- 1Center for Watershed Sciences, University of California, Davis, Davis, CA, United States
- 2Department of Sustainable and Civil Technologies, Tecnológico de Monterrey, Monterrey, Mexico
- 3School of Engineering, University of California, Merced, Merced, CA, United States
Environmental flow management in watersheds with multi-objective reservoirs is often presented as an additional constraint to an already strained and over-allocated stream system. Nevertheless, environmental flow legislation and regulatory policies are increasingly being developed and implemented globally. In California, USA, recent legislative and regulatory policies place environmental flows at the forefront of the state’s water management objectives; however, the increased reliance on hydropower to support climate change mitigation goals may complicate efforts on both issues. This study modelled alternative environmental flow strategies in the major tributaries to the San Joaquin River in California. Strategies included detailed water management rules for hydropower production, flood control, and water deliveries, and three methodological approaches to environmental flow releases: minimum instream flows (“baseline”) year-round, 40% of full natural flow (FNF) during the spring runoff season and minimum releases the remainder of the year, and functional flows year-round. Results show that environmental flow strategies affect downstream flow releases in each of the San Joaquin’s four sub-basins differently depending on infrastructure capacity, water management objectives, and hydrologic year types. While hydropower production was comparable or declined in the Stanislaus, Tuolumne, and Merced basins, functional flow and 40% FNF strategies increased hydropower production in the Upper San Joaquin basin by 11%. Uncontrolled spill of high flow events decreased when high flow releases were based on hydrologic cues rather than exclusively on flood storage capacity. Water deliveries were reduced in all years regardless of environmental flow strategy. The 40% FNF and functional flow strategies both increased water released to the river relative to baseline, but in different ways. The functional flow strategy allocated water in a holistic approach that enhanced ecological functions in all years, but particularly in moderate and wet years. In contrast, the 40% FNF strategy provided increased flows relative to baseline and some ecological benefit in dry years, but less ecological benefit in other years. This study shows that alternative environmental flow strategies will have different and important trade-offs for integrated water management, and may mutually benefit seemingly conflicting objectives.
1 Introduction
As climate change mitigation is increasingly prioritized in nations around the globe, hydropower occupies a paradoxical space where it is touted as a renewable energy resource (Hamududu and Killingtveit, 2017) while simultaneously being responsible for extreme degradation of river environments (Frey and Linke, 2002; Richter and Thomas, 2007). Globally, hydropower provides ∼17% of the world’s energy demands (IHA, 2020), with the majority produced by regulation-based facilities (Deyou et al., 2019). Planned hydropower projects are projected to reduce Earth’s free-flowing rivers by 21%, with the greatest development in Southeast Asia, South America, Africa, and the Balkans, Anatolia, and Caucasus regions (Zarfl et al., 2015; Couto and Olden, 2018). These projects will further exacerbate humans’ replumbing of the Earth’s hydrologic cycle. While semi-arid and arid regions show the greatest influence of human water management on the hydrologic cycle, hydroelectric reservoir operations strongly influence surface water hydrology—even in areas where natural water bodies are abundant and water scarcity is rare (Cooley et al., 2021).
At the same time that hydropower development has boomed, environmental flow research steadily investigated the relationship between stream flow, ecology, and water management (Tharme, 2003; Palmer et al., 2008; Horne et al., 2017). The negative impacts of stored and diverted water on ecosystems are well understood (Poff and Zimmerman, 2010); but understanding the aspects of flow quantity, quality, place, and timing that are needed to restore and sustain native aquatic ecosystems has been a key focus of research studies for decades (Poff et al., 1997; Arthington, 2012; Poff, 2018). The natural flow regime (Poff et al., 1997) characterizes the dynamic and nuanced characteristics of stream flow that integrate to sustain ecosystems, acknowledgement of which has led to the development of environmental flow approaches that recognize the importance of natural flow variability and related ecological responses (Poff et al., 2010; Richter et al., 2012). More recent research has increasingly recognized the role of physical and biogeochemical factors in mediating the relationship between flow and ecology (Beechie et al., 2010; Wohl et al., 2015; Yarnell et al., 2015), and resource managers have advocated for holistic environmental flow assessment methods designed to support the physical, chemical, and biological functions of streams that sustain ecosystem health (Poff and Matthews, 2013; Palmer and Ruhi, 2019; Tickner et al., 2020). Yet, how to translate these holistic methods into an environmental flow strategy that includes water extraction for human uses via dam regulation remains elusive. Indeed, while hydrologically based environmental flow approaches are common in water management analyses given their relative simplicity, some have been criticized for insufficiently connecting flow patterns with broader ecological functions (Tharme, 2003).
In the western United States, and California in particular, adapting water management to climate change and environmental flow requirements challenges the stability of hydropower production on multiple fronts (Tarroja et al., 2016; Voisin et al., 2016; Voisin et al., 2018). Because hydropower operations are set through a long-term and poorly adapted licensing process through the Federal Energy Regulatory Commission (FERC; see Table 1 for a list of acronyms), they are vulnerable to climate change (Viers and Nover, 2018). Climate change has already altered hydrologic patterns, shifting snow to rain and thus the timing and variability of runoff (Hidalgo et al., 2009); these changes will increasingly strain hydropower operations that are designed for a stationary hydrology (Viers, 2011) and are a major part of California’s climate change mitigation strategy (Ziaja, 2019). Further, recent shifts towards the prioritization of environmental flows present a similar and more immediate challenge to hydropower operations, as environmental flow strategies may alter patterns of flow releases from those typically associated with storage-prioritized water management, potentially disrupting the timing and quantity of water available for hydropower production.
Previous studies have explored environmental flows and hydropower primarily through optimization frameworks (Rheinheimer et al., 2013; Porse et al., 2015; Adams et al., 2017; Zamani Sabzi et al., 2019). These studies were designed under the assumption that the ultimate objective is to maximize value across all water uses. In other words, human water uses may be equal to, or prioritized above, environmental uses. This framing perpetuates the false dichotomy of human communities vs. ecological function (Corral-Verdugo et al., 2008). Few studies frame ecological function as integral to human sustainability, despite recent calls to do so (Reid et al., 2019; Tickner et al., 2020). However, quantifying the ecological benefits of alternative environmental flow strategies and their effects on hydropower production has been challenging (Widén et al., 2022). Other studies have explored the nexus of water management, hydropower production, and ecosystem function, but mainly focused on small hydropower plants (e.g., run-of-the-river facilities) rather than larger, multi-objective reservoirs (Kuriqi et al., 2020, 2021). Thus, understanding the effects of prioritizing environmental flows above remaining uses that may conflict, such as hydropower or water deliveries, is often unknown.
Here, we explored alternative environmental flow strategies to quantify the effects of prioritized ecological flows on hydropower, water supply, and flood control at four multi-objective reservoirs—each of which is considered paramount to the stability of a multi-basin region and influence the amount of stored water available for non-dispatchable hydropower production. The objectives of this study are 1) identify differences between environmental flow alternatives, including how those differences may affect ecological outcomes; 2) quantify the hydropower production, water supply delivery, or flood control trade-offs in the context of those alternatives, and 3) discuss the implications for environmental flow policy and management in the context of a multi-basin, multi-objective setting. We use the multi-basin San Joaquin watershed as a case study as it is considered a promising region in California for integrated water management actions (Georgakakos et al., 2018), as well as the focus of recent environmental flow policies (SWRCB, 2018; CEFWG, 2020).
Water allocations in the San Joaquin basin are directly influenced by how each user manages shared infrastructure. Historical water management activities have had an adverse impact on river ecosystems, leaving native fish populations (most notably anadromous salmon Oncorhynchus spp.) in a precarious state (Moyle et al., 2017). The continuous decline of salmon populations over the past several decades demonstrate that the existing minimum flows do not achieve their goal of maintaining the health and integrity of ecosystems (Katz et al., 2013). The 2006 San Joaquin River Restoration Settlement and the subsequent San Joaquin River Restoration Settlement Act of 2009 required an improved understanding of the linkages between instream flows, fish population requirements, and competing water demands by people. Subsequently, there has been renewed interest in improving environmental flows, and a recent State Water Resources Control Board’s (“State Board”) plan aims to maintain 30–50% of the unimpaired flows in the San Joaquin River and its tributaries for ecological purposes (SWRCB 2018; CEFWG 2020). At the same time, the State Board and other resource agencies have supported the development of the California Environmental Flows Framework that provides guidance to resource managers on how to determine environmental flows using a “functional flows” approach (Stein et al., 2022), where functional flows are those distinct aspects of a natural flow regime that sustain ecological, geomorphic, or biogeochemical functions (Yarnell et al., 2015). The water-energy-environmental policy nexus of this multi-basin watershed, and the proposed environmental flow policies, makes the San Joaquin an ideal candidate with which to explore alternative flow strategies and their effects on a wide range of objectives.
The novelty of the modeling approach lies in the development of reservoir operations to support ecosystem function based on observed hydrological conditions while simultaneously generating hydropower in the context of multi-objective operations. The results of this study help identify the vulnerabilities and opportunities of hydropower in California’s climate and energy portfolio, particularly in relation to the need to support sustainable river ecosystems, and can be applied to any region where multiple, independently managed basins support a common freshwater ecosystem.
2 Methods
2.1 Study Area
This study includes the four major basins in the Central Sierra Nevada, California, that contribute the bulk of the flow to the San Joaquin River, one of two major rivers that flow through the Central Valley into the Sacramento-San Joaquin River Delta. From north to south, these basins include the Stanislaus, Tuolumne, Merced, and Upper San Joaquin (Figure 1). Water infrastructure includes highly regulated networks of high-altitude reservoirs and hydropower facilities and low-altitude, multi-purpose “rim” reservoirs, whose dams regulate the flow entering California’s Central Valley. Along with flood control, the rim reservoirs store water for recreation, urban, and agricultural demands from downstream communities, environmental quality, and hydropower production. This infrastructure is operated by several utility companies.
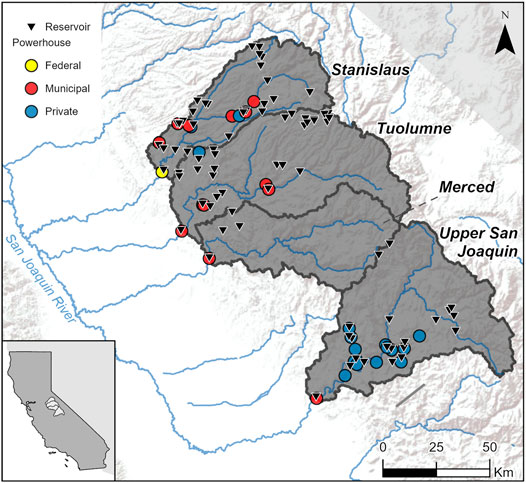
FIGURE 1. Map of the study area including the four river basins: Stanislaus, Tuolumne, Merced, and Upper San Joaquin rivers. Grey areas show the watershed upstream of each rim dam.
The San Joaquin River system supports one of the most agriculturally productive regions in the country, provides water to more than 4.5 million people, and has a capacity to generate 3,000 megawatts (MW) of hydropower. The region represents highly diversified reservoir and hydropower facility operations. Reservoir capacities range from less than 123 million cubic meters (mcm) to 3,083 mcm. Hydropower plants range in capacity from less than 5 MW to over 500 MW, and produce roughly 25% of California’s hydropower. There are more than 11 utilities and energy organizations that manage the hydropower facilities in the region. The four rim dams, whose operations are modelled in this study, are New Melones (reservoir capacity = 3,083 mcm) in the Stanislaus, Don Pedro (2,503 mcm) in the Tuolumne, New Exchequer (1,295 mcm) in the Merced, and Friant (642 mcm) in the Upper San Joaquin.
2.2 CenSierraPywr Model
Water management operations were simulated in the four study basins using CenSierraPywr, a daily water allocation model combined with a monthly planning model with limited hydrologic foresight (Rheinheimer et al., 2021). In general, CenSierraPywr allocates water within the system each day, with water allocations determined by a simulation-style linear programming algorithm developed with Pywr (Tomlinson et al., 2020). Water allocations are driven by a combination of constraints on operations and water costs, with a goal of minimizing costs each time step. All operations are defined via either rules (e.g., flood control operations) or numerical input (e.g., canal capacity), with discretionary hydropower releases informed by the monthly planning model. The strength of this framework lies in the flexibility and extensibility of Pywr. CenSierraPywr incorporates inflows from a Variable Infiltration Capacity (VIC) hydrologic model, wholesale energy prices from an energy model (Holistic Grid Resources Integration and Deployment or HiGRID; Eichman et al., 2013), and instream flow requirements from FERC licenses and other regulatory agreements as “realistic constraints” (Ziaja, 2019).
The CenSierraPywr modeling framework was developed independently for each of the four basins and run for the historical period 1950 to 2013. The water system schematics were initially derived from the suite of WEAP models developed in previous efforts (Rheinheimer et al., 2014). The original schematic was updated, corrected, and extended to include rim reservoirs and downstream dependent agricultural water users (irrigation districts and the Central Valley Project), as well as downstream hydropower and instream flow requirements. Of the four basins, two include optimization as part of their hydropower production: the Stanislaus and Upper San Joaquin optimize hydropower as part of monthly planning forecasts and daily production. Hydropower optimization occurs after environmental flow releases have been satisfied.
For hydrology inputs, the model requires unimpaired runoff at the sub-basin level. For this study, we used the VIC daily gridded (1/16°) runoff data developed by Livneh et al. (2015) [hereafter referred to as Full Natural Flow (FNF)], who used the VIC hydrologic model (Liang et al., 1994), forced with observed meteorological data from NOAA/OAR/ESRL PSD, Boulder, Colorado, United States. A version of the FNF dataset clipped to California and Nevada, as developed for the project to model managed flow for Sacramento/San Joaquin basins (Knowles and Cronkite-Ratcliff, 2018) and hosted by a UC Berkeley server, was used in this study. Full natural flow was bias-corrected for this study at a sub-basin level using historical stream gauge data.
2.3 Environmental Flow Strategies
Three environmental flow strategies—the baseline instream flow strategy and two alternative strategies—were implemented to quantify their effects on hydropower, flood control, and water deliveries. The baseline minimum instream flow strategy (“baseline”) was defined for each sub-basin based on existing policies and specified releases for minimum and maximum instream flows, ramping rates, flushing flows, and other supplemental flows (Supplementary Table S1). Specific flows depended on a variety of factors, including timing, hydrologic conditions (water year type and/or short-term hydrologic conditions), and storage conditions.
The first alternative environmental flow strategy was a fixed percentage of full natural flow (FNF), based on recent policy prescriptions for the Stanislaus, Tuolumne, and Merced basins developed by the State Board, who recommended that 30–50% of FNF be released from February through June to support fish populations in the mainstem of the San Joaquin River (CAEPA, 2018). In this study, the State Board policy (“40% FNF”) was simulated as a requirement to release a 7-day average of 40% of FNF from February through June. From July through January, baseline environmental flow requirements were applied.
The second alternative environmental flow strategy was based on functional flows for California (Yarnell et al., 2015; Yarnell et al., 2020; Stein et al., 2022). The functional flows approach characterizes key flow components, via a suite of flow metrics, which are ecologically protective across rivers and species (Figure 2, Yarnell et al., 2020). The suite of 24 flow metrics describes the magnitude, timing, duration, frequency, and/or rate-of-change of each functional flow component, and can be implemented as environmental flow standards in a variety of ways. For each basin in this study, the natural range of functional flow metrics was calculated from the FNF dataset using signal processing algorithms that characterize seasonal flow features of the annual hydrograph (Patterson et al., 2020). Annual functional flow metrics for each basin were calculated using the Functional Flows Calculator (FFC) API client package in R (version 0.9.7.2, https://github.com/ceff-tech/ffc_api_client), which incorporates the hydrologic feature detection algorithms developed by Patterson et al. (2020) and the Python functional flows calculator (https://github.com/NoellePatterson/ffc-readme). Annual metrics for each basin were sorted using a tercile analysis of total annual flow into three water year types—dry, moderate, and wet. Within the subset of annual metric values for each water year type, the median value of each metric was calculated; these median metrics were then used to guide operation rules in the CenSierraPywr modeling framework for environmental flow releases. The median values of functional flow metrics for each water year type in each basin are provided in Table S2.
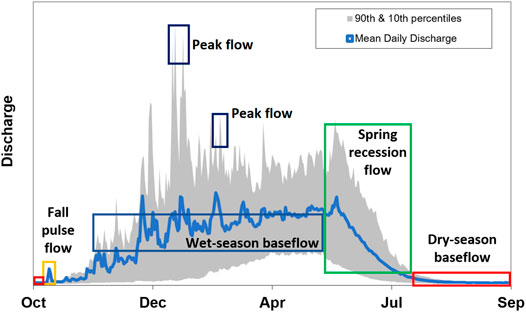
FIGURE 2. Functional flow components for California depicted on a representative hydrograph. Blue line represents median (50th percentile) daily discharge. Gray shading represents 90th–10th percentiles of daily discharge over the period of record. Adapted with permission from Yarnell et al. (2020).
The functional flow releases below each rim reservoir were further refined to incorporate cues from daily hydrologic conditions as releases shifted from one metric to another (Table 2). At the onset of each water year (October 1), the model used a look-up table to determine the appropriate water year type for that year. Except for the first year of the analysis, the dry season baseflow remained the same as in the previous water year. The fall pulse event was released once the daily FNF (inflow into the reservoir) met or exceeded the flow required for the fall pulse event for the given year type, after which baseflows resumed. Flow releases were then governed by that water year type’s metrics and returned to either the dry season baseflow or FNF, whichever was less, until the wet season began.
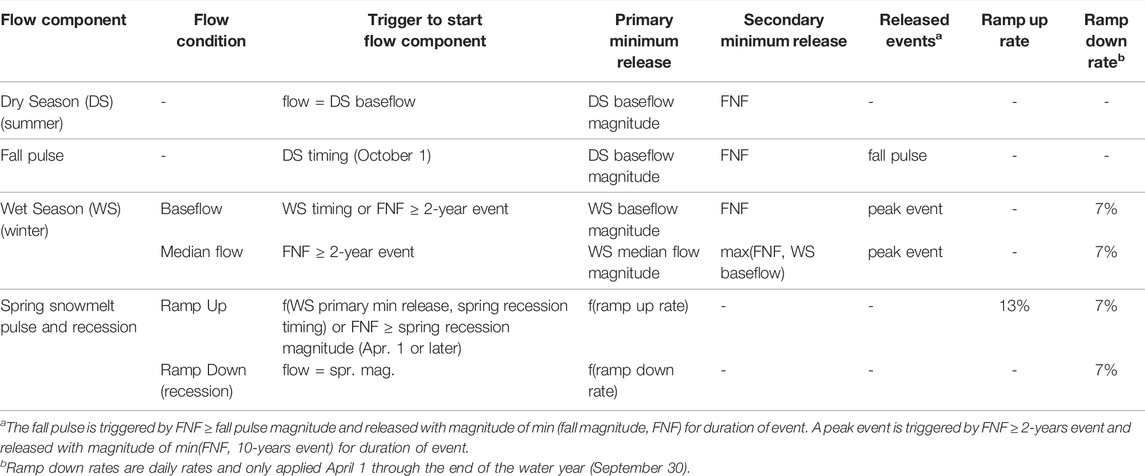
TABLE 2. Minimum releases, ramping rates, released events, and sub-season change cues to implement functional flows for different seasons and sub-seasons.
The wet season baseflow was initially defined as the 10th percentile wet season flow, and was initiated either by the date of the wet season timing metric or the occurrence of a flow event greater than the 2-year peak magnitude and less than the 10-year peak magnitude. Wet season baseflow was increased to wet season median flow following any 2-year or greater peak flow event that occurred after February 1. All peak events between the 2-year and 10-year peak magnitude were released during the wet season; the flow releases during the wet season were thus triggered by incoming flow events, while small floods between the baseflow (or median flow if applicable) and the 2-year peak flow were retained for storage. Peak flow events greater than the 10-year peak magnitude were also retained for storage if space was available in the reservoir.
Wet season flow transitioned to the spring runoff at a date back-calculated from the spring recession start timing and magnitude. At this time, flow ramped up from the wet season baseflow (or median flow if applicable) to the spring magnitude at 13% per day. From this spring magnitude, flow then ramped down at the spring recession rate of change (7%). Further, any peak flow events occurring after April 1 were ramped down at the spring recession rate to limit abrupt changes in flow releases during the spring season. The dry season began when spring recession flow returned to the dry season baseflow; the dry season baseflow or FNF, whichever was less, was then released until the end of the water year (September 30). Ultimately, this ruleset allowed for daily flow prescriptions for each basin for each water year type that were triggered by incoming flows and supported ecologically beneficial functional flows.
Once the daily environmental flow prescriptions were developed, the final step was to integrate the flow prescription into rim dam operations that accounted for other operational goals within each system, including flood control release rules and water storage for hydropower and supply. Rather than optimizing releases across all operational goals, the modeling framework prioritized environmental flows over other demands, such as hydropower or water storage. However, environmental flows were independent from flood control releases, such that the latter could result in releases in excess of the former.
2.4 Analyses
Trade-offs between environmental flows, hydropower, water deliveries, and flood control were quantified by comparing cumulative and seasonal outcomes for each scenario based on daily results. Outflows from the baseline and environmental flow strategies were summarized by using the daily modeled output to calculate the mean total annual volume of environmental flow, annual hydropower production, annual water deliveries, and number of uncontrolled spill days, where uncontrolled spill reflected water flowing over dam spillways under full reservoir conditions. Environmental flow volumes were compared to FNF for each water year type, and daily flow releases were visually assessed in hydrograph form for representative wet, moderate, and dry years. In addition to the mean total annual flow volume, the monthly range of environmental releases were also explored to illustrate differences in seasonal trends for each strategy. Mean annual hydropower energy production, number of uncontrolled spill days, and total water deliveries were calculated over all water year types.
2.5 Limitations
While the study design was developed as an initial framework for actionable reservoir operations, there were some limitations to this method. First, the existing operational logic for the functional flow scenario presumed prior knowledge of the coming water year type. Second, the functional flow schedule was developed based on a statistical analysis of the historical flow patterns, rather than a suite of projected climate change hydrology. Finally, this method was developed for a study area where monitored, real-time inflows and modelled unimpaired stream flows at each of the four rim reservoirs were publicly available. For systems that lack this level of monitoring and modelling, application of this methodology would be challenging.
3 Results
Environmental flow strategies varied by water year type and basin. In general, functional flow releases successfully replicated ecologically important flow components across all year types. Fall pulse flows and snowmelt recession flows occurred across all year types, and peak flows were typically released in wet and some moderate years. In contrast, the 40% FNF strategy provided higher, more variable flows than the baseline strategy during the spring season, but did not provide as many peak flows during the wet season. Figure 3 shows an example of flow releases for each of the three flow strategies as compared to FNF in the Merced River during representative water year types. In the functional flows strategy, peak flows were released during the wet year (Figure 3A); in the moderate and dry years (Figures 3B,C), water was captured throughout the wet season as peak flows did not meet the 2- to 10-year return frequency that would trigger their release. In the 40% FNF strategy, the snowmelt recession was abruptly cut off in the wet year when it extended beyond June, the period when the policy would no longer apply (Figure 3A). Wet season peak flows were not released in any year in the 40% FNF strategy.
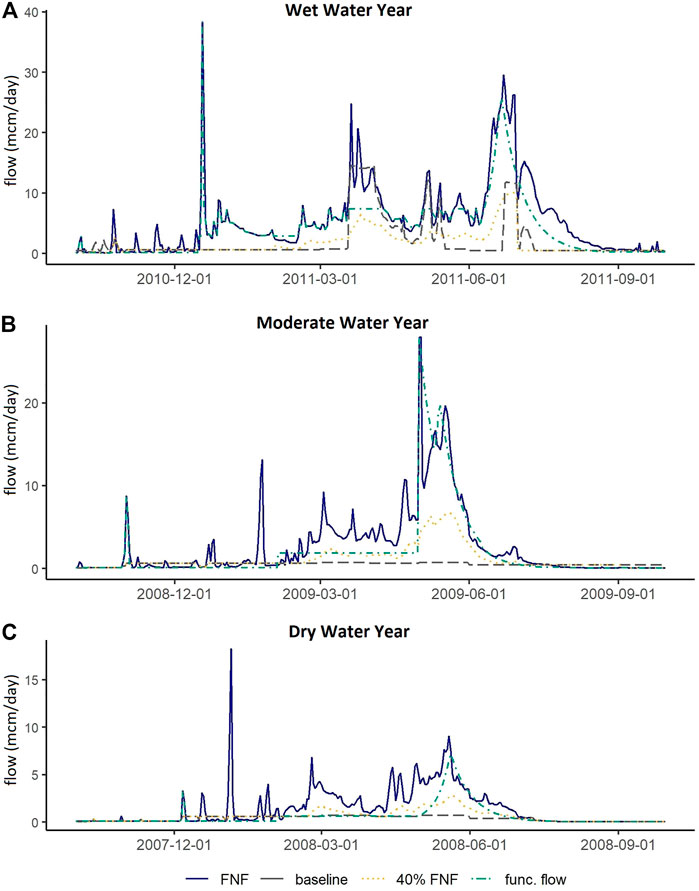
FIGURE 3. Full natural flows are compared to the baseflow, 40% FNF, and functional flow strategies for example (A) wet, (B) moderate, and (C) dry water years.
The alternative environmental flow strategies provided similar or increased mean total annual environmental flow volume as compared to the baseline strategy, but this varied by water year type. Total annual flow volumes averaged across all water year types were 1–22% higher with the functional flows strategy than the 40% FNF strategy, which in turn was 1–17% higher than the baseline strategy (Table 3). The Stanislaus and Upper San Joaquin basins showed similar annual volumes for each alternative environmental flow strategy (1–2% differences) when averaged across water years, while the Tuolumne and Merced basins showed greater differences between the two strategies (11–22%). However, annual flow volumes varied widely between the three strategies depending on water year type. As a percent of mean total annual flow, the functional flows strategy tended to release higher flow volumes during moderate and wet years compared to the 40% FNF strategy, but lower flow volumes during dry years, with the exception of the Tuolumne basin. For example, on the Stanislaus River, each of the three strategies released similar proportions of the annual FNF volume averaged over all water year types; however, in dry years, the functional flows strategy released 53% of annual FNF volume compared to 66% for the 40% FNF strategy. During wet years, the functional flows strategy released 55% of annual FNF volume compared to 45% for the 40% FNF strategy.
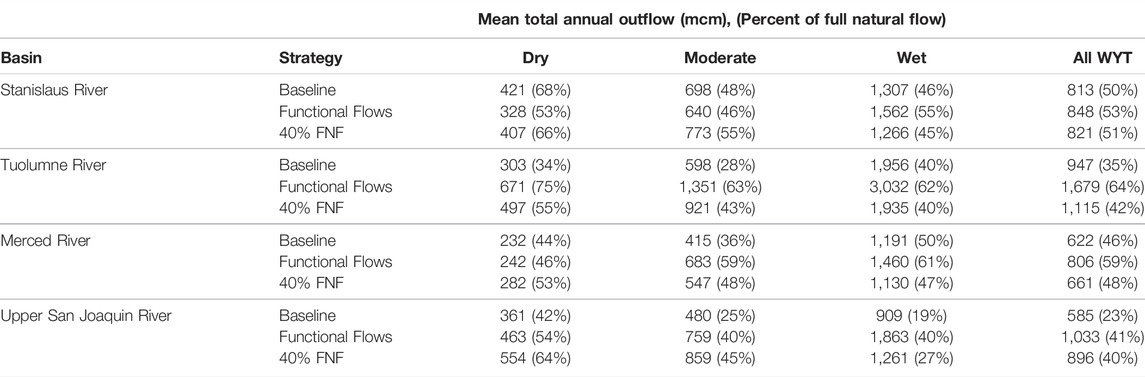
TABLE 3. The mean total annual flow volume released for the baseflow, 40% FNF, and functional flow strategies. Outflows include flood releases. Flow volumes are categorized by basin, strategy, and water year type (WYT).
Differences between the environmental flow strategies in terms of monthly and seasonal flow variability is illustrated by looking at the range of monthly outflows (i.e., the spread of flow for each month) across all water years (Figure 4). During dry season months (July-October), the functional flows strategy resulted in greater flow variability and less outflow than the 40% FNF and baseline strategies (which are the same during this timeframe). The Stanislaus and Merced rivers illustrate this difference, where the baseline and 40% FNF strategies showed almost constant flow from July through December, with a slightly wider range of flows for the baseline strategy in January due to uncontrolled spill. In contrast, the functional flows strategy showed more variable outflows, both within months and across the dry season, and less total outflow than either of the other strategies. During the wet season when peak flow events are more frequent, such as in February, the functional flow strategy typically showed higher average outflows than the baseline or 40% FNF strategies.
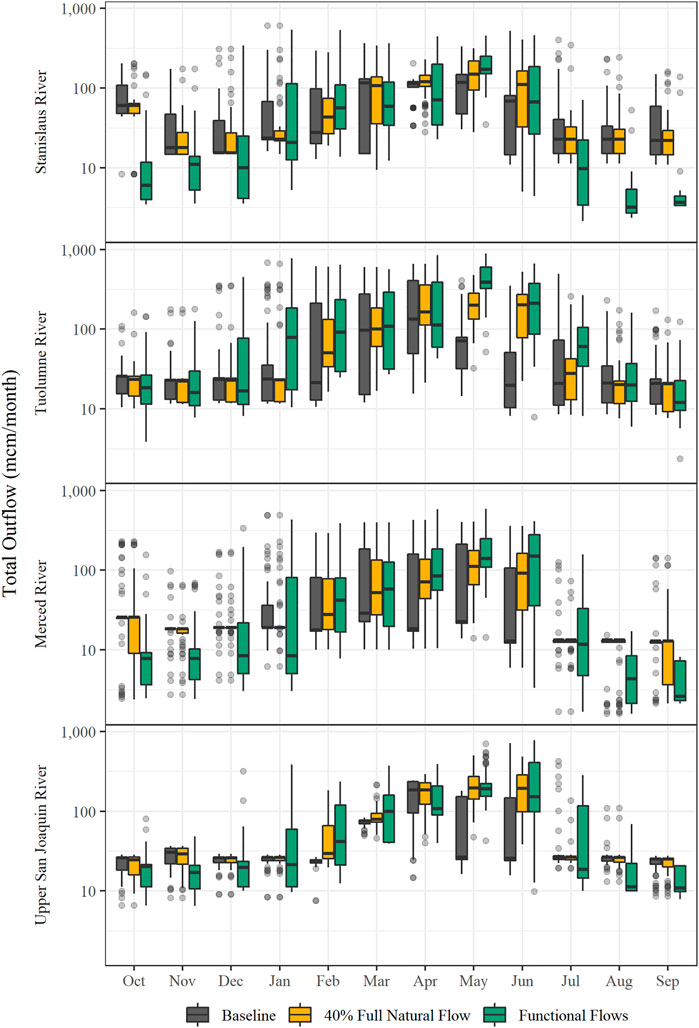
FIGURE 4. Total outflow from each of the four basins for three strategies: baseline, 40% FNF, and functional flows. Note log scale y-axis.
The Tuolumne and San Joaquin River results illustrate the greatest differences in monthly patterns between the baseline and alternative environmental flow strategies. Under baseline operations, outflows from the Tuolumne River are relatively steady from September through December, before increasing to their annual peak in April and then steadily decreasing through August (Figure 4). The greatest variability occurs in February and March. Under both environmental flow strategies, peak stream flows occurred 1–2 months later: functional flows showed peak annual flows in May; 40% FNF showed peak annual flows occurring in June. Functional flows showed the greatest variability in January, when peak flow events typically occurred. Extreme high flow events tended to occur as outliers in a 40% full natural flow strategy. The Upper San Joaquin illustrated similar differences: under baseline operations, outflows remained stable from July through February before increasing to their peak in April and then decreasing. Under both environmental flow strategies, stable baseflows occurred from July through December before increasing to their peak in June, 2 months later than the peak timing of baseline operations. Functional flows showed greater variability and generally lower baseflows than 40% FNF.
Both alternative environmental flow strategies differed from the baseline strategy with regard to hydropower, flood control, and water delivery outcomes. When stream flow releases were prioritized for ecological objectives, mean annual hydropower production decreased negligibly (2–7%) in the Stanislaus and Tuolumne, but increased by 11% in the Upper San Joaquin (Table 4). One exception was in the Merced basin, where the functional flows strategy resulted in 22% less mean annual hydropower.
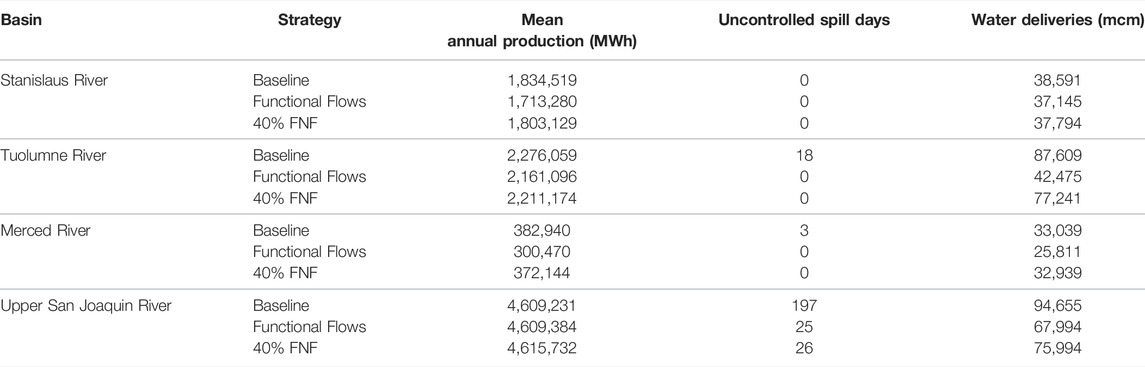
TABLE 4. Mean annual hydropower production, number of uncontrolled spill days, and total water deliveries for each basin given alternative environmental flow requirements.
When analyzing hydropower generated per month, each basin exhibited consistent seasonal patterns of hydropower production across all strategies. The Stanislaus, Tuolumne, and Merced basins reached their median generation peaks in May; the Upper San Joaquin produced the most hydropower in July (Figure 5). The Merced basin showed the most notable differences: median monthly generation decreased by 54–95% from July through September in the functional flow strategy. The difference in median monthly production was less pronounced in the 40% FNF strategy when compared to the baseline strategy, with the exception of notably higher hydropower production in May and June.
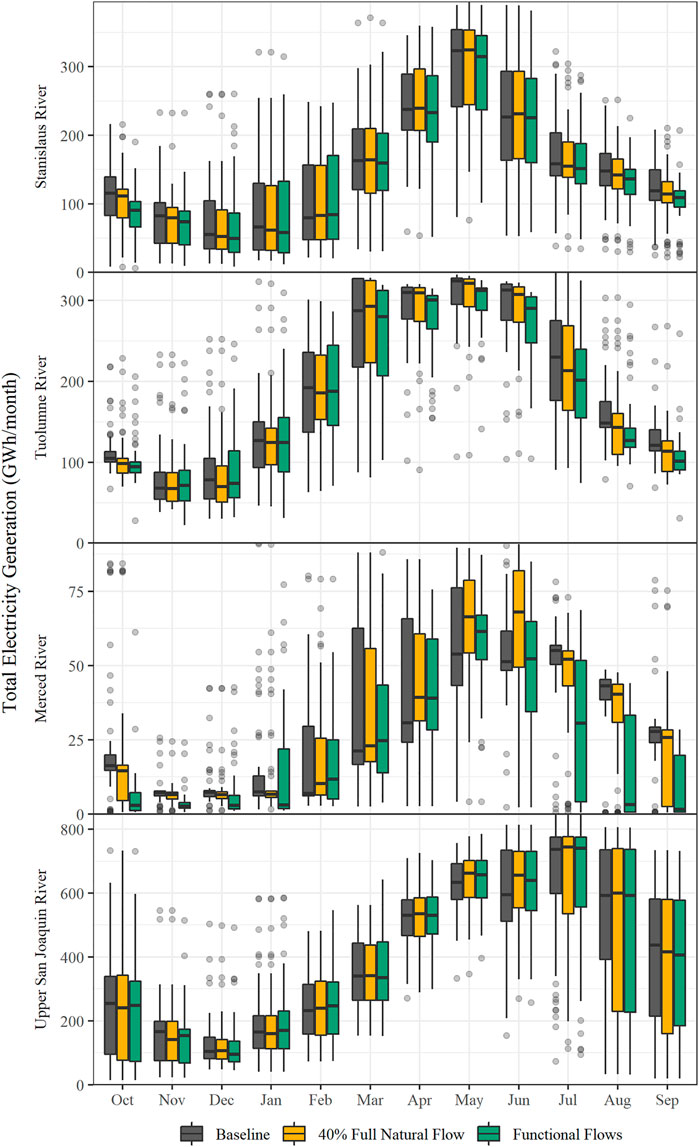
FIGURE 5. Total monthly hydropower production for each of the four basins for three strategies: baseline, 40% FNF, and functional flows. Note the different scales.
Differences in flood control operations were similar to trends observed in hydropower production: negligible differences were noted between the three strategies in most basins, with greater changes observed in the Upper San Joaquin (Table 4). Both alternative environmental flow strategies resulted in a similar number of uncontrolled spill days across all basins. The Upper San Joaquin basin showed the largest difference, with 197 spill days observed in the baseline strategy and 25 and 26 spill days in the functional flows and 40% FNF strategies, respectively (Table 4).
Of the three non-environmental flow uses, water deliveries were most affected by the alternative environmental flow strategies. All basins showed reduced deliveries as compared to baseline strategy, though the extent varied by strategy and geography. The Stanislaus showed the least change, with deliveries reduced by less than 4% in either alternative environmental flow strategy (Table 4). The functional flows strategy showed more than 20% reduction in water deliveries in the Merced and Upper San Joaquin basins and a 52% reduction in water deliveries in the Tuolumne basin. In contrast, the 40% FNF strategy showed a reduction of 20% in water deliveries in the Upper San Joaquin a 12% reduction of water deliveries in the Tuolumne, and a similar volume of deliveries in the Merced.
4 Discussion
4.1 Hydropower and Environmental Flows
California’s Sierra Nevada has steadily transitioned from a diverse and variable freshwater environment to one that is disconnected, homogenized, and, as a result, degraded (Viers and Rheinheimer, 2011). Most endemic species have declined, some to the point of extirpation and many listed for protection under state and/or federal Endangered Species Acts (Moyle et al., 2017). Maintaining the freshwater flow regime is paramount to addressing environmental objectives yet directly affected by hydropower operations. Our study showed that environmental flow regimes supportive of ecosystems can be released while still maintaining most of the potential production of facilities in the San Joaquin watershed.
Negligible to moderate declines in hydropower production occurred where available water was optimized for hydropower production after environmental flow releases were prioritized. In the Upper San Joaquin basin, shifting releases to more ecologically desirable patterns had the serendipitous advantage of increasing hydropower production. In basins where available water was not optimized, production declines were larger. The Merced basin illustrated the least resilience (Boltz et al., 2019) to reconciling environmental flows with hydropower production. This may be due to the lower level of regulation throughout the upper watershed (i.e., no high-altitude reservoirs or powerhouses) and lack of optimization planning for hydropower production. Indeed, the considerable decline in hydropower production during the final months of the dry season reflect the likelihood that any carryover storage is eliminated by existing demands once ecosystem function is taken into account. These results suggest that, in the short-term, hydropower resilience could be improved while simultaneously providing sustainable ecological function by incorporating optimization analyses. Such operational changes may require adjusting existing FERC licenses. While FERC licenses are rarely adjusted outside of the standard 30-year relicensing schedule, reopening existing licensing provides an opportunity to address a near-term challenge to production capacity while meeting licensing requirements for freshwater ecosystem needs (Viers and Nover, 2018).
Understanding the best environmental flow strategy may reduce the frequency of interim licensing adjustments and provide more stability to operation planning. While the 40% FNF strategy generally resulted in greater hydropower production than functional flows, it did not provide the same ecological benefits (e.g., wet season peak flows, larger spring recession flows) as the functional flows strategy, and it often required greater annual outflow in dry years. Current licensing and regulatory shortfalls (e.g., NEPA) would be remedied by including overall ecosystem function outcomes of various environmental flow strategies rather than using single-factor analyses to determine the “impact” of proposed projects (Viers and Nover, 2018). Furthermore, integrating environmental flow planning with climate change projections would provide clarity to both short and long-term planning horizons (Viers and Nover, 2018). Such an approach may make FERC relicensing, which focuses on operations over decades, more aligned with policies to restore and sustain native ecosystems (Bestgen et al., 2020).
4.2 Sustainable Ecosystems and Regulated Flow
4.2.1 Operations
Integrating flood control and water delivery operations into an analysis of hydropower resilience is critical to accurately assess the constraints and opportunities in a water management system (Khan et al., 2017). The complexities of water management that co-exist with hydropower operations are not generally included into energy research or planning, and conversely, energy objectives are often not well-represented in water research and planning (Karambelkar, 2017; Ziaja, 2019). By including water delivery and flood control operations, our study not only provides a more holistic view of hydropower production, but also illustrates areas where reservoir operations can be adjusted to better align with environmental needs.
Flood control operations illustrated the greatest potential to both support and constrain the implementation of environmental flow policies. Flood control and hydropower operations for reservoirs with large storage capacities tend to eliminate all but the most extreme floods and artificially prolong higher flows following these floods (Richter and Thomas, 2007; Yarnell et al., 2010). Strategies that target higher baseflow and higher peak flows have resulted in large benefits for ecological processes, even when those strategies simply shift releases without increasing the overall volume of water released for environmental objectives (Bestgen et al., 2020). In this study, focusing on opportunities to increase peak flow release magnitudes and frequency by following natural hydrological cues both improved ecologically beneficial flows and reduced reservoir spill events. Our functional flows logic was designed to reintroduce ecologically valuable floods (e.g., 2- to 10-year recurrence), while storing water from small wet season floods (less than 2-year recurrence) as well as extreme peak events (greater than 10-year recurrence). The results suggest that restoring moderate and wet hydrologic function may be more critical to sustaining California’s stream ecosystems than providing enhanced flows during dry years: functional flow water allocations during the wet season and moderate and wet years showed the greatest difference from baseline operations. While releasing additional water for ecosystems often triggers conflict from completing users, releasing higher flows during wet and moderate years may be more acceptable if relatively reduced (but still functional) environmental flows are expected during dry years. In contrast, the 40% FNF strategy provided a range of moderate baseflows during the spring, but releases never exceeded the 2-year return interval, which is necessary for wet season and flood-related functionality.
Similar to other studies, the results highlighted how current flood control design is the greatest constraint to restoring effective environmental flow strategies (Bednarek and Hart, 2005; Krause et al., 2005; McManamay et al., 2013). This study explored environmental flow releases that ignored existing downstream infrastructure constraints, such as downstream channel capacity and potential damages to existing agricultural and municipal development. Currently, none of the basins are designed to support prescribed peak flow releases from either alternative environmental flow strategy, regardless of the willingness of stakeholders to provide them. Even passing lower peak flows (i.e., 2-year events) would exceed the design flood or downstream channel capacity in each basin, illustrating the stark misalignment between restoring functional hydrology and existing water management constraints. Infrastructure and policy modifications will likely be necessary to implement an effective environmental flow regime. Ameliorating this challenge may seem less daunting once the ecological (and human) benefits of prioritized environmental flows are accounted for (Richter and Thomas 2007). Although such changes might incur short-term one-time costs to implement (e.g., relocating communities built in floodplains to allow for improved floodplain services), the long-term benefit to ecological and human communities may make such efforts worthwhile (Tickner et al., 2020; Serra-Llobet et al., 2022).
The modeling results pertaining to water deliveries also illustrated the physical limits of each basin’s underlying hydrology to satisfy all objectives. The differences in environmental flow strategies for storing surface water highlighted the overall capacity of these watersheds to meet additional water demand. The capture of small wet season floods and extreme peak events in the functional flow strategy allowed for ecologically important winter baseflow and peak flow releases without relying on opportunistic capture of peak flows alone, yet provided less annual water delivery on average. The 40% FNF strategy provided more reliable opportunities for storage by allowing reservoirs to capture a percentage of all flows, but then failed to provide ecological functionality during the wet season and provided limited functionality during spring and fall. These results suggest that delivery reductions may be necessary in some instances to achieve successful environmental flow management. The study results also showed that, even when operational refinements such as variable water year type prescriptions, daily time steps, and optimization were included, water deliveries were reduced in many instances while maintaining ecosystem functionality and flood control. The Stanislaus basin was the most resilient to shifting environmental flow policies in that the total amount of water released for the environment, hydropower production, uncontrolled spill, and deliveries remained comparable to baseline; the Tuolumne and Merced basins were the least resilient when additional factors like water deliveries were considered. With climate change altering hydrologic pathways that reduce runoff through increased evapotranspiration, sublimation, and infiltration (Hamlet et al., 2007), runoff-dependent water supplies will become more limited (Nover et al., 2019). However, it is possible that modest reductions in water deliveries could have large benefits for ecosystem function if flows are strategically reallocated by timing and location (Zamani Sabzi et al., 2019). Although optimal water allocation strategies were not considered in this study, results suggest that an overall reduction in water deliveries may be necessary to support sustainable freshwater ecosystems. This may be challenging given consistent projections that future water demands will grow, though these projections tend to overestimate the demand that is ultimately observed (Gleick and Cooley, 2021).
4.2.2 Environmental Flow Policy
As well as highlighting opportunities to improve operational constraints, our study illustrates where California may need to realign its water management policy to better integrate with stream ecosystems. Our study explored the trade-offs that would occur if alternative environmental flow strategies were implemented as prioritized objectives rather than subsequent outcomes. Recent policy changes from California’s State Water Resources Control Board suggest that environmental flow prioritization, rather than optimization of multi-objectives, is a likely pathway for regulation. Modifying flow regimes to prioritize environmental objectives is commonly viewed as unfeasible because of the cost to other objectives (Richter, 2010; Lessard et al., 2013). However, when environmental flows are designed to achieve specific ecological goals and potentially managed in conjunction with other policy objectives, new opportunities arise (Richter, 2010).
As suggested by Acreman et al. (2014), most environmental flow methods are based upon one of two general concepts: 1) limiting flow regime alterations from a natural condition to conserve biodiversity (e.g., the “acceptable” percent deviation, policy-driven 40% FNF strategy) and 2) a management-based approach in which environmental flows aim for specific outcomes (e.g., the data-driven, process-based functional flow strategy). When compared to exceedance flow strategies, percent-of-flow regimes have been shown to provide the highest energy production (Kuriqi et al., 2017), but they had yet to be compared to a functional flows strategy prior to this study. Generally, a hydrologic alteration of <20% of unimpaired flow is the standard to maintain ecosystem function (Richter et al., 2012), though allocations of 40–60% of unimpaired flows for the environment have been explored for their ability to support hydropower and other societal objectives (McManamay et al., 2016; Zamani Sabzi et al., 2019). While the 40% FNF strategy in this study more closely aligned with the baseline strategy flow releases and hydropower production, it failed to address the need for wet season peak flows and curtailed critical snowmelt recession function during wet years. The curtailed snowmelt recession function may be addressed by revising the period during which the 40% FNF strategy is required; however, addressing the lack of wet season peak flows would require larger releases beyond the magnitudes that would be accommodated by a proposed percent-of-flow strategy. As such, it seems unlikely to achieve the environmental objectives it is designed to address.
While the functional flows strategy was designed to mimic specific ecological functions of the unimpaired hydrograph, it also faces considerable implementation challenges. While our logic ruleset in the modelling framework addressed operational challenges, the magnitudes of required functional flows highlighted the infrastructure constraints on ecosystem function. The objective of a functional flows-based strategy is not simply releasing environmental flows; it is releasing water that interacts with the surrounding landscape (e.g., floodplains) to achieve comprehensive ecological function (Yarnell et al., 2015; Whipple and Viers, 2019). Thus, environmental flow policies should align with other policy objectives, such as the recent Sustainable Groundwater Management Act (AB 1739, SB 1168, and SB 1319), to ensure the full range of ecosystem functions are achieved (Yarnell et al., 2022). Pairing winter peak flow releases with groundwater recharge and reconnected floodplains, either through multi-objective land use strategies (e.g., the Yolo Bypass, an important floodwater-groundwater interchange north of the study region) or managed retreat of developed riparian lands (Dybala et al., 2019) could be a strategy to achieve synergistic conservation goals (Serra-Llobet et al., 2022). Enhanced environmental flows for groundwater dependent ecosystems is one benefit of agricultural managed aquifer recharge (ag-MAR; Damigos et al., 2017; Levintal et al., 2022), including the San Joaquin watershed (Kourakos et al., 2019; Alam et al., 2020; Levintal et al., 2022). Ag-MAR may be a scalable strategy in a highly agricultural region like the San Joaquin watershed, where high magnitude flows that balance environmental flow function and managed aquifer recharge are available 4.7 out of 10 years (Kocis and Dahlke, 2017; Levintal et al., 2022). Water management that is guided by policy synergies and multiple benefits, rather than evaluations of human vs. environmental trade-offs, is the most likely path to sustainability and climate resilience.
4.3 Portfolio Management and Trade-Offs
Analyzing alternative environmental flow strategies in multiple basins showed nuanced but important differences in trade-offs with respect to managing hydrologic variability. Diversity—defined broadly with respect to habitat complexity and hydrological dynamism—is a key concept underlying successful ecosystem function (Bestgen et al., 2020), and ecosystems—defined broadly to include species assemblages and supporting biogeochemical fluxes—are rarely endemic to single catchments. Indeed, when focusing on recovery efforts for anadromous fish, which is often the objective for environmental flows below California’s Central Valley rim dams, the integrated diversity of mainstem and tributary streams is the underlying foundation for ecological function (Phillis et al., 2018). Our study results show that managing for annual flow variability across basins could improve both ecological conditions and water supply for non-environmental uses. The functional flow strategy results support using a regional portfolio framework that leverages the diversity of hydrologic conditions and services provided by each watershed. Such regional coordination would impose coherent management for conservation and optimize energy production for the power grid.
In addition, managing for diverse hydrologic conditions tied to full natural inflows in each basin, rather than storage (e.g., Yin et al., 2011) or a homogenized, regional hydrologic index, could also have important implications for the overall resilience for each basin. While general results may not seem uniformly favorable (or disadvantageous) for hydropower, water supply, and flood control, it is important to consider each basin in coordination with the others. For example, the functional flows strategy partly benefitted the Upper San Joaquin via enhanced hydropower production, though was less impactful in the Stanislaus with regard to non-environmental objectives. The tradeoff between environmental flows and other objectives may be worthwhile if benefits in the San Joaquin result in lower regulatory burdens in the other watersheds, even if fewer direct benefits occur in those watersheds. Managing each watershed based on its specific hydrologic condition, rather than a common, regional index, is key. Currently, the four basins in the San Joaquin are mostly managed using a single-metric index, the San Joaquin Valley Index (SJVI) (see Null and Viers (2013) for expanded discussion). The tercile approach used in the functional flows strategy showed that in 21% of all years, hydrologic year types differed across the four basins (Supplementary Table S3). Under the existing policy for management based on the SJVI, basins that are drier (see Table 2) may be required to release more water than the unimpaired runoff could support. Defining the hydrologic condition for each basin independently, as in the functional flows strategy in this study, provides a more realistic accounting of water available for non-environmental uses and preserves the hydrologic variability that sustains ecosystems.
5 Future Work
Our study focused on hydropower production given alternative environmental flow strategies below rim dams in the San Joaquin watershed. However, there are other aspects of flow management that are important to consider in future studies that build on this work. The operational logic guiding functional flow releases could be further developed to define water year types given on-going observations of each basin’s hydrological condition, which would represent a more realistic adaptive management strategy than the current, a priori method. More broadly, managing flow independent of other stream ecosystem elements like geomorphology or riparian and floodplain habitat may improve instream hydrologic conditions, but have little influence over other critical components like stream temperature or biodiversity (Krause et al., 2005). Also, the focus on rim dams in this study overlooks the potentially cascading effects of implementing a functional flows strategy in high elevation reaches, which would more directly overlap with hydropower facilities that are often positioned in series. Climate change-induced hydrological alteration poses a similar risk to both hydropower and environmental flows; future work should explore whether shifts in stream flow fundamentally alter the ecological functions supported by climate changed hydrology. Finally, while studies have shown that it is possible to balance existing human demands while achieving key ecosystem targets under a functional flows approach (Kiernan et al., 2012; Chen and Olden, 2017; Sabo et al., 2017), human demands are unlikely to remain stationary. In addition to getting better ecological value from environmental flow management (Viers, 2017), understanding shifts in both hydrologic and non-environmental demands will be critical to identify the total capacity of water systems to support extractive water use.
Data Availability Statement
The datasets, functional flow calculator, and hydrologic detection algorithms used in this study can be found in online repositories. The names of the repository/repositories and accession number(s) can be found below: https://github.com/.
Author Contributions
AW, JV, DR, SY, and AR contributed to the conception and design of the study. AW, DR, SY, and GFD developed the alternative environmental flow strategies. GFD and DR developed the model code for CenSierraPywr. AW wrote the first draft of the manuscript and made primary revisions. All authors contributed to manuscript revision, read, and approved the submitted version.
Funding
The research was funded by the California Energy Commission (300-15-004) and the Department of Energy Clean Energy Research Center for Water and Energy Technologies (DE-IA0000018).
Conflict of Interest
The authors declare that the research was conducted in the absence of any commercial or financial relationships that could be construed as a potential conflict of interest.
Publisher’s Note
All claims expressed in this article are solely those of the authors and do not necessarily represent those of their affiliated organizations, or those of the publisher, the editors and the reviewers. Any product that may be evaluated in this article, or claim that may be made by its manufacturer, is not guaranteed or endorsed by the publisher.
Acknowledgments
Thank you to the California Energy Commission Technical Advisory Committee and Amy Lind for their helpful suggestions and feedback on the project. Thank you to Mahesh Maskey and Lorenzo Scaturchio for their help with model development and implementation. Thank you to Nicholas Santos and Ryan Peek for their help with the functional flows calculator. Thank you to two reviewers for their feedback, which greatly improved this manuscript.
Supplementary Material
The Supplementary Material for this article can be found online at: https://www.frontiersin.org/articles/10.3389/fenvs.2022.787711/full#supplementary-material
References
Acreman, M., Arthington, A. H., Colloff, M. J., Couch, C., Crossman, N. D., Dyer, F., et al. (2014). Environmental Flows for Natural, Hybrid, and Novel Riverine Ecosystems in a Changing World. Front. Ecol. Environ. 12 (8), 466–473. doi:10.1890/130134
Adams, L. E., Lund, J. R., Moyle, P. B., Quiñones, R. M., Herman, J. D., and O'Rear, T. A. (2017). Environmental Hedging: A Theory and Method for Reconciling Reservoir Operations for Downstream Ecology and Water Supply. Water Resour. Res. 53 (9), 7816–7831. doi:10.1002/2016WR020128
Alam, S., Gebremichael, M., Li, R., Dozier, J., and Lettenmaier, D. P. (2020). Can Managed Aquifer Recharge Mitigate the Groundwater Overdraft in California's Central Valley? Water Resour. Res. 56 (8), e2020WR027244. doi:10.1029/2020wr027244
Arthington, A. H. (2012). Environmental Flows: Saving Rivers in the Third Millennium. Berkeley, California, United States: Univ of California Press.
Bednarek, A. T., and Hart, D. D. (2005). Modifying Dam Operations to Restore Rivers: Ecological Responses to Tennessee River Dam Mitigation. Ecol. Appl. 15 (3), 997–1008. doi:10.1890/04-0586
Beechie, T. J., Sear, D. A., Olden, J. D., Pess, G. R., Buffington, J. M., Moir, H., et al. (2010). Process-based Principles for Restoring River Ecosystems. BioScience 60 (3), 209–222. doi:10.1525/bio.2010.60.3.7
Bestgen, K. R., Poff, N. L., Baker, D. W., Bledsoe, B. P., Merritt, D. M., Lorie, M., et al. (2020). Designing Flows to Enhance Ecosystem Functioning in Heavily Altered Rivers. Ecol. Appl. 30 (1), e02005. doi:10.1002/eap.2005
Boltz, F., LeRoy Poff, N., Folke, C., Kete, N., Brown, C. M., St. George Freeman, S., et al. (2019). Water Is a Master Variable: Solving for Resilience in the Modern Era. Water Secur. 8, 100048. doi:10.1016/j.wasec.2019.100048
California Environmental Flows Working Group (2020). California Water Quality Monitoring Council Technical Report: California Environmental Flows Framework. Sacramento, California, United States: California Water Quality Monitoring Council.
California Environmental Protection Agency (2018). State Water Resources Control Board Policy on Supplement Environmental Projects. Sacramento, California, United States: California Water Quality Monitoring Council. Effective May 3, 2018.
Chen, W., and Olden, J. D. (2017). Designing Flows to Resolve Human and Environmental Water Needs in a Dam-Regulated River. Nat. Commun. 8 (1), 2158–2210. doi:10.1038/s41467-017-02226-4
Cooley, S. W., Ryan, J. C., and Smith, L. C. (2021). Human Alteration of Global Surface Water Storage Variability. Nature 591 (7848), 78–81. doi:10.1038/s41586-021-03262-3
Corral-Verdugo, V., Carrus, G., Bonnes, M., Moser, G., and Sinha, J. B. P. (2008). Environmental Beliefs and Endorsement of Sustainable Development Principles in Water Conservation. Environ. Behav. 40 (5), 703–725. doi:10.1177/0013916507308786
Couto, T. B., and Olden, J. D. (2018). Global Proliferation of Small Hydropower Plants - Science and Policy. Front. Ecol. Environ. 16 (2), 91–100. doi:10.1002/fee.1746
Damigos, D., Tentes, G., Balzarini, M., Furlanis, F., and Vianello, A. (2017). Revealing the Economic Value of Managed Aquifer Recharge: Evidence from a Contingent Valuation Study in Italy. Water Resour. Res. 53 (8), 6597–6611. doi:10.1002/2016wr020281
Deyou, L., Heng, L., Xianlai, W., and Kremere, E. (2019). World Small Hydropower Development Report. 3 ed. Vienna, Austria: United National Industrial Development Organization and International Center on Small Hydro Power.
Dybala, K. E., Steger, K., Walsh, R. G., Smart, D. R., Gardali, T., and Seavy, N. E. (2019). Optimizing Carbon Storage and Biodiversity Co‐benefits in Reforested Riparian Zones. J. Appl. Ecol. 56 (2), 343–353. doi:10.1111/1365-2664.13272
Eichman, J. D., Mueller, F., Tarroja, B., Schell, L. S., and Samuelsen, S. (2013). Exploration of the Integration of Renewable Resources into California's Electric System Using the Holistic Grid Resource Integration and Deployment (HiGRID) Tool. Energy 50, 353–363. doi:10.1016/j.energy.2012.11.024
Frey, G. W., and Linke, D. M. (2002). Hydropower as a Renewable and Sustainable Energy Resource Meeting Global Energy Challenges in a Reasonable Way. Energy Policy 30 (14), 1261–1265. doi:10.1016/S0301-4215(02)00086-1
Georgakakos, K. P., Modrick, T. M., Sperfslage, J. A., Spencer, C. R., Banks, R. J., Georgakakos, A. P., et al. (2018). Integrated Forecast and Reservoir Management (INFORM): Implementation of a Stand-Alone Operational INFORM System for the California Department of Water Resources (DWR). Hydrologic Research Center - Georgia Water Resources Institute.
Gleick, P. H., and Cooley, H. (2021). Freshwater Scarcity. Annu. Rev. Environ. Resour. 46 (1), 319–348. doi:10.1146/annurev-environ-012220-101319
Hamlet, A. F., Mote, P. W., Clark, M. P., and Lettenmaier, D. P. (2007). Twentieth-Century Trends in Runoff, Evapotranspiration, and Soil Moisture in the Western United States*. J. Clim. 20 (8), 1468–1486. doi:10.1175/jcli4051.1
Hamududu, B., and Killingtveit, A. (2017). Assessing Climate Change Impacts on Global Hydropower. Palm Bay, FL: Climate Change and the Future of Sustainability Apple Academic Press, 109–132.
Hidalgo, H. G., Das, T., Dettinger, M. D., Cayan, D. R., Pierce, D. W., Barnett, T. P., et al. (2009). Detection and Attribution of Streamflow Timing Changes to Climate Change in the Western United States. J. Clim. 22 (13), 3838–3855. doi:10.1175/2009jcli2470.1
Horne, A., Webb, A., Stewardson, M., Richter, B., and Acreman, M. (2017). Water for the Environment: From Policy and Science to Implementation and Management. Palm Bay, FL: Academic Press.
International Hydropower Association (2020). Hydropower Status Report. 7 ed. London, United Kingdom: International Hydropower Association.
Karambelkar, S. (2017). Hydropower Development in India: The Legal-Economic Design to Fuel Growth? Nat. Resour. J. 57 (2), 361–394. http://www.jstor.org/stable/26202202.
Katz, J., Moyle, P. B., Quiñones, R. M., Israel, J., and Purdy, S. (2013). Impending Extinction of Salmon, Steelhead, and Trout (Salmonidae) in California. Environ. Biol. Fishes 96 (10), 1169–1186. doi:10.1007/s10641-012-9974-8
Khan, Z., Linares, P., and García-González, J. (2017). Integrating Water and Energy Models for Policy Driven Applications. A Review of Contemporary Work and Recommendations for Future Developments. Renew. Sustain. Energy Rev. 67, 1123–1138. doi:10.1016/j.rser.2016.08.043
Kiernan, J. D., Moyle, P. B., and Crain, P. K. (2012). Restoring Native Fish Assemblages to a Regulated California Stream Using the Natural Flow Regime Concept. Ecol. Appl. 22 (5), 1472–1482. doi:10.1890/11-0480.1
Knowles, N., and Cronkite-Ratcliff, C. (2018). Modeling Managed Flows in the Sacramento/San Joaquin Watershed, California, under Scenarios of Future Change for CASCaDE2. Reston, Virginia: US Department of the Interior, US Geological Survey.
Kocis, T. N., and Dahlke, H. E. (2017). Availability of High-Magnitude Streamflow for Groundwater Banking in the Central Valley, California. Environ. Res. Lett. 12 (8), 084009. doi:10.1088/1748-9326/aa7b1b
Kourakos, G., Dahlke, H. E., and Harter, T. (2019). Increasing Groundwater Availability and Seasonal Base Flow through Agricultural Managed Aquifer Recharge in an Irrigated Basin. Water Resour. Res. 55 (9), 7464–7492. doi:10.1029/2018wr024019
Krause, C. W., Newcomb, T. J., and Orth, D. J. (2005). Thermal Habitat Assessment of Alternative Flow Scenarios in a Tailwater Fishery. River Res. applic. 21 (6), 581–593. doi:10.1002/rra.829
Kuriqi, A., Pinheiro, A. N., Sordo-Ward, A., Bejarano, M. D., and Garrote, L. (2021). Ecological Impacts of Run-Of-River Hydropower Plants-Current Status and Future Prospects on the Brink of Energy Transition. Renew. Sustain. Energy Rev. 142, 110833. doi:10.1016/j.rser.2021.110833
Kuriqi, A., Pinheiro, A. N., Sordo-Ward, A., and Garrote, L. (2017). Trade-off between Environmental Flow Policy and Run-Of-River Hydropower Generation in Mediterranean Climate. Eur. Water 60, 123–130. http://www.ewra.net/ew/pdf/EW_2017_60_17.pdf.
Kuriqi, A., Pinheiro, A. N., Sordo-Ward, A., and Garrote, L. (2020). Water-energy-ecosystem Nexus: Balancing Competing Interests at a Run-Of-River Hydropower Plant Coupling a Hydrologic-Ecohydraulic Approach. Energy Convers. Manag. 223, 113267. doi:10.1016/j.enconman.2020.113267
Lessard, J., Murray Hicks, D., Snelder, T. H., Arscott, D. B., Larned, S. T., Booker, D., et al. (2013). Dam Design Can Impede Adaptive Management of Environmental Flows: a Case Study from the Opuha Dam, New Zealand. Environ. Manag. 51 (2), 459–473. doi:10.1007/s00267-012-9971-x
Levintal, E., Kniffin, M. L., Ganot, Y., Marwaha, N., Murphy, N. P., and Dahlke, H. E. (2022). Agricultural Managed Aquifer Recharge (Ag-Mar)-A Method for Sustainable Groundwater Management: A Review. Crit. Rev. Environ. Sci. Technol. 2022, 1–24. doi:10.1080/10643389.2022.2050160
Liang, X., Lettenmaier, D. P., Wood, E. F., and Burges, S. J. (1994). A Simple Hydrologically Based Model of Land Surface Water and Energy Fluxes for General Circulation Models. J. Geophys. Res. 99 (D7), 14415–14428. doi:10.1029/94jd00483
Livneh, B., Bohn, T., Pierce, D., Muñoz‐Arriola, F., Nijssen, B., Vose, R., et al. (2015). A Spatially Comprehensive, Hydrometeorological Data Set for Mexico, the U.S., and Southern Canada 1950-2013. Sci. Data 2 (1), 150042. doi:10.1038/sdata.2015.42
McManamay, R. A., Brewer, S. K., Jager, H. I., and Troia, M. J. (2016). Organizing Environmental Flow Frameworks to Meet Hydropower Mitigation Needs. Environ. Manag. 58 (3), 365–385. doi:10.1007/s00267-016-0726-y
McManamay, R. A., Orth, D. J., Dolloff, C. A., and Mathews, D. C. (2013). Application of the ELOHA Framework to Regulated Rivers in the Upper Tennessee River Basin: a Case Study. Environ. Manag. 51 (6), 1210–1235. doi:10.1007/s00267-013-0055-3
Moyle, P. B., Lusardi, R. A., Samuel, P., and Katz, J. (2017). State of the Salmonids: Status of California's Emblematic Fishes 2017. Davis; California Trout: University of California.
Nover, D. M., Dogan, M. S., Ragatz, R., Booth, L., Medellín‐Azuara, J., Lund, J. R., et al. (2019). Does More Storage Give California More Water? J. Am. Water Resour. Assoc. 55 (3), 759–771. doi:10.1111/1752-1688.12745
Null, S. E., and Viers, J. H. (2013). In Bad Waters: Water Year Classification in Nonstationary Climates. Water Resour. Res. 49 (2), 1137–1148. doi:10.1002/wrcr.20097
Palmer, M., and Ruhi, A. (2019). Linkages between Flow Regime, Biota, and Ecosystem Processes: Implications for River Restoration. Science 365 (6459), 2087. doi:10.1126/science.aaw2087
Palmer, M. A., Reidy Liermann, C. A., Nilsson, C., Flörke, M., Alcamo, J., Lake, P. S., et al. (2008). Climate Change and the World's River Basins: Anticipating Management Options. Front. Ecol. Environ. 6 (2), 81–89. doi:10.1890/060148
Patterson, N. K., Lane, B. A., Sandoval-Solis, S., Pasternack, G. B., Yarnell, S. M., and Qiu, Y. (2020). A Hydrologic Feature Detection Algorithm to Quantify Seasonal Components of Flow Regimes. J. Hydrology 585, 124787. doi:10.1016/j.jhydrol.2020.124787
Phillis, C. C., Sturrock, A. M., Johnson, R. C., and Weber, P. K. (2018). Endangered Winter-Run Chinook Salmon Rely on Diverse Rearing Habitats in a Highly Altered Landscape. Biol. Conserv. 217, 358–362. doi:10.1016/j.biocon.2017.10.023
Poff, N. L., Allan, J. D., Bain, M. B., Karr, J. R., Prestegaard, K. L., Richter, B. D., et al. (1997). The Natural Flow Regime. BioScience 47 (11), 769–784. doi:10.2307/1313099
Poff, N. L. (2018). Beyond the Natural Flow Regime? Broadening the Hydro-Ecological Foundation to Meet Environmental Flows Challenges in a Non-stationary World. Freshw. Biol. 63 (8), 1011–1021. doi:10.1111/fwb.13038
Poff, N. L., and Matthews, J. H. (2013). Environmental Flows in the Anthropocence: Past Progress and Future Prospects. Curr. Opin. Environ. Sustain. 5 (6), 667–675. doi:10.1016/j.cosust.2013.11.006
Poff, N. L., Richter, B. D., Arthington, A. H., Bunn, S. E., Naiman, R. J., Kendy, E., et al. (2010). The Ecological Limits of Hydrologic Alteration (ELOHA): a New Framework for Developing Regional Environmental Flow Standards. Freshw. Biol. 55 (1), 147–170. doi:10.1111/j.1365-2427.2009.02204.x
Poff, N. L., and Zimmerman, J. K. H. (2010). Ecological Responses to Altered Flow Regimes: a Literature Review to Inform the Science and Management of Environmental Flows. Freshw. Biol. 55 (1), 194–205. doi:10.1111/j.1365-2427.2009.02272.x
Porse, E. C., Sandoval-Solis, S., and Lane, B. A. (2015). Integrating Environmental Flows into Multi-Objective Reservoir Management for a Transboundary, Water-Scarce River Basin: Rio Grande/bravo. Water Resour. Manage 29 (8), 2471–2484. doi:10.1007/s11269-015-0952-8
Reid, A. J., Carlson, A. K., Creed, I. F., Eliason, E. J., Gell, P. A., Johnson, P. T. J., et al. (2019). Emerging Threats and Persistent Conservation Challenges for Freshwater Biodiversity. Biol. Rev. 94 (3), 849–873. doi:10.1111/brv.12480
Rheinheimer, D. E., Sood, A., Tran, D., Scaturchio, L., and Facincani Duorado, G. (2021). CenSierraPywr. Merced, California, United States. 2021-08-30.
Rheinheimer, D. E., Viers, J. H., Sieber, J., Kiparsky, M., Mehta, V. K., and Ligare, S. T. (2014). Simulating High-Elevation Hydropower with Regional Climate Warming in the West Slope, Sierra Nevada. J. Water Resour. Plann. Manage. 140 (5), 714–723. doi:10.1061/(asce)wr.1943-5452.0000373
Rheinheimer, D. E., Yarnell, S. M., and Viers, J. H. (2013). Hydropower Costs of Environmental Flows and Climate Warming in California's Upper Yuba River Watershed. River Res. applic. 29 (10), 1291–1305. doi:10.1002/rra.2612
Richter, B. D., Davis, M. M., Apse, C., and Konrad, C. (2012). A Presumptive Standard for Environmental Flow Protection. River Res. applic. 28 (8), 1312–1321. doi:10.1002/rra.1511
Richter, B. D. (2010). Re-thinking Environmental Flows: from Allocations and Reserves to Sustainability Boundaries. River Res. applic. 26 (8), 1052–1063. doi:10.1002/rra.1320
Richter, B. D., and Thomas, G. A. (2007). Restoring Environmental Flows by Modifying Dam Operations. Ecol. Soc. 12, 1. doi:10.5751/es-02014-120112
Sabo, J. L., Ruhi, A., Holtgrieve, G. W., Elliott, V., Arias, M. E., Ngor, P. B., et al. (2017). Designing River Flows to Improve Food Security Futures in the Lower Mekong Basin. Science 358 (6368), 1053. doi:10.1126/science.aao1053
Serra-Llobet, A., Jähnig, S. C., Geist, J., Kondolf, G. M., Damm, C., Scholz, M., et al. (2022). Restoring Rivers and Floodplains for Habitat and Flood Risk Reduction: Experiences in Multi-Benefit Floodplain Management from California and Germany. Front. Environ. Sci. 9, 788568. doi:10.3389/fenvs.2021.778568
State Water Resources Control Board (2018). Water Quality Control Plan for the San Francisco Bay/Sacramento-San Joaquin Delta Estuary. Sacramento, California, United States: California Environmental Protection Agency.
Stein, E. D., Zimmerman, J., Yarnell, S. M., Stanford, B., Lane, B., Taniguchi-Quan, K. T., et al. (2022). The California Environmental Flows Framework: Meeting the Challenges of Developing a Large-Scale Environmental Flows Program. Front. Environ. Sci. 9, 769943. doi:10.3389/fenvs.2021.769943
Tarroja, B., AghaKouchak, A., and Samuelsen, S. (2016). Quantifying Climate Change Impacts on Hydropower Generation and Implications on Electric Grid Greenhouse Gas Emissions and Operation. Energy 111, 295–305. doi:10.1016/j.energy.2016.05.131
Tharme, R. E. (2003). A Global Perspective on Environmental Flow Assessment: Emerging Trends in the Development and Application of Environmental Flow Methodologies for Rivers. River Res. applic. 19 (5-6), 397–441. doi:10.1002/rra.736
Tickner, D., Kaushal, N., Speed, R., and Tharme, R. (2020). Editorial: Implementing Environmental Flows: Lessons for Policy and Practice. Front. Environ. Sci. 8, 106. doi:10.3389/fenvs.2020.00106
Tomlinson, J. E., Arnott, J. H., and Harou, J. J. (2020). A Water Resource Simulator in Python. Environ. Model. Soft. 126, 104635. doi:10.1016/j.envsoft.2020.104635
Viers, J. H. (2011). Hydropower Relicensing and Climate Change1. JAWRA J. Am. Water Resour. Assoc. 47 (4), 655–661. doi:10.1111/j.1752-1688.2011.00531.x
Viers, J. H. (2017). Meeting Ecosystem Needs while Satisfying Human Demands. Environ. Res. Lett. 12 (6), 061001. doi:10.1088/1748-9326/aa6e87
Viers, J. H., and Nover, D. M. (2018). Too Big to Fail: Limiting Public Risk in Hydropower Licensing. Hastings Envt'l LJ 24, 143. https://escholarship.org/uc/item/1d77g1wc.
Viers, J. H., and Rheinheimer, D. E. (2011). Freshwater Conservation Options for a Changing Climate in California's Sierra Nevada. Mar. Freshw. Res. 62 (3), 266–278. doi:10.1071/mf09286
Voisin, N., Kintner-Meyer, M., Skaggs, R., Nguyen, T., Wu, D., Dirks, J., et al. (2016). Vulnerability of the US Western Electric Grid to Hydro-Climatological Conditions: How Bad Can it Get? Energy 115, 1–12. doi:10.1016/j.energy.2016.08.059
Voisin, N., Kintner-Meyer, M., Wu, D., Skaggs, R., Fu, T., Zhou, T., et al. (2018). Opportunities for Joint Water-Energy Management: Sensitivity of the 2010 Western U.S. Electricity Grid Operations to Climate Oscillations. Bull. Am. Meteorological Soc. 99 (2), 299–312. doi:10.1175/bams-d-16-0253.1
Whipple, A. A., and Viers, J. H. (2019). Coupling Landscapes and River Flows to Restore Highly Modified Rivers. Water Resour. Res. 55 (6), 4512–4532. doi:10.1029/2018WR022783
Widén, Å., Malm Renöfält, B., Degerman, E., Wisaeus, D., and Jansson, R. (2022). Environmental Flow Scenarios for a Regulated River System: Projecting Catchment‐Wide Ecosystem Benefits and Consequences for Hydroelectric Production. Water Resour. Res. 58 (1), e2021WR030297. doi:10.1029/2021WR030297
Wohl, E., Bledsoe, B. P., Jacobson, R. B., Poff, N. L., Rathburn, S. L., Walters, D. M., et al. (2015). The Natural Sediment Regime in Rivers: Broadening the Foundation for Ecosystem Management. BioScience 65 (4), 358–371. doi:10.1093/biosci/biv002
Yarnell, S. M., Petts, G. E., Schmidt, J. C., Whipple, A. A., Beller, E. E., Dahm, C. N., et al. (2015). Functional Flows in Modified Riverscapes: Hydrographs, Habitats and Opportunities. BioScience 65 (10), 963–972. doi:10.1093/biosci/biv102
Yarnell, S. M., Stein, E. D., Webb, J. A., Grantham, T., Lusardi, R. A., Zimmerman, J., et al. (2020). A Functional Flows Approach to Selecting Ecologically Relevant Flow Metrics for Environmental Flow Applications. River Res. Applic 36 (2), 318–324. doi:10.1002/rra.3575
Yarnell, S. M., Viers, J. H., and Mount, J. F. (2010). Ecology and Management of the Spring Snowmelt Recession. Bioscience 60 (2), 114–127. doi:10.1525/bio.2010.60.2.6
Yarnell, S. M., Willis, A., Obester, A., Peek, R. A., Lusardi, R. A., Zimmerman, J., et al. (2022). Functional Flows in Groundwater-Influenced Streams: Application of the California Environmental Flows Framework to Determine Ecological Flow Needs. Front. Environ. Sci. 9, 788295. doi:10.3389/fenvs.2021.788295
Yin, X. A., Yang, Z. F., and Petts, G. E. (2011). Reservoir Operating Rules to Sustain Environmental Flows in Regulated Rivers. Water Resour. Res. 47 (8), W08509. doi:10.1029/2010wr009991
Zamani Sabzi, H., Rezapour, S., Fovargue, R., Moreno, H., and Neeson, T. M. (2019). Strategic Allocation of Water Conservation Incentives to Balance Environmental Flows and Societal Outcomes. Ecol. Eng. 127, 160–169. doi:10.1016/j.ecoleng.2018.11.005
Zarfl, C., Lumsdon, A. E., Berlekamp, J., Tydecks, L., and Tockner, K. (2015). A Global Boom in Hydropower Dam Construction. Aquat. Sci. 77 (1), 161–170. doi:10.1007/s00027-014-0377-0
Ziaja, S. (2019). Role of Knowledge Networks and Boundary Organizations in Coproduction: A Short History of a Decision-Support Tool and Model for Adapting Multiuse Reservoir and Water-Energy Governance to Climate Change in California. Weather, Clim. Soc. 11 (4), 823–849. doi:10.1175/wcas-d-19-0007.1
Keywords: environmental flows, environmental water, functional flows, hydropower, multiobjective reservoir, san joaquin river, water management
Citation: Willis AD, Rheinheimer DE, Yarnell SM, Facincani Dourado G, Rallings AM and Viers JH (2022) Shifting Trade-offs: Finding the Sustainable Nexus of Hydropower and Environmental Flows in the San Joaquin River Watershed, California. Front. Environ. Sci. 10:787711. doi: 10.3389/fenvs.2022.787711
Received: 01 October 2021; Accepted: 11 May 2022;
Published: 08 June 2022.
Edited by:
Jonathan Tonkin, University of Canterbury, New ZealandReviewed by:
Nitin Kaushal, World Wide Fund for Nature, IndiaAlban Kuriqi, Universidade de Lisboa, Portugal
Copyright © 2022 Willis, Rheinheimer, Yarnell, Facincani Dourado, Rallings and Viers. This is an open-access article distributed under the terms of the Creative Commons Attribution License (CC BY). The use, distribution or reproduction in other forums is permitted, provided the original author(s) and the copyright owner(s) are credited and that the original publication in this journal is cited, in accordance with accepted academic practice. No use, distribution or reproduction is permitted which does not comply with these terms.
*Correspondence: Ann D. Willis, awillis@ucdavis.edu