- 1Department of Geography, University of Bergen, Bergen, Norway
- 2Department of Biological Sciences, University of Bergen, Bergen, Norway
- 3Beja Cultural Studies Center, Red Sea University, Port Sudan, Sudan
- 4Ministry of Agriculture and Natural Resources, Port Sudan, Sudan
The endangered and endemic Nubian dragon blood tree, Dracaena ombet, has been feared extirpated from core distribution areas in the Red Sea Hills, Sudan, after reported mass death events in the 20th century. Populations of dragon tree species are generally reported to be in decline, with a noticeable lack of recruitment and possible poor resilience. Rare recruitment events are, however, normal for species with remnant population dynamics, and when eventually occurring, such events can restore seemingly degraded populations. In response to recently reported observations of dracaena saplings in a historically core distribution area of the Red Sea Hills, we assess the status of this long-lived arboreal species. We describe a current realized niche, investigate a potential range shift by comparing the spatial distribution of saplings and older individuals, and assess population recovery based on pre-disturbance system identity derived from qualitative, historical observations. We document a beginning recovery of the dracaena population in the study area. Around half of the mapped population are individuals in the sapling stage, and they are in good health. Its current realized niche is described by higher altitudes, steeper slopes, more concave landscape forms and east-facing aspects compared to areas where dracaena individuals are absent. However, for the new generation of dracaena saplings we find signs of a leaning range shift where saplings are shifted towards higher altitudes near the mist-influenced escarpment. A full collapse and eventual extirpation of the endangered Dracaena ombet population may at best be averted, or at least delayed in the study area. Our resilience analysis indicates that a full recovery will be a slow process due to the inherent natural climate variability of arid lands, only allowing sporadic regeneration. Considering this species’ information legacy, saplings seem to be well equipped to survive such variability, but perhaps within a restricted safe operating space. Conservation measures should therefore be taken to secure the survival of the new generation along with broader spatial scale studies to confirm whether our findings reflect a regional phenomenon.
Introduction
Abrupt changes in ecosystems resulting in possible population extirpations or species extinctions are of increasing concern in the age of Anthropocene (Ratajczak et al., 2018; IPBES, 2019; IPCC, 2019). Nature is declining globally at rates unprecedented in human history, and the rate at which species are lost is accelerating (IPBES, 2019). Changes are often driven by combined and complex effects of climate, land use and land cover change. Our ability to prevent abrupt changes and mitigate their effects, including to restore ecosystems and populations, depends on knowledge about the underlying mechanisms and dynamics of the system in question. However, such knowledge is often limited. Lack of time series data and historical parallels is further delimiting our ability to respond effectively (Reynolds et al., 2007; Pecl et al., 2017).
Resilience is a central concept for understanding change as it describes how dynamic systems respond to disturbances (Holling, 1973; Folke et al., 2004). Newly proposed bivariate resilience measures distinguish among recovery and resistance (Hodgson et al., 2015; Ingrisch and Bahn, 2018; Gladstone-Gallagher et al., 2019). In the case of abrupt changes, systems have not been able to resist disturbance, and its ability to re-establish the pre-disturbance system state is therefore through recovery.
The ability of a population or species to recover depends on its ecological memory, including its traits and life-history cycle (Johnstone et al., 2016; Gladstone-Gallagher et al., 2019). In drylands longevity is a vital adaptation among drought-enduring species (Andersen and Krzywinski, 2007). Remnant population dynamics, secured by material legacies (e.g., seeds and mature individuals), plays along with the storage effect and make it possible for a seemingly declining population to recover when optimal recruitment events occur (Warner and Chesson, 1985; Eriksson, 1996; Higgins et al., 2000). Old, seed producing trees can therefore “connect” rare occasions of optimal recruitment events and secure their continued existence. For long-lived species, long timeframes could therefore be needed to assess properly the effect of a population collapse and its ability to recover.
To assess recovery, knowledge about the reference point/baseline, or a systems’ identity, is required. Cumming and Peterson (2017) define system identity by key components and relationships that must be maintained for the system to be considered the same system through time and space. In systems with slow dynamics, like drylands, very long time-series are needed to capture inherent system variability, and such data are rare (Reynolds et al., 2007). Many and complex disturbances in the age of Anthropocene makes full recovery to past states uncertain and can result in regime shifts or alternative stable states that can be currently unknown states of the system (Folke et al., 2004; Pecl et al., 2017). Possible effects on species and populations are smaller realized niches or safe operating spaces, resulting in species redistribution and range shifts (Lenoir and Svenning, 2015).
In this paper we investigate recovery after the abrupt change, i.e., the decline, of the endangered Nubian dragon blood tree, Dracaena ombet Heuglin x Kotschy and Peyr. subsp. ombet (hereafter referred to as D. ombet or Tombat; its local name), in a historical core area in the Red Sea Hills (RSH) of Sudan (Figure 1). Tombat was first described by Heuglin (1869) when crossing the RSH towards Suakin in 1864. He was astonished by this rare lifeform and its abundant numbers. Later studies recognized it as a defining species of the mist-influenced vegetation belt in the area (Schweinfurth, 1868; Troll, 1935; Kassas, 1956). Together with Euphorbia abyssinica, D. ombet characterized one zone of this vegetation belt, hereafter referred to as the Dracaena Dominated Vegetation (DDV).
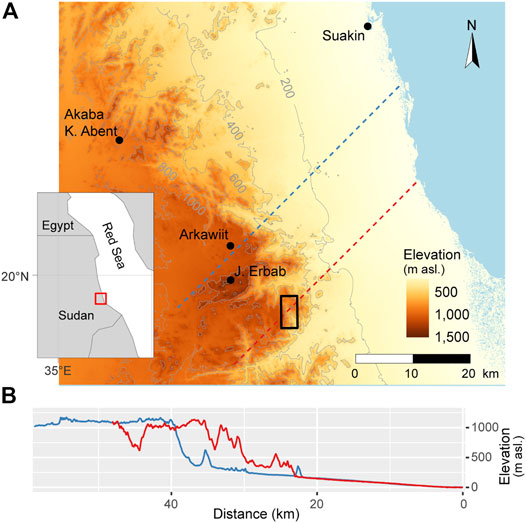
FIGURE 1. (A) Map of study area and inset showing its regional location (red outline). Dashed lines indicate the dominant wind direction, i.e., from NE and crosses Arkawiit (blue) and Baryakay (red). Study area in Baryakay is outlined in black (B) Altitude profiles for the dashed lines in (A) from coastline (to the right) across the coastal plain to the plateau of Arkawiit and Baryakay. Since Tombat is endangered the study site will not be named, nor will maps show coordinates or details that can precisely locate the site investigated. Our own artwork. Topographic information is from SRTMGL1.
Through a series of similar but temporally and geographically distinct events, the large Tombat population was nearly extirpated from the area. The mass death event in Arkawiit1, delimited to a few years in the late 1950 s, is the most well-known of these. During this event Tombat disappeared almost completely (Karkanis, 1958; Kassas, 1960; Bari, 1968). Only one single individual was noted after the collapse and was for a long period thought to be the only surviving specimen in Arkawiit and its surroundings. Later, another nearby population was discovered by one of the authors in the remote area of Baryakay, but here also mass-death events (the latest around 1984) had severely diminished the population. For a long time, recruitment of new individuals was rarely observed in these areas (personal observation; Vetaas, 1993; Vetaas et al., 2012).
Other endemic sister species of D. ombet with native ranges around the Red Sea Basin are also experiencing rapid decline and lack of natural regeneration (Ghazaly, 2008; Habrova et al., 2009; Kamel et al., 2015; Elnoby and Moustafa, 2017). They appear on the IUCN list of threatened species as endangered (D. ombet, D. serrulata) and vulnerable (D. cinnabari) (IUCN, 1998). Hypothesized causes of the decline includes disease caused by fungal leaf spot infection, increased aridity/climate change, human activities, overgrazing, soil erosion and/or a combination of these (Baka and Krzywinski, 1996; Attorre et al., 2007; Ghazaly, 2008; Habrova et al., 2009).
The objective of our study is to assess the current state of the D. ombet population in Baryakay c. 40 years after the last mass-death in the area by:
1) describing its demographic composition, vitality and realized niche
2) investigating possible range shift
3) defining the pre-collapse system identity of the DDV, thereby assessing the recovery of the system.
The Dragon Tree Group and D. ombet
The Dracaena genus belongs to the family Asparagaceae and comprises 120 living species Catalogue of Life (2020). Tombat belongs to the arborescent group of the genus referred to as the dragon tree group (Marrero et al., 1998). In Africa and the Middle East, this group has a disjunct distribution with an eastern group native to the semi-desert regions surrounding the Red Sea and a western group native to Macaronesia and Morocco (Marrero et al., 1998; Lu and Morden, 2014). D. ombet has its native range in Egypt, Sudan, Eritrea, Djibouti, Somalia, Ethiopia and Saudi Arabia. Other members of the eastern group include: D. ombet Heuglin ex Kotschy and Peyr. subsp. schizantha (Baker) Bos (native in Ethiopia and Somalia); Dracaena serrulata Baker (native range in SW. and S. Arabian Peninsula), which consists of three still discussed infraspecifics (Maděra et al., 2020); and D. cinnabari Balf. f. (native range on the island of Socotra, Jemen).
There is only sketchy knowledge about the phylogeny and phylogeography of the dragon tree group. Marrero et al. (1998) hypothesize that the geological events related to the Great Rift in the early Miocene resulted in the fragmentation of the original populations of dragon trees. However, a more recent study based on DNA suggests a common origin on the Arabian peninsula (Lu and Morden, 2014), while Miocene fossil finds from Turkey indicates that a predecessor of D. draco/dragon tree group lived there under rapidly shifting moisture conditions and represent a classic example of pre-adaptation and niche shift (Denk et al., 2014).
The monocotyledonous dragon trees are unique with their secondary stem and root growth and Dracoid habitus, with one main trunk and an umbrella-like canopy consisting of multiple branches with terminal leaf-rosettes. Flowering, apical in leaf rosettes, induces dichotomous branching. Branching periodicity has been used for age estimates (Adolt and Pavlis, 2004), but as time before and interval between flowering remains obscure age estimates vary both within and across species. In general, the dragon tree group is characterized by slow growth and longevity (Maděra et al., 2020).
The typical morphological characters of dragon trees are summarized by Maděra et al. (2020). Dragon trees have succulence in leaves and trunk tissue, and D. ombet is among the most xeric dragon tree species. It typically grows on escarpments and mountain slopes, often sea-facing and under mist influence. It can form forests or woodland vegetation (Bos, 1998). It grows on shallow rocky soil and takes at least part of its water from condensed air moisture that is captured at the basis of the leaf rosette and enters woody organs through the leaf axils (Nadezhdina and Nadezhdin, 2017; Kalivodova et al., 2020).
Materials and Methods
Study Area
The study area of Baryakay is in the wider surroundings of the mist oasis of Arkawiit in the RSH. The RSH mountain chain is a northern extension of the Ethiopian plateau. As a part of the Arabian—Nubian shield it is dominated by a Precambrian crystalline basement complex, overlain by Phanerozoic rocks, including gneiss, basalt, granites, shales, marbles, etc. (Kassas, 1956; Babiker and Gudmundsson, 2004).
In terms of physiography, the study area has a c. 30 km wide coastal desert plain, west of which the RSH mountain chain rises with peaks up to c. 1,500 m asl. To the west the area changes character from mountainous to a gently sloping open landscape towards the river Nile. Arkawiit is located on a mountainous plateau, c. 1,100 m asl, directly east of the escarpment that rises abruptly c. 600 m from the coastal plain. The escarpment is less pronounced both north and south of Arkawiit as there are foothills and chains of increasingly high mountains as one moves inland from the coastal plain towards the mountain plateau (Figure 1).
Climatically, the region is a transition zone between a desert environment to the north and semi-desert to the south. However, the climate of Arkawiit is renowned for its winter mist, hence its characterization as a mist oasis (Troll, 1935; Kassas, 1956). The north-easterly winds brought over the Red Sea become moisture laden and are lifted abruptly along the escarpment. As the air masses are lifted and cooled the moisture condenses and produce clouds, resulting in occasional winter rain showers and dense mist. This is particularly pronounced in winter (weeklong events) and more marked at the higher elevations and closer to the escarpment (op. cit.). The area also receives monsoonal summer precipitation. There is a lack of local meteorological observations, but it has been estimated that the temperature (10 years running mean) in Port Sudan increased steadily by 1.3°C from the 1960 s to 2012 (Vetaas et al., 2012). The average precipitation in Arkawiit is around 200 mm/year (Kassas, 1956; Mahdi, 1991).
This special moisture regime lays the foundation for the unique vegetation with its Abyssinian/Afromontane elements. The c. 10 km moisture gradient, decreasing to the west across the Arkawiit plateau, has created distinct vegetation zones. The abundant growth of D. ombet together with Euphorbia abyssinica was characteristic for one of these zones (Troll, 1935; Kassas, 1956). This will be referred to as the Dracaena-Dominated Vegetation (DDV) and is the pre-collapse system that we aim to define.
Before the British period, Arkawiit was used by pastoral nomadic groups of the Hadandawa tribe that roamed the area with their domestic animals, typically goats and camels. Arkawiit was particularly attractive during summer because of its cooler climate and rich vegetation. A summer resort and game reserve established under the British rule, however, forced local nomads out, and when this rule ceased in 1956 permanent residents in Arkawiit village increased from only a few to 1,000 in the 1990 s and 4,200 in 2008 (Vetaas et al., 2012). The shift towards sedentary land use induced vegetation changes (Vetaas, 1993; Vetaas et al., 2012).
Baryakay is a smaller mountain plateau south of Arkawiit only reachable by foot/camelback and is still primarily inhabited by pastoral groups that move between the coastal plain, intermediate valleys and elevated plateau. The Tombat population in Baryakay was first discovered by the authors in 1988, at a time when scientists believed Tombat was nearly extirpated from the whole area. The Tombat population had experienced recent abrupt declines and many dead Tombat trees were observed in the landscape. Since then the area has been visited by the authors regularly.
Field Work
In response to information from local tribesmen of a significant Tombat recovery in Baryakay, we surveyed (15th—19th March 2019) the eastern parts of a watershed and its surroundings where we have observed D. ombet in the past. For efficient sampling we used local tribesmen as guides and mapped all individuals within visible distance along inspected tracks.
Direct observations, i.e., of individuals growing in or in the immediate vicinity of the track, were accurately positioned and inspected. We recorded life stage, growth form, number of rosettes, signs of browsing and harvesting, leaf spots (fungal infection) and number of inflorescences (Table 1). Each individual was photographed.
Indirect observations, i.e., of individuals observed in the distance, could not be accurately positioned nor inspected. We estimated position by recording the approximate distance and direction from the observation point. We used binoculars to identify these individuals and classify growth form and life stage when possible. The position was estimated in retrospect using distance and direction tools in ArcGIS Pro (ver. 2.4.1).
We used a simple life stage and growth form classification (cf. Table 1) and estimated the height of saplings visually. Our classification of the seedling stage corresponds with the juvenile and partly with the medium juvenile stages of the classification used in studies of D. cinnabari (Maděra et al., 2020). Growth rate of dragon trees is still poorly known, but based on available growth rates (Adolt and Pavlis, 2004; Lengálová et al., 2020; Maděra et al., 2020), we assume saplings can be up to c. 25 years.
Mapping was done with the ArcGIS Collector app installed on a Samsung Galaxy S7 mobile device. Recordings were saved in an associated geodatabase using a coordinate reference system (CRS) with datum WGS84 and web Mercator projection. Routes were tracked (every 2 s) with Garmin handheld GNSS receivers (GPSmap s64 and Oregon 650).
Analyses
The demographic composition and vitality of the Tombat population is described by summary statistics derived from field records. To investigate current realized niche and potential range shifts, we used environmental variables as proxies for moisture conditions since local meteorological data is lacking.
To investigate the realized niche, we compared environmental conditions where dracaena grow (coded present; n = 152) with conditions where it is absent (coded absent; n = 152). Absence points were spread randomly within a 200 m wide buffer zone surrounding the inspected transects. This is the range within which we were confident Tombat individuals were not overlooked. Absence points were above 600 m asl (lowest known record; cf. Results). and outside a 20 m buffer around present observations. This is referred to as the presence-absence (PA) data/analysis (n = 304; Figure 2A).
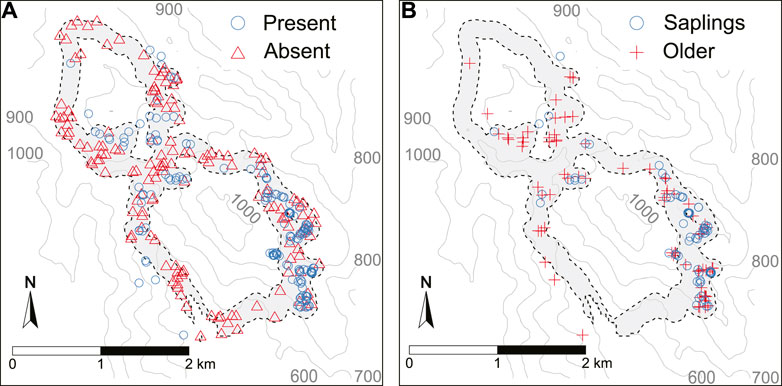
FIGURE 2. (A) Present (blue circles) and Absent (red triangles) Tombat observation points. (B) Saplings (blue circles) and Older (red crosses) Tombat observations. Both datasets are seen in relation to buffered transects (grey polygon) and the topography in the study area. Some secondary observations are outside the buffered transects. Height contours are derived from SRTMGL1.
To investigate range shift, we compared environmental conditions where adult or dead (coded to older) trees are located with conditions where saplings grow. This is referred to as the sapling—older (SO) data/analysis (n = 141; Figure 2B).
Environmental variables describing topographical and hydrological conditions were derived from the 30 m resolution SRTMGL1 Digital Elevation Model (DEM) with CRS WGS84 and unprojected geographical coordinates (NASA JPL, 2013). Appropriate CRS transformation of field observations was done to avoid raster resampling (to CRS of DEM) and to secure planar measurements in case of distance measures (to CRS WGS84 UTM 37N).
Topographic variables were estimated in QGIS ver. 3.4.2 (Grass tool r. slope.aspect; gdal tools topographic position index and terrain ruggedness index) (Wilson et al., 2007; QGIS, 2018). Hydrological variables (flow accumulation and basins) and distances (to coast and water divide) were estimated in ArcGIS Pro (ver. 2.4.1). Values were extracted for all present and absent observations. Aspect was recoded into eight categories and transformed into two linear variables. The multicollinearity of variables were checked by computing variance inflation factors and removed one by one until factors were <10 (James et al., 2013). All variables are summarized in Supplementary Table S1.
The following analyses were run in the statistical programming environment R, ver. 3.6.3 (RCoreTeam, 2020).
To investigate the factors determining the presence of Tombat we studied the probability of finding a Tombat individual on a certain location using a multiple logistic regression on the PA dataset. The outcome (dependent) variable was thus binary (0: Tombat absent; 1 Tombat present). Predictors were the environmental variables (Supplementary Table S1). The logistic regression estimates the odds ratio (OR) of presence. The odds of presence are defined as the probability of presence divided by the probability of absence. The OR (eβ1, where β1 is the slope of the predictor variable) for a continuous variable is the odds for a given predictor value divided by the odds when the predictor is increased by an increment. The interpretation of OR is similar for categorical variables where each level corresponds to a one-unit increase. The reference level for the factorial aspect variable was southwest (SW).
We modelled PA with a generalized linear model (GLM) using package stats v 3.6.1. A best subset (exhaustive) selection procedure (bestglm package) was used to find the optimal model (James et al., 2013; McLeod and Xu, 2020).
It is a standard assumption of logistic regression that measurements are independent. However, due to the spatially explicit character of our sampling strategy we expected spatial autocorrelation. We tested for this in the model residuals over 10 and 100 m lags using ncf package (Bjornstad, 2020). We corrected for spatial autocorrelation by evaluating the optimal model with a 100-repeated 5-fold spatial cross-validation (CV) procedure (package mlr ver. 2.17.1; Bischl et al., 2016; Lovelace et al., 2019). The mean performance measure (AUC) of the 500 fitted models is the model’s overall predictive power. We also fitted the optimal model using a generalized linear mixed model (GLMM), including the spatial correlation structure as a random effect. We tested an exponential, Gaussian and spherical spatial structure (function glmmPQL in MASS ver. 7.3.51.5; Venables and Ripley, 2002; Dormann et al., 2007).
The PA data was also analyzed by fitting a classification tree that was subsequently pruned to find the optimal tree (package tree; Ripley, 2019). We split the data into two equally sized training and test datasets, and the final pruned tree was evaluated based on the test dataset (James et al., 2013).
The SO data was analyzed using a similar procedure, as described above.
A baseline, or a reference point, is needed to assess recovery. However, baselines should not be a snapshot nor set arbitrarily in a variable system. An alternative and more robust approach is to describe the system identity (Cumming and Peterson, 2017 and references therein). Answering the question “What is the system?” helps to specify identity. We used different sources, mainly of qualitative character (Table 2), to find information about the ecological thresholds of the DDV prior to the mass-death events. We derived estimates of pre-disturbance niche and probable tree density (ind/daa). Estimates were clearly imprecise; nevertheless, they established the best possible system identity towards which we can assess recovery.
The spatial distribution of adult and sapling individuals, in relation to the baseline tree density (ind/daa), was used to estimate baseline normalized impact and recovery measures according to Ingrisch and Bahn (2018). We used a 30 m grid as it scales well with the width of buffered transects (200 m). We set the baseline state,
The baseline-normalized impact,
The 2019 state of the system is equivalent to the post-disturbance change of the system,
The rate of baseline-normalized recovery
where dt = 30 years (2019—1989)
Results
Pre-Collapse System Identity
Troll (1935) estimated that the DDV zone in Arkawiit is c. 3 km wide. He remarked that D. ombet has a very restricted “Lebensspielraum” between the driest zone in the southwest and the moist, evergreen vegetation zone in the north-eastern part of the plateau. He hypothesized that the typical DDV has a much wider distribution and surrounds the evergreen vegetation zone, e.g., implying that Tombat also grows to the east of the evergreen zone, at lower altitudes in the escarpment itself. He supported his hypothesis with Schweinfurth’s observations of Tombat from about 600 m asl. in K. Abent. The growth of Tombat in our study area supported this hypothesis.
The illustration from K. Abent in 1868 (Schweinfurth, 1874) is probably a good picture of the DDV zone before the collapse (Figure 3). The predominant species are D. ombet and Euphorbia abyssinica. Kassas (1956) found the same in Arkawiit: D. ombet is present on all 10 hills investigated (100% occurrence) and is either co/dominant or abundant. E. abyssinica has 100% occurrence but is more often abundant than co/dominant.
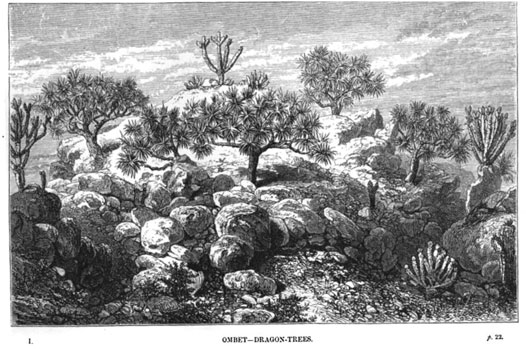
FIGURE 3. The vegetation in the Akaba pass in upper K. Abent was illustrated by Schweinfurth in 1868. Well grown D. ombet trees are seen together with other typical species of this vegetation belt, such as Euphorbia abyssinica and Caralluma acutangula (lower right corner, according to Schweinfurth).
D. ombet predominates on stony and rocky spots (Troll, 1935), in particular on the north-eastern facing slopes of the hills (Kassas, 1956; Schweinfurth, 1868; Heuglin, 1869; Schweinfurth, 1874). The lack of D. ombet on SW-facing slopes is specially remarked in the Akaba pass (Schweinfurth, 1868; Heuglin, 1869; Schweinfurth, 1874) and in Arkawiit (Troll, 1935; Kassas, 1956). Nevertheless, on J. Erbab (c. 1,530 m asl.) just south of Arkawiit, Schweinfurth (1868) noted that D. ombet grows “in countless numbers”2 on the western slopes. He further observed that it grows “to the top”3 of J. Erbab. This is probably the highest recorded observation in the area. On the lower altitudinal range, he observes it around 2000 feet asl (measured with an aneroid barometer) in K. Abent (draining to the coast).
We are not aware that density or coverage has been quantified before the collapse, but according to Schweinfurth (1868, p.8574, own translation): “. . . it [D. ombet] reaches a number of individuals that can be counted in millions in the coastal mountain chain,. . .”. Another passage, describing his first view of Arkawiit, also indicates high densities and forest-like conditions (Schweinfurth, 1868, p.8535, own translation):
However, I had to rejoice when—as approaching—all the thousands of dots with which the valley bottom seemed to be freckled from a distance, dissolved into colossal ombets [D. ombet] and euphorbias, that looked down, arm in arm, bursting with juice and scornfully, on the surrounding sand drought and the wretched vegetating of the lower flora.
A photograph from J. Auliai, Arkawiit (Kassas, 1956, Plate 6) confirms this impression. Local informants also used terms like “forest” and “grow close together” when they described the past DDV, also from locations outside of Arkawiit. Further, they estimated that D. ombet was the most abundant of arboreal species.
Schweinfurth made some notes about the size of Tombat and E. abyssinica individuals. For Tombat, he estimated that “The usual dimensions of the tree are 12–15’ in height, with the crown width alike.” (Schweinfurth, 1868, p. 8556, own translation). Assuming a near circular canopy outline, this corresponds to a canopy size of c. 12—19 m2. For E. abyssinica he observed individuals with up to 100 branches and height of 12–15 feet (4–5 m).
We used the above description of the pre-disturbance system identity to arrive at a density estimate. We assumed an equal mix of D. ombet and E. abbysinica as they often are described as codominant. Based on the “usual dimensions” (cf. above, corresponding to c. 5 m crown diameter) we guesstimated that 5 m spacing between trees (of either species) could reflect dense/forest-like growth. This gave a crude estimate of c. five dracaenas/daa (1,000 m2).
Status and Current Realized Niche
In total, 152 dracaena observations (109 primary) were recorded in the field (Figure 4). Notably, most individuals were young (Figure 4). The majority had one leaf rosette (n = 90), and 67 of these were classified as saplings. Only 11 individuals had 10 or more rosettes. The largest individual, with c. 40 rosettes, grew on the margin of a sandy wadi bed. It was the only Tombat observed with remains of inflorescence stands (n = 3). Dead observations (n = 19) were of fallen and fragmented trunks on the ground, typically of large individuals. A “hut” largely built of dry Tombat trunks indicated that many dead remains were removed from the surroundings.
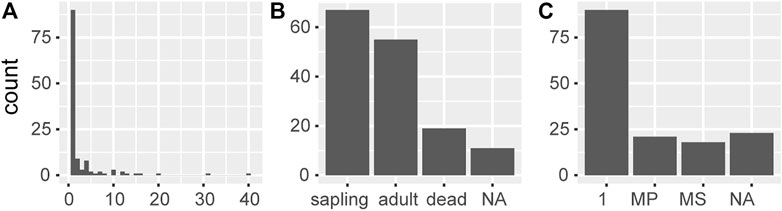
FIGURE 4. Demographics of the population (A) number of rosettes (B) life stage and (C) growth form according to rosettes (MP = multiple primary; MS = multiple secondary). NA denotes missing data.
The recorded individuals were in good health. Fungal leaf spot infections were observed on six individuals (3 saplings), but they had few spots. Only two adult individuals (w/12 rosettes) had some signs of cutting, and another two (1 sapling) had signs of severe browsing. 53 individuals (36 saplings) had smaller marks on leaves, possibly related to browsing.
The current realized niche of Tombat was best described by elevation, slope, planar curvature and factorial aspect (Table 3 and Figure 5). The AUC of the logistic model based on the dataset was 0.78. There was a significant positive autocorrelation in the model residuals, mainly over distances up to 90 m. Spatial cross-validation (CV) gave a test-based AUC of 0.73. When spatial structure was added as a random effect in the logistic regression, the Gaussian spatial structure gave the best model (AUC of 0.78; see Supplementary Table S2).
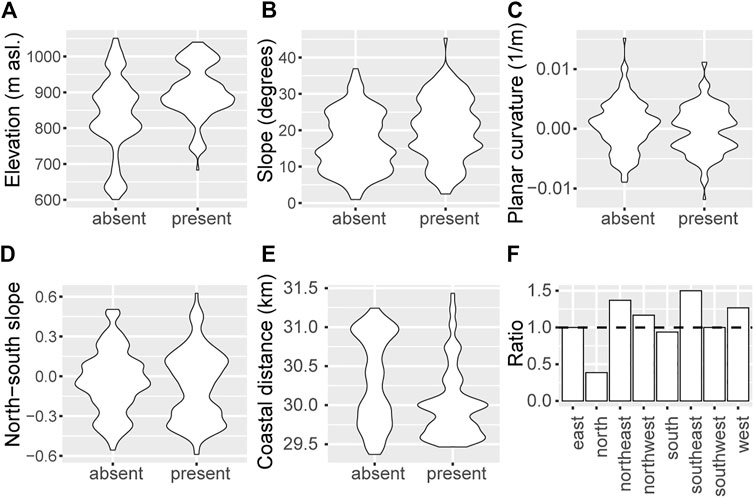
FIGURE 5. Distribution of present and absent observations according to (A) elevation (B) slope (C) planar curvature (D) north south slope and (E) coastal distance (F) Factorial aspect as ratio of present over absent observations within each of the eight cardinal directions. Values above one indicate that there are more present observations than expected at random.
The effects of slope and elevation were positive on the probability of finding Tombat, i.e., if slope increased with 10, it was 2.5 times more likely to find Tombat compared to not finding it; for altitude, a 100 m increase corresponded to a 3 fold increase in the likelihood of finding Tombat. Planar curvature had a negative effect, i.e., if the landscape was characterized by throughs (increment −0.004), there was 60% increased likelihood of finding Tombat. Compared to SW aspects, there was an increased likelihood of finding Tombat at NE (3 times) and SE (6 times) aspects.
When modelling the current realized niche with a pruned classification tree, it was best described by the variables elevation, north-south slope and coastal distance (Supplementary Figure S1). The test-based error rate was 0.29 (n = 152); i.e., 71% of the observations in the test dataset were correctly predicted. Tombat grew mainly at elevations above 850 m asl, and not at flatter slopes. At the steeper, northern facing slopes it grew at further distances from the coast.
Range Shift
Saplings were more likely (21%) to grow at closer distances (every 100 m) to the coast, compared to older life-stages. Coastal distance was the only significant variable in the optimal logistic regression model (Table 4). Coastal distance and elevation were negatively correlated (r = 0.84) in the study area, i.e., the probability of finding saplings compared to older life-stages also increased with increasing altitude in the eastern/coastal facing escarpment. While older life-stages were found most frequently at c. 900 m, saplings had a bimodal distribution with another optimum at c. 1000 m asl. (Figure 6). Overall, there was no spatial autocorrelation in the residuals of the model; however, it occurred at some intervals on a larger scale (some 10 m lags up to 120 m). Hence, we performed both regular and spatial CV (5-fold, 100 repetitions), giving a mean test AUC of 0.702 and 0.608, respectively. The pruned classification tree included the variables coastal distance and planar curvature; however, the predictive power was no better than random (CV test error rate = 0.51).

TABLE 4. Summary of the logistic regression model of range shift. Results are given with 95% confidence intervals (CI) and p-values. OR is the odds ratio of finding a sapling relative to finding an older individual. Increment gives the increment value of predictor values. Variables: coast_dist = coastal distance. AIC = 180.57, n = 141.
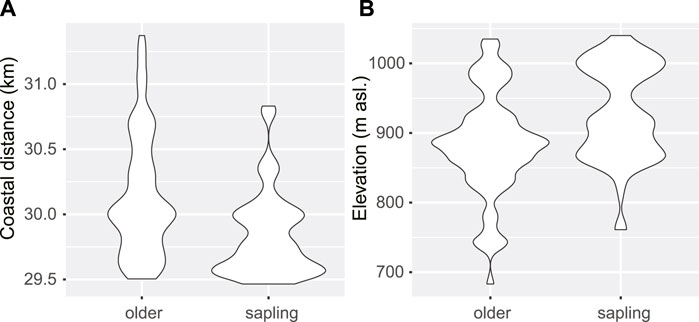
FIGURE 6. Density distribution of older and sapling Tombat observations according to (A) Coastal distance and (B) Elevation. Adjust parameter was set to 0.5 (ggplot2).
Resilience Analysis
In 2019 Tombat grew in 1% (101 cells, i.e., 91 daa) of the investigated area. There was recovery in 55% of the cells and no recovery in the remaining 45%. The spatial pattern indicates that recovery is mainly occurring in the south-eastern part, the steepest and highest part, of the investigated area (Figure 7B).
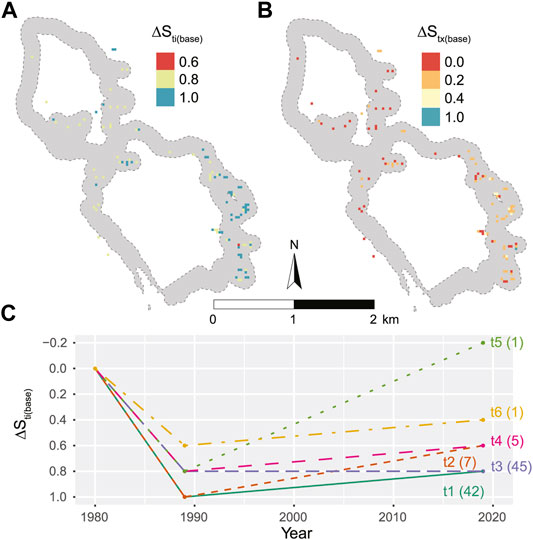
FIGURE 7. (A) baseline normalized impact and (B) post-disturbance recovery on a 30 m grid within the area surveyed (grey) based on a system identity of five dracaena individuals pr 30 m grid cell. In total 101 cells had Tombat individuals in 2019. In (A) cells with 100% impact (
The baseline normalized impact,
Discussion
Observations made by locals suggest that a new generation of Tombat is a rare and remarkable event. For a long time, lack of recruitment has been reported, leading to the presumption that Tombat already was or would extirpate from the area. The lack of successful recruitment events in the past can be due to various factors, alone or in combination. Long periods without enough moisture to break seed dormancy and maintain seedling growth, perhaps related to the Sahelian drought in the 1980 s or other drought events, is a line of reasoning used in the case of Tombat in J. Elba (Ghazaly, 2008; Kamel et al., 2015). Measurements from Socotra show that dewfall/horizontal precipitation caused by dragon trees can be as much as 40% of annual rainfall (Kalivodova et al., 2020). The significant reduction in dragon trees after the mass death event might therefore also have intensified the aridity in our study area. Another explanation is high seedling/sapling mortality. In Socotra high browsing pressure is suggested as a cause of seedling/sapling mortality, since young individuals are observed primarily in places inaccessible to livestock (Attorre et al., 2007; Habrova et al., 2009). Baka and Krzywinski (1996) found that leaves of withering and dead Tombat individuals were attacked by pathogenic fungi and hypothesized that fungal infection in a stressed population can develop into an epidemic attack and cause mass death events after rainy events that spread spores effectively. Whether leaf spot infection has affected seedling/sapling mortality in our area is unknown.
Does the recent recruitment event(s) that we see the results of now, represent a change in factors that are decisive for recruitment? As the general status of saplings were good, it seems unlikely that fungal infection has been or is a threat to the current population. The critical factors for successful recruitment and successive survival, therefore, seem to be moisture conditions and browsing pressure. Previous studies from the area show that traditional tending practices are low impact (Andersen et al., 2014; Hobbs et al., 2014). Impact is further expected to have decreased in the hills over the last decades, since the pastoral population and herd size, and hence browsing pressure, have decreased. We found significant numbers of saplings in areas that were accessible and used actively for herding (goats). Also, observations from Arkawiit indicate a beginning Tombat recovery (Mahmoud, 2004; Mohammed, 2015). Notably, this has happened during the same period as the number of inhabitants have increased and browsing tolerant species has become more abundant (Vetaas et al., 2012). These observations suggest that browsing pressure has not been a critical (negative) factor for the success of the recent recruitment event(s).
Moisture conditions seem therefore to have been optimal for periods over the last decades. In general, recruitment in arid areas typically occurs after pulses of increased moisture, which are rare and variable in both space and time (Noy-Meir, 1973). Few studies of Tombat’s ecology, seed biology and ecophysiology make it difficult to characterize optimal conditions for a recruitment event (Dewir et al., 2019). Moisture in some form is probably needed to break seed dormancy; however, growth and survival in early stages might be independent of rainfall events due to their ability to trap mist and dewfall with subsequent tissue-storage (Nadezhdina and Nadezhdin, 2017; Kalivodova et al., 2020). This is a probable scenario as Tombat distribution is confined to areas with regular mist. We know from historical sources that mist events could last for weeks in Arkawiit (Troll, 1935; Kassas, 1956) and J. Elba (Ball, 1912, p. 23, 24, 226). Hence, more frequent or longer periods of mist and dewfall events can be a possible explanation of the recruitment event(s) observed. Variations in the occurrence of mist and dewfall events can be part of normal variability or a long-term trend. However, which explanation is most likely cannot be concluded without local meteorological time-series observations. On a more regional scale a trend might be supported as observations from Eastern Sahel (east to 10 dgr E) indicate a hydro-climatic intensification since the beginning of the 2000 s (Panthou et al., 2018). Although uncertain, some precipitation projections suggest that more intensive and variable rainfall events are likely in future climate scenarios (Giannini et al., 2013).
We found that Tombat distribution in the study area, independent of life-stage, depends on a set of topographic variables (Table 3). It grows on higher, steeper locations that preferably face the coast (NE, SE). Valley bottoms could also be advantageous, perhaps because mist/moisture can be trapped for longer periods here. Generally, these factors are in line with what is known from previous studies (Troll, 1935; Kassas, 1956).
The sapling distribution is, however, shifted compared to the distribution of older Tombat individuals. This falls within the leaning type of range shift, where abundance within the existing range is shifted towards the leading edge (Lenoir and Svenning, 2015). Saplings were more abundant than older individuals at higher altitudes near the escarpment.
The increased abundance of Tombat saplings at higher altitudes near the escarpment can be related to an increased probability of mist and dewfall, but also rainy events. Mist formation depends on the absolute humidity of the north-easterly air masses, related to evaporation from the Red Sea, and on increased relative humidity due to adiabatic cooling as the air rises at the escarpment. Condensation occurs at and above the altitude where temperature reaches the dewpoint and the air becomes saturated. Upward shift in mist formation may indicate that dewpoint is more frequently reached at a higher altitude, due to lower absolute humidity or due to the higher temperature of rising air. Vetaas et al. (2012) estimate that temperature in Port Sudan increased around 1.3°C in the period 1960 to 2012. If there has been a similar temperature trend in the study area it can have caused an upward shift of the mist-influenced zone of c. 100 m. This is in the same order of magnitude as the difference between the altitudinal optima of adults (c. 900 m asl.) and the shifted optima of saplings (1000 m asl.; Figure 6B).
Our findings strongly suggest a beginning recovery of Tombat. However, they build on observations from the last c. 30 years only. The resilience analyses suggested recovery times up to 150 years, and for the many cells with no saplings it can be longer. Recovery times of more than 150 years probably gives a realistic timeframe for assessing the success of this beginning recovery, but it is still an inaccurate estimate.
Estimated recovery time is inaccurate in arid environments due to the temporal variability of recruitment events. Long timeframes are therefore needed to acquire a representative estimate of recovery time. Further, our estimate is based on a guesstimated pre-collapse Tombat density. Our study area is on the outskirts of the main DDV area, and the ability to derive a local density estimate would be preferable. Nevertheless, our results showed that the density estimate is realistic. A few grid cells had up to six individuals. High densities of Tombat are also reported from J. Elba (i.e., Camerba mountain; Kamel et al., 2015), and D. cinnabari still grow at high densities in some parts of Socotra (i.e., Firmhin; Attorre et al., 2007; Maděra et al., 2019). Utilizing historical, qualitative sources seems to be a valuable approach when better sources for system identity data are lacking.
Recovery time must also be interpreted in relation to expected lifespan. If recovery time is much longer than expected lifespan, population extirpation is likely. Our own and informants’ observations of growth over decades suggest a significant longevity of Tombat trees. This is supported by longevity estimates of Tombat and sister species (Adolt and Pavlis, 2004; Lengálová et al., 2020; Maděra et al., 2020). Up to 150 years of recovery time seems therefore to be of similar/shorter magnitude than the expected lifespan of Tombat. Since few adults are alive, the survival of the new generation and presence of a viable seed bank, i.e., material legacy, is a prerequisite for full recovery. Few adult Tombat individuals remained after the past mass-death events, and therefore it seems likely that the seed bank has played a critical role in the recovery. This is particularly evident for the reported recovery in Arkawiit, where close to no adults survived the late 1950 s mass-death. Preliminary information about the location of the saplings in Arkawiit, i.e., that they grow in the same area as the pre-collapse population, also suggests that presence of a viable soil seed bank is more important than long-range seed dispersal by birds (Gonzalez-Castro et al., 2019). Very high germination rates in other dragon tree species have been reported recently (Bauerova et al., 2020; Kheloufi et al., 2020) and this might indicate that a seed bank is not able to build up. However, Dewir et al. (2019) report that only 20% of D. ombet seeds germinated, but it should be noted that only few seeds were included in their experiment.
We know of no studies of seed bank viability or seed dispersal of Tombat, and this knowledge gap needs to be filled to better understand the mechanisms securing its recovery.
Conclusion
Although rare and endemic species with a restricted distribution are more vulnerable to extinctions, our study indicated a beginning recovery for the Tombat population in Baryakay. Around half of this population was saplings, and they were in good health, in terms of signs of fungi infection, cutting and browsing. The full collapse and eventual extirpation of the endangered Tombat population may therefore at best be averted, or at least delayed.
We also found indications of a range shift with increased abundance of saplings at higher altitudes near the escarpment. This pattern was confirmed by the resilience analysis, and further it suggested that a full recovery will be a slow process. This is due to the inherent natural climate variability of arid lands, which only allow for sporadic regeneration. In fact, this inherent variability, together with remnant population dynamics and the storage effect, seems to have been essential for this system’s resilience. Considering the species’ information legacy having evolved under and adapted to a highly variable moisture regime (Denk et al., 2014), saplings seem to be well equipped to survive such variability, but as our results indicated, perhaps within a restricted safe operating space. Conservation measures should therefore be taken to secure the survival of the new generation, along with building a better knowledge base for Tombat. Broader spatial scale studies are strongly recommended to confirm whether our findings reflect a regional phenomenon.
Data Availability Statement
The raw data supporting the conclusions of this article will be made available by the authors, without undue reservation.
Author Contributions
GA and KK contributed to the study conception and design. Field work was performed by all authors and MT and MM did the required interpretation and translation. GA did the analysis and made all figures. The first draft of the manuscript was written by GA with contributions from KK, and all authors commented on previous versions of the manuscript. All authors read and approved the final manuscript.
Funding
Field work was supported by the grants from “Olaf Grolle Olsens and Miranda Bødtkers legat” (ID 20087 and 20099).
Conflict of Interest
The authors declare that the research was conducted in the absence of any commercial or financial relationships that could be construed as a potential conflict of interest.
Publisher’s Note
All claims expressed in this article are solely those of the authors and do not necessarily represent those of their affiliated organizations, or those of the publisher, the editors and the reviewers. Any product that may be evaluated in this article, or claim that may be made by its manufacturer, is not guaranteed or endorsed by the publisher.
Acknowledgments
We thank employees at Red Sea University and the Beja Cultural Studies Center that helped with logistics and arranged permits. We are grateful to our local guides from the Tirkway tribe as this study would not be possible without their deep environmental knowledge and interest. We thank Kerstin Potthoff for translating German quotes. We also thank two referees for valuable comments that improved the manuscript.
Supplementary Material
The Supplementary Material for this article can be found online at: https://www.frontiersin.org/articles/10.3389/fenvs.2022.793583/full#supplementary-material
Abbreviations
AUC, Area Under Curve; a mean performance measure; CV, Cross Validation; CRS, Coordinate Reference System; DDV, Dracaena Dominated Vegetation; DEM, Digital Elevation Model; GNSS, Global Navigation Satellite System; GLM, Generalized Linear Model; GLMM, Generalized Linear Mixed Model; OR, Odds Ratio; PA, Presence Absence; RSH, Red Sea Hills; SO, Sapling Older.
Footnotes
1Placenames are spelled according to Bidhaawyeet pronunciation. Arkawiit is also spelled Erkowit, Erkwit and Erkauit in sources.
2“in zahlloser Menge”
3“bis zur Spitze”
4“. . . er [D. ombet] in den Bergen der dem Meere benachbarten Kette eine nach Millionen zu berechnende Individuenzahl erreicht,. . . ”.
5“Jauchzen aber musste ich vor Freude, als beim Annähern alle die Tausende von Punkten, mit welchen die weite Thalniederung von Weitem getüpfelt erschien, sich in kolossale Ombet und Euphorbien auflösten, die Arm in Arm, von Saft strotzend und mit Verachtung auf die umgebende Sanddürre und das erbärmliche Vegetiren der niederen Flora herabblickten.”
6“Die gewöhnlichen Dimensionen des Baumes sind 12 bis 15’ Höhe bei gleicher Kronenbreite.”
References
Adolt, R., and Pavlis, J. (2004). Age Structure and Growth of Dracaena Cinnabari Populations on Socotra. Trees - Struct. Funct. 18 (1), 43–53. doi:10.1007/s00468-003-0279-6
Andersen, G. L., and Krzywinski, K. (2007). Longevity and Growth of Acacia Tortilis; Insights from 14C Content and Anatomy of wood. BMC Ecol. 7 (4), 4. doi:10.1186/1472-6785-7-4
Andersen, G. L., Krzywinski, K., Talib, M., Saadallah, A. E. M., Hobbs, J. J., and Pierce, R. H. (2014). Traditional Nomadic Tending of Trees in the Red Sea Hills. J. Arid Environments 106, 36–44. doi:10.1016/j.jaridenv.2014.02.009
Attorre, F., Francesconi, F., Taleb, N., Scholte, P., Saed, A., Alfo, M., et al. (2007). Will Dragonblood Survive the Next Period of Climate Change? Current and Future Potential Distribution of Dracaena Cinnabari (Socotra, Yemen). Biol. Conservation 138 (3-4), 430–439. doi:10.1016/j.biocon.2007.05.009
Babiker, M., and Gudmundsson, A. (2004). The Effects of Dykes and Faults on Groundwater Flow in an Arid Land: the Red Sea Hills, Sudan. J. Hydrol. 297 (1-4), 256–273. doi:10.1016/j.jhydrol.2004.04.018
Baka, Z. A. M., and Krzywinski, K. (1996). Fungi Associated with Leaf Spots of Dracaena Ombet (Kotschy and Peyr). Microbiol. Res. 151 (1), 49–56. doi:10.1016/s0944-5013(96)80055-3
Bari, E. (1968). Sudan Conservation of Vegetation in Africa South of the Sahara. Uppsala: Acta Phytogeographica Suecica.
Bauerová, L., Munie, S. A., Houšková, K., and Habrová, H. (2020). Germination of Dracaena Cinnabari Balf.F. Seeds under Controlled Temperature Conditions. Forests 11 (5), 521. doi:10.3390/f11050521
Bischl, B., Lang, M., Kotthoff, L., Schiffner, J., Richter, J., Studerus, E., et al. (2016). Mlr: Machine Learning in R. J. Machine Learn. Res. 17 (1), 5938. https://www.jmlr.org/papers/volume17/15-066/15-066.pdf.
Bjornstad, O. N. (2020). Spatial Covariance Functions. R package version 1.2-9. Available: https://CRAN.R-project.org/package=ncf.
Bos, J. J. (1998). “Dracaenaceae,” in Flowering Plants· Monocotyledons (Springer), 238–241. doi:10.1007/978-3-662-03533-7_30
Catalogue of Life (2020). Available: http://www.catalogueoflife.org/col/browse/classification/genus/dracaena/fossil/0/match/1 (Accessed 09 17, 2020).
Cumming, G. S., and Peterson, G. D. (2017). Unifying Research on Social-Ecological Resilience and Collapse. Trends Ecol. Evol. 32 (9), 695–713. doi:10.1016/j.tree.2017.06.014
Denk, T., Güner, H. T., and Grimm, G. W. (2014). From Mesic to Arid: Leaf Epidermal Features Suggest Preadaptation in Miocene Dragon Trees (Dracaena). Rev. Palaeobotany Palynology 200, 211–228. doi:10.1016/j.revpalbo.2013.09.009
Dewir, Y. H., Aldubai, A. A., Al-Obeed, R. S., El-Hendawy, S., Seliem, M. K., and Al-Harbi, K. R. (2019). Micropropagation to Conserve the Endangered Gabal Elba Dragon Tree (Dracaena Ombet Heuglin Ex Kotschy & Peyr). horts 54 (1), 162–166. doi:10.21273/hortsci13656-18
Dormann, C., McPherson, J., Bivand, R., Carl, G., Davies, R., Hirzel, A., et al. (2007). Methods to Account for Spatial Autocorrelation in the Analysis of Species Distributional Data: a Review. Ecography 30 (5), 609–628. doi:10.1111/j.2007.0906-7590.05171.x
Elnoby, S., and Moustafa, A. (2017). Impact of Climate Change on the Endangered Nubian Dragon Tree (Dracaena Ombet) in the South Eastern of Egypt. Catrina: Int. J. Environ. Sci. 16 (1), 25–31. doi:10.21608/cat.2017.14256
Eriksson, O. (1996). Regional Dynamics of Plants: A Review of Evidence for Remnant, Source-Sink and Metapopulations. Oikos 77 (2), 248–258. doi:10.2307/3546063
Folke, C., Carpenter, S., Walker, B., Scheffer, M., Elmqvist, T., Gunderson, L., et al. (2004). Regime Shifts, Resilience, and Biodiversity in Ecosystem Management. Annu. Rev. Ecol. Evol. Syst. 35, 557–581. doi:10.1146/annurev.ecolsys.35.021103.105711
Ghazaly, U. (2008). Effect of Climatic Change on the Endangered Dracaena Ombet Tree in the Gabel Elba Protected Area in Egypt. Mountain Forum Bull. VIII (1), 11.
Giannini, A., Salack, S., Lodoun, T., Ali, A., Gaye, A. T., and Ndiaye, O. (2013). A Unifying View of Climate Change in the Sahel Linking Intra-seasonal, Interannual and Longer Time Scales. Environ. Res. Lett. 8 (2), 024010. doi:10.1088/1748-9326/8/2/024010
Gladstone-Gallagher, R. V., Pilditch, C. A., Stephenson, F., and Thrush, S. F. (2019). Linking Traits across Ecological Scales Determines Functional Resilience. Trends Ecol. Evol. 34 (12), 1080–1091. doi:10.1016/j.tree.2019.07.010
González-Castro, A., Pérez-Pérez, D., Romero, J., and Nogales, M. (2019). Unraveling the Seed Dispersal System of an Insular "Ghost" Dragon Tree (Dracaena Draco) in the Wild. Front. Ecol. Evol. 7, 39. doi:10.3389/fevo.2019.00039
Habrova, H., Cermak, Z., and Pavlis, J. (2009). Dragon's Blood Tree - Threatened by Overmaturity, Not by Extinction: Dynamics of a Dracaena Cinnabari woodland in the Mountains of Soqotra. Biol. Conservation 142 (4), 772–778. doi:10.1016/j.biocon.2008.12.022
Heuglin, T. v. (1869). Reise in das Gebiet des Weissen Nil und seiner westlichen Zuflusse i. d. Jahren 1862 - 64. Leipzig und Heidelberg: C. F. Winter'sche Verlagshandlung.
Higgins, S. I., Pickett, S. T. A., and Bond, W. J. (2000). Predicting Extinction Risks for Plants: Environmental Stochasticity Can Save Declining Populations. Trends Ecol. Evol. 15 (12), 516–520. doi:10.1016/s0169-5347(00)01993-5
Hobbs, J. J., Krzywinski, K., Andersen, G. L., Talib, M., Pierce, R. H., and Saadallah, A. E. M. (2014). Acacia Trees on the Cultural Landscapes of the Red Sea Hills. Biodivers Conserv 23 (12), 2923–2943. doi:10.1007/s10531-014-0755-x
Hodgson, D., McDonald, J. L., and Hosken, D. J. (2015). What Do You Mean, 'resilient'? Trends Ecol. Evol. 30 (9), 503–506. doi:10.1016/j.tree.2015.06.010
Holling, C. S. (1973). Resilience and Stability of Ecological Systems. Annu. Rev. Ecol. Syst. 4, 1–23. doi:10.1146/annurev.es.04.110173.000245
Ingrisch, J., and Bahn, M. (2018). Towards a Comparable Quantification of Resilience. Trends Ecol. Evol. 33 (4), 251–259. doi:10.1016/j.tree.2018.01.013
IUCN (1998). Dracaena Ombet the IUCN Red List Of Threatened Species. World Conservation Monitoring Centre. Available: (Accessed February 10, 2020).
IPBES (2019). Global Assessment Report on Biodiversity and Ecosystem Services of the Intergovernmental Science-Policy Platform on Biodiversity and Ecosystem Services. Editors J. S. E. S. Brondizio, S. Díaz, and H. T. Ngo (Bonn, Germany: IPBES secretariat).
IPCC (2019). Climate Change and Land: An IPCC Special Report on Climate Change, Desertification, Land Degradation, Sustainable Land Management, Food Security, and Greenhouse Gas Fluxes in Terrestrial Ecosystems. Editors J. S. P. R. Shukla, E. Calvo Buendia, V. Masson-Delmotte, H.-O. Pörtner, D. C. Roberts, P. Zhaiet al.
James, G., Witten, D., Hastie, T., and Tibshirani, R. (2013). An Introduction to Statistical Learning. New York, NY: Springer.
Johnstone, J. F., Allen, C. D., Franklin, J. F., Frelich, L. E., Harvey, B. J., Higuera, P. E., et al. (2016). Changing Disturbance Regimes, Ecological Memory, and forest Resilience. Front. Ecol. Environ. 14 (7), 369–378. doi:10.1002/fee.1311
Kalivodová, H., Culek, M., Čermák, M., Maděra, P., and Habrová, H. (2020). Potential Importance of Socotra Dragon's Blood Tree Cloud Forests and Woodlands for Capturing Horizontal Precipitation. Rend. Fis. Acc. Lincei 31 (3), 607–621. doi:10.1007/s12210-020-00933-7
Kamel, M., Ghazaly, U. M., and Callmander, M. W. (2015). Conservation Status of the Endangered Nubian Dragon Tree Dracaena Ombet in Gebel Elba National Park, Egypt. Oryx 49 (4), 704–709. doi:10.1017/S0030605313001385
Kassas, M. (1960). Certain Aspects of Landforms on Plant Water Resources. Bull. de la Societe de geographie d'Egypte 33, 45–52.
Kheloufi, A., Boukhecha, M., and Ouachi, A. (2020). Effect of Pre-soaking Substrate and Light Availability on Seed Germination and Seedling Establishment of Dracaena Draco (L.) L., a Threatened Species. Reforesta (9), 20–29. doi:10.21750/refor.9.03.77
Lengálová, K., Kalivodová, H., Habrová, H., Maděra, P., Tesfamariam, B., and Šenfeldr, M. (2020). First Age-Estimation Model for Dracaena Ombet and Dracaena Draco Subsp. Caboverdeana. Forests 11 (3), 264. doi:10.3390/f11030264
Lenoir, J., and Svenning, J.-C. (2015). Climate-related Range Shifts - a Global Multidimensional Synthesis and New Research Directions. Ecography 38 (1), 15–28. doi:10.1111/ecog.00967
Lovelace, R., Nowosad, J., and Muenchow, J. (2019). Geocomputation with R. Boca Raton, London, New York: CRC Press.
Lu, P.-L., and Morden, C. W. (2014). Phylogenetic Relationships Among Dracaenoid Genera (Asparagaceae: Nolinoideae) Inferred from Chloroplast DNA Loci. Syst. Bot. 39 (1), 90–104. doi:10.1600/036364414x678035
Maděra, P., Forrest, A., Hanáček, P., Vahalík, P., Gebauer, R., Plichta, R., et al. (2020). What We Know and what We Do Not Know about Dragon Trees? Forests 11 (2), 236. doi:10.3390/f11020236
Maděra, P., Volařík, D., Patočka, Z., Kalivodová, H., Divín, J., Rejžek, M., et al. (2019). Sustainable Land Use Management Needed to Conserve the Dragon's Blood Tree of Socotra Island, a Vulnerable Endemic Umbrella Species. Sustainability 11 (13), 3557. doi:10.3390/su11133557
Mahdi, A. (1991). The Climate of the Red Sea Region of the Sudan: an Outline. RESAP Tech. Paper 1, 1–19.
Mahmoud, M. A. (2004). Feasibility Study Report on Conservation of the Dracaena Ombet, the Red Sea Hills, Sudan. Oxford, UK).
Marrero, A., Almeida, R. S., and Gonzalez-Martin, M. (1998). A New Species of the Wild Dragon tree,Dracaena(Dracaenaceae) from Gran Canaria and its Taxonomic and Biogeographic Implications. Bot. J. Linn. Soc. 128 (3), 291–314. doi:10.1006/bojl.1998.0193
McLeod, A., and Xu, C. (2020). Bestglm: Best Subset GLM and Regression Utilities R Package. Available at: https://CRAN.R-project.org/package=bestglm.
Mohammed, U. (2015). Dracaena Ombet Status Survey in Erkowit Mountains in East Sudan. Dragon Tree Land Project Report, 21. Available at: https://www.mdpi.com/1999-4907/11/2/236/htm#B59-forests-11-00236.
Nadezhdina, N., and Nadezhdin, V. (2017). Are Dracaena Nebulophytes Able to Drink Atmospheric Water? Environ. Exp. Bot. 139, 57–66. doi:10.1016/j.envexpbot.2017.04.005
NASA JPL (2013). "NASA Shuttle Radar Topography Mission Global 1 Arc Second. NASA EOSDIS Land Processes DAAC. Available at: https://doi.org/10.5067/MEaSUREs/SRTM/SRTMGL1.003. (Accessed 30 June, 2020).
Noy-Meir, I. (1973). Desert Ecosystems: Environment and Producers. Annu. Rev. Ecol. Syst. 4, 25–51. doi:10.1146/annurev.es.04.110173.000325
Panthou, G., Lebel, T., Vischel, T., Quantin, G., Sane, Y., Ba, A., et al. (2018). Rainfall Intensification in Tropical Semi-arid Regions: The Sahelian Case. Environ. Res. Lett. 13 (6), 064013. doi:10.1088/1748-9326/aac334
Pecl, G. T., Araújo, M. B., Bell, J. D., Blanchard, J., Bonebrake, T. C., Chen, I. C., et al. (2017). Biodiversity Redistribution under Climate Change: Impacts on Ecosystems and Human Well-Being. Science 355 (6332). doi:10.1126/science.aai9214
Plants of the world online (2020). Kew Science. Available: http://powo.science.kew.org/taxon/urn:lsid:ipni.org:names:534308-1 (Accessed 04 17, 2020).
QGIS (Version 3.4.2) (2018). QGIS Geographic Information System. Open Source Geospatial Foundation Project. Available at: http://qgis.org.
Ratajczak, Z., Carpenter, S. R., Ives, A. R., Kucharik, C. J., Ramiadantsoa, T., Stegner, M. A., et al. (2018). Abrupt Change in Ecological Systems: Inference and Diagnosis. Trends Ecol. Evol. 33 (7), 513–526. doi:10.1016/j.tree.2018.04.013
RCoreTeam, (2020). R: A Language and Environment for Statistical Computing. 3 ed., 3.6. Vienna, Austria: R Foundation for Statistical Computing.
Reynolds, J. F., Smith, D. M. S., Lambin, E. F., Turner, B. L., Mortimore, M., Batterbury, S. P. J., et al. (2007). Global Desertification: Building a Science for Dryland Development. Science 316 (5826), 847–851. doi:10.1126/science.1131634
Ripley, B. (2019). Tree: Classification and Regression Trees. R package version 1.0-40. Availabel at: https://CRAN.R-project.org/package=tree.
Schweinfurth, G. (1874). The Heart of Africa, Travels and Adventures from 1868 to 1871. London: Sampson Low, Marston, Low, and Searle. Translated by Ellen E. Frewer.
Schweinfurth, G. (1868). Vegetationsskizzen aus dem südnubischen Küstengebirge. Botanische Zeitung XXVI (50), 849–860.
Svenning, J.-C., and Sandel, B. (2013). Disequilibrium Vegetation Dynamics under Future Climate Change. Am. J. Bot. 100 (7), 1266–1286. doi:10.3732/ajb.1200469
Troll, V. (1935). Wüstensteppen und Nebeloasen im südnubischen Küstengebirge. Studien zur Vegetations- und Landschaftskunde der Tropen I. Z. der Gesellschaft für Erdkunde zu Berlin 7 (8), 241–281.
Vetaas, O. R., Salih, E. A., and Jurasinski, G. (2012). Vegetation Changes in the Red Sea Hills: from Mist Oasis to Arid Shrub. Plant Ecol. Divers. 5 (4), 527–539. doi:10.1080/17550874.2012.749954
Vetaas, O. R. (1993). Spatial and Temporal Vegetation Changes along A Moisture Gradient in Northeastern Sudan. Biotropica 25 (2), 164–175. doi:10.2307/2389180
Warner, R. R., and Chesson, P. L. (1985). Coexistence Mediated by Recruitment Fluctuations: A Field Guide to the Storage Effect. The Am. Naturalist 125 (6), 769–787. doi:10.1086/284379
Keywords: nubian dragon blood tree, resilience, recovery, leaning range shift, abrupt change, IUCN red list, GIS, mist influence
Citation: Andersen GL, Krzywinski K, Talib M and Musa MAM (2022) Endangered Dracaena ombet Population in the Red Sea Hills, Sudan, Recovers After Abrupt Change. Front. Environ. Sci. 10:793583. doi: 10.3389/fenvs.2022.793583
Received: 12 October 2021; Accepted: 07 March 2022;
Published: 31 March 2022.
Edited by:
Agnes Begue, Centre de Coopération Internationale en Recherche Agronomique pour le Développement (CIRAD), FranceReviewed by:
Jennifer Lesley Silcock, The University of Queensland, AustraliaPetr Maděra, Mendel University in Brno, Czechia
Copyright © 2022 Andersen, Krzywinski, Talib and Musa. This is an open-access article distributed under the terms of the Creative Commons Attribution License (CC BY). The use, distribution or reproduction in other forums is permitted, provided the original author(s) and the copyright owner(s) are credited and that the original publication in this journal is cited, in accordance with accepted academic practice. No use, distribution or reproduction is permitted which does not comply with these terms.
*Correspondence: Gidske L. Andersen, Gidske.Andersen@uib.no