- 1School of Mining and Geosciences, Nazarbayev University, Nur-Sultan, Kazakhstan
- 2Institute of Geodesy and Geoinformation Science, Technische Universität Berlin, Berlin, Germany
- 3Institute for Cartography, Dresden University of Technology, Dresden, Germany
- 4Kazakhstan Maritime Academy, Kazakh-British Technical University, Almaty, Kazakhstan
This study focused on the quantitative assessment of the vertical displacement velocities retrieved using Sentinel-1 and Cosmo-SkyMed synthetic aperture radar images for the Tengiz oilfield. Tengiz oilfield was selected as a study area because of its historically reported continuous subsidence and limited up-to-date studies during recent years. The small baseline subset time-series technique was used for the interferometric processing of radar images acquired for the period of 2018–2020. The geospatial and statistical analyses allowed to determine the existing hotspots of the subsidence processes induced by oil extraction in the study area. Ground deformation measurements derived from the Sentinel-1 and COSMO-SkyMed satellite missions showed that the Tengiz oilfield continuously subsided during 2018–2020 with the maximum annual vertical displacement velocity around −77.4 mm/y and −71.5 mm/y, respectively. The vertical displacement velocities derived from the Sentinel-1 and the COSMO-SkyMed images showed a good statistical relationship with R2≥0.73 and RMSE ≤3.68 mm. The cumulative vertical displacement derived from both satellites for the most subsiding location also showed a good statistical relationship with R2 equal to 0.97 and RMSE = ± 4.69. The observed relative differences of measurements by both satellites were acceptable to determine the ongoing vertical surface displacement processes in the study area. These studies demonstrated a practical novelty for the petroleum industry in terms of the comparative assessment of surface displacement measurements using time-series of medium-resolution Sentinel-1 and high-resolution COSMO-SkyMed radar images.
Introduction
Tengiz oilfield is located at the coast of the Caspian Sea with low-lying wetlands. The recent successful studies by (Bayramov et al., 2021, Grebby et al., 2019 and Orynbassarova 2019) proved that the Tengiz oilfield was under the impact of ground deformation processes induced by the petroleum and gas operational activities.
Nowadays, industry significantly benefits from the ground deformation mapping as one of the important advancements for the surveillance programs of onshore oil and gas reservoirs. It is well known that the interferometric technologies were verified as effective for the measurement of the sensor-to-target line of sight projection of the ground deformation mapping of large onshore reservoirs rather than traditional in-situ measurements using geodetic tools (Zhou et al., 2006; Mirzaii et al., 2019; Bayramov et al., 2020a; Bayramov et al., 2020b; Bayramov et al., 2020c). Besides the Tengiz oilfield, other successful ground deformation studies which proved the effectiveness and quality of interferometric technologies had also been performed for the Karazhanbas oilfield, the Karaganda Coal Basin and other petroleum and mining sites in Kazakhstan (Junisbekova et al., 2016; Mozer et al., 2017; Togaibekov 2020). The precision of interferometric measurements is not comparable with the one achieved by geodetic measurements. However, in-situ geodetic measurements don’t provide the broad coverage of vertical displacements (Shi et al., 2019).
The studies by Grebby et al. (2019) and Orynbassarova (2019) clearly indicated the vertical displacements caused by oil and gas exploitation in the Tengiz oilfield during 2004–2009 and 2016–2017, by using Envisat and Sentinel-1 radar images, correspondingly. The investigations by Grebby et al. (2019) and Orynbassarova (2019) applied the Intermittent Small Baseline Subset (ISBAS) method from Sowter et al., 2013 which contributed to the determination of the continuously increasing cumulative vertical displacement (maximum of -79.3 mm/year) in the Tengiz oilfield during 2016–2017. ISBAS method was examined to be more effective for the increased density of ground deformation measurements.
The studies of Zhantaev et al. (2012) applied the Small Baseline Subset (SBAS) method from Berardino et al. (2002) for the observation of vertical displacements in the Tengiz oilfield using ENVISAT images acquired during 2004–2009 and ALOS PALSAR images acquired during 2007–2010.
Comola et al. (2016) and Del Conte et al. (2013) applied the SqueeSAR method from Ferretti et al. (2011a) to optimize the inputs for the geomechanical modeling of reservoir using Radarsat-1 satellite images acquired during 2004–2007. (Comola et al., 2016). proved that the integration of ground displacements into the geomechanical modeling principles would significantly contribute to the characterization of the hydrocarbon reservoir properties for the duration of its production life. (Katrenov et al., 2012). studied the influences to the reservoir compaction as a result of the pressure depletion along with the surface subsiding processes.
Recent studies by (Bayramov et al., 2021) focused on the differences of vertical (uplift and subsidence) and horizontal deformations (east-west and north-south) derived from 3D and 2D decompositions and cosine correction of line-of-sight (LOS) measurements in the Tengiz oilfield. These studies demonstrated a practical value for the operators to realize actual differences between InSAR line-of-sight and vertical measurements. These studies also demonstrated the benefits of measured horizontal movements to oil and gas operators in the analyses of the natural tectonic processes.
Based on the fore-mentioned studies it is possible to once again conclude about the ongoing Tengiz oilfield ground deformation processes caused by oil and gas industry.
To the extent of our awareness, there have been few publically accessible studies on the multi-satellite monitoring of vertical displacements in the Tengiz oilfield, in particular with the focus on the comparative assessment of vertical displacements derived from multiple Sentinel-1 and Cosmo-SkyMed Synthetic Aperture Radar (SAR) imagery. There were successful studies for the measurement of surface displacements in other Kazakhstan oil and gas and mining fields using multiple medium- and high-resolution radar sensors (Mozer et al., 2017; Pickering 2020; Togaibekov 2020). Therefore, multi-satellite interferometric analysis of recent vertical displacements would significantly contribute to the reliability assessment of the SAR measurements from different Earth observation missions in the Tengiz oilfield.
However there were many other related comparative studies between Sentinel-1 and Cosmo-SkyMed radar images which did not focus specifically on the oil and gas fields or study areas outside of Kazakhstan. The studies by (Costantini et al., 2017) allowed to determine that InSAR analyses based on the COSMO-SkyMed radar images provided higher deformation precision, density and 3D positioning in comparison to the wide coverage of Sentinel-1 images. The studies by (Çomut et al., 2016) demonstrated that a count of persistent scatterer points derived from the high-resolution COSMO-SkyMed was 3.5 times higher than from the medium-resolution Sentinel-1 radar images. The studies of (Ho Tong Minh et al., 2020) also demonstrated similar results with larger number of persistent scatterer points derived from COSMO-SkyMed images with higher spatial resolution in comparison to Sentinel-1 images. This was reflected in the higher root mean square difference and standard deviation of COSMO-SkyMed images in comparison to Sentinel-1 images. Based on these studies it is possible to conclude that InSAR analyses using both COSMO-SkyMed and Sentinel-1 provided identical cumulative vertical displacement but with a different density and precision of measurements.
Our research specifically focused on the assessment of vertical displacements derived from COSMO-SkyMed and Sentinel-1 SAR imagery using SBAS multi-temporal interferometric technique and geostatistical interpolation techniques followed by the cosine corrections to derive vertical movements from LOS measurements. The primary advantage of the present research is to quantitatively examine the differences in vertical displacement measurements using C-band Sentinel-1 and X-band Cosmo-SkyMed SAR imagery since in-situ geodetic measurements were not accessible for the present research.
The detailed goals of the present research are as follows:
1) SBAS-based detection of vertical displacement hotspots using COSMO-SkyMed and Sentinel-1 imagery acquired during 2018–2020
2) Quantitative analyses of the vertical displacement velocities and cumulative vertical displacement derived from Sentinel-1 and COSMO-SkyMed satellite images
3) Determination of spatial relationships between the detected patterns and the hotspots of vertical displacements, wells and faults
Data Processing
Research Area
Tengiz oilfield is one of the largest in the world and it is located at the coast of the Caspian Sea with an area of 2,500 km2 (Figure 1). The climate in the study area is semi-arid with temperature range −30 °C - 40° in summer and winter, respectively. The average annual precipitation is in the range of 100–200 mm (Klein et al., 2012). Tengiz field is located in the seismically active region of Kazakhstan. The seismic faults derived from (Anissimov et al., 2000) are indicated in Figure 1. According to (Grebby et al., 2019), the reservoir is estimated to be around 25 billion barrels of oil and is located at the depth of 3885–5117 m. High pressure with large proportion of gas was observed in the oil coming out of wells. The production is estimated to be 720,000 barrels per day. Sour gas injection enhanced oil recovery method is used in the Tengiz oilfield (Bealessio et al., 2020).
Quantitative Assessment of Vertical Displacement in the Tengiz Oilfeld Using SBAS Processing of Sentinel-1 and Cosmo-SkyMed Images
Sentinel-1 A/B TOPS with C-band (5.6 cm wavelength and 5.4 GHz) images from the European Space Agency (ESA) and COSMO-SkyMed (CSK) with X-band (3.1 cm wavelength and 9.6 GHz) from the Italian Space Agency were used for the present study to assess the vertical displacement velocities and cumulative vertical displacement in the Tengiz oilfield (Table 1). The extents of the Sentinel-1 and COSMO-SkyMed imagery are presented in Figure 2. The connection graphs of the Sentinel-1 images in Figures 3A,B and COSMO-SkyMed images in Figures 3C,D show that all radar images were well connected in time in order to follow the vertical displacement monitoring over the period of 2018–2020.
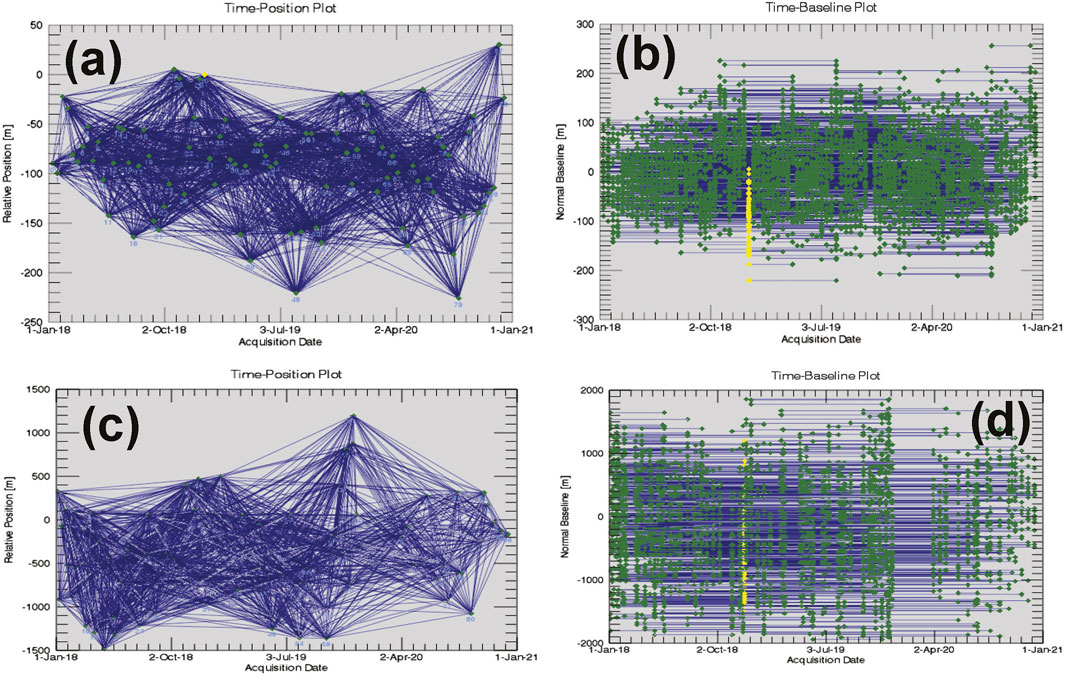
FIGURE 3. (A) Sentinel-1 time-position plot for SBAS; (B) Sentinel-1 time-baseline plot for SBAS; (C) COSMO-SkyMed time-position plot for SBAS; (D) COSMO-SkyMed time-baseline plot for SBAS.
96 Sentinel-1 images were acquired on descending Path 35, Frame 441, Absolute Orbit 30,557 in the TOPSAR Interferometric Wide Swath (IW) mode, with VV + VH Polarization between 1 January 2018 and 31 December 2020 (Table 1; Figure 2). VV polarization band of Sentinel-1 was used because of a higher coherence (Imamoglu et al., 2019). Sentinel-1 images in wide-swath mode provide a wide coverage of about 250 km with a range resolution of 5 m and an azimuth resolution of 20 m (Yang C et al., 2019).
87 COSMO-SkyMed images were acquired on descending Frame 2, Absolute Orbit 39,000 in the interferometric StripMap HIMAGE mode with HH polarization between 1 January 2018 and 31 December 2020. HH polarization band of COSMO-SkyMed that was only available had been used for the present research. COSMO-SkyMed images in StripMap Himage mode provide coverage of about 40 km with a range resolution of 3 m and an azimuth resolution of 3 m (Tapete and Cigna, 2019) (Table 1; Figure 2).
Descending tracks of Sentinel-1 and COSMO-SkyMed satellite images provided a complete coverage of the Tengiz oilfield. However in case of high-resolution COSMO-SkyMed satellite images (3 × 3 m), the processed area of interest was limited to the subsidence hotspot because of limited computing power and space.
SBAS - based interferometric processing technique was used for the assessment of the vertical displacement. SBAS workflow is presented in Figure 4 with the following processing steps: creation of a connection graph, definition of AOI, generation of interferograms, refinement and re-flattening, first inversion, second inversion and geocoding (Loesch and Sagan, 2018; Sarmap 2021). One arcsecond digital elevation model (DEM) with 30 m spatial resolution from the Shuttle Radar Topography Mission (SRTM) was applied for the correction of the topographic contribution to the radar phase (Farr et al., 2007). The ground control points (GCP) were collected for the refinement and ref-flattening stage on the unwrapped phase data with the careful consideration of the following criteria: no high frequency residual topography fringes, no displacement fringes preventing from being collected on the displacement areas, no phase jumps corresponding to unwrapping errors (Sarmap 2021). For the overlapping areas of Sentinel-1 and CSK interferometric stacks, GCPs were collected in the same locations to produce relatively accurate measurements for the reliability of further comparative analyses.
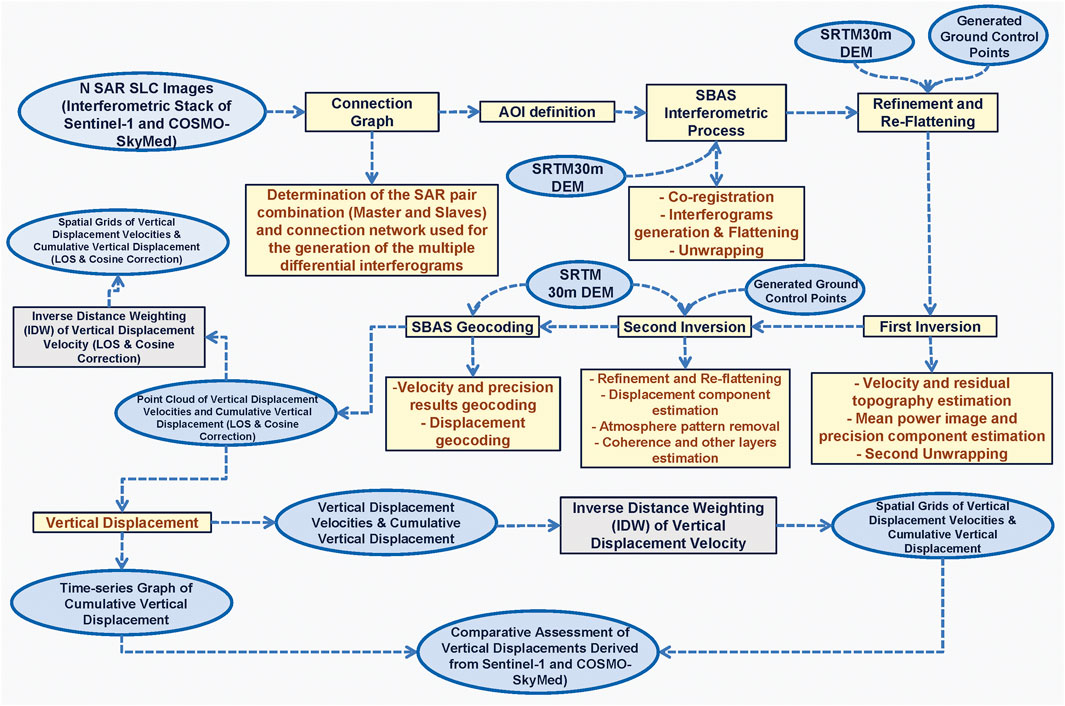
FIGURE 4. Workflow of SBAS interferometric processing followed by the cosine correction for the assessment of vertical displacements and spatial interpolations.
It is well known that the SAR interferometry can measure deformation in the LOS direction (Vassileva et al., 2017). As a significant limitation of the present study related to the restricted computing power needed for the processing of the high resolution COSMO-SkyMed images, the vertical displacement was only derived from a single track and cosine correction that means projecting the LOS deformation along the vertical direction considering the cosine of the local incidence angle. It was considered to be sufficient for the present study area since the studies by (Bayramov et al., 2021) showed the non-significant differences of vertical displacements derived from 3D and 2D decompositions of LOS measurements and cosine corrections in the Tengiz oilfield. However, it is necessary to emphasize that the use of the cosine correction approach may not be suitable for other oil and gas fields worldwide.
Cosine correction approach assumes that surface movements are vertical and neglects horizontal ones. The vertical displacement velocity using the cosine correction approach is derived through the division of the LOS movement rates by the cosine of the radar incidence angle as it is shown below in Eq. 1 (Gee et al., 2016; Yang Y.-J et al., 2019):
The produced LOS and vertical displacements from the Sentinel-1 and COSMO-SkyMed satellite images were interpolated using the Inverse Distance Weighting (IDW) method to achieve the continuous grid models. The interpolation was only applied for the points with the coherence value higher than 0.5 that corresponds to the threshold of reliable measurements. This deterministic method of interpolation contributed to the simplified interpretation and comparative overlay analysis of the vertical displacements by means of the geospatial analysis. The wells and seismic faults derived from (Anissimov et al., 2000) were used as the contextual information to analyze the spatial relationships between the detected hotspots of the vertical displacements and natural and man-made factors.
Results
LOS displacement velocity derived from the Sentinel-1 radar satellite images was observed to be in the range of −58.9–22.7 mm/y (Figure 5A). The vertical displacement velocity derived through the division of the LOS displacement rates by the cosine of the radar incidence angle is presented in Figure 5B with the range of −77.4–29.4 mm/y. The spatial distribution histogram of the measured LOS and vertical displacement velocities derived from the cosine corrections are presented in Figure 5C. It is possible to clearly observe the prevailing number of pixels with negative values which confirms the ongoing subsidence in the Tengiz oilfield.
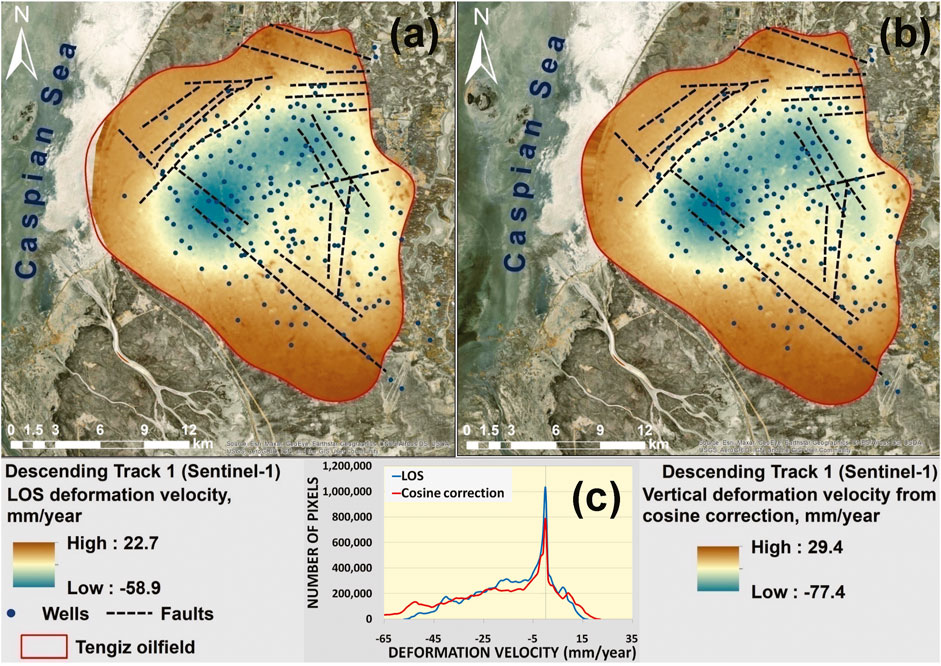
FIGURE 5. (A) LOS and (B) vertical displacement velocity from cosine correction of the Tengiz oilfield; (C) Histogram of LOS and vertical displacement velocity.
The hotspot of significant subsidence can be observed at the crossing of profile lines in Figure 6A. The same location was observed to be as the most subsiding. As it is possible to observe in Figure 6B, the maximum annual subsidence velocity along the profiles reaches about −70 mm/y. The cumulative vertical displacement is shown in Figure 7A with the range of −243.7–79.9 mm. The maximum cumulative vertical displacement along the profiles on 23 December 2020 reaches—235 mm (Figure 7B).
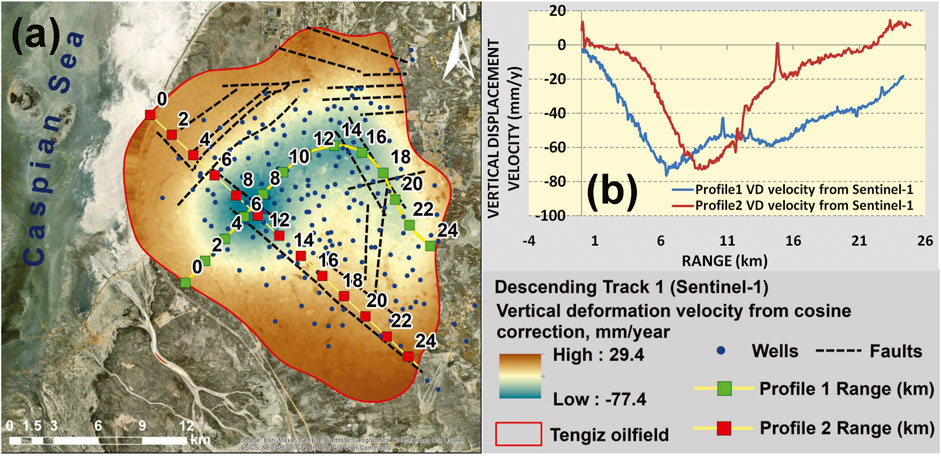
FIGURE 6. (A) Map of vertical displacement velocity derived from the Sentinel-1 images with the indication of profile lines; (B) profile lines of vertical displacement velocities.
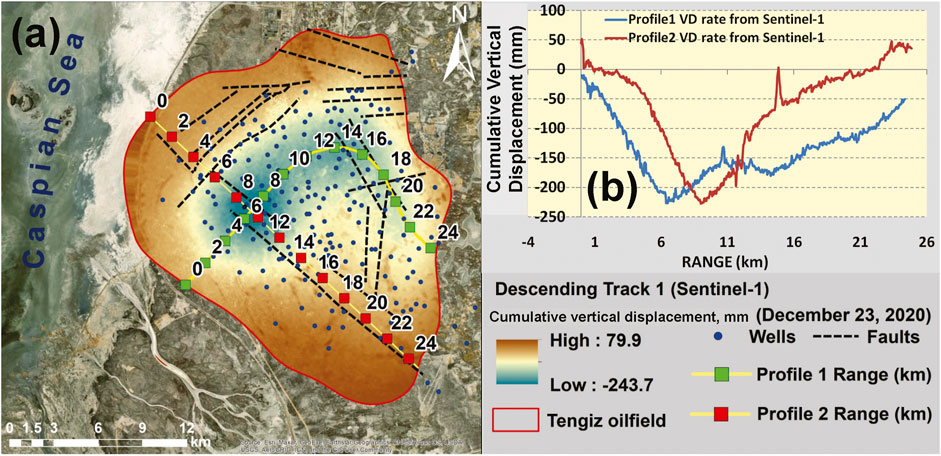
FIGURE 7. (A) Map of cumulative vertical displacement derived from the Sentinel-1 images with the indication of profile lines; (B) profile lines of cumulative vertical displacement.
Unfortunately, because of no accessibility to the historical in-situ high-precision geodetic measurements in the present research, it was not possible to validate the reliability and accuracy of the SBAS measurements derived from the Sentinel-1 images. Therefore, it was considered to use the COSMO-SkyMed images for the cross-validation of measurements from both satellites. The processed area of the COSMO-SkyMed images was limited to the subsiding hotspots derived from the processing of the Sentinel-1 images. The spatially interpolated vertical displacement velocity derived from the COSMO-SkyMed images is presented in Figure 8A. Figure 8B presents the spatial distribution of SBAS measured points derived from the COSMO-SkyMed and the Sentinel-1 images. These points with measured vertical velocities were used in the interpolation of spatial grids used in the comparative analysis (Figure 8A). The enhanced view of the vertical displacement velocity derived from the COSMO-SkyMed images and profile lines are presented in Figure 8C. The histogram of negative vertical displacement velocities derived from the COSMO-SkyMed images is presented in Figure 8D. This implies that the SBAS analyses using the COSMO-SkyMed images also showed the subsidence processes in the same hotspot detected by the processing of the Sentinel-1 images.
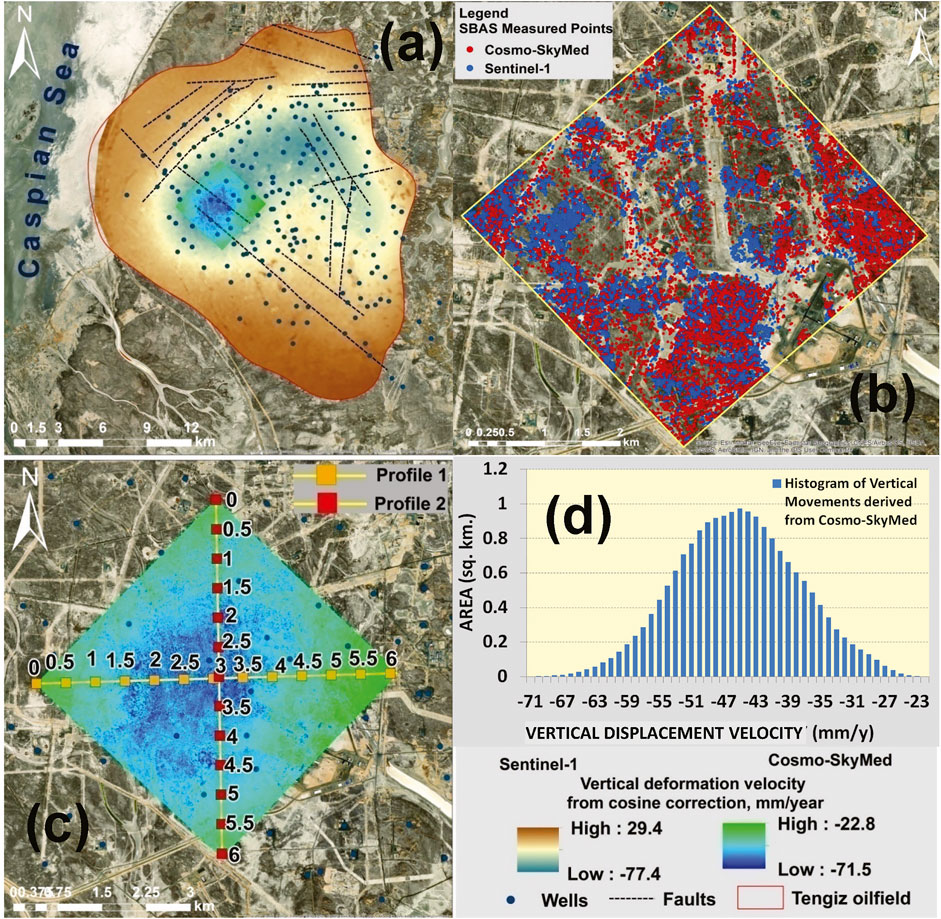
FIGURE 8. (A) Map of the spatially interpolated vertical displacement velocity derived from the Sentinel-1 and COSMO-SkyMed satellite images; (B) SBAS measured points from the Sentinel-1 and COSMO-SkyMed satellite images used for the spatial interpolation; (C) profile lines of vertical displacement velocity derived from the COSMO-SkyMed images; (D) histogram of vertical displacement velocities derived from the COSMO-SkyMed satellite images.
The profiles of the vertical displacement velocities derived from the Sentinel-1 and the COSMO-SkyMed images are presented in Figure 8C and Figures 9A–D. As it is possible to observe, the vertical displacement velocities derived from the Sentinel-1 and the COSMO-SkyMed images for the Profile 1 showed a good statistical relationship with R2 equal to 0.93, p-value < 0.05, RMSE equal to ±2.86 (Figures 9A,C). Good statistical relationship was also observed for the Profile 2 with R2 equal to 0.73, p-value < 0.05, RMSE equal to ±3.68 (Figures 9B,D). The standard deviation of the phase calculated over the profiles 1 and 2 was observed to be 8.9 for profile 1 and 7.9 for the profile 2 (Figures 9A,B).
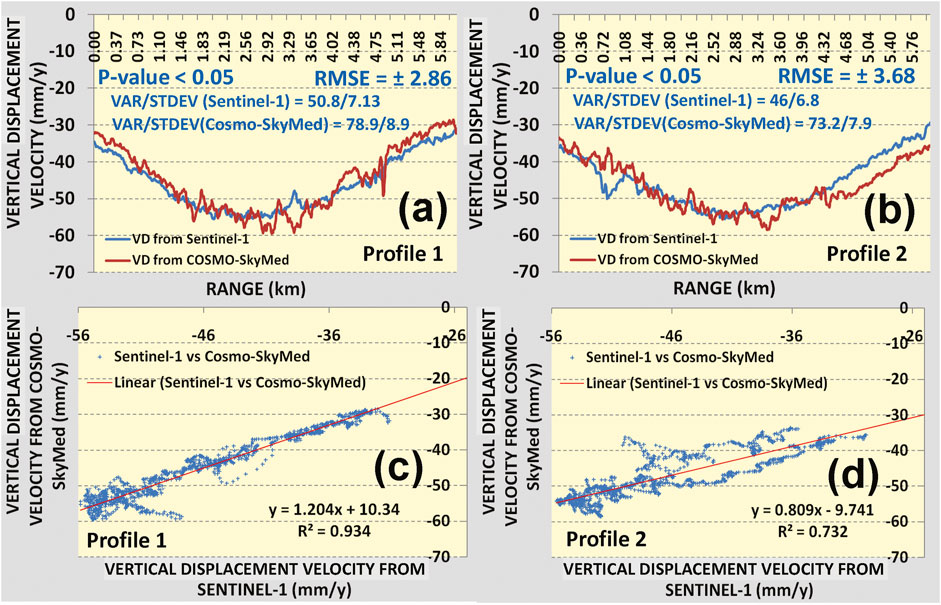
FIGURE 9. Vertical displacement velocity derived from the Sentinel-1 and COSMO-SkyMed satellite images for: (A) profile 1, (B) profile two; regression analysis between vertical displacement velocities derived from the Sentinel-1 and COSMO-SkyMed satellite images for: (C) profile 1, (D) profile two.
The location for the maximum subsidence derived from the Sentinel-1 images is presented in Figure 10A. The cumulative vertical displacement derived from the Sentinel-1 and the COSMO-SkyMed images for the most subsiding location are presented in Figure 10B. The cumulative vertical displacement in the most subsiding location reaches around 245 mm on 23 December 2020 (Figure 10B). The regression analysis between the cumulative vertical displacements derived from the Sentinel-1 and COSMO-SkyMed images for the most subsiding location showed a strong statistical relationship with R2 equal to 0.97. This was also reflected in RMSE = ± 4.69 and p-value < 0.05. This allows to conclude that SBAS produced identical results from the Sentinel-1 and the COSMO-SkyMed images. It is obvious that the ground deformation measurements from the COSMO-SkyMed images are more detailed because of the higher spatial resolution of images.
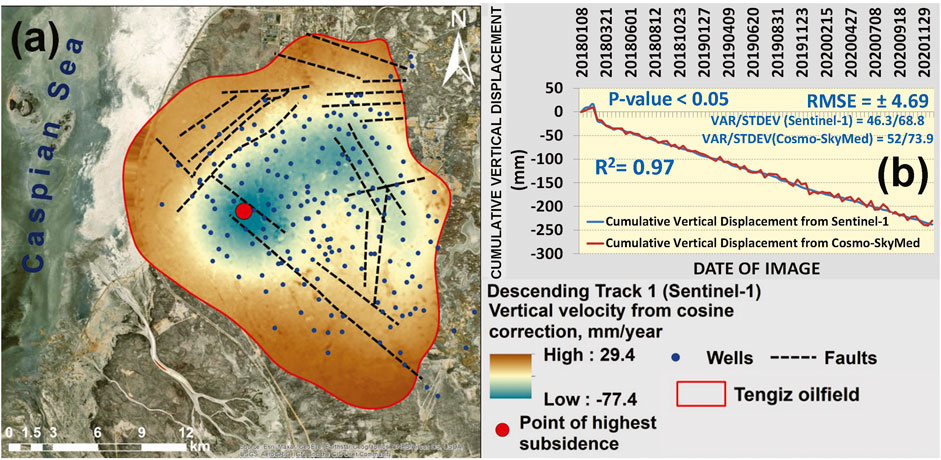
FIGURE 10. (A) Location of the most subsiding location based on the cumulative vertical displacement derived from the Sentinel-1 images; (B) regression analysis between the cumulative vertical displacements derived from the Sentinel-1 and COSMO-SkyMed satellite images for the most subsiding location.
Since the hotspot of the most subsiding areas is not anyhow spatially related to the concentration of wells, it is possible to assume that the primary factors controlling the vertical displacements in the Tengiz oilfield are the oil and gas extraction industrial processes and natural subsurface tectonics. However, it is possible to observe that a number of wells are located within the detected hotspot of the subsidence in Figure 8A. The oil terminal is located outside of the subsidence hotspot (Figure 1; Figure 8A) and it is obviously less vulnerable to the potential geohazards. Besides these areas are crossed by faults which might be subject to the potential reactivation (Figure 8A). Hence, some of the wells located within the detected subsidence hotspot should always be prioritized for the regular geotechnical and geohazard risk assessment.
Discussion
The assessment of the vertical displacement velocities and cumulative vertical displacement derived from the Sentinel-1 and the COSMO-SkyMed images showed a good statistical relationship. This allows to assume that the measurements by both satellites are reliable. The present research results also exhibit shortcomings related to the non-accessibility to the historical in-situ geodetic measurements to verify the accuracy of the measurements. Therefore this study used both Sentinel-1 and COSMO-SkyMed images to cross-validate the achieved results from both satellites. The qualitative judgment for the verification of the present research results was also based on the previously published studies which also observed and detected continuous subsidence processes in the Tengiz oilfield (Del Conte et al., 2013; Comola et al., 2016; Grebby et al., 2019; Orynbassarova 2019; Bayramov et al., 2021). The identical spatial deformation patterns and trends with a maximum subsidence rate rising up-to −79.3 mm/year were observed in the previous studies by Grebby et al., 2019 and Orynbassarova 2019.
Based on the published studies by (Rocca et al., 2013; Del Conte et al., 2013; Ferretti et al., 2011b), it is crucial to have a high-density and a high-precision of vertical displacement measurements to incorporate this critical parameter into the procedure of the reservoir characterization and the geomechanical analysis by oil and gas industry.
Another shortcoming is that only SBAS method was used for the present research. It would also be recommended to run the PSI method since it is proved to provide a high-precision of measurements for the oil and gas infrastructure (terminals, wells, pump stations etc.) considered as the persistently reflecting ground features (Ferretti et al., 2000; Ferretti et al., 2005; Lauknes et al., 2005; Hooper 2006; Ferretti et al., 2007).
Based on the published studies by Even et al., 2020, the vertical (uplift, subsidence) and horizontal (east-west, north-south) displacement derived from LOS measurements are critical as one of the parameters for the regular reservoir monitoring.
It is known that surface motions occur in three spatial dimensions (east, north and up) (Fuhrmann and Garthwaite, 2019). Many studies successfully used both descending and ascending tracks to assess actual vertical and horizontal displacements (Fialko 2006; Klemm et al., 2010; Chang et al., 2017; Liu et al., 2017; Motagh et al., 2017; Fernandez et al., 2018; Aslan et al., 2019a; Aslan 2019b; Yang C et al., 2019; Alatza et al., 2020; Ho Tong Minh et al., 2020; Pawluszek-Filipiak and Borkowski, 2020; Staniewicz et al., 2020). As it was mentioned before, the use of the cosine correction approach for the Tengiz oilfield was only decided based on the studies by Bayramov et al., 2021 which had shown non-significant differences of vertical displacements derived from the 3D and 2D decompositions and cosine corrections. This means that the cosine correction approach is suitable for the Tengiz oilfield on the basis of previously identified not significant rates of horizontal movements, but this may not be the case for other oil and gas fields worldwide.
(Tamburini et al., 2010) recommended to consider the reservoir depth to evaluate the usefulness of the interferometric measurements in conjunction with the subsurface measurements. This should also be considered in the future to understand the actual value of these measurements for oil and gas industry.
It is quite difficult to judge about the criticality level of the ongoing and increasing vertical displacement velocities and cumulative vertical displacement of the Tengiz oilfield subsidence in the relationship to the petroleum and gas industry standards for the effective risk management. However the previous studies by (Mahajan et al., 2018) indicated that the reservoir compaction cases in Oman resulted in the well damages, well integrity, and subsidence damage to the facilities of surface tremors because of fault reactivation. As it was possible to observe in the present studies, around 15 wells of the Tengiz oilfield are located in the detected subsidence hotspot from two satellites. Besides, it is necessary to emphasize the fact of the increasing subsidence velocity that was also well spotted by the present research and previous studies of (Grebby et al., 2019). The studies by (Nagel, 2001) indicated that the impact of reservoir compaction and subsidence are not always negative but may contribute to the possibilities for the increased production and also recovery. Hence as it was mentioned before, along with the prediction and mitigation of risks to petroleum and gas infrastructure, interferometric technologies play a significant role for the reservoir characterization and management (Grebby et al., 2019).
The application of Sentinel-1 or COSMO-SkyMed images should be selected depending on the scale of analysis, required density and precision of measurements.
Conclusion
The present research compared the vertical displacements derived from the Sentinel-1 and COSMO-SkyMed radar images collected during 2018–2020 for the Tengiz oilfield.
- The vertical displacement velocities and cumulative vertical displacement derived from the Sentinel-1 and COSMO-SkyMed images showed good agreement. The vertical displacement velocities derived from the Sentinel-1 and COSMO-SkyMed images showed a good statistical relationship with R2 equal to 0.93, P-value < 0.05 and RMSE equal to ±2.86 for the Profile one and R2 equal to 0.73, P-value < 0.05 and RMSE equal to ±3.68 for Profile 2. The cumulative vertical displacement derived from the Sentinel-1 and COSMO-SkyMed images for the most subsiding location showed a good statistical relationship with R2 equal to 0.97, P-value < 0.05 and RMSE = ± 4.69. This allowed to assume that the SBAS measurements from both satellites are reliable and also confirmed the ongoing subsidence processes in the Tengiz oilfield.
- Vertical displacement velocities and cumulative vertical displacement derived from the COSMO-SkyMed images were observed to be more dispersed than from the Sentinel-1 images. This was reflected in the higher sample variance and standard deviation of the vertical displacement values derived from the COSMO-SkyMed images.
- Ground deformations derived from the Sentinel-1 and COSMO-SkyMed images showed that the Tengiz oilfield subsided during 2018–2020 with the maximum annual vertical displacement velocity around −77.4 mm/y and −71.5 mm/y, respectively.
- The detected hotspot of the most subsiding areas was not anyhow spatially related to the concentration of wells. Therefore, it is possible to assume that primary factors controlling the vertical displacements in the Tengiz oilfield are the oil and gas extraction industrial processes and also natural subsurface tectonics.
- The selection among the Sentinel-1 or COSMO-SkyMed images should be decided depending on the scale of analysis, required density and precision of measurements.
Data Availability Statement
Publicly available datasets were analyzed in this study. This data can be found here European Space Agency Sentinel-1 Images (Open access to public) and Cosmo-SkyMed Images were granted for the research under the Open Call for Science Project ID 767. Acknowledgement to the Italian Space Agency is provided in the article.
Author Contributions
Scientific research and writing of original draft: EB Review and editing: MB and MK. AD and YZ: literature review and data collection.
Funding
This present research was funded by the Nazarbayev University through the Faculty-development Competitive Research Grant (FDCRGP)—Funder Project Reference: 080420FD1917. This project was carried out using CSK images of ASI (Italian Space Agency), delivered under an ASI license to use in the framework of COSMO-SkyMed Open Call for Science Project ID 767.
Conflict of Interest
The authors declare that the research was conducted in the absence of any commercial or financial relationships that could be construed as a potential conflict of interest.
The reviewer SI declared a shared affiliation with one of the authors, EB, to the handling editor at time of review.
Publisher’s Note
All claims expressed in this article are solely those of the authors and do not necessarily represent those of their affiliated organizations, or those of the publisher, the editors and the reviewers. Any product that may be evaluated in this article, or claim that may be made by its manufacturer, is not guaranteed or endorsed by the publisher.
Acknowledgments
The authors would like to thank Nazarbayev University. The author would like to acknowledge the Italian Space Agency (Agenzia Spaziale Italiana) for the provision of COSMO-SkyMed (CSK) images within the Open Call for Science Project ID 767.
References
Alatza, S., Papoutsis, I., Paradissis, D., Kontoes, C., and Papadopoulos, G. A. (2020). Multi-Temporal InSAR Analysis for Monitoring Ground Deformation in Amorgos Island, Greece. Sensors 20 (2), 338. doi:10.3390/s20020338
Anissimov, L., Postnova, E., and Merkulov, O. (2000). Tengiz Oilfield: Geological Model Based on Hydrodynamic Data. Pet. Geosci. 6, 59–65. doi:10.1144/petgeo.6.1.59
Aslan, G., Cakir, Z., Lasserre, C., and Renard, F. (2019a). Investigating Subsidence in the Bursa Plain, Turkey, Using Ascending and Descending Sentinel-1 Satellite Data. Remote Sensing 11, 85. doi:10.3390/rs11010085
Aslan, G. (2019b). “Monitoring of Surface Deformation in Northwest turkey from High-Resolution Insar: Focus on Tectonic a Seismic Slip and Subsidence,” in Tectonics. Istanbul Teknik Üniversitesi. English. ffNNT : 2019GREAU014ff. fftel02271771f.
Bayramov, E., Buchroithner, M., Kada, M., and Bayramov, R. (2020c). Quantitative Assessment of Ground Deformation Risks, Controlling Factors and Movement Trends for Onshore Petroleum and Gas Industry Using Satellite Radar Remote Sensing and Spatial Statistics. Georisk: Assess. Manage. Risk Engineered Syst. Geohazards, 1–18. doi:10.1080/17499518.2020.1806334
Bayramov, E., Buchroithner, M., and Kada, M. (2020a). Quantitative Assessment of Ground Deformations for the Risk Management of Petroleum and Gas Pipelines Using Radar Interferometry. Geomatics, Nat. Hazards Risk 11 (1), 2540–2568. doi:10.1080/19475705.2020.1853611
Bayramov, E., Buchroithner, M., and Kada, M. (2020b). Radar Remote Sensing to Supplement Pipeline Surveillance Programs through Measurements of Surface Deformations and Identification of Geohazard Risks. Remote Sensing 12 (23), 3934. doi:10.3390/rs12233934
Bayramov, E., Buchroithner, M., Kada, M., and Zhuniskenov, Y. (2021). Quantitative Assessment of Vertical and Horizontal Deformations Derived by 3D and 2D Decompositions of InSAR Line-Of-Sight Measurements to Supplement Industry Surveillance Programs in the Tengiz Oilfield (Kazakhstan). Remote Sensing 13, 2579. doi:10.3390/rs13132579
Bealessio, B. A., Blánquez Alonso, N. A., Mendes, N. J., Sande, A. V., and Hascakir, B. (2020). A Review of Enhanced Oil Recovery (EOR) Methods Applied in Kazakhstan. Petroleum 7 (1), 1–9.
Berardino, P., Fornaro, G., Lanari, R., and Sansosti, E. (2002). A New Algorithm for Surface Deformation Monitoring Based on Small Baseline Differential SAR Interferograms. IEEE Trans. Geosci. Remote Sensing 40 (11), 2375–2383. doi:10.1109/tgrs.2002.803792
Chang, Z., Yu, W., Wang, W., Zhang, J., Liu, X., and Zhu, J. (2017). An Approach for Accurately Retrieving the Vertical Deformation Component from Two-Track InSAR Measurements. Int. J. Remote Sensing 38 (6), 1702–1719. doi:10.1080/01431161.2017.1285448
Comola, F., Janna, C., Lovison, A., Minini, M., Tamburini, A., and Teatini, P. (2016). Efficient Global Optimization of Reservoir Geomechanical Parameters Based on Synthetic Aperture Radar-Derived Ground Displacements. Geophysics 81 (3), M23–M33. doi:10.1190/geo2015-0402.1
Çomut, F. C., Ustun, A., Lazecký, M., and Perissin, D. (2016). “Capability of Detecting Rapid Subsidence with COSMO SKYMED and Sentinel-1 Dataset over Konya City,” in Proceedings of Living Planet Symposium 2016, Prague, Czech Republic.
Costantini, M., Malvarosa, F., Minati, F., Trillo, F., and Vecchioli, F. (2017). “Complementarity of High-Resolution COSMO-SkyMed and Medium-Resolution Sentinel-1 SAR Interferometry: Quantitative Analysis of Real Target Displacement and 3D Positioning Measurement Precision, and Potential Operational Scenarios,” in 2017 IEEE International Geoscience and Remote Sensing Symposium (IGARSS). doi:10.1109/igarss.2017.8128026
Del Conte, S., Tamburini, A., Cespa, S., Rucci, A., and Ferretti, A. (2013). “Advanced InSAR Technology for Reservoir Monitoring and Geomechanical Model Calibration,” in SPE Kuwait Oil and Gas Show and Conference. doi:10.2118/167339-ms
Even, M., Westerhaus, M., and Simon, V. (2020). Complex Surface Displacements above the Storage Cavern Field at Epe, NW-Germany, Observed by Multi-Temporal SAR-Interferometry. Remote Sensing 12 (20), 3348. doi:10.3390/rs12203348
Farr, T., Rosen, P., Caro, E., Crippen, R., Duren, R., Hensley, S., et al. (2007). The Shuttle Radar Topography mission. Rev. Geophys. 45. doi:10.1029/2005rg000183
Fernandez, J., Prieto, J. F., Escayo, J., Camacho, A. G., Luzón, F., Tiampo, K. F., et al. (2018). Modeling the Two- and Three-Dimensional Displacement Field in Lorca, Spain, Subsidence and the Global Implications. Sci. Rep. 8, 14782. doi:10.1038/s41598-018-33128-0
Ferretti, A., Fumagalli, A., Novali, F., Prati, C., Rocca, F., and Rucci, A. (2011a). A New Algorithm for Processing Interferometric Data-Stacks: SqueeSAR. IEEE Trans. Geosci. Remote Sensing 49 (9), 3460–3470. doi:10.1109/tgrs.2011.2124465
Ferretti, A., Prati, C., Rocca, F., Casagli, N., Farina, P., and Young, B. (2005). Permanent Scatterers Technology: A Powerful State of the Art Tool for Historic and Future Monitoring of Landslides and Other Terrain Instability Phenomena. Vancouver: Proceedings of the International landslide Symposium – ISL2005.
Ferretti, A., Prati, C., and Rocca, F. (2000). Nonlinear Subsidence Rate Estimation Using Permanent Scatterers in Differential SAR Interferometry. IEEE Trans. Geosci. Remote Sensing 38 (5), 2202–2212. doi:10.1109/36.868878
Ferretti, A., Prati, C., and Rocca, F. (2001). Permanent Scatterers in SAR Interferometry. IEEE Trans. Geosci. Remote Sensing 39 (8–20). doi:10.1109/36.898661
Ferretti, A., Savio, G., Barzaghi, R., Borghi, A., Musazzi, S., Novali, F., et al. (2007). Submillimeter Accuracy of InSAR Time Series: Experimental Validation. IEEE Trans. Geosci. Remote Sensing 45 (5), 1142–1153. doi:10.1109/tgrs.2007.894440
Ferretti, A., Tamburini, A., Novali, F., Fumagalli, A., Falorni, G., and Rucci, A. (2011b). Impact of High Resolution Radar Imagery on Reservoir Monitoring. Energ. Proced. 4, 3465–3471. doi:10.1016/j.egypro.2011.02.272
Fialko, Y. (2006). Interseismic Strain Accumulation and the Earthquake Potential on the Southern San Andreas Fault System. Nature 441 (7096), 968–971. doi:10.1038/nature04797
Fuhrmann, T., and Garthwaite, M. C. (2019). Resolving Three-Dimensional Surface Motion with InSAR: Constraints from Multi-Geometry Data Fusion. Remote Sensing 11 (3), 241. doi:10.3390/rs11030241
Gee, D., Sowter, A., Novellino, A., Marsh, S., and Gluyas, J. (2016). Monitoring Land Motion Due to Natural Gas Extraction: Validation of the Intermittent SBAS (ISBAS) DInSAR Algorithm over Gas fields of North Holland, The Netherlands. Mar. Pet. Geology. 77, 1338–1354. doi:10.1016/j.marpetgeo.2016.08.014
Grebby, S., Orynbassarova, E., Sowter, A., Gee, D., and Athab, A. (2019). Delineating Ground Deformation over the Tengiz Oil Field, Kazakhstan, Using the Intermittent SBAS (ISBAS) DInSAR Algorithm. Int. J. Appl. Earth Observation Geoinformation 81, 37–46. doi:10.1016/j.jag.2019.05.001
Ho Tong Minh, D., Ngo, Y., Lê, T. T., Le, T. C., Bui, H. S., Vuong, Q. V., et al. (2020). Quantifying Horizontal and Vertical Movements in Ho Chi Minh City by Sentinel-1 Radar Interferometry. Preprints, 2020120382.
Hooper, A. (2006). Persistent Scatterer Radar Interferometry for Crustal Deformation Studies and Modeling of Volcanic DeformationPhD Dissertation. Stanford University.
Imamoglu, M., Kahraman, F., Cakir, Z., and Sanli, F. B. (2019). Ground Deformation Analysis of Bolvadin (W. Turkey) by Means of Multi-Temporal InSAR Techniques and Sentinel-1 Data. Remote Sensing 11, 1069. doi:10.3390/rs11091069
Junisbekova, V., Filatov, A., Kuznetsova, I., and Yelisseyeva, A. (2016). SAR Interferometry Technique for Ground Deformation Assessment on Karazhanbas Oilfield. Proced. Comput. Sci. 100, 1163–1167.
Katrenov, Z., Tlepbergenov, N., Flodin, E., Buchmann, T., and Connolly, P. (2012). “Mechanical Earth Modeling of the Tengiz Field,” in Conference Proceedings, 2nd EAGE International Conference KazGeo, Oct 2012. cp-315-00043. doi:10.3997/2214-4609.20142932
Klein, I., Gessner, U., and Kuenzer, C. (2012). Regional Land Cover Mapping and Change Detection in Central Asia Using MODIS Time-Series. Appl. Geogr. 35, 219–234. doi:10.1016/j.apgeog.2012.06.016
Klemm, H., Quseimi, I., Novali, F., Ferretti, A., and Tamburini, A. (2010). Monitoring Horizontal and Vertical Surface Deformation over a Hydrocarbon Reservoir by PSInSAR. First Break 28, 5. doi:10.3997/1365-2397.2010014
Lauknes, T. R., Dehls, J., Larsen, Y., Høgda, K. A., and Weydahl, D. J. (2005). “A Comparison of SBAS and PS ERS InSAR for Subsidence Monitoring in Oslo, Norway,” in Proceedings of the Fringe 2005 Workshop, ESA ESRIN, Frascati, Italy, 28 November–2 December 2005.
Liu, X., Hu, J., Sun, Q., Li, Z., and Zhu, J. (2017). Deriving 3-D Time-Series Ground Deformations Induced by Underground Fluid Flows with InSAR: Case Study of Sebei Gas Fields, China. Remote Sensing 9, 1129. doi:10.3390/rs9111129
Loesch, E., and Sagan, V. (2018). SBAS Analysis of Induced Ground Surface Deformation from Wastewater Injection in East Central Oklahoma, USA. Remote Sensing 10, 283. doi:10.3390/rs10020283
Mahajan, S., Hassan, H., Duggan, T., and Dhir, R. (2018). “Compaction and Subsidence Assessment to Optimize Field Development Planning for an Oil Field in Sultanate of Oman,” in Dhabi International Petroleum Exhibition & Conference, Abu Dhabi, November 2018 (UAE). Paper presented at the Abu. doi:10.2118/192744-MS
Mirzaii, Z., Hasanlou, M., Samieie-Esfahany, S., Rojhani, M., and Ajourlou, P. (2019). Land Subsidence Monitoring in Azar Oil Field Based on Time Series Analysis. Proceedings 18 (1), 2. doi:10.3390/ecrs-3-06190
Motagh, M., Shamshiri, R., Haghshenas Haghighi, M., Wetzel, H.-U., Akbari, B., Nahavandchi, H., et al. (2017). Quantifying Groundwater Exploitation Induced Subsidence in the Rafsanjan plain, southeastern Iran, Using InSAR Time-Series and In Situ Measurements. Eng. Geology. 218, 134–151. doi:10.1016/j.enggeo.2017.01.011
Mozer, D. V., Tuyakbai, A. S., and Toleubekova, Z. Z. (2017). State of the Undermined Karaganda Coal Basin Area by Satellite Monitoring Data. J. Min Sci. 53, 389–395. doi:10.1134/s1062739117022271
Nagel, N. B. (2001). Compaction and Subsidence Issues within the Petroleum Industry: From wilmington to Ekofisk and beyond. Phys. Chem. Earth, A: Solid Earth Geodesy 26 (1–2). doi:10.1016/s1464-1895(01)00015-1
Orynbassarova, E. (2019). Improvement of the Method of Integrated Preparation and Use of Space Images in Tasks of Assessment of Sedimentation of Industrial Surface in the Conditions of Operation of Tengiz Oil and Gas fieldPhD Dissertation. Satbayev University.
Pawluszek-Filipiak, K., and Borkowski, A. (2020). Integration of DInSAR and SBAS Techniques to Determine Mining-Related Deformations Using Sentinel-1 Data: The Case Study of Rydułtowy Mine in Poland. Remote Sensing 12, 242. doi:10.3390/rs12020242
Pickering, M. V. (2020). Improved Reservoir Characterization by Incorporating Geodetic Data in a Western Kazakhstan oilfieldMSc Thesis. Massachusetts Institute of Technology.
Rocca, F., Rucci, A., Ferretti, A., and Bohane, A. (2013). Advanced InSAR Interferometry for Reservoir Monitoring. First Break 31, 77–85. doi:10.3997/1365-2397.31.5.68075
Sarmap, (2021). SBAS Tutorial. Available at: https://www.sarmap.ch/index.php/software/sarscape.
Shi, J., Yang, H., Peng, J., Wu, L., Xu, B., Liu, Y., et al. (2019). InSAR Monitoring and Analysis of Ground Deformation Due to Fluid or Gas Injection in Fengcheng Oil Field, Xinjiang, China. J. Indian Soc. Remote Sens 47, 455–466. doi:10.1007/s12524-018-0903-y
Sowter, A., Bateson, L., Strange, P., Ambrose, K., and Syafiudin, M. F. (2013). DInSAR Estimation of Land Motion Using Intermittent Coherence with Application to the South Derbyshire and Leicestershire Coalfields. Remote Sensing Lett. 4 (10), 979–987. doi:10.1080/2150704x.2013.823673
Staniewicz, S., Chen, J., Lee, H., Olson, J., Savvaidis, A., Reedy, R., et al. (2020). InSAR Reveals C Omplexsurface Deformation Patterns over An80,000 Km2oil-Producing Region in thePermian Basin. Geophys. Res. Lett. 47, e2020GL090151. doi:10.1029/2020gl090151
Tamburini, A., Bianchi, M., Giannico, C., and Novali, F. (2010). Retrieving Surface Deformation by PSInSAR Technology: A Powerful Tool in Reservoir Monitoring. Int. J. Greenhouse Gas Control. 4 (6), 928–937. doi:10.1016/j.ijggc.2009.12.009
Tapete, D., and Cigna, F. (2019). COSMO-SkyMed SAR for Detection and Monitoring of Archaeological and Cultural Heritage Sites. Remote Sensing 11 (11), 1326. doi:10.3390/rs11111326
Togaibekov, A. Z. (2020). Monitoring of Oil-Production-Induced Subsidence and Uplift. MSc Thesis. Massachusetts Institute of Technology.
Vassileva, M., Giulio Tonolo, F., Riccardi, P., Lecci, D., Boccardo, P., and Chiesa, G. (2017). Satellite SAR Interferometric Techniques in Support to Emergency Mapping. Eur. J. Remote Sensing 50 (1), 464–477. doi:10.1080/22797254.2017.1360155
Yang, C., Zhang, D., Zhao, C., Han, B., Sun, R., Du, J., et al. (2019). Ground Deformation Revealed by Sentinel-1 MSBAS-InSAR Time-Series over Karamay Oilfield, China. Remote Sensing 11, 2027. doi:10.3390/rs11172027
Yang, Y.-J., Hwang, C., Hung, W.-C., Fuhrmann, T., Chen, Y.-A., and Wei, S.-H. (2019). Surface Deformation from Sentinel-1A InSAR: Relation to Seasonal Groundwater Extraction and Rainfall in Central Taiwan. Remote Sensing 11, 2817. doi:10.3390/rs11232817
Zhantaev, J., Fremd, A., Ivanchukova, A., Kaldybayev, A., Nurakynov, S., Kantemirov, Y., et al. (2012). Satellite Radar Monitoring of Land Surface Subsidence over Tengiz Oil and Gas Field. Geomatics Mag. 1 (14), 61–71. [In Russian].
Keywords: remote sensing, geospatial, oil reservoir, SAR, InSAR, COSMO-SkyMed, Sentinel-1
Citation: Bayramov E, Buchroithner M, Kada M, Duisenbiyev A and Zhuniskenov Y (2022) Multi-Temporal SAR Interferometry for Vertical Displacement Monitoring from Space of Tengiz Oil Reservoir Using SENTINEL-1 and COSMO-SKYMED Satellite Missions. Front. Environ. Sci. 10:783351. doi: 10.3389/fenvs.2022.783351
Received: 27 September 2021; Accepted: 22 February 2022;
Published: 14 March 2022.
Edited by:
Antonio Pepe, National Research Council (IREA-CNR), ItalyReviewed by:
Mukesh Gupta, Catholic University of Louvain, BelgiumSonny Irawan, Nazarbayev University, Kazakhstan
Giulia Tessari, Sarmap Sa, Switzerland
Copyright © 2022 Bayramov, Buchroithner, Kada, Duisenbiyev and Zhuniskenov. This is an open-access article distributed under the terms of the Creative Commons Attribution License (CC BY). The use, distribution or reproduction in other forums is permitted, provided the original author(s) and the copyright owner(s) are credited and that the original publication in this journal is cited, in accordance with accepted academic practice. No use, distribution or reproduction is permitted which does not comply with these terms.
*Correspondence: Emil Bayramov, ZW1pbC5iYXlyYW1vdkBudS5lZHUua3o=