- 1Institute of Environmental Engineering, Wroclaw University of Environmental and Life Sciences, Wrocław, Poland
- 2Institute of Soil Science, Plant Nutrition and Environmental Protection, Wroclaw University of Environmental and Life Sciences, Wrocław, Poland
This study aimed to assess the impact of a hydropower plant (HP) on the Bystrzyca River (left tributary of the Odra River, Poland) on selected parameters of the bottom sediment within this hydrotechnical structure. The following parameters were examined as part of the analyses: particle size distribution, pH, electrical conductivity (EC), and concentrations of Cd, Cu, Cr, Ni, Pb and Zn. Field studies were carried out upstream and downstream of the HP and at reference points. In addition, the risk of heavy metals in aquatic organisms was assessed using an ecotoxicological method. Based on the Wilcoxon signed-rank test, it was found that the HP influenced all of the parameters tested, except pH. Comparing the points closest to and downstream of the HP, an increase in the average grain diameter of D50 (on average by 750%), and a decrease in the concentrations of most heavy metals: Ni (271%), Zn (216%), Cu (163%), Cr (83.0%), and Cd (74.2%), was observed. The ecotoxicological classification of heavy metal concentrations in sediments indicates, in most cases, little or no impact on aquatic organisms; the values of Ni and Zn in some samples upstream of the HP are exceptions, where remediation using synthetic zeolites, metal accumulation plants, or by electrokinetic methods, is necessary. Fine-grained fractions dominate the clays and sandy clays upstream of the hydropower plants, while sands, sandy clay loams, or sandy clays are dominated downstream. Frequent occurrence of skeletal fractions (>2.0 mm) was observed downstream, i.e., an average of 23.30% in each sample compared to 1.82% upstream of the HP, which is mainly due to greater anthropogenic pressure, especially tourism. The dominance of fine-grained fractions upstream of the HPs favors the accumulation of metals due to the high sorption capacity of these fractions. When comparing the analyzed groups of points, the average pH values are arranged in the following sequence: upstream HP (U) > downstream HP (D) > reference points (R), while EC has opposite values (i.e., U < D < R). This study may support sustainable sediment management and may allow for the development of recommendations related to the rational management of HPs.
Introduction
Bottom sediments support the function of water-related and water-dependent ecosystems, and are home to various organisms. They store numerous organic and inorganic substances; however, some are pollutants such as metals (Rajfur et al., 2011; Alyazichi et al., 2017).
The sources of metals may be natural (e.g., from the geological structure of the surrounding area, the processes of weathering organic matter or atmospheric deposition) or artificial (e.g., sewage discharges, runoff from agricultural land, mines, landfills, urban areas) (Asaeda & Rashid, 2012; Usman et al., 2021). These elements are strongly adsorbed by the smallest fractions of sediments (silts and clays), and studies have shown that up to 99% of metals present in ecosystems can accumulate in sediments (Ndeda et al., 2017). Metals are dangerous because of their ability to migrate to various elements of the environment, such as water, sediment, and soil (Ahamad et al., 2020). Moreover, they exhibit low solubility (Capillo et al., 2018). They accumulate in organisms (no biodegradability) and combined with their toxic effects in the event of overdosing, they can seriously affect the health and life of organisms (Pagano et al., 2017; Dra et al., 2019; Miao et al., 2021). Because of such toxicity, monitoring areas exposed to metals is important (e.g., mining, industrial) (Manoj & Padhy 2014; Bhuyan et al., 2019), for introducing various remediation methods (i.e., neutralization by removing them from the environment or immobilization) (Peng et al., 2009; Cuske, Karczewska & Gałka 2017).
Hydropower plants located at the barrages separating rivers are also modifiers with regard to the transport conditions of the bottom sediment, including the spatial distribution of contained substances (Winton et al., 2019). Hydrotechnical structures constitute a transverse obstacle in river beds, seriously affecting the flow conditions and hydrological regime, geomorphological conditions, and living conditions of aquatic organisms (Li et al., 2012; Kasperek & Wiatkowski, 2014; Ashraf et al., 2018; Nistoran et al., 2018; Boulange et al., 2021; Kuriqi et al., 2021). Their action in relation to bottom sediments consists of their retention upstream of damming thresholds. It is estimated that more than 53% of the sediment flow worldwide is trapped in upstream dams (Wild and Loucks, 2014). As a result of this phenomenon, significant reduction in transported sediment load in rivers is observed in the downstream of dams with hydropower plants, affecting the function of aquatic and water-related ecosystems (Wiatkowski, 2010; Gabbud and Lane, 2016). For example, after the construction of the Great Aswan Dam in 1964 in the Nile Delta in Egypt, the transported net sediment load decreased from over 100 Mt annually to almost zero (Stanley and Clemente, 2011). Moreover, the capacity of suspended sediments in the Danube River (the Ceatal Izmail station) fell from 80 t/km2 in 1931 to 30 t/km2 in 1997 (Walling and Fang, 2003). Considering the sedimentary texture and composition, the average diameter of the sediment grains decreases with the distance from the hydropower plant, due to the decreasing transport capacity of the river (turbulent movement resulting from the phenomenon of hydraulic bounce in downstream of the hydrotechnical structures) (Tomczyk et al., 2022). Additionally, increased erosion is observed downstream of the dams; for example, the annual amount of the material thus eroded ranged from 3.5 · 103 to 7.2 · 103 t in the Arc River (Antoine et al., 2020).
Because of the above-mentioned impacts of dams, developing solutions and technologies that enable the sustainable management of sediments is necessary; for example, the following three strategies are commonly adopted for dam reservoirs: directing sediments through or around the reservoir, removing sediments from the reservoir to regain their capacity, and minimizing the amount of sediment reaching reservoirs from the headwaters (Kondolf et al., 2014; Hauer et al., 2018; Katano et al., 2021). Conversely, implementing international energy policies is crucial; for example, the European Union planned to reduce greenhouse gas emissions by 2030, increase the energy efficiency of member states, and increase the share of renewable energy sources (including hydropower) in final energy consumption (European Parliament and the Council 2009; Arabatzis and Myronidis, 2011; Wiatkowski, Rosik-Dulewska and Tomczyk, 2017).
This study aimed to assess the impact of a hydropower plant on the Bystrzyca River (left tributary of the Odra River, Poland) considering selected parameters of the bottom sediment within this hydrotechnical structure. The following parameters were analyzed: particle size distribution, pH, electrical conductivity (EC), Cd, Cu, Cr, Ni, Pb and Zn. In addition, the risk of metals on aquatic organisms was assessed using an ecotoxicological method. The need for sediment remediation was determined, which is in line with the assumptions of sustainable sediment management. This study is an important contribution to research on the environmental impacts of hydropower plants, which may facilitate future recommendations for the rational management of such facilities. The issues raised are important from the social, economic, and environmental perspectives. There has been little research on the influence of hydropower plants on the properties of river sediments.
Materials and Methods
Field Research
Field tests were carried out in two series of measurements, in spring and autumn of 2019. Bottom sediment was collected at points located on the Bystrzyca River (left tributary of the Odra River, Poland), near the Sadowice hydropower plant (51°03′15″N 16°47′42″E—51°03′22″N 16° 47′49″E) and at reference points in Sadowice and Małkowice (51° 03′30″N 16° 47′51″E and 51°04′39″N 16°49′05″E). The hydropower plant is located 26.05 km from the mouth of the Bystrzyca River, and has a capacity and damming height of 0.06 MW and 1.8 m, respectively; it began operating in 1921 (Tomczyk and Wiatkowski, 2021). The locations of the test area and sediment sampling points are shown in Figure 1.
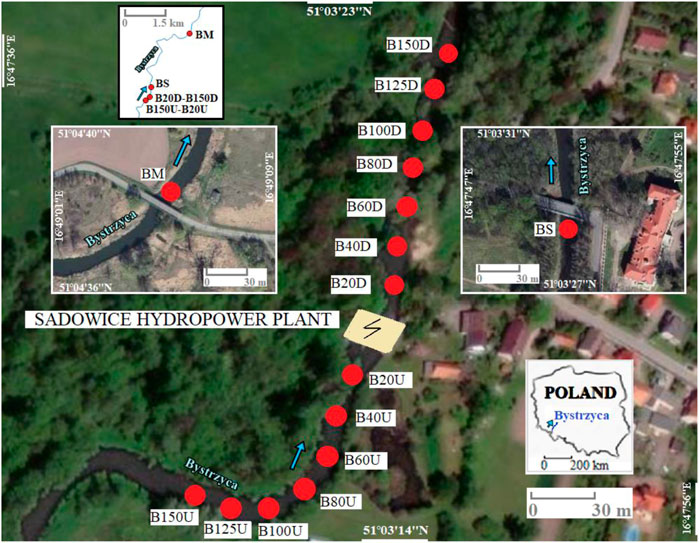
FIGURE 1. Location of the sediment sampling area on the Bystrzyca River (B—Bystrzyca River, numbers—distance from the hydropower plant, U—point upstream the hydropower plant, D—point downstream the hydropower plant, M—Małkowice, S—Sadowice).
Bottom sediments were collected from the near-surface layer, approximately 5 cm thick, using a 2,500 cm3 Van Veen grab sampler. The samples were then transferred to polyethylene bags and transported to the laboratory in a refrigerator (Duodu, Goonetilleke and Ayoko 2016).
Laboratory Tests
The sediment samples delivered to the laboratory were stored at room temperature and protected from sunlight. Bottom sediment samples dried under such conditions were tested for their particle size distribution (the Bouyoucos areometric method modified by Casagrande and Prószyński) (Bojko et al., 2017; Waroszewski et al., 2019), metals such as Cu, Ni, Pb, Cr, Zn, and Cd (mineralization in aqua regia, atomic absorption spectrophotometry (AAS) (Sarmani 1989; He, Shi & Wu 2016), conductometer, and pH (the potentiometric method in water). The research was carried out in the laboratory of the Institute of Soil Sciences, Plant Nutrition and Environmental Protection of the Wrocław University of Environmental and Life Sciences. The procedure for the determination of metals and the particle size distribution of the tested sediment is presented in Figures 2, 3.
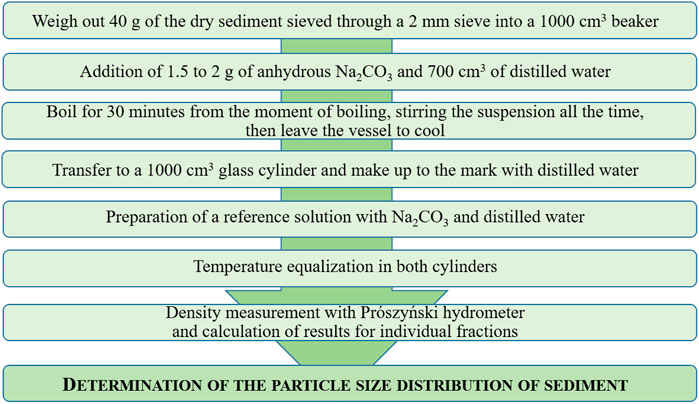
FIGURE 3. Procedure for the determination of the particle size distribution of investigated sediment.
Data Analysis
The classification of the grain size composition was determined on the basis of its texture, i.e., the ratio of the content of silt (≤0.002 mm), clay (0.002–0.05 mm), and sand (0.05–2.0 mm), summed up to 100% (Bieganowski et al., 2013; Kruczkowska et al., 2020). The soil taxonomy established by the United States Department of Agriculture and the National Cooperative Soil Survey (USDA classification) was used (Arfania et al., 2018; Borselli et al., 2008). The following are the types of soil: sand, loamy sand, sandy loam, sandy clay loam, sandy clay, loam, clay loam, clay, silty clay, silty clay loam, and silt. The study also presents the division of sediments into sediment skeleton particles (>2.0 mm) and fine Earth (≤2.0 mm). Additionally, the mean particle diameter of the bottom sediment (D50) in the Earth parts of the tested sediment samples was calculated for determining the weighted average for the upper values of the diameters in the designated ranges (i.e., 0.002, 0.006, 0.02, 0.05, 0.1, 0.25, 0.5, 1.0, and 2.0 mm).
The content of metals in the sediments was assessed in terms of their potential toxicity to aquatic organisms, using the ecotoxicological method (MacDonald et al., 2000; Tomić et al., 2019; Jaskuła et al., 2021; Wdowczyk et al., 2022). The method distinguishes three levels of toxicity characteristics:
• Threshold effect concentration (TEC): maximum content of the element that does not adversely affect benthic organisms.
• Probable effect concentration (PEC): concentration at which the negative effect of the substance on benthic organisms becomes noticeable.
• Midpoint Effect Concentration (MEC): mean of TEC and PEC values.
Based on the determined levels of TEC, MEC, and PEC, four classes of metal toxicity can be distinguished: in class I, there are no symptoms of toxicity; in II and III, toxicity may occur with varying severity; in IV, toxicity is certain. Table 1 presents the ecotoxicological classifications of the tested metals.
To illustrate the potential impact of the hydropower plant, the percentage difference between the results obtained for the sediment samples located closest to the hydropower structure (i.e., at a distance of 20 m from the hydropower plant) was calculated. Additionally, the results were sorted according to the sediment collection date.
This article presents basic descriptive statistics for all determined parameters (minimum, maximum, mean, and standard deviation). The statistical significance of the results between the two groups of points (upstream and downstream of the hydropower plant) was also tested using the two-tailed Mann–Whitney U test (Wilcoxon signed-rank test). Therefore, assessing the parameters influenced by the operation of the hydrotechnical construction is possible. The null hypothesis for this test is that the medians in both study groups are equal, and the distribution of the dependent variables does not match the normal distribution. The test calculates U and z-ratio values using the formulas presented in Table 2 (significance level p < 0.01 and p < 0.05) (McKnight et al., 2010).
The analyses were performed using Statistica 13 (Dell Computer Corporation, 2021), SPSS Statistics 26 (IBM Corporation, 2019), OriginLab Corporation, 2021 (OriginLab, Northampton, MA, United States; boxplots) and Office 2013 (Microsoft Corporation, 2013). The area was mapped using QGIS 2.8.4 (QGIS Development Team, 2015; Open Source Geospatial Foundation Project). The analytical procedure used in the article is shown in Figure 4.
Results and Discussion
Basic Statistics
The values of the examined parameters varied within the range, as shown in Table 3. With regards to the average values, the following parameters decreased at points downstream of the hydropower plant compared to the points upstream of the hydropower plant: EC (change by 112%), Cd (232%), Cr (145%), Cu (227%), Ni (208%), Pb (131%) and Zn (241%), and an increase in D50 (489%) and pH (17.90%). Considering the groups of points in relation to the reference points, mean values for D50, Cu, Ni, Cr, Zn, and Cd were observed, the highest for pH and Pb, and the lowest for EC. Detailed analyses are presented in Particle Size Distribution and Changes in Parameter Values Within a Hydropower Plant.
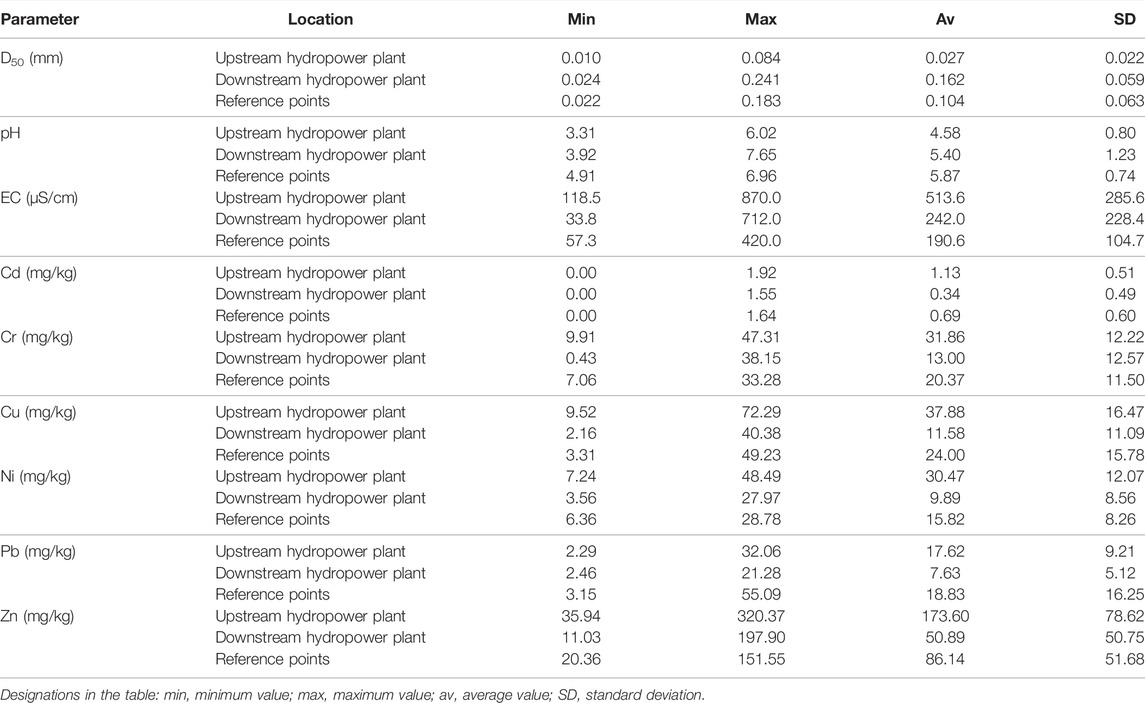
TABLE 3. Basic statistics of the results obtained for the tested parameters in the sediments of the Bystrzyca River.
Particle Size Distribution
The texture of the investigated sediment differed between the upstream and downstream points of the hydropower plant and the reference points. In upstream of the hydropower plant, the sediment belonging to clays or sandy clays dominated; sediment belonging to sands, sandy loams and clay dominated in the downstream; at reference points, sediments belonging to sands, sandy clay loams, or sandy clays, dominated. The results varied between seasons; in spring, the texture was more concentrated in coarse sands and loams, while in autumn, it was more heterogeneous and also counted among the clays (Figure 5).
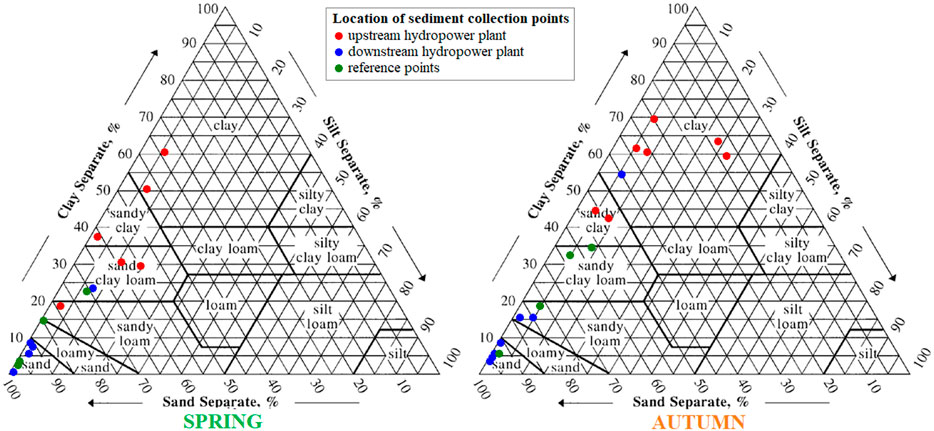
FIGURE 5. The texture of the tested sediment from points within the hydropower plant on the Bystrzyca River.
A significant increase in D50 is visible downstream of the hydropower plant, regardless of the season (Figure 6). The reference points represent the full cross-section of the sediment graining, which is intermediate between the compared groups. Considering seasons, there was a decrease in the D50 particle size upstream of the hydropower plant (spring: 0.108 mm, autumn: 0.101 mm) and at the reference points (spring: 0.041 mm, autumn: 0.016 mm), while an increase was observed downstream of the hydro-engineering structure (spring: 0.152 mm, autumn: 0.169 mm). Considering the reference points, there was a similar dispersion of the D50 values between the seasons, while in the remaining points there were differences between spring and autumn (upstream of the hydropower plant in spring: 0.014–0.084 mm, autumn: 0.010–0.025 mm; downstream the hydropower plant in spring: 0.090–0.216 mm, autumn: 0.024–0.241 mm).
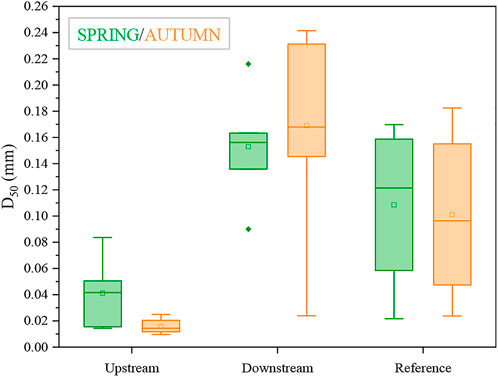
FIGURE 6. The average diameter of the sediment grains (D50) from the Bystrzyca River at the studied measuring points.
The reflection of the previous results can also be seen in the fraction of gravel and stones, i.e., with a diameter greater than 2 mm (Figure 7). A higher percentage of coarse grains was recorded at downstream of the hydropower plant and at the reference points, than at upstream of the hydropower plant (average total share: 23.30, 19.61, 1.82%). The results differ depending on the season, especially at upstream of the hydropower plant, skeleton particles were recorded in spring (an average share of 3.94% in the studied sediments), while in autumn, they were not observed. For the remaining points, the differences were small. The coarse-grained fraction included gravel, stones, branches, leaves, and waste, which accumulated mainly downstream of the hydropower plant and at reference points, due to trees and shrubs overgrowing the banks of the watercourse, and anthropogenic pressure (mainly tourism and recreation, artificial beaches, canoeing, fishing, and recreation for local residents). This phenomenon is caused by a lower water depth downstream of the damming than upstream, and the pressures mentioned are greater there (there are better conditions for safe use of the river, e.g., for bathing purposes). In addition, the effect of the hydraulic jump is influencing, causing the formation of a vortex motion, so that directly downstream the damming, more boulders, stones and gravel are visible, carried along with the river current 125.5 mm (38.1).
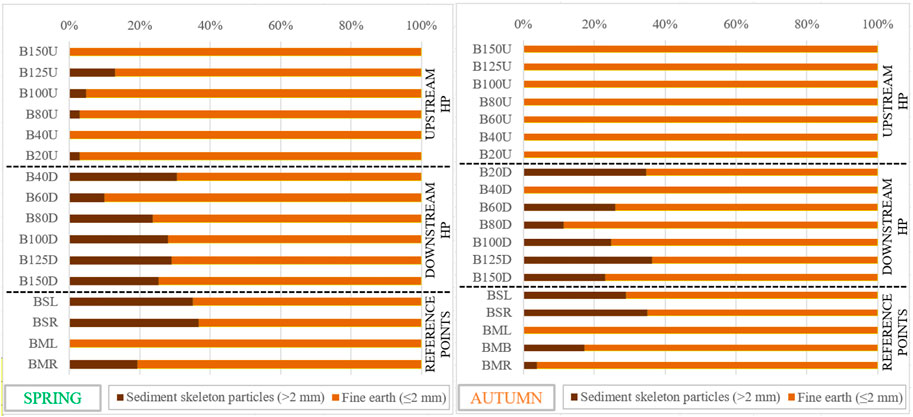
FIGURE 7. The share of skeleton particles and fine Earth fractions in the tested sediment samples from the Bystrzyca River.
pH and Electrical Conductivity
The pH values were lower during autumn than in spring at each point. Additionally, the values are in the following order: reference points > downstream hydropower plants > upstream hydropower plants. The mean values of the reaction were strongly acidic (<5.0; upstream of the hydropower plant and downstream of the hydropower plant in autumn) or acidic (5.0–6.0; downstream of the hydropower plant in spring and at reference points) (Jaggi et al., 2005). The ranges of the 25th to 75th percentile values downstream of the hydropower plant and at the reference points were similar in both seasons, and upstream of the hydropower plant, the amplitude differed markedly (spring: 0.36, autumn: 1.19). Figure 8A presents the described pH dependencies.
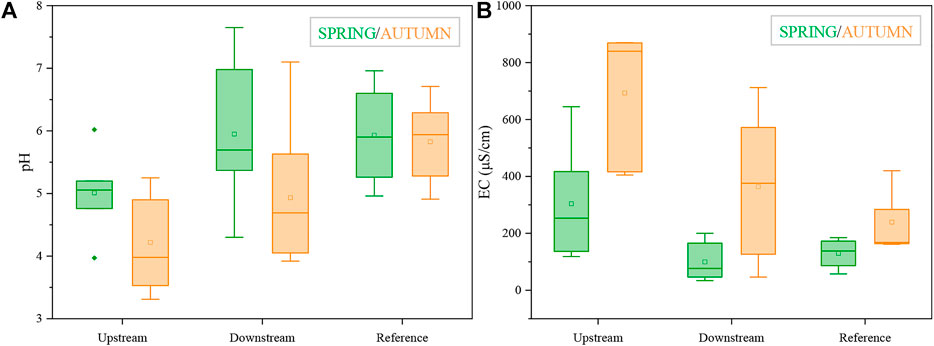
FIGURE 8. (A,B). pH and electrical conductivity (EC) values in the sediment from the Bystrzyca River at the studied measuring points.
After passing the hydropower plant, decreases in conductivity were recorded, while at the reference points, these values were intermediate (spring) or lowest (autumn). In autumn, the conductivity increased compared to that in the spring. This may reflect the greater amount of pollutants accumulated during the growing season (e.g., dead organic matter and debris). The amplitude of the conductivity value was higher in autumn than in spring in each group of points, considering the range from the 25th to 75th percentile (upstream of hydropower plant: 253 μS/cm in spring; 352 μS/cm in autumn; downstream of hydropower plant: 92.5 μS/cm in autumn, 390 μS/cm; reference points: 65.6 μS/cm in spring; 151 μS/cm in autumn). Figure 8B shows the variation in the conductivity across the described point groups.
Metals
Regardless of the season, the average concentration of each of the tested metals decreased downstream of the hydropower plant (Figures 9A–F). At the reference points, the values were indirectly developed. In most cases, higher concentrations of elements were observed during autumn, potentially attributed to the accumulation of various pollutants in the bottom sediment during the growing season. The decrease in the D50 value upstream of the hydropower plant and at the reference points may also be significant. A higher concentration of fine-grained fractions favors the sorption of various impurities (Buyang et al., 2019). In addition, the upstream damming conditions are conducive for the accumulation of large loads of various substances, and water zones with limited flow significantly lower sediment transport capacity are created, in contrast to the zones downstream damming, where the current is rapid and hydraulic bounce occurs (Ali et al., 2019; Tomczyk & Wiatkowski, 2020). Moreover, various studies have proved that mainly sand fractions pass through the damming thresholds, not clay and silt, which show strong sorption capacity (Yao et al., 2015; Bao et al., 2020). In addition, the mechanisms of the properties of metals in sediments are also influenced by the content of organic carbon or geological properties (Zhang et al., 2014; Jokinen et al., 2020).
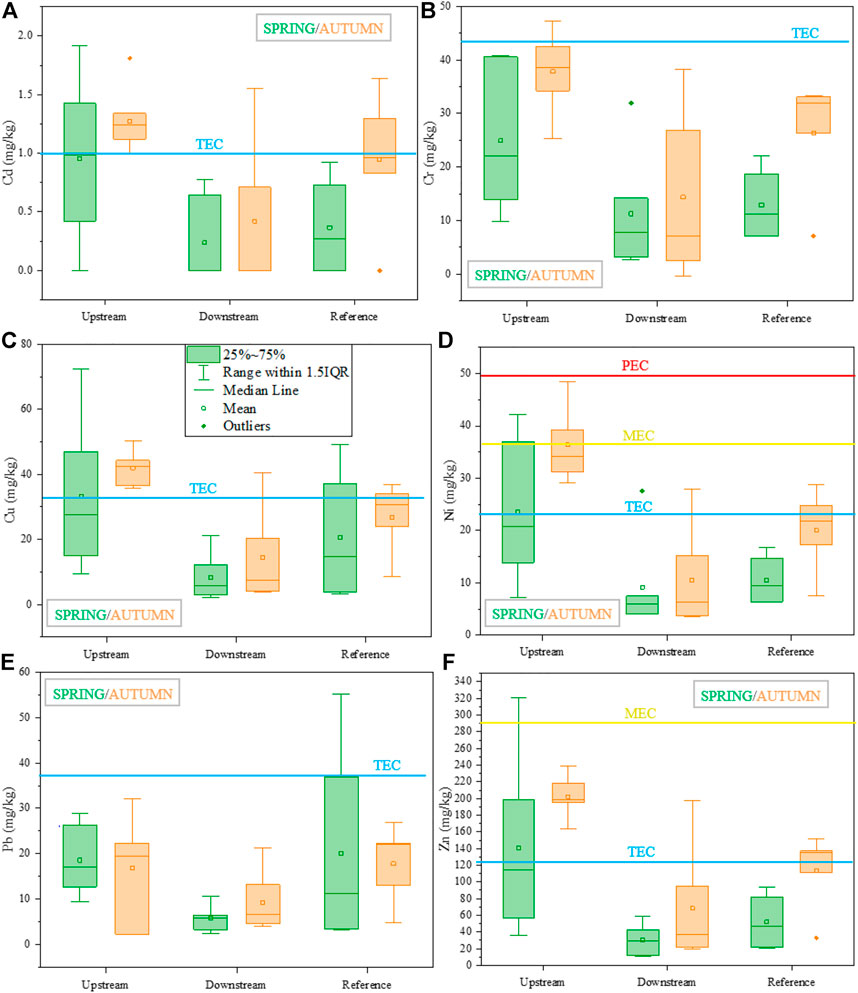
FIGURE 9. (A–F). Cd, Cr, Cu, Ni, Pb and Zn values in the sediment from the Bystrzyca River at the studied measuring points.
Regarding the ecotoxicological classification, most of the sediment samples as compared to the concentration of the metals tested, did not pose a risk to aquatic organisms (class I, values lower than or equal to TEC); an adverse effect on these organisms is possible, but the risk is low (class II; values higher than TEC but less than or equal to MEC). The concentrations of Ni and Zn in some of the samples upstream of the hydropower plant are anomalies, which may have negative consequences for aquatic organisms, and the risk of these consequences is higher (class III) or reaches values close to the toxic (class IV, PEC). Considering the groups of points, the most unfavorable results were recorded upstream of the hydropower plant, especially in autumn (except for Pb). The average metal concentrations were higher during autumn.
Assuming that for classes III and IV, remediation measures should be taken for removing or immobilizing Ni and Zn (Kabała et al., 2020; Zhao et al., 2020). In the first group of methods, pollutants travel from the solid phase to the sediment solution, while in the second group, they are retained in the solid phase (recommended methods) by changing the properties of the sediment (methods not recommended due to the risk to the environment) or a combination of these two methods (Ou et al., 2018; Chen et al., 2020). Considering Ni and Zn, using synthetic zeolites with high sorption capacity (in the form of briquettes that immobilize easily soluble forms of metals accumulated in the sediment) (Ali et al., 2019; Cataldo et al., 2021; Szerement et al., 2021), phytoremediation using plants that accumulate large amounts of such elements in their tissues without a noticeable phytotoxic effect (hyperaccumulators, e.g., energy willow) (Giordani et al., 2005; Sainger et al., 2014; Wu et al., 2021), and electrokinetic methods using the electric field generated by the flow of current, as a result of which the pollutants travel toward the appropriate electrodes on which they precipitate (Saleem et al., 2011; Hawal et al., 2021; Kamal et al., 2021), are recommended.
Changes in Parameter Values Within a Hydropower Plant
The statistical analysis carried out using the Wilcoxon signed-rank test indicates that the results upstream and downstream of the hydropower plant differ significantly for most of the parameters studied. By sorting the obtained U values in ascending order, the following values were obtained: Pb < D50 < Ni < Cr < Cu, Zn < Cd (significance for p < 0.01), and EC (p < 0.05). No statistically significant relationships were found for pH (Table 4).
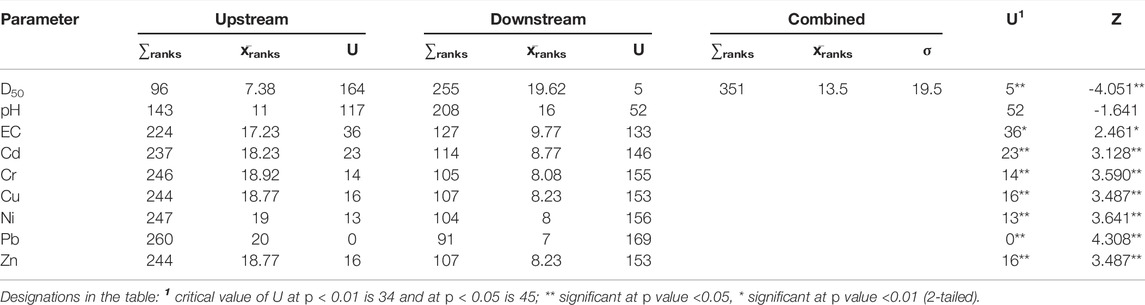
TABLE 4. Summary of the results of the Mann–Whitney U test for the tested parameters between the sections upstream and downstream of the hydropower plant on the Bystrzyca river.
When analyzing the percentage changes in the values between the points located directly upstream and downstream of the hydropower plant, convergent relationships were observed for seven of the nine parameters considered (Figure 10). The biggest changes concern the D50 value; in autumn, downstream of the hydropower plant, values were higher by almost 1,300%, and by over 220% in spring. For Cd, Cr, Cu, Ni, and Zn, there was a decrease in value after passing through a hydropower plant; these changes were lower in the fall than in the spring. The largest changes in concentrations were observed for Ni (approximately 270%) and Zn (approximately 270 and 165%), and the smallest changes were observed for Cd (82 and 66%). Considering Pb, pH, and EC, the same trend was not observed in both seasons of the year; for Pb and EC, the decreases occurred in spring, while for pH, the opposite trend was observed. However, as mentioned earlier, the results for Pb and EC are statistically significant in relation to the results from all consumption points upstream and downstream of the hydropower plant, treated as two groups of dependent variables.
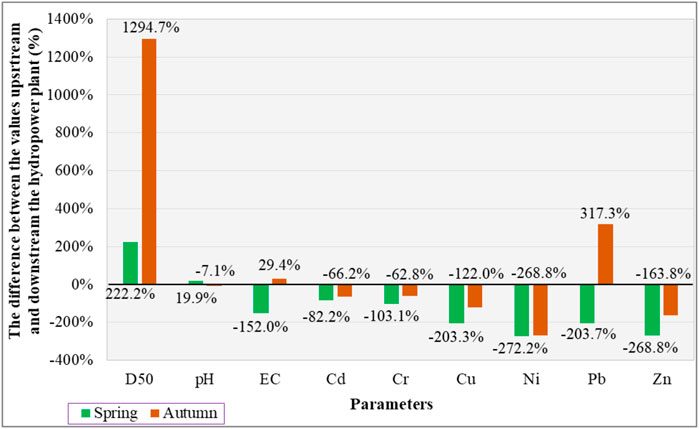
FIGURE 10. Percentage changes in the values of bottom sediment parameters–comparison of the results from the points located closest to the hydropower plant on the Bystrzyca River.
Most of the obtained results were consistent with those of other studies. The accumulation of metals in sediments upstream of dams (also having an energetic function) has been reported, for example, in studies at the Three Gorges Dam on the Yangtze River in China (Zhao et al., 2017), the Geum River dams in South Korea (Shim et al., 2015) or dams on the Sabalan River in Iran (Aradpour et al., 2020).
It was also observed that after passing through a hydropower plant, the texture of the bottom sediment is coarser, belonging to the sand or clay fraction, and upstream, usually to silt [e.g., studies at the Klingenberg dam in Germany (Hahn et al., 2018) or at the Platanovrisi dam] on the Nestos River in Greece (Kamidis and Sylaios, 2017). This is due to the accumulation of large amounts of sediments upstream of hydropower plants, which are a large storehouse of various substances (e.g., it has been estimated that 15% of the world’s river phosphorus load is upstream dams) (Maavara et al., 2015).
The results are different with regards to pH; for example, at the Vaussaire dam on the Rhue River in France and at the hydropower plant on the Ślęza River in Poland, the value for pH increased by an average of 18.9% and by 6.26% downstream of the damming (Fremion et al., 2016; Tomczyk et al., 2021), whereas at the Klingenberg Dam in Germany, no changes were observed (Hahn et al., 2018).
Conclusion
The conclusions from the analysis presented in the article are as follows:
1. Hydropower plants influenced eight of the nine tested parameters. Based on the Wilcoxon signed-rank test, statistical significance was found for the mean grain diameter (D50), Pb, Ni, Cr, Cu, Zn, Cd, and EC. No statistical significance was found for pH.
2. Comparing the points closest and downstream to the hydropower plant, an increase in D50 (on average by 750%), and a decrease in the concentrations of most metals Ni (271%), Zn (216%), Cu (163%), Cr (83.0%), and Cd (74.2%), was observed.
3. The ecotoxicological classification of metal concentrations in sediments indicates, in most cases, little or no impact on aquatic organisms (class I or II). The values of Ni and Zn in some samples upstream of the hydropower plant are anomalies, where the risk of adverse effects on aquatic organisms was higher (class III or IV). It requires remediation with the use of synthetic zeolites, metal accumulating plants (hyperaccumulators), or by the electrokinetic method.
4. Upstream of the hydropower plant, fine-grained fractions dominate clays or sandy clays, while in the downstream, mainly sands are dominated, less often sandy clay loams or sandy clays. In addition, downstream of the hydropower plant, a more frequent occurrence of skeletal fractions (>2.0 mm) was found, i.e., an average of 23.30% in each sample compared to 1.82% upstream of the hydropower plants. This is mainly due to greater anthropogenic pressure, especially from tourism (these fractions often include waste). The dominance of fine-grained fractions upstream of the hydropower plant favors the accumulation of metals due to the high sorption capacity of these fractions.
5. When comparing the analyzed groups of points, the average pH values are arranged in the following sequence (regardless of the season): upstream hydropower plant (U)> downstream hydropower plant (D)> reference points (R), and vice versa, i.e., U < D < R. Lower pH and higher conductivity were observed in each point group during autumn. The mean pH values of the tested samples were strongly acidic or acidic (pH ≤ 6.0).
6. This research agrees with the current issues of the sustainable use of sediments deposited within hydropower plants.
Data Availability Statement
The original contributions presented in the study are included in the article/supplementary material, further inquiries can be directed to the corresponding author.
Author Contributions
Conceptualization, BG, MW, and PT; methodology, BG and PT; software, PT; validation, BG and PT; formal analysis, PT; investigation, BG, ŁG, MW, and PT; resources, BG and PT; data curation, PT; writing—original draft preparation, PT; writing—review and editing, BG and MW; visualization, PT; supervision, BG and MW.
Conflict of Interest
The authors declare that the research was conducted in the absence of any commercial or financial relationships that could be construed as a potential conflict of interest.
Publisher’s Note
All claims expressed in this article are solely those of the authors and do not necessarily represent those of their affiliated organizations, or those of the publisher, the editors and the reviewers. Any product that may be evaluated in this article, or claim that may be made by its manufacturer, is not guaranteed or endorsed by the publisher.
References
Ahamad, M. I., Song, J., Sun, H., Wang, X., Mehmood, M. S., Sajid, M., et al. (2020). Contamination Level, Ecological Risk, and Source Identification of Heavy Metals in the Hyporheic Zone of the Weihe River, China. Ijerph 17 (3), 1070. doi:10.3390/ijerph17031070
Ali, U., Bashir, S., Shaaban, M., Zhou, X., Gao, R., Zhu, J., et al. (2019). Influence of Various Passivators for Nickel Immobilization in Contaminated Soil of China. Environ. Eng. Sci. 36 (11), 1396–1403. doi:10.1089/ees.2019.0120
Alyazichi, Y. M., Jones, B. G., McLean, E., Pease, J., and Brown, H. (2017). Geochemical Assessment of Trace Element Pollution in Surface Sediments from the Georges River, Southern Sydney, Australia. Arch. Environ. Contam. Toxicol. 72, 247–259. doi:10.1007/s00244-016-0343-z
Antoine, G., Camenen, B., Jodeau, M., Némery, J., and Esteves, M. (2020). Downstream Erosion and Deposition Dynamics of Fine Suspended Sediments Due to Dam Flushing. J. Hydrology 585, 124763. doi:10.1016/j.jhydrol.2020.124763
Arabatzis, G., and Myronidis, D. (2011). Contribution of SHP Stations to the Development of an Area and Their Social Acceptance. Renew. Sustain. Energy Rev. 15 (8), 3909–3917. doi:10.1016/j.rser.2011.07.026
Aradpour, S., Noori, R., Tang, Q., Bhattarai, R., Hooshyaripor, F., Hosseinzadeh, M., et al. (2020). Metal Contamination Assessment in Water Column and Surface Sediments of a Warm Monomictic Man-Made Lake: Sabalan Dam Reservoir, Iran. Hydrology Res. 51 (4), 799–814. doi:10.2166/NH.2020.160
Arfania, H., Samadi, A., Asadzadeh, F., Sepehr, E., and Jaisi, D. (2018). Distribution of Phosphorous Pools in Western River Sediments of the Urmia Lake Basin, Iran. Environ. Sci. Pollut. Res. 25, 11614–11625. doi:10.1007/s11356-018-1191-3
Asaeda, T., and Rashid, M. H. (2012). The Impacts of Sediment Released from Dams on Downstream Sediment Bar Vegetation. J. Hydrology 430-431, 25–38. doi:10.1016/j.jhydrol.2012.01.040
Ashraf, F. B., Haghighi, A. T., Riml, J., Alfredsen, K., Koskela, J. J., Kløve, B., et al. (2018). Changes in Short Term River Flow Regulation and Hydropeaking in Nordic Rivers. Sci. Rep. 8, 17232. doi:10.1038/s41598-018-35406-3
Bao, L., Li, X., and Su, J. (2020). Alteration in the Potential of Sediment Phosphorus Release along Series of Rubber Dams in a Typical Urban Landscape River. Sci. Rep. 10 (1), 2–11. doi:10.1038/s41598-020-59493-3
Bhuyan, M. S., Bakar, M. A., Rashed-Un-Nabi, M., Senapathi, V., Chung, S. Y., and Islam, M. S. (2019). Monitoring and Assessment of Heavy Metal Contamination in Surface Water and Sediment of the Old Brahmaputra River, Bangladesh. Appl. Water Sci. 9, 125. doi:10.1007/s13201-019-1004-y
Bieganowski, A., Witkowska-Walczak, B., Gliñski, J., Sokołowska, Z., Sławiński, C., Brzezińska, M., et al. (2013). Database of Polish Arable Mineral Soils: a Review. Int. Agrophysics 27, 335–350. doi:10.2478/intag-2013-0003
Bojko, O., Kabala, C., Wu, Shi, Borselli, L., Cassi, P., and Torri, D. (2017). Organic Carbon Pools in Mountain Soils - Sources of Variability and Predicted Changes in Relation to Climate and Land Use changesProlegomena to Sediment and Flow Connectivity in the Landscape: A GIS and Field Numerical Assessment. CATENACatena 14975 (13), 209268–220277. doi:10.1016/j.catena.2016.09.022He10.1016/j.catena.2008.07.006
Borselli, L., Cassi, P., and Torri, D. (2008). Prolegomena to Sediment and Flow Connectivity in the Landscape: A GIS and Field Numerical Assessment. Catena. 75 (3), 268–277. doi:10.1016/j.catena.2008.07.006
Boulange, J., Hanasaki, N., Yamazaki, D., and Pokhrel, Y. (2021). Role of Dams in Reducing Global Flood Exposure under Climate Change. Nat. Commun. 12, 417. doi:10.1038/s41467-020-20704-0
Buyang, S., Yi, Q., Cui, H., Wan, K., and Zhang, S. (2019). Distribution and Adsorption of Metals on Different Particle Size Fractions of Sediments in a Hydrodynamically Disturbed Canal. Sci. Total Environ. 670, 654–661. doi:10.1016/j.scitotenv.2019.03.276
Capillo, G., Silvestro, S., Sanfilippo, M., Fiorino, E., Giangrosso, G., Ferrantelli, V., et al. (2018). Assessment of Electrolytes and Metals Profile of the Faro Lake (Capo Peloro Lagoon, Sicily, Italy) and Its Impact on Mytilus galloprovincialis. Chem. Biodivers. 15 (5), 1800044. doi:10.1002/cbdv.201800044
Cataldo, E., Salvi, L., Paoli, F., Fucile, M., Masciandaro, G., Manzi, D., et al. (2021). Application of Zeolites in Agriculture and Other Potential Uses: A Review. Agronomy 11 (8). doi:10.3390/agronomy11081547
Chen, X., Kumari, D., Cao, C. J., Plaza, G., and Achal, V. (2020). A Review on Remediation Technologies for Nickel-Contaminated Soil. Hum. Ecol. Risk Assess. Int. J. 26 (3), 571–585. doi:10.1080/10807039.2018.1539639
Cuske, M., Karczewska, A., and Gałka, B. (2017). Speciation of Cu, Zn, and Pb in Soil Solutions Extracted from Strongly Polluted Soils Treated with Organic Materials. Pol. J. Environ. Stud. 26 (2), 567–575. doi:10.15244/pjoes/66710
Dra, A., El Gaidoumi, A., Tanji, K., Benabdallah, A. C., Taleb, A., and Kherbeche, A. (2019). 7496576. doi:10.1155/2019/7496576Characterization and Quantification of Heavy Metals in Oued Sebou SedimentsSci. World J.
Duodu, G. O., Goonetilleke, A., and Ayoko, G. A. (2016). Optimization of In-Cell Accelerated Solvent Extraction Technique for the Determination of Organochlorine Pesticides in River Sediments. Talanta 150, 278–285. doi:10.1016/j.talanta.2015.12.049
European Parliament and the Council (2009). Directive 2009/28/EC of the European Parliament and of the Council of 23 April 2009 on the Promotion of the Use of Energy from Renewable Sources and Amending and Subsequently Repealing Directives 2001/77/EC and 2003/30/EC. Brussels, Belgium: European Parliament and the Council.
Gabbud, C., and Lane, S. N. (2016). Ecosystem Impacts of Alpine Water Intakes for Hydropower: the Challenge of Sediment Management. WIREs Water 3, 41–61. doi:10.1002/wat2.1124
Giordani, C., Cecchi, S., and Zanchi, C. (2005). Phytoremediation of Soil Polluted by Nickel Using Agricultural Crops. Environ. Manag. 36 (5), 675–681. doi:10.1007/s00267-004-0171-1
Hahn, J., Opp, C., Evgrafova, A., Groll, M., Zitzer, N., and Laufenberg, G. (2018). Impacts of Dam Draining on the Mobility of Heavy Metals and Arsenic in Water and Basin Bottom Sediments of Three Studied Dams in Germany. Sci. Total Environ. 640-641, 1072–1081. doi:10.1016/j.scitotenv.2018.05.295
Hauer, C., Leitner, P., Unfer, G., Pulg, U., Habersack, H., and Graf, W. (2018). The Role of Sediment and Sediment Dynamics in the Aquatic Environment. In: Riverine Ecosystem Management Aquatic Ecology Series. Editors S. Schmutz, and J. Sendzimir. Cham: Springer 8. doi:10.1007/978-3-319-73250-3_8
Hawal, L., Al-Zubaidi, L., and Allah, S. (2021). Electro-Kinetic Remediation of Nickel from Contaminated Soil Using Bio Remedies Banana Peels and Surfactant-Enhanced. J. Ecol. Eng. 22 (5), 214–220. doi:10.12911/22998993/135315
He, D., Shi, X., and Wu, D. (2016). Particle-size Distribution Characteristics and Pollution of Heavy Metals in the Surface Sediments of Kuitun River in Xinjiang, China. Environ. Earth Sci. 75, 104. doi:10.1007/s12665-015-4882-9
Jaggi, R. C., Aulakh, M. S., and Sharma, R. (2005). Impacts of Elemental S Applied under Various Temperature and Moisture Regimes on pH and Available P in Acidic, Neutral and Alkaline Soils. Biol. Fertil. Soils 41 (1), 52–58. doi:10.1007/s00374-004-0792-9
Jaskuła, J., Sojka, M., Fiedler, M., and Wróżyński, R. (2021). Analysis of Spatial Variability of River Bottom Sediment Pollution with Heavy Metals and Assessment of Potential Ecological Hazard for the Warta River, Poland. Minerals 11 (3). doi:10.3390/min11030327
Jokinen, S. A., Jilbert, T., Tiihonen-FilppulaKoho, R. K., and Koho, K. (2020). Terrestrial Organic Matter Input Drives Sedimentary Trace Metal Sequestration in a Human-Impacted Boreal Estuary. Sci. Total Environ. 717, 137047. doi:10.1016/j.scitotenv.2020.137047
Kabala, C., Galka, B., and Jezierski, P. (2020). Assessment and Monitoring of Soil and Plant Contamination with Trace Elements Around Europe's Largest Copper Ore Tailings Impoundment. Sci. Total Environ. 738, 139918. doi:10.1016/j.scitotenv.2020.139918
Kamal, A. A., Mahmood, A. K., and Duja, S. (2021). Remediation of Clayey Soil Contaminated with Nickel Nitrate Using Enhanced Electro-Kinetics Process and Study the Geotechnical Properties of the Remediated Soil Samples. Mater. Today Proc. 42, 2516–2520. doi:10.1016/j.matpr.2020.12.572
Kamidis, N., and Sylaios, G. (2017). Impact of River Damming on Sediment Texture and Trace Metals Distribution along the Watershed and the Coastal Zone of Nestos River (NE Greece). Environ. Earth Sci. 76, 373. doi:10.1007/s12665-017-6707-5
Kasperek, R., and Wiatkowski, M. (2014). Hydropower Generation on the Nysa Klodzka River. Ecol. Chem. Eng. S 21 (2), 327–336. doi:10.2478/eces-2014-0025
Katano, I., Negishi, J. N., Minagawa, T., Doi, H., Kawaguchi, Y., and Kayaba, Y. (2021). Effects of Sediment Replenishment on Riverbed Environments and Macroinvertebrate Assemblages Downstream of a Dam. Sci. Rep. 11, 7525. doi:10.1038/s41598-021-86278-z
Kondolf, G. M., Gao, Y., Annandale, G. W., Morris, G. L., Jiang, E., Zhang, J., et al. (2014). Sustainable Sediment Management in Reservoirs and Regulated Rivers: Experiences from Five Continents. Earth's Future 2 (5), 256–280. doi:10.1002/2013EF000184
Kruczkowska, B., Błaszkiewicz, M., Jonczak, J., Uzarowicz, Ł., Moska, P., Brauer, A., et al. (2020). The Late Glacial Pedogenesis Interrupted by Aeolian Activity in Central Poland - Records from the Lake Gościąż Catchment. CATENA 185, 104286. doi:10.1016/j.catena.2019.104286
Kuriqi, A., Pinheiro, A. N., Sordo-Ward, A., Bejarano, M. D., and Garrote, L. (2021). Ecological Impacts of Run-Of-River Hydropower Plants-Current Status and Future Prospects on the Brink of Energy Transition. Renew. Sustain. Energy Rev. 142, 110833. doi:10.1016/j.rser.2021.110833
Li, J., Dong, S., Yang, Z., Peng, M., Liu, S., and Li, X. (2012). Effects of Cascade Hydropower Dams on the Structure and Distribution of Riparian and Upland Vegetation along the Middle-Lower Lancang-Mekong River. For. Ecol. Manag. 284, 251–259. doi:10.1016/j.foreco.2012.07.050
Maavara, T., Parsons, C. T., Ridenour, C., Stojanovic, S., Dürr, H. H., Powley, H. R., et al. (2015). Global Phosphorus Retention by River Damming. Proc. Natl. Acad. Sci. U.S.A. 112 (51), 15603–15608. doi:10.1073/pnas.1511797112
MacDonald, D. D., Ingersoll, C. G., and Berger, T. A. (2000). Development and Evaluation of Consensus-Based Sediment Quality Guidelines for Freshwater Ecosystems. Archives Environ. Contam. Toxicol. 39 (1), 20–31. doi:10.1007/s002440010075
Manoj, K., and Padhy, P. K. (2014). Distribution, Enrichment and Ecological Risk Assessment of Six Elements in Bed Sediments of a Tropical River, Chottanagpur Plateau: a Spatial and Temporal Appraisal. J. Environ. Prot. 5, 1419e1434. doi:10.4236/jep.2014.514136
McKnight, P. E., Najab, J., and Mann-Whitney, U. (2010). Editors I. B. Weiner, and W. E. Craighead. 4th Edn (Hoboken, NJ: Wiley). corpsy0524. doi:10.1002/9780470479216The Corsini Encyclopedia of Psychology
Miao, X., Hao, Y., Liu, H., Xie, Z., Miao, D., and He, X. (2021). Effects of Heavy Metals Speciations in Sediments on Their Bioaccumulation in Wild Fish in Rivers in Liuzhou-A Typical Karst Catchment in Southwest China. Ecotoxicol. Environ. Saf. 214, 112099. doi:10.1016/j.ecoenv.2021.112099
Microsoft Corporation (2013). Office, Version 2013 (Excel 2013, PowerPoint 2013 and Word 2013). Richmond, WA, USA: Microsoft Corporation.
Ndeda, L. A., Manohar, S., and Macharia, G. M. (2017). Accumulation of Heavy Metals on Bed Sediments of Nairobi Dam, Kenya. J. Environ. Chem. Ecotoxicol. 9 (1), 1–7. doi:10.5897/JECE2015.0353
Nistoran, D. E. G., Ionescu, C. S., Braşoveanu, L., et al. (2018). Modeling Hydrodynamic Changes Induced by Run-Of-River Hydropower Plants along the Prahova River in Romania. J. Energy Eng. 144 (2), 04017078. doi:10.1061/(asce)ey.1943-7897.0000515
OriginLab Corporation (2021). Origin Pro. Northampton, MA, USA: OriginLab Corporation. Version 2021b.
Ou, J., Li, H., Yan, Z., Zhou, Y., Bai, L., Zhang, C., et al. (2018). In Situ immobilisation of Toxic Metals in Soil Using Maifan Stone and Illite/smectite Clay. Sci. Rep. 8 (1), 1–9. doi:10.1038/s41598-018-22901-w
Pagano, M., Porcino, C., Briglia, M., Fiorino, E., Vazzana, M., Silvestro, S., et al. (2017). The Influence of Exposure of Cadmium Chloride and Zinc Chloride on Haemolymph and Digestive Gland Cells from Mytilus galloprovincialis. Int. J. Environ. Res. 11 (2), 207–216. doi:10.1007/s41742-017-0020-8
Peng, J.-f., Song, Y.-H., YuanCui, P. X.-Y., Cui, X.-y., and Qiu, G.-l. (2009). The Remediation of Heavy Metals Contaminated Sediment. J. Hazard. Mater. 161 (2-3), 633–640. doi:10.1016/j.jhazmat.2008.04.061
QGIS Development Team (2015). “Open Source Geospatial Foundation Project,” in QGIS Geographic Information System (QGIS Association). Version 2.8.4Availabe at: http://www.qgis.org.
Rajfur, M., Kłos, A., and Wacławek, M. (2011). Algae Utilization in Assessment of the Large Turawa Lake (Poland) Pollution with Heavy Metals. J. Environ. Sci. Health, Part A 46 (12), 1401–1408. doi:10.1080/10934529.2011.606717
Sainger, M., Sharma, A., Bauddh, K., Sainger, P. A., and Singh, R. P. (2014). Remediation of Nickel-Contaminated Soil byBrassica junceaL. Cv. T-59 and Effect of the Metal on Some Metabolic Aspects of the Plant. Bioremediation J. 18 (2), 100–110. doi:10.1080/10889868.2014.888393
Saleem, M., Chakrabarti, M. H., Irfan, M. F., Hajimolana, S. A., Hussain, M. A., Diya’uddeen, B. H., et al. (2011). Electrokinetic Remediation of Nickel from Low Permeability Soil. Int. J. Electrochem. Sci. 6 (9), 4264–4275.
Sarmani, S. B. (1989). The Determination of Heavy Metals in Water, Suspended Materials and Sediments from Langat River, Malaysia. Hydrobiologia 176-177, 233–238. doi:10.1007/BF00026558
Shim, M. J., Yang, Y. M., Oh, D. Y., Lee, S. H., and Yoon, Y. Y. (2015). Spatial Distribution of Heavy Metal Accumulation in the Sediments after Dam Construction. Environ. Monit. Assess. 187 (12), 1–14. doi:10.1007/s10661-015-4967-7
Stanley, D. J., and Clemente, P. L. (2011). Clay Distributions, Grain Sizes, Sediment Thicknesses, and Compaction Rates to Interpret Subsidence in Egypt's Northern Nile Delta. J. Coast. Res. 30 (1), 88–101. doi:10.1130/GSATG312A.1
Szerement, J., Szatanik-Kloc, A., Jarosz, R., Bajda, T., and Mierzwa-Hersztek, M. (2021). Contemporary Applications of Natural and Synthetic Zeolites from Fly Ash in Agriculture and Environmental Protection. J. Clean. Prod. 311, 127461. doi:10.1016/j.jclepro.2021.127461
Tomczyk, P., Gałka, B., Wiatkowski, M., Buta, B., and Gruss, Ł. (2021). Analysis of Spatial Distribution of Sediment Pollutants Accumulated in the Vicinity of a Small Hydropower Plant. Energies 14 (18), 5935. doi:10.3390/en14185935
Tomczyk, P., and Wiatkowski, M. (2021). Impact of a Small Hydropower Plant on Water Quality Dynamics in a Diversion and Natural River Channel. J. Environ. Qual. 50 (5), 1156–1170. doi:10.1002/jeq2.20274
Tomczyk, P., Gałka, B., Wiatkowski, M., Wdowczyk, A., and Gruss, Ł. (2022). Toxicity Studies on Sediments near Hydropower Plants on the Ślęza and Bystrzyca Rivers, Poland, to Establish their Potential for use for Soil Enrichment. Land Degrad. Dev. 33 (5), 756–770. doi:10.1002/ldr.4210
Tomić, T., Mihajlović, V., Jurca, T., Tubić, A., Krčmar, D., Tričković, J., et al. (2019). A Contribution towards Improving the Applicability of the Myriophyllum Aquaticum Sediment Contact Test. Environ. Sci. Eur. 31 (1), 20. doi:10.1186/s12302-019-0200-6
Usman, Q. A., Muhammad, S., Ali, W., Yousaf, S., and Jadoon, I. A. K. (2021). Spatial Distribution and Provenance of Heavy Metal Contamination in the Sediments of the Indus River and its Tributaries, North Pakistan: Evaluation of Pollution and Potential Risks. Environ. Technol. Innovation 21, 101184. doi:10.1016/j.eti.2020.101184
Walling, D. E., and Fang, D. (2003). Recent Trends in the Suspended Sediment Loads of the World’s Rivers. Glob. Planet. Change 39 (1-2), 111–126. doi:10.1016/s0921-8181(03)00020-1
Waroszewski, J., Sprafke, T., Kabała, C., Kobierski, M., Kierczak, J., Musztyfaga, E., et al. (2019). Tracking Textural, Mineralogical and Geochemical Signatures in Soils Developed from Basalt-Derived Materials Covered with Loess Sediments (SW Poland). Geoderma 337, 983–997. doi:10.1016/j.geoderma.2018.11.008
Wdowczyk, A., Szymańska-Pulikowska, A., and Domańska, M. (2022). Analysis of the Bacterial Biocenosis of Activated Sludge Treated with Leachate from Municipal Landfills. Int. J. Environ. Res. Public Health. 19 (3), 1801. doi:10.3390/ijerph19031801
Wiatkowski, M. (2010). Impact of the Small Water Reservoir Psurów on the Quality and Flows of the Prosna River. Archives Environ. Prot. 36 (3), 83–96.
Wiatkowski, M., Rosik-Dulewska, C., and Tomczyk, P. (2017). Hydropower Structures in the Natura 2000 Site on the River Radew: an Analysis in the Context of Sustainable Water Management. Annu. Set Environ. Prot. 19, 65–80.
Wild, T. B., and Loucks, D. P. (2014). Managing Flow, Sediment, and Hydropower Regimes in the Sre Pok, Se San, and Se Kong Rivers of the Mekong Basin. Water Resour. Res. 506, 5141–5157. doi:10.1002/2014wr015457
Winton, R. S., Calamita, E., and Wehrli, B. (2019). Reviews and Syntheses: Dams, Water Quality and Tropical Reservoir Stratification. Biogeosciences 16, 1657–1671. doi:10.5194/bg-16-1657-2019
Wu, B., Peng, H., Sheng, M., Luo, H., Wang, X., Zhang, R., et al. (2021). Evaluation of Phytoremediation Potential of Native Dominant Plants and Spatial Distribution of Heavy Metals in Abandoned Mining Area in Southwest China. Ecotoxicol. Environ. Saf. 220 (24), 112368. doi:10.1016/j.ecoenv.2021.112368
Yao, Q., Wang, X., Jian, H., Chen, H., and Yu, Z. (2015). Characterization of the Particle Size Fraction Associated with Heavy Metals in Suspended Sediments of the Yellow River. Ijerph 12 (6), 6725–6744. doi:10.3390/ijerph120606725
Zhang, C., Yu, Z.-g., Zeng, G.-m., Jiang, M., Yang, Z.-z., Cui, F., et al. (2014). Effects of Sediment Geochemical Properties on Heavy Metal Bioavailability. Environ. Int. 73, 270–281. doi:10.1016/j.envint.2014.08.010
Zhao, R., Li, J., Ma, Y., and Lv, Y. (2020). A Field Study of Vertical Mobility and Relative Bioavailability of Cu and Ni in Calcareous Soil. Environ. Pollut. Bioavailab. 32 (1), 121–130. doi:10.1080/26395940.2020.1813053
Keywords: hydropower plants, environmental impacts, Bystrzyca river, sediment, metals, ecotoxicology, renewable energy sources, sustainable development
Citation: Tomczyk P, Wiatkowski M, Gałka B and Gruss Ł (2022) Assessing the Impact of a Hydropower Plant on Changes in the Properties of the Sediment of the Bystrzyca River in Poland. Front. Environ. Sci. 10:795922. doi: 10.3389/fenvs.2022.795922
Received: 15 October 2021; Accepted: 17 June 2022;
Published: 07 July 2022.
Edited by:
Xander Wang, University of Prince Edward Island, CanadaReviewed by:
Satyanarayan Bramha, Indira Gandhi Centre for Atomic Research (IGCAR), IndiaTatiana Saint Pierre, Pontifical Catholic University of Rio de Janeiro, Brazil
Copyright © 2022 Tomczyk, Wiatkowski, Gałka and Gruss. This is an open-access article distributed under the terms of the Creative Commons Attribution License (CC BY). The use, distribution or reproduction in other forums is permitted, provided the original author(s) and the copyright owner(s) are credited and that the original publication in this journal is cited, in accordance with accepted academic practice. No use, distribution or reproduction is permitted which does not comply with these terms.
*Correspondence: Paweł Tomczyk, pawel.tomczyk@upwr.edu.pl