chemosphere.2021.132195
Gust, M., Gélinas, M., Fortier, M., Fournier, M., and Gagné, F. (2012). In Vitro immunotoxicity of Environmentally Representative Antibiotics to the Freshwater Mussel Elliptio Complanata. Environ. Pollut. 169, 50–58. doi:10.1016/j.envpol.2012.05.020
Hayashi, M., Nakajima, Y., and Fishman, W. H. (1964). The Cytologic Demonstration of Β-Glucuronidase Employing Naphthol as-bi Glucuronide and Hexazonium Pararosanilin; A Preliminary Report. J. Histochem. Cytochem. 12, 293–297. doi:10.1177/12.4.293
Ju, H., Li, S., Xu, Y., Zhang, G., and Zhang, J. (2019). Intensive Livestock Production Causing Antibiotic Pollution in the Yinma River of Northeast China. Water. J. 11, 2006. doi:10.3390/w11102006
Kirsch-volders, M., Sofuni, T., Aardema, M., Albertini, S., Eastmond, D., Fenech, M., et al. (2000). Report from the In Vitro Micronucleus Assay Working Group. Environ. Mol. Mutagen. 35, 167–172. doi:10.1002/(sici)1098-2280(2000)35:3<167:aid-em3>3.0.co;2-g
Klein, E. Y., Van Boeckel, T. P., Martinez, E. M., Pant, S., Gandra, S., Levin, S. A., et al. (2018). Global Increase and Geographic Convergence in Antibiotic Consumption between 2000 and 2015. Proc. Natl. Acad. Sci. USA 115, E3463–E3470. doi:10.1073/pnas.1717295115
Kostich, M. S., Batt, A. L., and Lazorchak, J. M. (2014). Concentrations of Prioritized Pharmaceuticals in Effluents from 50 Large Wastewater Treatment Plants in the US and Implications for Risk Estimation. Environ. Pollut. 184, 354–359. doi:10.1016/j.envpol.2013.09.013
Lacaze, E., Pédelucq, J., Fortier, M., Brousseau, P., Auffret, M., Budzinski, H., et al. (2015). Genotoxic and Immunotoxic Potential Effects of Selected Psychotropic Drugs and Antibiotics on Blue Mussel (Mytilus edulis) Hemocytes. Environ. Pollut. 202, 177–186. doi:10.1016/j.envpol.2015.03.025
Leung, H. W., Minh, T. B., Murphy, M. B., Lam, J. C. W., So, M. K., Martin, M., et al. (2012). Distribution, Fate and Risk Assessment of Antibiotics in Sewage Treatment Plants in Hong Kong, South China. Environ. Int. 42, 1–9. doi:10.1016/j.envint.2011.03.004
Liu, X., Zhang, L., You, L., Yu, J., Zhao, J., Li, L., et al. (2011). Differential Toxicological Effects Induced by Mercury in Gills from Three Pedigrees of Manila Clam Ruditapes Philippinarum by NMR-Based Metabolomics. Ecotoxicology 20, 177–186. doi:10.1007/s10646-010-0569-x
López-Roldán, R., De Alda, M. L., Gros, M., Petrovic, M., Martín-Alonso, J., and Barceló, D. (2010). Advanced Monitoring of Pharmaceuticals and Estrogens in the Llobregat River basin (Spain) by Liquid Chromatography-Triple Quadrupole-Tandem Mass Spectrometry in Combination with Ultra Performance Liquid Chromatography-Time of Flight-Mass Spectrometry. Chemosphere 80, 1337–1344. doi:10.1016/j.chemosphere.2010.06.042
Lowe, D. M. (1995). Lysosomal Membrane Impairment in Blood Cells of Perna Viridis: An In Vitro Marker of Contaminant Induced Damage. Res. Bull. 60, 79–82.
Lu, J., Wu, J., Zhang, C., Zhang, Y., Lin, Y., and Luo, Y. (2018). Occurrence, Distribution, and Ecological-Health Risks of Selected Antibiotics in Coastal Waters along the Coastline of China. Sci. Total Environ. 644, 1469–1476. doi:10.1016/j.scitotenv.2018.07.096
Matozzo, V., Ballarin, L., and Marin, M. G. (2002). In Vitro effects of Tributyltin on Functional Responses of Haemocytes in the clamTapes Philippinarum. Appl. Organometal. Chem. 16, 169–174. doi:10.1002/aoc.278
Matozzo, V., Bertin, V., Battistara, M., Guidolin, A., Masiero, L., Marisa, I., et al. (2016). Does the Antibiotic Amoxicillin Affect Haemocyte Parameters in Non-target Aquatic Invertebrates? the Clam Ruditapes Philippinarum and the Mussel Mytilus galloprovincialis as Model Organisms. Mar. Environ. Res. 119, 51–58. doi:10.1016/j.marenvres.2016.05.017
Matozzo, V., De Notaris, C., Finos, L., Filippini, R., and Piovan, A. (2015). Environmentally Realistic Concentrations of the Antibiotic Trimethoprim Affect Haemocyte Parameters but Not Antioxidant Enzyme Activities in the Clam Ruditapes Philippinarum. Environ. Pollut. 206, 567–574. doi:10.1016/j.envpol.2015.08.021
Matozzo, V. (2014). Effects of Pharmaceuticals on Immune Parameters of Aquatic Invertebrates. Invertebr. Surviv. 11, 163–173.
Matozzo, V., Fabrello, J., Masiero, L., Ferraccioli, F., Finos, L., Pastore, P., et al. (2018). Ecotoxicological Risk Assessment for the Herbicide Glyphosate to Non-target Aquatic Species: a Case Study with the Mussel Mytilus galloprovincialis. Environ. Pollut. 233, 623–632. doi:10.1016/j.envpol.2017.10.100
Matozzo, V., and Marin, M. G. (2010). The Role of Haemocytes from the Crab Carcinus Aestuarii (Crustacea, Decapoda) in Immune Responses: a First Survey. Fish Shellfish Immunol. 28 (4), 534–541. doi:10.1016/j.fsi.2009.12.003
Matozzo, V., Zampieri, C., Munari, M., and Marin, M. G. (2019). Glyphosate Affects Haemocyte Parameters in the Clam Ruditapes Philippinarum. Mar. Environ. Res. 146, 66–70. doi:10.1016/j.marenvres.2019.03.008
McEneff, G., Barron, L., Kelleher, B., Paull, B., and Quinn, B. (2014). A Year-Long Study of the Spatial Occurrence and Relative Distribution of Pharmaceutical Residues in Sewage Effluent, Receiving marine Waters and marine Bivalves. Sci. Total Environ. 476-477, 317–326. doi:10.1016/j.scitotenv.2013.12.123
McEwen, S. A., and Fedorka‐Cray, P. J. (2002). Antimicrobial Use and Resistance in Animals. Clin. Infect. Dis. 34, S93–S106. doi:10.1086/340246
Minh, T. B., Leung, H. W., Loi, I. H., Chan, W. H., So, M. K., Mao, J. Q., et al. (2009). Antibiotics in the Hong Kong Metropolitan Area: Ubiquitous Distribution and Fate in Victoria Harbour. Mar. Pollut. Bull. 58, 1052–1062. doi:10.1016/j.marpolbul.2009.02.004
Musrri, C. A., Palma-Rojas, C., Von Brand, E., and Abessa, D. M. S. (2021). Environmental Genotoxicity Assessment Using Micronucleus (And Nuclear Abnormalities) Test on Intertidal Mussel Perumytilus Purpuratus: A Tool for Biomonitoring the Chilean Coast. Bull. Environ. Contam. Toxicol. 107, 77–83. doi:10.1007/s00128-021-03132-8
Nunes, B., Leal, C., Rodrigues, S., and Antunes, S. C. (2018). Assessment of Ecotoxicological Effects of Ciprofloxacin in Daphnia magna: Life-History Traits, Biochemical and Genotoxic Effects. Water Sci. Technol. 2017, 835–844. doi:10.2166/wst.2018.255
Orozco-Hernández, J. M., Gómez Oliván, L. M., Heredia-García, G., Luja-Mondragón, M., Islas-Flores, H., SanJuan-Reyes, N., et al. (2019). Genotoxic and Cytotoxic Alterations Induced by Environmentally-Relevant Concentrations of Amoxicillin in Blood Cells of Cyprinus carpio. Chemosphere 236, 124323. doi:10.1016/j.chemosphere.2019.07.054
Papich, M. G. (2017). Ciprofloxacin Pharmacokinetics in Clinical Canine Patients. J. Vet. Intern. Med. 31, 1508–1513. doi:10.1111/jvim.14788
Parolini, M., Pedriali, A., and Binelli, A. (2013). Application of a Biomarker Response Index for Ranking the Toxicity of Five Pharmaceutical and Personal Care Products (PPCPs) to the Bivalve Dreissena polymorpha. Arch. Environ. Contam. Toxicol. 64, 439–447. doi:10.1007/s00244-012-9847-3
Pavlica, M., Klobučar, G. I. V., Vetma, N., Erben, R., and Papeš, D. (2000). Detection of Micronuclei in Haemocytes of Zebra Mussel and Great Ramshorn Snail Exposed to Pentachlorophenol. Mutat. Res. 465, 145–150. doi:10.1016/S1383-5718(99)00222-3
Pulicharla, R., Hegde, K., Brar, S. K., and Surampalli, R. Y. (2017). Tetracyclines Metal Complexation: Significance and Fate of Mutual Existence in the Environment. Environ. Pollut. 221, 1–14. doi:10.1016/j.envpol.2016.12.017
Ramesh, M., Sujitha, M., Anila, P. A., Ren, Z., and Poopal, R. K. (2021). Responses of Cirrhinus Mrigala to Second‐generation Fluoroquinolone (Ciprofloxacin) Toxicity: Assessment of Antioxidants, Tissue Morphology, and Inorganic Ions. Environ. Toxicol. 36, 887–902. doi:10.1002/tox.23091
Reis-Santos, P., Pais, M., Duarte, B., Caçador, I., Freitas, A., Vila Pouca, A. S., et al. (2018). Screening of Human and Veterinary Pharmaceuticals in Estuarine Waters: A Baseline Assessment for the Tejo Estuary. Mar. Pollut. Bull. 135, 1079–1084. doi:10.1016/j.marpolbul.2018.08.036
Rosas-Ramírez, J. R., Orozco-Hernández, J. M., Elizalde-Velázquez, G. A., Raldúa, D., Islas-Flores, H., and Gómez-Oliván, L. M. (2022). Teratogenic Effects Induced by Paracetamol, Ciprofloxacin, and Their Mixture on Danio rerio Embryos: Oxidative Stress Implications. Sci. Total Environ. 806, 150541. doi:10.1016/j.scitotenv.2021.150541
Sabuncu, E., David, J., Bernède-Bauduin, C., Pépin, S., Leroy, M., Boëlle, P.-Y., et al. (2009). Significant Reduction of Antibiotic Use in the Community after a Nationwide Campaign in France, 2002-2007. Plos Med. 6, e1000084. doi:10.1371/journal.pmed.1000084
Sarmah, A. K., Meyer, M. T., and Boxall, A. B. A. (2006). A Global Perspective on the Use, Sales, Exposure Pathways, Occurrence, Fate and Effects of Veterinary Antibiotics (VAS) in the Environment. Chemosphere 65 (5), 725–759. doi:10.1016/j.chemosphere.2006.03.026
Schumock, G. T., Li, E. C., Suda, K. J., Matusiak, L. M., Vermeulen, L. C., Hoffman, J. M., et al. (2014). National Trends in Prescription Drug Expenditures and Projections for 2014. Am. J. Health Syst. Pharm. 71, 482–499. doi:10.2146/ajhp130767
Song, Y.-L., and Hsieh, Y.-T. (1994). Immunostimulation of Tiger Shrimp (Penaeus monodon) Hemocytes for Generation of Microbicidal Substances: Analysis of Reactive Oxygen Species. Develop. Comp. Immunol. 18, 201–209. doi:10.1016/0145-305X(94)90012-4
Srain, H. S., Beazley, K. F., and Walker, T. R. (2021). Pharmaceuticals and Personal Care Products and Their Sublethal and Lethal Effects in Aquatic Organisms. Environ. Rev. 29, 142–181. doi:10.1139/er-2020-0054
Sweetman, S. C. (2005). Martindale: The Complete Drug Reference. 34th ed. London: Pharmaceutical Press, 161, 241, 245, 257.
Trombini, C., Kazakova, J., Montilla-López, A., Fernández-Cisnal, R., Hampel, M., Fernández-Torres, R., et al. (2021). Assessment of Pharmaceutical Mixture (Ibuprofen, Ciprofloxacin and Flumequine) Effects to the Crayfish Procambarus clarkii: A Multilevel Analysis (Biochemical, Transcriptional and Proteomic Approaches). Environ. Res. 200, 111396. doi:10.1016/j.envres.2021.111396
Van Boeckel, T. P., Brower, C., Gilbert, M., Grenfell, B. T., Levin, S. A., Robinson, T. P., et al. (2015). Global Trends in Antimicrobial Use in Food Animals. Proc. Natl. Acad. Sci. USA 112, 5649–5654. doi:10.1073/pnas.1503141112
Wachstein, M., and Meisel, E. (1957). A Comparativr Study of Enzymatic Staining Reactions in the Rat Kidney with Necrobiosis Induced by Ischemia and Nephrotoxic Agents (Mercuhydrin and Dl-Serine). J. Histochem. Cytochem. 5, 204–220. doi:10.1177/5.3.204
Wagil, M., Kumirska, J., Stolte, S., Puckowski, A., Maszkowska, J., Stepnowski, P., et al. (2014). Development of Sensitive and Reliable LC-MS/MS Methods for the Determination of Three Fluoroquinolones in Water and Fish Tissue Samples and Preliminary Environmental Risk Assessment of Their Presence in two rivers in Northern Poland. Sci. Total Environ. 493, 1006–1013. doi:10.1016/j.scitotenv.2014.06.082
Yang, Y., Ok, Y. S., Kim, K.-H., Kwon, E. E., and Tsang, Y. F. (2017). Occurrences and Removal of Pharmaceuticals and Personal Care Products (PPCPs) in Drinking Water and Water/sewage Treatment Plants: A Review. Sci. Total Environ. 596-597, 303–320. doi:10.1016/j.scitotenv.2017.04.102
Zhang, M., Shen, J., Zhong, Y., Ding, T., Dissanayake, P. D., Yang, Y., et al. (2020). Sorption of Pharmaceuticals and Personal Care Products (PPCPs) from Water and Wastewater by Carbonaceous Materials: A Review. Crit. Rev. Environ. Sci. Technol. 52, 727–766. doi:10.1080/10643389.2020.1835436
Zhang, M., Shi, Y., Lu, Y., Johnson, A. C., Sarvajayakesavalu, S., Liu, Z., et al. (2017). The Relative Risk and its Distribution of Endocrine Disrupting Chemicals, Pharmaceuticals and Personal Care Products to Freshwater Organisms in the Bohai Rim, China. Sci. Total Environ. 590-591, 633–642. doi:10.1016/j.scitotenv.2017.03.011
Zhang, Q.-Q., Ying, G.-G., Pan, C.-G., Liu, Y.-S., and Zhao, J.-L. (2015). Comprehensive Evaluation of Antibiotics Emission and Fate in the River Basins of China: Source Analysis, Multimedia Modeling, and Linkage to Bacterial Resistance. Environ. Sci. Technol. 49, 6772–6782. doi:10.1021/acs.est.5b00729
Zhang, R., Zhang, G., Zheng, Q., Tang, J., Chen, Y., Xu, W., et al. (2012). Occurrence and Risks of Antibiotics in the Laizhou Bay, China: Impacts of River Discharge. Ecotoxicology Environ. Saf. 80, 208–215. doi:10.1016/j.ecoenv.2012.03.002
Keywords: antibiotics, pharmaceuticals, in vitro, haemocytes, bivalves
Citation: Nicolussi G, Fabrello J, Asnicar D, Ciscato M, Matozzo V and Marin MG (2022) Effects of Three Widely Used Antibiotics and Their Mixture on the Haemocytes of the Clam Ruditapes philippinarum. Front. Environ. Sci. 10:845213. doi: 10.3389/fenvs.2022.845213
Received: 29 December 2021; Accepted: 20 January 2022;
Published: 10 February 2022.
Edited by:
Hai Ocean Xu, Jiangsu University, ChinaReviewed by:
Haitham Abo-Al-Ela, Suez University, EgyptKwang-Sik Choi, Jeju National University, South Korea
Copyright © 2022 Nicolussi, Fabrello, Asnicar, Ciscato, Matozzo and Marin. This is an open-access article distributed under the terms of the Creative Commons Attribution License (CC BY). The use, distribution or reproduction in other forums is permitted, provided the original author(s) and the copyright owner(s) are credited and that the original publication in this journal is cited, in accordance with accepted academic practice. No use, distribution or reproduction is permitted which does not comply with these terms.
*Correspondence: Maria Gabriella Marin, mgmar@bio.unipd.it