Corrigendum: Suitability and eligibility of Phyllostachys pubescens (Moso Bamboo) afforestation for GHG (greenhouse gases) projects: case study in central Italy
- 1Dipartimento di Scienze Biomediche, Odontoiatriche e delle Immagini Morfologiche e Funzionali (Biomorf), Università di Messina, Polo Universitario Annunziata, Messina, Italy
- 2Science4Life s.r.l., University of Messina, Messina, Italy
A case study concerning an experimental moso bamboo plantation (5 ha), located in central Italy, is presented. In order to evaluate CO2 capturing potential, a computational study was developed and applied to the bamboo field to predict and quantify the tons of CO2 equivalent annually sequestered, during a project range of 15 years. The computational model, based on allometric parameters and field density, was integrated with an accurate analysis of the geological, pedological and climatic scenario, and of the socio-economic context. The study aimed to correlate the growth and thickening trend of moso bamboo field, namely its CO2 capturing potential, with the geopedoclimatic context. This allowed to evaluate if the bamboo afforestation projects are capable to offset carbon dioxide emissions in the Italian context. The highest segregation power of the bamboo plantation equated 3,651.32 tCO2e ⋅ yr−1, segregated by the whole field in the 12th year (out of a total timeframe of 15 years). The study demonstrated the eligibility requirements for a GHG project and the suitability of moso bamboo farming finalized to carbon trading. Our findings represent a valid model to encourage the voluntary carbon market in Italy from afforestation with bamboo species.
Introduction
Climate change and its strong connection with anthropic greenhouse effect and global warming is considered one of the most significant global emergencies and it is no longer a topic of exclusive scientific interest. There is a growing commitment of governments and international policies in search of containment solutions to limit the serious consequences of global warming, such as the rising ocean water temperatures, the increasing sea levels and the melting of glaciers (IPCC, 2019). The evidence of a strong correlation between carbon dioxide emissions and the increasing global temperature makes urgent to stabilize the levels of CO2, and in general of atmospheric greenhouse gases (GHG), through a series of contrasting strategies. The atmospheric CO2 concentration has exceeded 30% increase in the past 60 years (Le Quéré et al., 2018). In Italy, more than 335 million tonnes of CO2 emissions have been reported only for 2019 (Global Carbon Project, 2021). The challenge of climate change requires simultaneous action of mitigation, adaptation and development (MAD), where the afforestation projects may supply a crucial contribution , thanks to the multiple service that forests are able to provide, such as carbon sequestration, timber provision and income generation (UNFCCC, 2007). Reducing Emissions from Deforestation and forest Degradation, along with the sustainable management of forests (REDD+), is an international framework to encourage voluntary efforts for GHG emissions reduction (Streck 2020). Carbon offsetting involves the acquisition of carbon credits from certified GHG projects to compensate the equivalent of one ton of carbon dioxide emitted in a specific area. The Kyoto Protocol introduced the Clean Development Mechanism (CDM) which consists of the purchase by industrialized countries (Annex-I) of carbon credits sold by developing countries (non-Annex-1), and generated from GHG projects based on afforestation and reforestation (Lefebvre et al., 2021). Among the different types of carbon credits envisaged by the voluntary market, there are VERs (Verified Emission Reduction) deriving from non-CDM-projects (UNFCCC, 2014; Lang et al., 2019). One VER corresponds to one metric ton of CO2 equivalent emissions (mtCO2e). Overall, voluntary credits representing 104 mtCO2e were traded in 2019 amounting to a cumulative market value of 320 million USD (Kreibich and Hermwille, 2021). In this context, a significant role can be played by bamboo forests; indeed, although strictly botanically bamboo is considered as a woody grass, and not a tree, the role of bamboo forests in the carbon cycle is broadly comparable with that of other types of forests. For this reason the CDM Executive Board, in its 39th meeting, decided bamboos to be equivalent to trees in the context of afforestation and reforestation (UNFCCC, 2008). The common term of bamboo includes the members of a taxonomic group of woody grasses (subfamily Bambusoideae, family Andropogoneae/Poaceae) that is composed of 1,250 species and 75 genera, most of which are relatively fast-growing, attaining stand maturity within 5 years (Zhang et al., 2015). Approximately 80% of the species are widely distributed in the Asian continent, but they also grow in Africa, and Central and South America (Canavan et al., 2016). Phyllostachys pubescens, also known as moso bamboo, is the world’s widespread bamboo species: from the underground rhizome, a seedling grows quickly to a culm, reaching a height of 25 m or more, and a diameter of 10–13 cm. The bamboo growth rate depends on numerous factors, including the environmental temperature, water availability and moisture. In fact, nutrients adsorption in Moso bamboo is strictly dependent on the integrity of roots, generally amassed in the superficial layers of the soil with the form of a leptomorphic and creeping root system (Kleinhenz and Midmore, 2001). According to several scientific studies, Moso bamboo has proven to be one of the most suitable plants for carbon sequestration from the atmosphere mainly due to its fast growth and easy management of plantations (King et al., 2021). Moso bamboo forests have an estimated above ground carbon density (i.e., the mass of carbon per unit land area) ranging from 34 to 70 Mg·ha−1, with an average rate of 8 Mg·ha−1 per year (Dwivedi et al., 2019). For this reason, moso afforestation may be included in eco-restoration strategies for rejuvenation of degraded ecosystems, bringing them back to their near original state (Singh et al., 2020). It also helps to control soil erosion, sustain microbial ecology, enhance biomass production and socio-economic development (Singh et al., 2021). However, new procedures for the computation of carbon credits from bamboo cultivations are needed, given the general conflict that emerges from literature data. These conflicting results can be explained by the difficulty of harmonizing several variables, including biomes, ecosystems, management regimes, timeframes, carbon pools, etc., At present, many computational and predictive models are available for establishing the dimensions of carbon stocks in forests (Zhang et al., 2015). Quite often, though, such models are difficult to be applied, and are tested exclusively in Asia. The present paper describes the application of a moso plantation management model to an unexplored context (Italy) with the aim of evaluating its potential suitability and eligibility in a REDD project.
The major objective of this study is to assess if small-scale bamboo afforestation interventions can contribute to: 1) the compensation of CO2 emissions through the carbon trading system; 2) the hydrogeological preservation of the Italian territory; 3) the development of the agro-economy through the creation of employment; 4) raise the sustainability score that at present is medium to low in central Italy, where this study took place (D’Adamo et al., 2021). For the evaluation of capturing potential of the experimental field a simple and replicable predictive computational model was used, based on field data integrated with information on the geological and climate scenarios. The resulting perspective is to transfer the methodology used in this study to other domestic and European contexts, contributing to the spread of bamboo afforestation as a key strategy to fight climate change, for the development of bioeconomy and for the eco-restoration of degraded lands.
The Italian Context
In Italy, the voluntary markets for ecosystem services generated by forests have not reached yet a stage of maturity. Nonetheless, the voluntary market shows great potential to stimulate virtuous actions in the forestry and agricultural sectors, also representing a precious opportunity for private companies, non-profit organizations, public bodies, and individuals. Moreover, it could ensure a concrete contribution to the pursuit of the international objectives signed by the Italian government. There is still no institutional recognition of guidelines nor a national register of credits generated on the national territory. The European Regulation 841/2018 (Regulation, 2018) (Reg UE 2018/841) provides that the credits generated by the LULUCF (Land Use, Land Use Change and Forestry) can contribute to the achievement of 30% of the emission reductions compared to 2005 in the sectors governed by the Effort Sharing regulation (ESR), as an objective that contributes to the wider 40% reduction target compared to 1990. Carbon credits generated can only be partially used in order to achieve the 30% ESR target, or up to a limit, established at European level, of 280 mtCO2e for the decade 2020–2030 (intersector flexibility). For Italy this limit equates 11.5 mtCO2e, corresponding to 0.3% of the ESR target. Between 2016–2017, a number of nine active projects were identified in the Italian forestry carbon voluntary market, which involved a total area of30,453 ha, with generation and exchange of 158,436 tCO2e. The average price of carbon credit assumed very different values in the 2 years of the survey, going from 17.7 €/tCO2e in 2016 to 36 €/tCO2e in 2017 (Brotto et al., 2018). These values are much higher than the average value recorded internationally (5.2 $/tCO2e). Volumes and prices have fluctuated widely over the years, suggesting that the forest-based carbon market in Italy experiences considerable variability and price volatility. All the project activities above described concern woods and forests, whereas bamboo carbon farming is still a little explored practice in Italy. The rapid growth and thickening that characterizes the moso species and its wide and varied geographical distribution indicate that the moso management aimed at carbon offset must certainly be a way forward for Italy in the coming years. Certified GHG projects could become true promoters of the transition to a low-carbon economy. Likewise, it is necessary to take into account additional benefits of such projects, deriving from the special features of bamboo itself, first of all its root system. In fact, the root apparatus is not only a fundamental carbon sink, but due to its morpho-structural characteristics, it represents a real bulwark in the prevention of hydrogeological instability; the latter has acquired, in recent years, the dimension of a real emergency in Italy, that in the last 20 years has been the scene of dramatic events such as landslides and floods (Paleari, 2018). Indeed bamboo forests preserve the soil from hydro-erosion and from a wider variety of events, i.e. slope instability, landslides and mudslides (Santoro et al., 2020). Furthermore, bamboo’s farming oriented to carbon offset provides numerous environmental services, it can help reducing rural poverty by increasing the levels of rural and extra-rural employment and empowering women in their communities (King et al., 2021).
Case Study: The CO2 Sequestration Capacity of a Moso Bamboo Field
The present case study concerns an experimental moso bamboo field (5 ha) located in central Italy whose management was oriented to carbon offset. A computational model based on allometric parameters and field density, was integrated with an accurate analysis of the geological, pedological and climatic scenario, and of the economic and social context. The model has been processed and applied to the bamboo field in order to evaluate CO2 capturing potential, and to predict and quantify the tons of CO2 equivalent annually sequestered, during a project range of 15 years. This study aimed to evaluate if a small-scale afforestation project with bamboo can be suitable to reduce GHG emissions and eligible for carbon farming, also in Italy where it is still a niche practice. The aims of the study were: 1) characterization of the geopedoclimatic and socio-economic scenario where the bamboo plantation is located; 2) evaluation of the CO2 capturing potential of the species P. pubescens in the specific scenario aimed at VERs production; 3) prediction and quantification through the computational model of the CO2 equivalent tons sequestered per year, and consequently of the carbon credits that the experimental field can generate; 4) evaluation of suitability and eligibility of moso bamboo afforestation finalized to GHG (Greenhouse gases) projects in the Italian context. The computational model used for the calculation of carbon biomass captured by the bamboo plantation was formulated after a deep analysis of scientific literature and documentation released by officially and internationally recognized institutions; among these, the International Network for Bamboo and Rattan (INBAR) that is an independent and intergovernmental organization which promotes innovative solutions to support environmental sustainability. In particular, INBAR’s main lines of intervention fall within the so called REDD + projects by means of afforestation. INBAR’s methodology is internationally shared and defines requisites and procedures for accurate estimation of sequestered CO2, taking into account the dual carbon sink (plant/soil). Moso bamboo, a unique tree botanically speaking, shows an estimated segregating capacity of 7–9 t Ceq·ha−1·yr−1, that is even higher compared to a 25 years old pine tree forest (3 t Ceq ha−1 yr−1) (Nath et al., 2015). The INBAR protocol implements an afforestation project only if the principle of additionality, with respect to the baseline scenario, exists and can be verified.
Location of the Experimental Field
The bamboo plantation occupies 5 ha and it is located in Lazio district and precisely in a rural hamlet named Piè delle Piagge (LAT 41°37′00″N; LON 13°48′31″E; ALT 383 m a.s.l.) (Supplementary Figure S1), within the municipality of Atina, which is 38.08 Km distant from Frosinone, and 77.08 Km from Italian capital Rome. The municipality of Atina stretches across a hill that develops in the valley of Comino, at the western edge of a mountain complex, called Monti Bianchi, culminating in the locality Il Colle, the western offshoot of the historic center of Atina. The territory within the municipality of Atina is rather varied, and it laps both the National Park of Abruzzo, and the railway line Rome-Cassino-Caserta.
Economic and Social Context
The territory of Atina is classified as a socially disadvantaged area with an unemployment rate that is equal to 12.9% and an index of social and material vulnerability which is 100.2% according to the Italian National Statistic Institute (ISTAT, 2011). In this area, agriculture is the driving force of economy, the cultivation of wheat, corn and grapes are prevalent. The latter represent an excellent raw material because of the production of numerous high quality wines, which have been recognized as Controlled Designation of Origin (CDO). The cultivation of grapes is strongly favored by the climatic and pedological conditions of this area. Moreover, under development is the tourism, already supported by a good hospitality. The industrial sector is not particularly developed, but prominent activities are based on food, mineral, metallurgic, and building sectors. Regarding to the rural hamlet of Piè delle Piagge, the demographic profile consists of 52 inhabitants. The impact of SARS-CoV-2 pandemic on the Italian agri-food sector was really relevant, highlighting a national unprecedented economic crisis. The pandemic emergency has registered a number of dramatic consequences on the primary sector, by impacting on local agricultural productions and their food derivatives, including satellite activities (Barcaccia et al., 2020). Particularly penalized resulted the areas classified as Low intensity landscape type, category where the municipality of Atina is included. This type of classification was introduced by The Italian Ministry of Food, Agriculture and Forest Policies (ONPR, 2018), although used just in the context of the National Strategic Plan for Rural Development 2007–13. According to these classifications, the Italian rural landscape is divided into four distinct levels, based on intensity of agricultural activities, environmental features, and energy required in the primary crop production. All these factors are also relevant in the context of the Common Agricultural Policy and the Biodiversity Strategy towards 2030 (Agnoletti et al., 2020).
Geopedoclimatic Context
The geopedological context is characterized by the presence of an ancient sedimentary soil, with prevailing calcareous rocks typical of those geographic areas that are in proximity of the Appennini mountains. At high altitudes, it is possible to see calcareous outcrops, while in some zones terraces carved by deposition and erosion can be seen. This type of layering allowed the soil to save a strong fingerprint of the parent rock, without any climatic influence. In general, the land consists of calcareous soil, with abundant calcium carbonate; and sedimentary soil, made of sandstone and marl flood in the level part. Piè delle Piagge has been classified as an area with high seismic risk (level 1). Atina presents a warm and temperate climate, with considerable rainfall in winter, while low in summer (Climate Data-org, 2021). Köppen and Geiger (Köppen, 1936) classify Atina as a “Csa” climate type, where “Cs” means “temperate with dry summer”, and “a” means “temperature that exceeds 22°C in the hottest month”. Figure 1A shows the climatic plot referring to the Atina neighborhood in relation to year 2020: the line describes the annual trend of temperature which registers an average of 13.4°C; the histogram reports the data related to the monthly average rainfall, measured as millimeters of rain; the yearly rainfall registered equates 952 mm. Figure 1B shows a plot of the temperatures registered in 2020. It can be observed that August was the hottest month, with an average temperature of 22.2°C, whereas the coldest month resulted to be January, with an average temperature of 5.1°C. The climatic profile of the last 10 years (2009–2019) is characteristic of a temperate zone, with an abundant rainfall (1,234–1,606 mm y−1) and weak dryness in summer, especially in July/August. The average temperature is slightly high, ranging from 12.0 to 14.2°C: it is quite cold in winter, with an average temperature below 10° that lasts 4–5 months per year; and an average minimum temperature in the coldest month of the year ranging from 0.1 to 2.9°C. The entire province of Frosinone is characterized by a considerable hydrogeological instability; great part of the soils are threatened by serious events of floods and landslides which occur after extreme meteorological events, due to their physical and morphologic characteristics. Floods and mass movements of soil cause erosion, flow of sediments and loss of soil resources with major impacts on human activities and lives, damage to buildings and infrastructures, and depletion of agricultural land. Frosinone district holds the highest number of landslides within Lazio (Central Italy), being also considered a high-risk area (Gravina et al., 2017). Moreover, the Italian National Institute of Statistics (ISTAT) engaged a system of 175 indicators (economic, environmental and social) to quantify, for each Italian region, a sustainability score. This methodology is able to measure the performance in terms of sustainability by considering a multiple set of parameters. The sustainability score resulted to be middle to low for central Italy, with the region Lazio as the least performing (D’Adamo et al., 2021).
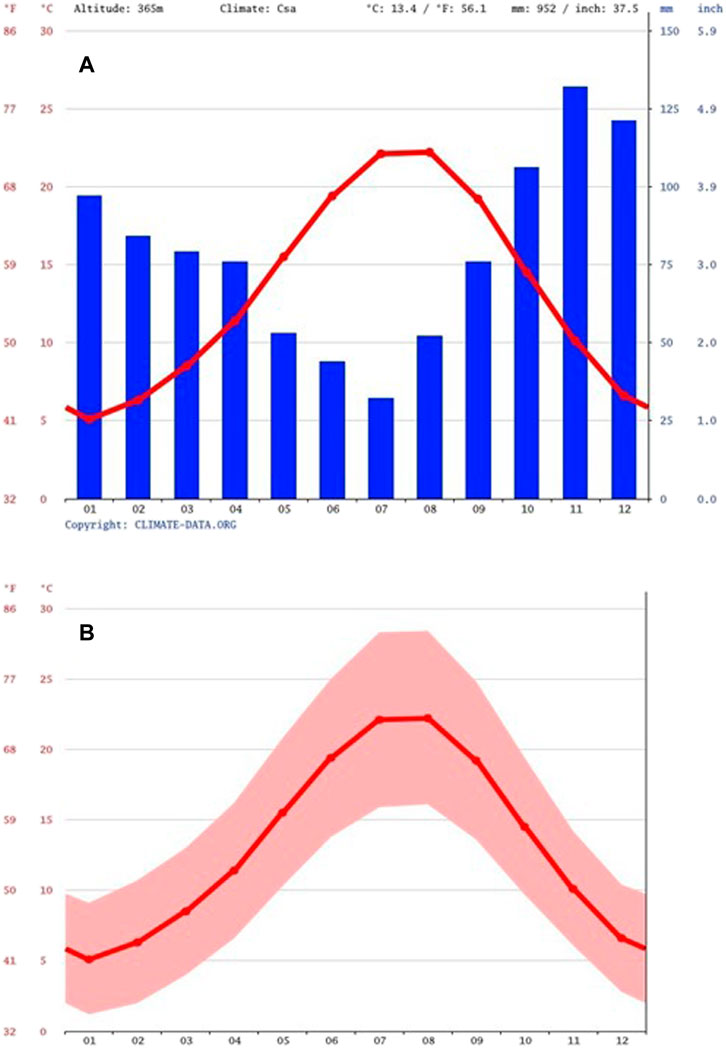
FIGURE 1. (A) Climate plot of Atina municipality related to year 2020; (B) Temperatures registered in Atina in year 2020.
Bamboo Experimental Field
In August 2015, 5,000 parent plants belonging to the species Phyllostachys pubescens (Pradelle) Mazel ex. Houz were planted in Piè delle Piagge. At the time of acquisition, the land destined to Moso cultivation was being classified as “irrigable crop land”. Parent plants were provided by “Vivai OnlyMoso” (Tavullia, Italy). In June 2016, a number of 2,600 damaged plants were displaced by new individuals. The planting scheme advised by the seller was D-840, corresponding to a density of 840 plants·ha−1. In November 2020, the good state of development of the field was evident: the plants were showing an exponential growth, usually allowed by fertile, deep and irrigated soils. In November 2020, the agronomic estimate revealed the presence of 6,500 plants·ha−1 , with an average culms height of 3.50 m. Annual productions of 7,500–12,000 Kg of sprouts·ha−1 , and of 2,500 – 6,000 culms·ha−1, respectively, were also predicted by the agronomist.
Development of Computation Model
In order to predict and quantify reliably the metric tons of carbon dioxide equivalent segregated by the bamboo field, it was necessary to develop a model, able to implement an efficient computation of the carbon stocked in the gross biomass of the bamboo field. In particular, sheaths, leaves and branches contribute to the above-ground fraction of bamboo, while the below-ground fraction consists of woody roots and rhizomes. The evaluation of carbon stock fluctuations within the plantation had to be carried out in a timeframe comprised between the planting and at least the completion of the 10th year of the bamboo life cycle. This time interval represented the “crediting period” of the actual case study, as well as the time required by plants to reach maturity. Only at this stage a neat balance between release and sequestration of carbon dioxide can be observed. Such computation of the CO2 removed by the bamboo field was based on the modified technical procedure of a CDM project within the Framework Convention on Climate Change (AR-AMS0007, UNFCCC, 2021).
where:
Cproj,t = The net carbon stock generated by the project in year t, measured in tonnes of carbon dioxide equivalent per year (tCO2e y−1);
DCproj,t = The change in carbon stock in year t under the project scenario (tCO2e y−1);
DCBSL,t = The baseline carbon stock change in year t (tCO2e y−1);
LKt = The leakage out of project boundaries caused by project activity in year t (tCO2e y−1);
t = the years after the project started.
For the prediction of culm’s pattern change during the development of the bamboo plantation up to the mature stage (Supplementary Table S1) the trend development by Guomo et al., 2013 was used. The increment observed for each year was measured vs. the previous one. After the seventh year (age of plant’s maturity), the predictive model must take into account an annual cutting intervention that is estimated to remove 20% of mature plants. Additionally, the density increment was calculated not only according to the model reported in Supplementary Table S1, but also considering that from the 8 year on, the first thousand bamboo individuals originally planted will be dead. For the determination of allometric parameters, namely, the average diameters (DBH, diameter at breast height) and heights, of the Moso bamboo population during the period 2016–2031, the following equations from the INBAR protocol were used (Guomo et al., 2013).
where DBH is “diameter at breast height,” H is “height,” T is “plant age” (year). The above parameters are fundamental for the calculation of the parameter W, corresponding to the value of the above ground biomass of each culm (expressed as kg · culm-1).” In order to make a correlation between the allometric equations and the field data, a correction factor (C = 0.32) was needed to take into account the geopedoclimatic context. Therefore, the culm heights were corrected by this factor, that also includes all other conditions that may have resulted in lower culm growth with respect to the INBAR protocol estimate. For the quantification of the gross above ground biomass (W), the following equation (Kuehl et al., 2013) was applied:
where DBH, diameter at breast height.
Once calculated the W parameter, it was used for the measurement of the above ground gross biomass per hectare:
In order to measure the carbon stored in the above ground biomass, the Bw value has to be further multiplied by a dimensionless value, called “carbon fraction of dry matter” (CF) (Penman et al., 2003). The CF default value is 0.5 and it is measured in tonnes C dry matter (d.m.).
The next step consists of the conversion of the Cstored value into the CO2 stored value, namely the tons of carbon dioxide equivalent per hectare. In order to obtain such value, the Cstored must be multiplied by a dimensionless factor (f), equal to 3.66, which is the ratio between the molecular mass of CO2 and the atomic mass of C:
Successively, to make an accurate prediction, the amount of segregated carbon dioxide that is lost after cutting must be taken into account. As above detailed, starting from the 8 years an aliquot of 20% mature culms is removed yearly, indeed decreasing the annual culm density (D). The effective amount of CO2 segregated by the above ground biomass of the bamboo plantation, after deduction of the aliquot captured by the cut biomass, has to be therefore recalculated. The calculated CO2 fraction segregated by the cut biomass has to be subtracted from the CO2 stored aliquot, in order to obtain the effective number of tCO2e captured by the entire field during the time interval of 0–15 years.
Discussion
Figure 2A reports a provision of the field density increment(the number of culms ⋅ ha−1 and the total number of culms in the field) related to the first 15 years of observation, starting from 2016, when the density measured was 1,000 culms ⋅ ha−1. According to the prediction model made by Guomo et al. on the density trend during the development of the bamboo plantation, in 2020 (year 5) the calculated density proves to be 6,510 culms ha−1, that corresponds to the value certified in situ in November 2020. The figure highlights that, starting from the seventh year, the effective population of bamboo plants is lower, in consideration of the aliquot of cut plants. As can be seen in Figure 2B, that reports the changes in diameter and height vs. time, in the 10th year a reduction in both values occurs; this finding is again attributable to the cutting practice introduced after the seventh year, which leads to the removal of old and dead plants, and their parallel replacement with seedlings and younger individuals of lower size. Table 1 reports the above ground biomass values with respect to bamboo size and density. Both DBH and W values show an increasing trend up to year 9, while a drop occurs at around the 13th–15th years of plantation age. The parameter D (density) is constantly increasing because of plants propagation, whereas the Bw value decreases from year 13 (485.19 t ⋅ ha−1) to year 15 (234.95 t ⋅ ha−1). Different methodologies are available in literature for the quantification of the gross above ground biomass (Bw) of moso bamboo forests/plantations. Guomo et al. reported three different methodologies: 1) W is calculated per each culm, by taking a sample of 97 individuals in a defined area (96% reliability); 2) W is calculated per each culm, by taking a sample of 63 individuals in two defined areas (91.5% reliability); 3) W is calculated per each culm, by taking a sample of 58 individuals in a single defined area (67.8% reliability) (Guomo et al., 2013). In the present study, a computational model has been developed by modifying the models present in literature and afore mentioned. This was due to a number of specific requirements and/or characteristics of the plantation under investigation located in the Italian environment. The number of reference areas was higher, as well as the sample size; the series of equations applied was extremely simple, and basically focused on the sole average DBH; the resulting reliability index was very good. Through the exploitation of the equation set, the gross above ground biomass with correspondent stocks of C and CO2 for each hectare have been reported in Table 2. As can be seen, all the values gradually increase until year 12, to drop down in the last 3 years of the period considered. The predictive model provides a maximum of 912.83 tCO2e ⋅ ha−1; according to the model, this value must be reduced to 730.26 tCO2e ⋅ ha−1 since it is necessary to subtract the portion removed by cutting. In fact, this sink must be subtracted from the values, as reported in Supplementary Table S2. The neat sequestration power of the whole bamboo plantation has been shown in Figure 3. The maximum amount of captured CO2 is predicted to be reached on the 12th year (∼3,651.32 ca. tCO2e). A comparison between the present findings and other studies results to be difficult because of great variability in the published data. Numerous factors, including environment, management practices, biological characteristics, analytical methodologies, not least location and bamboo species, deeply contribute to this variability (Yuen et al., 2017). For instance, the average value of the above ground biomass (Bw) for moso bamboo reported by Chen et al. (2018) for a DBH of 8.48 cm was about 960 t ⋅ ha−1 vs. 156.17 t ⋅ ha−1 of our study (year 5, DBH 8.49 cm).” This difference has to be basically attributed to either the geopedoclimatic conditions (namely China) or the forest management (spontaneous growth), with the only similarities laying in the bamboo species and the analytical methodology. However, the correlation between culm density and above ground biomass is in accord with findings by Nath et al. (2015), who observed a constant increase of Bw along with D.
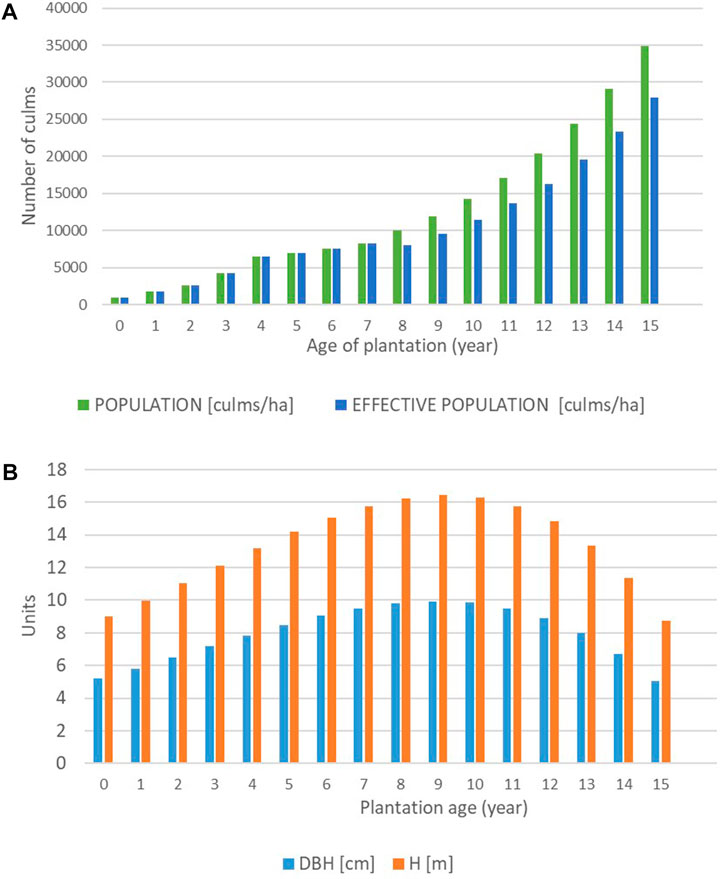
FIGURE 2. (A) Pattern of culm change during a timeframe of 15 years. Population: total number of bamboo individuals. Effective population: number of culms net of the cut fraction; (B) Culm changes in the time interval 0–15 years of bamboo plantation development. DBH, diameter at breast height; H, culm height.
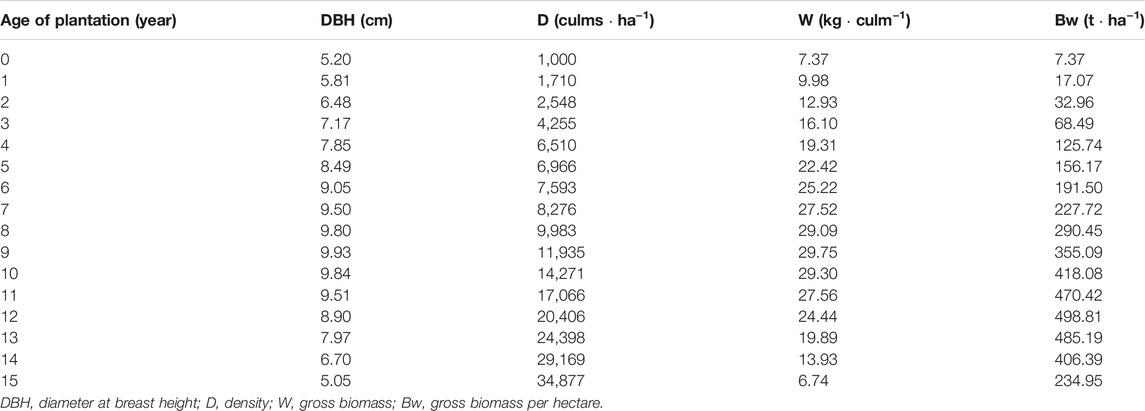
TABLE 1. Correlation of diameter (DBH) and density (D) with the amount of gross biomass above-ground of the entire plantation (W) and of each unit of land (Bw).
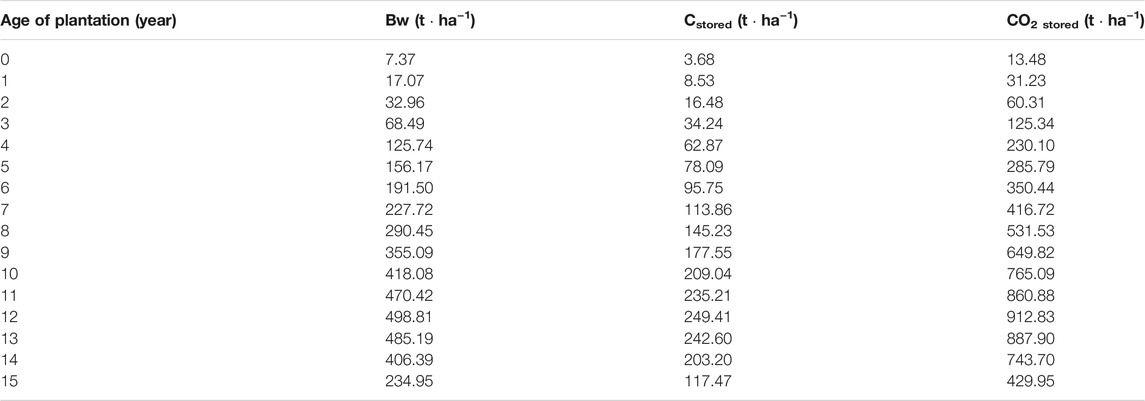
TABLE 2. Gross biomass above-ground (Bw), amount of carbon and of carbon dioxide stored in the above-ground biomass (Cstored and CO2 stored).
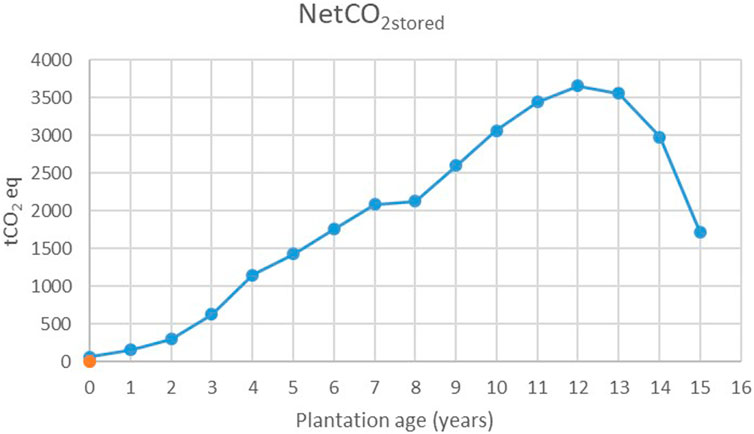
FIGURE 3. Net carbon dioxide fraction segregated by the entire Moso bamboo plantation after subtraction of the aliquot stored in the cut fraction (20% above-ground biomass).
Conclusion
From the results obtained in the present study through the application of a predictive and computational model, the following considerations can be drawn. The reconstruction of the geopedoclimatic and socioeconomic scenarios of the investigated plantation results to be fully suitable to the development of a GHG project. The climate is adequate to sustain both growth and thickening of the moso plantation as it has been widely demonstrated by field’s data, although plans for constant management are required in order to maximize its development. The important preventive action against hydrogeological instability exerted by Moso bamboo may give the GHG project additional advantages in terms of environmental protection. This management activity could be a valuable opportunity for social and economic recovery for the territory and local people. The data presented in this study confirm that the species Phyllostachys pubescens is among the most promising in contrasting climate change, and, more generally, in the implementation of strategies to reduce CO2 emissions. Future studies might be focused on the computation of the below ground carbon sink, given the lack on this specific topic shown by literature. The development and diffusion in the Italian territory of GHG projects based on afforestation with moso bamboo can be safely included within the activities oriented to an efficient socio-economic and territorial restart, and to a faster transition to sustainability in the frame of a circular bio-economy management.
Data Availability Statement
The original contributions presented in the study are included in the article/Supplementary Material, further inquiries can be directed to the corresponding author.
Author Contributions
GD and RV contributed to conceptualization; GD and NC contributed to project administration; RV contributed to software, methodology, and data collection; RC contributed to draft revision; RC and RV contributed to writing, review and editing of the draft; NC and GD contributed to supervision. All authors have read and agreed to the published version of the manuscript.
Conflict of Interest
The authors declare that the research was conducted in the absence of any commercial or financial relationships that could be construed as a potential conflict of interest.
Publisher’s Note
All claims expressed in this article are solely those of the authors and do not necessarily represent those of their affiliated organizations, or those of the publisher, the editors and the reviewers. Any product that may be evaluated in this article, or claim that may be made by its manufacturer, is not guaranteed or endorsed by the publisher.
Supplementary Material
The Supplementary Material for this article can be found online at: https://www.frontiersin.org/articles/10.3389/fenvs.2022.817177/full#supplementary-material
References
Agnoletti, M., Manganelli, S., and Piras, F. (2020). Covid-19 and Rural Landscape: The Case of Italy. Landscape Urban Plann. 204, 103955. doi:10.1016/j.landurbplan.2020.103955
Barcaccia, G., D’Agostino, V., Zotti, A., and Cozzi, B. (2020). Impact of the SARS-CoV-2 on the Italian Agri-Food Sector: An Analysis of the Quarter of Pandemic Lockdown and Clues for a Socio-Economic and Territorial Restart. Sustainability 12 (14), 5651. doi:10.3390/su12145651
Bleaney, A., Peskett, L., and Mwayafu, D. (2010). REDD-plus after Copenhagen: what Does it Mean on the Ground. REDD-net COP15 Brief. Available from: https://www.iisd.org/system/files/publications/redd_after_copenhagen.pdf (accessed Apr 11, 2021).
Boyd, E. (2009). Governing the Clean Development Mechanism: Global Rhetoric versus Local Realities in Carbon Sequestration Projects. Environ. Plan. A. 41 (10), 2380–2395. doi:10.1068/a41341
Brotto, L., Corradini, G., Chiriacò, M. V., Portaccio, A., Perugini, L., Pettenella, D., et al. (2018). Stato del Mercato Forestale del Carbonio in Italia 2018. Nucleo Monitoraggio del Carbonio, CREA, Rome. Available from: https://www.etifor.com/it/portfolio/nucleo-carbonio-report-2018 (Accessed June 21, 2021).
Canavan, S., Richardson, D. M., Visser, V., Le Roux, J. J., Vorontsova, M. S., and Wilson, J. R. U. (2016). The Global Distribution of Bamboos: Assessing Correlates of Introduction and Invasion. AOB Plants. Available from: www.aobplants.oxfordjournals.org (Accessed January, 2022). doi:10.1093/aobpla/plw078
Chen, C., Huang, Z., Jiang, P., Chen, J., and Wu, J. (2018). Belowground Phytolith-Occluded Carbon of Monopodial Bamboo in China: An Overlooked Carbon Stock. Front. Plant Sci. 9, 1615. doi:10.3389/fpls.2018.01615
Chen, T. H., Wang, D. H., and Wang, S. (2016). The Trend of Growth Characteristics of Moso Bamboo (Phyllostachys Pubescens) Forests under an Unmanaged Condition in central Taiwan. Taiwan J. For. Sci. 31 (2), 75–88.
Climate-data org (2021). Climate Data [Internet]. Available from: https://it.climate-data.org/(Accessed April 12, 2021).
D'Adamo, I., Gastaldi, M., Imbriani, C., and Morone, P. (2021). Assessing Regional Performance for the Sustainable Development Goals in Italy. Sci. Rep. 11 (1), 24117. doi:10.1038/s41598-021-03635-8
Dwivedi, A. K., Kumar, A., Baredar, P., and Prakash, O. (2019). Bamboo as a Complementary Crop to Address Climate Change and Livelihoods - Insights from India. For. Pol. Econ. 102, 66–74. doi:10.1016/j.forpol.2019.02.007
Global Carbon Project (GCP) (2021). Canberra: 2001-2021. Available from: https://www.globalcarbonproject.org (Accessed Apr 27, 2021).
Gravina, T., Figliozzi, E., Mari, N., and De Luca Tupputi Schinosa, F. (2017). Landslide Risk Perception in Frosinone (Lazio, Central Italy). Landslides 14 (4), 1419–1429. doi:10.1007/s10346-016-0787-2
Guomo, Z., Yongjun, S., Yiping, L., Jinliang, L., Kuehl, Y., Jianhua, C., et al. (2013). Beijing: INBAR. Available from https://www.inbar.int/wp-content/uploads/2020/05/1489548402.pdf (Accessed May 15, 2021). Methodology for Carbon Accounting and Monitoring of Bamboo Afforestation Projects in China.
IPCC (2019). Intergovernmental Panel on Climate Change (2019) Special Report. Climate Change and Land. Available from: https://www.ipcc.ch/report/srccl (Accessed May 21, 2021).
Isagi, Y., Kawahara*, T., Kamo*, K., and Ito, H. (1997). Net Production and Carbon Cycling in a Bamboo Phyllostachys Pubescens Stand. Plant Ecol. 130 (1), 41–52. doi:10.1023/A:1009711814070
ISTAT (2011). 8milaCensus. Available from: http://ottomilacensus.istat.it/fileadmin/report/060/report_060011.pdf (Accessed April 14, 2021).
King, C., van der Lugt, P., Trinh, T. L., and Li, Y. (2021). Integration of Bamboo Forestry into Carbon Markets. INBAR Working Paper. Beijing, China: International Bamboo and Rattan Organisation (INBAR). Available from: https://www.inbar.int/resources/inbar_publications/integration-of-bamboo-forestry-into-carbon-markets/.
Kleinhenz, V., and Midmore, D. J. (2001). Aspects of Bamboo Agronomy. Adv. Agron. 74, 99–153. doi:10.1016/s0065-2113(01)74032-1
Köppen, W. (1936). in Handbuch der Klimatologie. Editors W. Köppen, and R. Geiger (Berlin: Gebruder Borntraeger), C-1–C-44. [online] URL.
Kreibich, N., and Hermwille, L. (2021). Caught in between: Credibility and Feasibility of the Voluntary Carbon Market post-2020. Clim. Pol. 21 (7), 939–957. doi:10.1080/14693062.2021.1948384
Kuehl, Y., Li, Y., and Henley, G. (2013). Impacts of Selective Harvest on the Carbon Sequestration Potential in Moso Bamboo (Phyllostachys pubescens) Plantations. Forests, Trees and Livelihoods 22, 1–18. doi:10.1080/14728028.2013.773652
Lang, S., Blum, M., and Leipold, S. (2019). What Future for the Voluntary Carbon Offset Market after Paris? an Explorative Study Based on the Discursive Agency Approach. Clim. Pol. 19 (4), 414–426. doi:10.1080/14693062.2018.1556152
Le Quéré, C., Andrew, R. M., Friedlingstein, P., Sitch, S., Hauck, J., Pongratz, J., and Zheng, B. (2018). Global Carbon Budget 2018. Earth Syst. Sci. Data 10 (4), 2141–2194. doi:10.5194/essd-10-2141-2018
Lefebvre, D., Williams, A. G., Kirk, G. J. D., Paul, J., Burgess, J., Meersmans, J., et al. (202119907). Assessing the Carbon Capture Potential of a Reforestation Project. Sci. Rep. 11. doi:10.1038/s41598-021-99395-6
Lobovikov, M., Schoene, D., and Yping, L. (2012). Bamboo in Climate Change and Rural Livelihoods. Mitig Adapt Strateg. Glob. Change 17 (3), 261–276. doi:10.1007/s11027-011-9324-8
Marcu, A. (2015). Mitigation Value, Networked Carbon Markets and the Paris Climate Change Agreement. Available from http://pubdocs.worldbank.org/pubdocs/publicdoc/2015/9/840951442526241099/Mitigation-Value-Networked-Carbon-Markets-and-the-Paris-Climate-Change-Agreement.pdf.
Nath, A. J., Lal, R., and Das, A. K. (2015). Managing Woody Bamboos for Carbon Farming and Carbon Trading. Glob. Ecol. Conservation 3, 654–663. doi:10.1016/j.gecco.2015.03.002
ONPR (Osservatorio Nazionale del Paesaggio Rurale) (2018). Primo Rapporto sullo Stato del Paesaggio Rurale, Ministero delle Politiche Agricole Alimentari e Forestali. Roma, Settembre 2018. Available from: https://www.reterurale.it/flex/cm/pages/ServeBLOB.php/L/IT/IDPagina/18583 (Accessed May 20, 2021).
Paleari, S. (2018). Natural Disasters in Italy: Do We Invest Enough in Risk Prevention and Mitigation? Int. J. Environ. Stud. 75 (4), 673–687. doi:10.1080/00207233.2017.1418995
Penman, J., Gytarsky, M., Hiraishi, T., Krug, T., Kruger, D., Pipatti, R., et al. (2003). Good Practice Guidance for Land Use, Land-Use Change and Forestry. Kanagawa: IPCC 2003. Available from: https://www.ipcc-nggip.iges.or.jp/public/gpglulucf/gpglulucf.html.2003.
Regulation, E. U. (2018). 841 of the European Parliament and of the Council of 30 May 2018 on the Inclusion of Greenhouse Gas Emissions and Removals from Land Use, Land Use Change and Forestry in the 2030 Climate and Energy Framework, and Amending Regulation (EU) No 525/2013 and Decision No 529/2013/EU. Official J. Eur. Union 19, 1–25.
Santoro, A., Venturi, M., Bertani, R., and Agnoletti, M. (2020). A Review of the Role of Forests and Agroforestry Systems in the FAO Globally Important Agricultural Heritage Systems (GIAHS) Programme. Forests 11 (8), 860. doi:10.3390/f11080860
Singh, L., Sridharan, S., Thul, S. T., Kokate, P., Kumar, P., Kumar, S., et al. (2020). Eco-rejuvenation of Degraded Land by Microbe Assisted Bamboo Plantation. Ind. Crops Prod. 155, 112795. doi:10.1016/j.indcrop.2020.112795
Singh, L., Thul, S. T., and Mohan Manu, T. (2021). “Development of Bamboo Biodiversity on Mining Degraded Lands: A Sustainable Solution for Climate Change Mitigation,” in Phytorestoration of Abandoned Mining and Oil Drilling Sites (Amsterdam: Elsevier), 439–451. doi:10.1016/b978-0-12-821200-4.00002-9
Streck, C. (2020). Who Owns REDD+? Carbon Markets, Carbon Rights and Entitlements to REDD+ Finance. Forests 11 (8), 959. doi:10.3390/f11090959
UNFCCC (2021). AR-AMS0007/Version 02.0.0. Simplified Baseline and Monitoring Methodology for Small Scale CDM Afforestation and Reforestation Project Activities Implemented on Wetlands. Available from: https://cdm.unfccc.int/methodologies/DB/8LLTGVPG1SMMB1AMGSU0ZEYVO9P45P/view.html (Accessed May 5, 2021).
UNFCCC (2014). Compilation of Information on Mitigation Benefits of Actions, Initiatives and Options to Enhance Mitigation Action: List of Selected Cooperative Initiatives [online]. Available from: http://unfccc.int/meetings/bonn_jun_2013/items/7655.php (Accessed February 3, 2021).
UNFCCC (2008). Painting the Forest REDD? Prospects for Mitigating Climate Change through Reducing Emissions from Deforestation and Degradation. IED Working Paper 3. Available from: https://redd.unfccc.int/uploads/2_119_redd_20081117_ied.pdf (Accessed March 5, 2021).
UNFCCC (2007). Views on Issues Related to Further Steps under the Convention Related to Reducing Emissions from Deforestation in Developing Countries: Approaches to Stimulate Action Submissions from Parties. Available from http://unfccc.int/resource/docs/2007/sbsta/eng/misc14.pdf (Accessed May 24, 2021).
Yuen, J. Q., Fung, T., and Ziegler, A. D. (2017). Carbon Stocks in Bamboo Ecosystems Worldwide: Estimates and Uncertainties. For. Ecol. Manage. 393, 113–138. doi:10.1016/j.foreco.2017.01.017
Keywords: Phyllostachys pubescens, GHG, carbon farming, computational model, allometric parameters, moso bamboo, central Italy
Citation: Vadalà R, Cicero N, Dugo G and Costa R (2022) Suitability and Eligibility of Phyllostachys pubescens (Moso Bamboo) Afforestation for GHG (Greenhouse Gases) Projects: Case Study in Central Italy. Front. Environ. Sci. 10:817177. doi: 10.3389/fenvs.2022.817177
Received: 18 November 2021; Accepted: 11 March 2022;
Published: 04 April 2022.
Edited by:
Hong Liao, Nanjing University of Information Science and Technology, ChinaReviewed by:
Lal Singh, National Environmental Engineering Research Institute (CSIR), IndiaSunil Nautiyal, Institute for Social and Economic Change, India
Copyright © 2022 Vadalà, Cicero, Dugo and Costa. This is an open-access article distributed under the terms of the Creative Commons Attribution License (CC BY). The use, distribution or reproduction in other forums is permitted, provided the original author(s) and the copyright owner(s) are credited and that the original publication in this journal is cited, in accordance with accepted academic practice. No use, distribution or reproduction is permitted which does not comply with these terms.
*Correspondence: Rosaria Costa, costar@unime.it
†These authors share first authorship