- 1Key Laboratory of Geographical Processes and Ecological Security in Changbai Mountains, Ministry of Education, School of Geographical Sciences, Northeast Normal University, Changchun, China
- 2State Environmental Protection Key Laboratory of Wetland Ecology and Vegetation Restoration, Institute for Peat and Mire Research, Northeast Normal University, Changchun, China
- 3Jilin Provincial Joint Key Laboratory of Changbai Mountain Wetland and Ecology, Department of Science and Technology of Jilin Province, Changchun, China
Peatland areas have dramatically declined in the past century because of the demand for agriculture. Therefore, it is necessary to develop suitable techniques to preserve these unique ecosystems. We studied the effects of topsoil removal on vegetation restoration in silt- and sand-amended peatlands in Changbai Mountain, China. We observed that topsoil removal effectively improved soil nutrient levels and water holding capacity in the silt-amended peatland but exhibited no significant effect on the sand-amended peatland. Topsoil removal decreased the species richness in both silt- and sand-amended peatlands but did not have any effect on the plant cover and biomass in the sand-amended peatland. The coverage, density, and aboveground biomass of dominant species, namely, Carex schmidtii, significantly increased after topsoil removal in the silt-amended peatland. The target Carex species was absent from the sand-amended peatland. Redundancy analysis identified that the soil water content, soil organic carbon, total nitrogen, and total phosphorus explained the most variance in vegetation composition in the silt-amended peatland. Our results demonstrated that topsoil removal is necessary to reduce the weed seeds and promote the recolonization of peatland species, particularly the tussock-forming Carex, in the silt-amended peatland during restoration.
1 Introduction
Peatlands play critical roles in the global carbon cycle, water resource regulation, and biodiversity conservation (Yu, 2011; Verhoeven, 2014). However, peatland areas have been dramatically reduced in the past century because of the change in land usage, drainage, and other forms of anthropogenic activities (Hallema et al., 2015). Agricultural cultivation is one of the most important reasons contributing to peatland degradation (Frolking et al., 2011). Agricultural cultivation not only degrades the native vegetation but also changes the hydrological conditions and physicochemical characteristics of soil in peatlands (Berglund and Berglund, 2010; Kløve et al., 2010; Heller and Zeitz, 2012; Leifeld et al., 2019), which have severely compromised their functions and services (Foley et al., 2005).
Regeneration of dominant plant species is necessary for the recovery of the ecological function of degraded peatlands (Emsens et al., 2015; Wang et al., 2021; Zhang et al., 2022). Carex species is the foundation species in sedge peatlands. C. schmidtii dominates peatlands in northeast China and forms tussocks that have a carbon storage function (Wang et al., 2021) and engineer communities by enhancing microtopography and supporting biodiversity (Wang et al., 2019). However, many studies reported that Carex species are seldom recovered by natural regeneration, particularly when farmed for more than 10 years (Wang G et al., 2017).
Topsoil removal not only improves soil conditions but also eliminates non-target existing vegetation and weeds from soil seed banks (Beas et al., 2013; Giannini et al., 2019; Henriksson et al., 2019). Topsoil removal is proved to be an effective way for vegetation restoration in forests, grasslands, and wetland ecosystems (Beas et al., 2013; Soto and Puettmann, 2018; Resch et al., 2019; Řehounková et al., 2020). Many studies in peatland ecosystems have conducted topsoil removal as an attempt to achieve vegetation restoration (Emsens et al., 2015; Harpenslager et al., 2015; Giannini et al., 2019). However, the ecological results of this restoration practice differ among studies (Verhagen et al., 2001; Klimkowska et al., 2010). Some evidence states that the recovery of peatland vegetation, particularly the dominant Carex species, was largely dependent on soil seed banks and soil environmental conditions (Wang et al., 2020). Peatland soils were usually amended to increase the load-bearing capacity of soil and balance soil pH during farming, and the addition of sand and silt was the most frequent soil amendment (Zaidel′man et al., 2001). These materials are homogenized with surface soil horizons or were leached into the deeper horizons during cultivation on peatlands (Kalisz et al., 2021). Former studies reported that the soil amendment type significantly affected soil physical properties, soil nutrients, and the size of soil seed banks (Zakharova et al., 2020; Kalisz et al., 2021; Smolczynski et al., 2021). However, poor information is available on whether topsoil removal can help in restoring the target species, such as Carex species, and how soil amendment types affect vegetation restoration in farmed peatlands during restoration.
Changbai Mountain is one of the largest peatlands in China (Ma et al., 2013). However, large areas of peatlands have been farmed as paddy fields in this region since 1950s, which resulted in a dramatic decline in biodiversity and ecological services (Wang et al., 2020). To protect the peatland, the Jilin Provincial Government of China developed plans for peatland restoration of > 6,000 ha of farmlands in Changbai Mountain. In this study, we assessed the effects of topsoil removal on vegetation restoration in silt- and sand-amended peatlands. We investigated how soil environmental factors change after topsoil removal, whether the target peatland species is successfully established after topsoil removal, and whether the topsoil removal have the same effect on vegetation restoration between silt- and sand-amended peatlands.
2 Materials and methods
2.1 Study site
The silt- and sand-amended peatland sites were located in Changbai Mountain, northeast China (Figure 1). The region has a temperate continental monsoonal climate, and the frost-free period was approximately 138 days during each year. The annual mean precipitation is 737.4 mm, and the annual mean temperature is approximately 5°C (Wang et al., 2021). The silt-amended peatland site is located in Dayishan Town, Huinan County, Tonghua City, Jilin Province (126°13′10″E, 42°16′35″N, 456 m a.s.l). The peat thickness was 0.5–1 m. C. schmidtii was the dominant species before farming. In the 1980s, most of the peatlands in these regions were drained and filled with silt soil to increase the load-bearing capacity of the soil and balance pH for agricultural purposes. As part of the soil amendment process, approximately 10 cm of silt was covered on the surface of the peatlands. The sand-amended peatland was located in Sipeng town, Tonghua City, Jilin Province (125°34′44″E, 41°51′22″N, 560 m a.s.l). The peat thickness in this study site ranges from 0.6 m to 1.0 m. Peatlands in these regions were drained and intensively reclaimed to paddy fields in the 2000s, with the introduction of sand in the plowed horizon and mixing it with peat while plowing. Peatland restoration was conducted in April 2019. At each peatland site, two types of experimental treatments were performed close to each other: topsoil removal (removing 10 cm topsoil layer from the area of 0.5 h m2) and control (no action on the topsoil on the area of 0.5 h m2). The water level was kept at 0–10 cm below the soil surface in all the treatments.
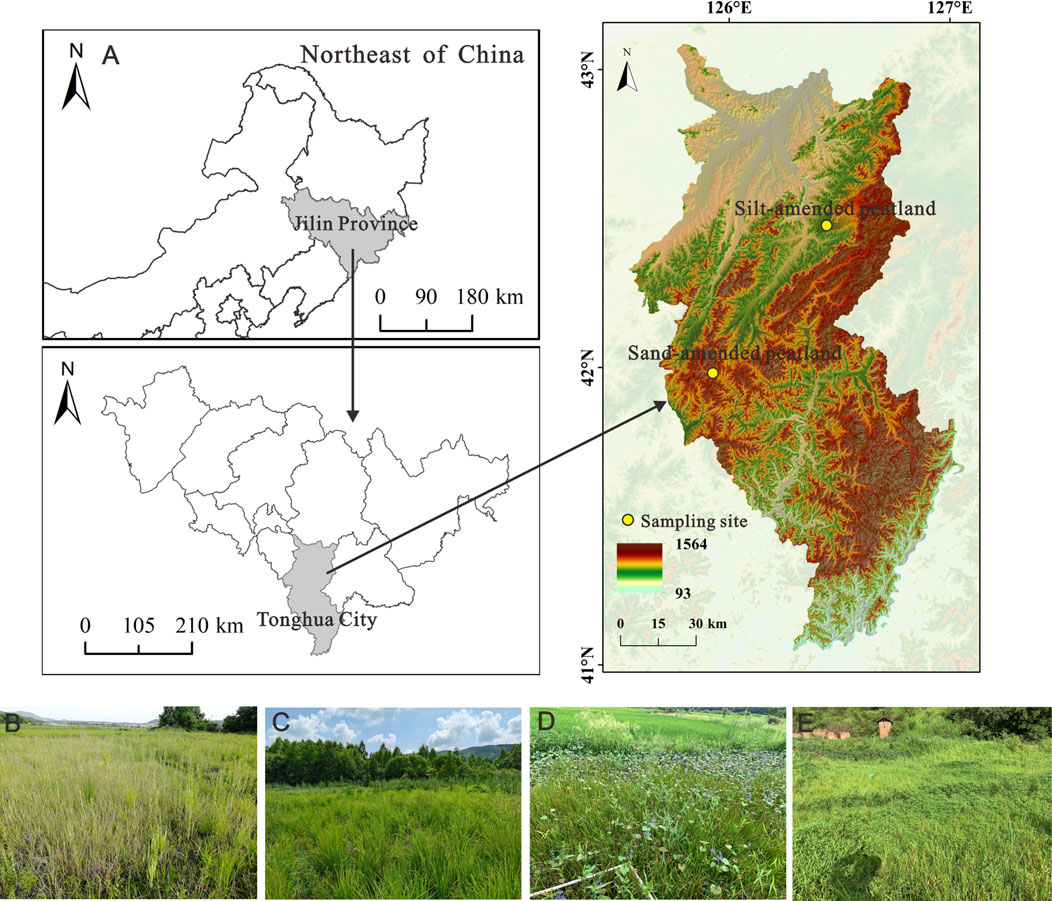
FIGURE 1. Site locations (A) of the silt-amended peatlands (control treatment plots) (B), topsoil removal treatment plots, (C) sand-amended peatlands (control treatment plots) (D), and topsoil removal treatment plots (E) in the Changbai Mountain region.
2.2 Field vegetation survey
Field vegetation survey was performed in July 2021. Four replicate plots were randomly selected at each site. Within each plot (≈.1 h m2), five quadrats (1 m × 1 m) were randomly sampled, which were more than 10 m away from each other. We investigated plant community characteristics including total coverage, aboveground biomass, and the height, density, and percentage cover of each species. In addition, we recorded characteristics of dominant Carex species including height, basal diameter, root length, tiller number, and above- and below-ground biomass. Vegetation was harvested using a sickle and dried at 65°C, and the dried biomass was weighed.
2.3 Soil sampling
Soil samples were collected from both treatment plots at each site. Four soil cores (depth 30 cm, interval 10 cm, and diameter 5 cm) were collected from each plot to measure the soil bulk density (BD), soil water content (SWC), soil organic carbon (SOC), soil total phosphorus (TP), soil total nitrogen (TN), and soil pH. BD was determined using the volumetric core method. SWC was determined using the gravimetric method (Jackson et al., 2000). SOC was determined using the dichromate oxidation method (Kalembasa and Jenkinson, 1973). TP and TN were measured using the Kjeldahl and molybdenum blue methods, respectively. Soil pH was determined using a glass electrode with a soil-to-water ratio of 1:10.
2.4 Statistical analysis
Species richness, the Shannon–Wiener Index, Pielou Index, Margalef Index, and Simpson Index were evaluated to describe plant diversity in each plot.
Species richness:
Shannon–Wiener index:
Margalef Index:
Pielou Index:
Simpson Index:
Where, S is the species number within the quadrat, N is the total number of individuals, and Pi is the importance value of the ith species; Pi is (the relative height of the ith species + relative cover of the ith species + relative abundance of the ith species)/3.
One-way ANOVA, followed by multiple comparisons using the LSD test, was performed to study the differences in soil properties and plant community characteristics between the two treatments at the same site and between the silt- and sand-amended sites for the same treatment. The significance level of p < .05 was considered statistically significant. Redundancy analysis (RDA) was conducted to study the correlation between vegetation composition and soil environmental factors using Canoco 5.0 (Microcomputer Power, Ithaca, NY, United States).
3 Results
3.1 Effects of topsoil removal on soil environmental factors
Soil environmental factors responded differently to topsoil removal between the two peatland sites. At the silt-amended peatland site, SOC, TP, TN, and SWC in the topsoil-removed plot were higher than those in the control plot (p < .05). No significant difference was observed in terms of BD, pH, and C: N between the two treatments.
3.2 Effects of topsoil removal on standing vegetation
Plant community coverage and aboveground biomass in the topsoil-removed plots were higher than those in the control plots at the silt-amended peatland site (p < .05; Figure 2A). No significant difference was observed in terms of plant community coverage and aboveground biomass between the two treatments at the sand-amended peatland site (Figure 2B).
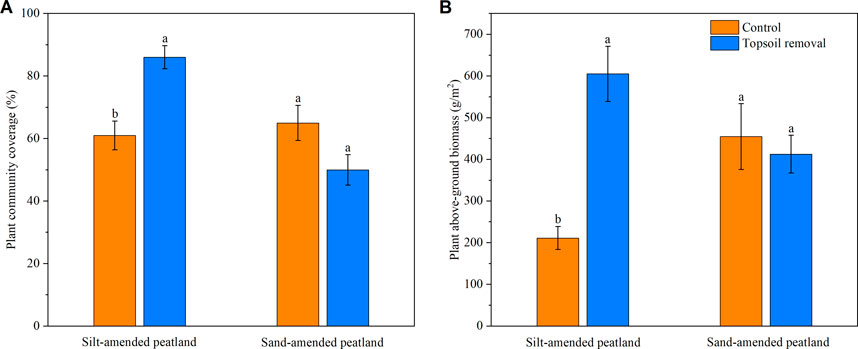
FIGURE 2. Plant community coverage (A) and aboveground biomass (B) of silt-amended and sand-amended peatlands. Different lowercase letters indicate significant differences between the two treatment plots within the same peatland site (p < .05).
At the silt- and sand-amended peatland sites, 23 and 18 species were recorded in the topsoil-removed plots and 32 and 22 species were recorded in the control plots, respectively (Table 2). Non-peatland species, such as Alopecurus aequalis and Echinochloa crus-galli, were not observed in the topsoil-removed plots at the silt-amended peatland site, and the dominant species C. schmidtii had a higher importance value (0.577) than the control plots (0.131). However, Carex species was not observed at the sand-amended peatland site. At this site, non-peatland species such as Eleocharis wichurae and Echinochloa crus-galli dominated in the control and topsoil-removed plots, respectively.
The species richness, Shannon–Wiener Index, Pielou Index, Margalef Index, and Simpson Index were lower in the topsoil-removed plots than in the control plots at the silt-amended peatland site (Figure 3). However, no significant difference existed between the two treatments at the sand-amended peatland site in terms of species richness and aforementioned indexes, except the Margalef Index (Figure 3).
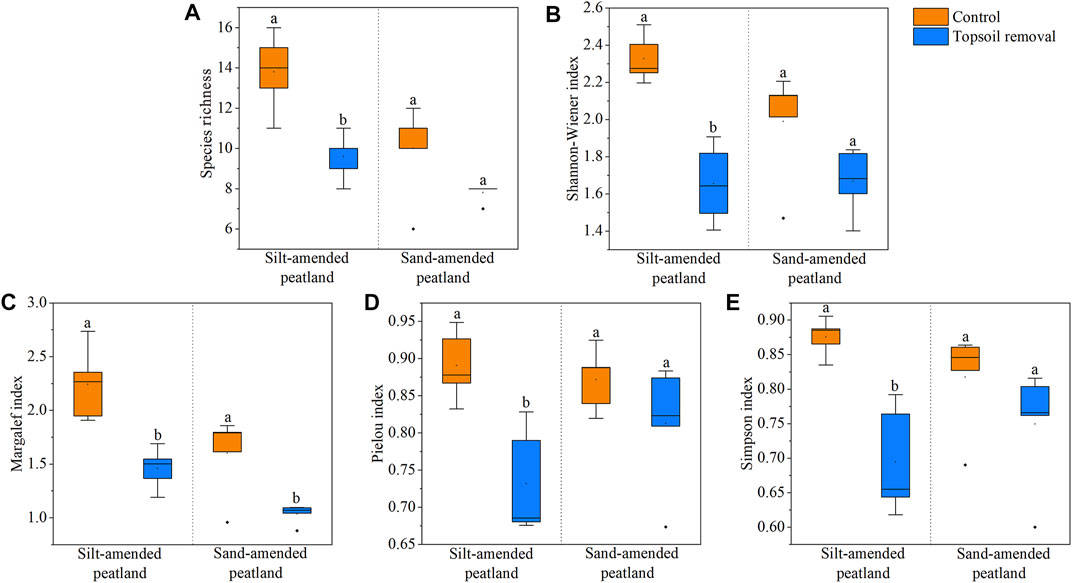
FIGURE 3. Species richness (A), Shannon–Wiener Index (B), Margalef Index (C), Pielou Index (D), and Simpson Index (E) in the silt-amended and sand-amended peatlands. Different lowercase letters indicate significant differences between the two treatment plots within the same peatland site (p < .05).
3.3 Effects of topsoil removal on Carex species at the silt-amended peatland site
The coverage, density, and biomass of Carex species in the topsoil-removed plots were higher than those in the control plots at the silt-amended peatland site (Figure 4). The basal diameter, root length, tiller number, and above- and below-ground biomass of Carex species in the topsoil-removed plots were higher than those in the control plots (Figure 5).
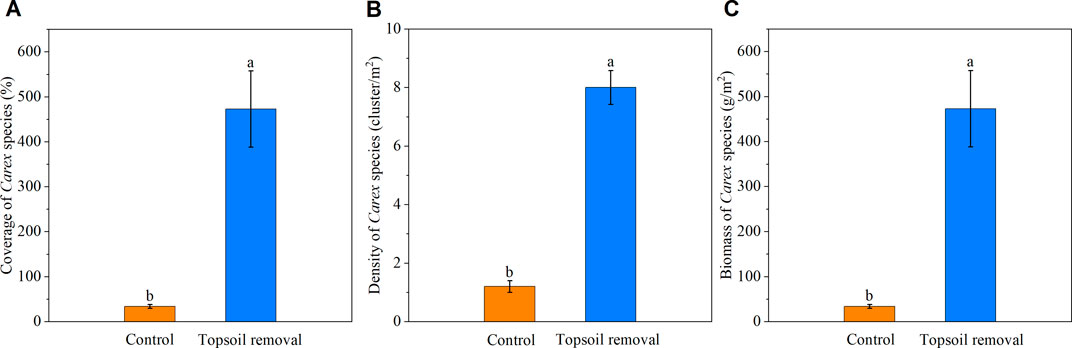
FIGURE 4. Coverage (A), density, (B) and biomass (C) of Carex species in the silt-amended peatland. Different lowercase letters indicate significant differences between the two treatment plots within the same peatland site (p < .05).
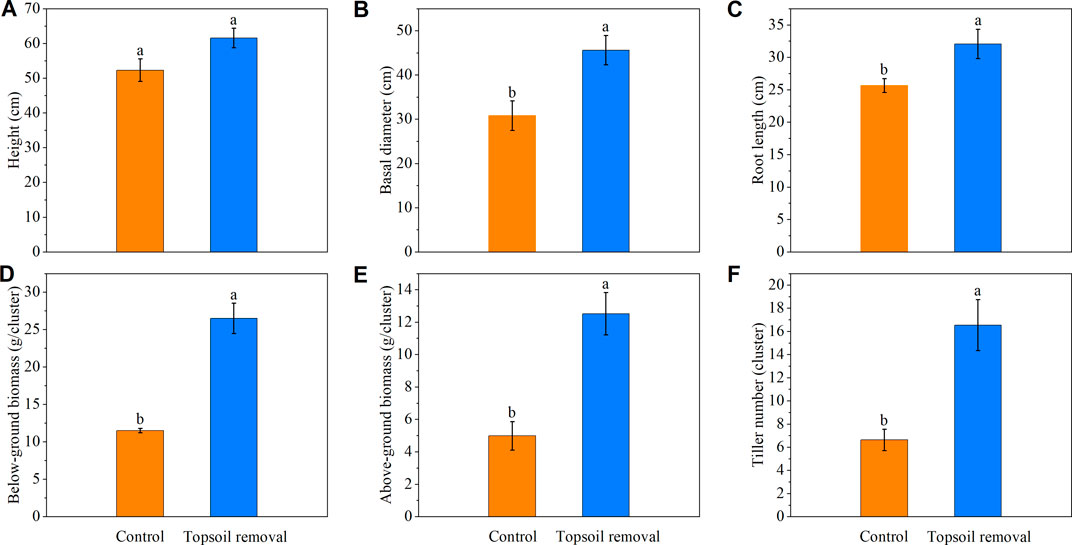
FIGURE 5. Height (A), basal diameter (B), root length (C), below-ground biomass (D), above-ground biomass (E), and tiller number (F) of Carex species in the silt-amended peatland. Different lowercase letters indicate significant differences between the two treatment plots within the same peatland site (p < .05).
3.4 The relationship between standing vegetation composition and soil environmental factors
RDA demonstrated that environmental factors explained 62.3% and 67.5% of the total variation of species composition at the silt- and sand-amended peatland sites, respectively. At the silt-amended peatland site, the peatland species including C. schmidtii were positively related to SOC, TP, SWC, and TN. At the sand-amended peatland site, C:N, SOC, and pH were the main controlling factors that affected species composition (Figure 6).
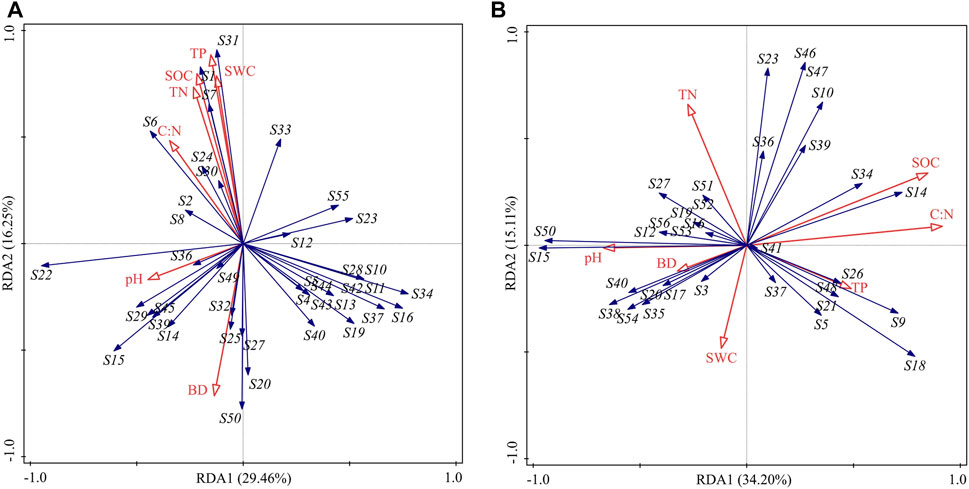
FIGURE 6. RDA ordination plots of the vegetation composition in the silt-amended peatland (A) and sand-amended peatland (B) constrained by environmental factors. SOC, soil organic carbon; SWC, soil water content; BD, bulk density; TN, total nitrogen; TP, total phosphorus; C:N, carbon to nitrogen ratio. Note: S1: Carex schmidtii, S2: Carex capricornis, S3: Schoenoplectus tabernaemontani, S4: Schoenoplectus triqueter, S5: Scirpus triqueter, S6: Scirpus wichurae, S7: Scirpus orientalis, S8: Bolboschoenus yagara, S9: Cyperus fuscus, S10: Cyperus orthostachyus, S11: Pycreus sanguinolentus, S12: Lythrum salicaria, S13: Alopecurus aequalis, S14: Echinochloa crus-galli, S15: Eleocharis wichurae, S16: Deyeuxia pyramidalis, S17: Deyeuxia purpurea, S18: Poa annua, S19: Phalaris arundinacea, S20: Typha latifolia, S21: Sanguisorba tenuifolia, S22: Arthraxon hispidus, S23: Epilobium hirsutum, S24: Glycine soja, S25: Kummerowia striata, S26: Lysimachia thyrsiflora, S27: Juncus bufonius, S28: Juncus effusus, S29: Juncus papillosus, S30: Hypericum monogynum, S31: Lysimachia davurica, S32: Salix myrtilloides, S33: Hypericum japonicum, S34: Bidens pilosa, S35: Synurus deltoides, S36: Alisma plantago-aquatica, S37: Sagittaria sagittifolia, S38: Sagittaria trifolia, S39: Polygonum sagittatum, S40: Polygonum hydropiper, S41: Polygonum thunbergia, S42: Polygonum viscosum, S43: Persicaria orientalis, S44: Pilea pumila, S45: Betula platyphylla, S46: Mentha canadensis, S47: Parnassia palustris, S48: Lobelia sessilifolia, S49: Iris sanguinea, S50: Murdannia keisak, S51: Galium trifidum, S52: Erigeron canadensis, S53: Cicuta virosa, S54: Monochoria korsakowii, S55: Erigeron acris, and S56: Galium trifidum.
4 Discussion
4.1 Farming practices affected soil environmental conditions in peatlands
Agricultural activities including reclamation, drainage, and fertilization significantly decreased the peatland area globally and changed BD, nutrient availability, and macropore space in farmed peatlands (Kløve et al., 2010; Smolczynski et al., 2021; Wang et al., 2022). In this study, soil environmental factors differed significantly between silt- and sand-amended peatlands and responded differently to the topsoil removal treatment (Table 1). At the silt-amended peatland site, mineral silt soil covered surface horizons of peatlands; therefore, the mineralization of peatlands can be hampered by the silted soil on the surface. This led to less oxygen availability and biological activity, thereby protecting peat below the surface against oxidation (Smolczynski et al., 2021). Therefore, such soils had higher SOC, TN, TP, and SWC at the silt-amended peatland site after removing top fine-textured material. However, coarse sand was mixed with peat soil, some of which leached into deeper soil layers (30–40 cm) at the sand-amended peatland site. It significantly changed physical and chemical properties of the soil and created an aerobic environment, leading to an increase in the decomposition of organic matter. Therefore, no significant difference was observed in terms of soil properties between the topsoil-removed and control plots at the sand-amended peatland site (Table 1).
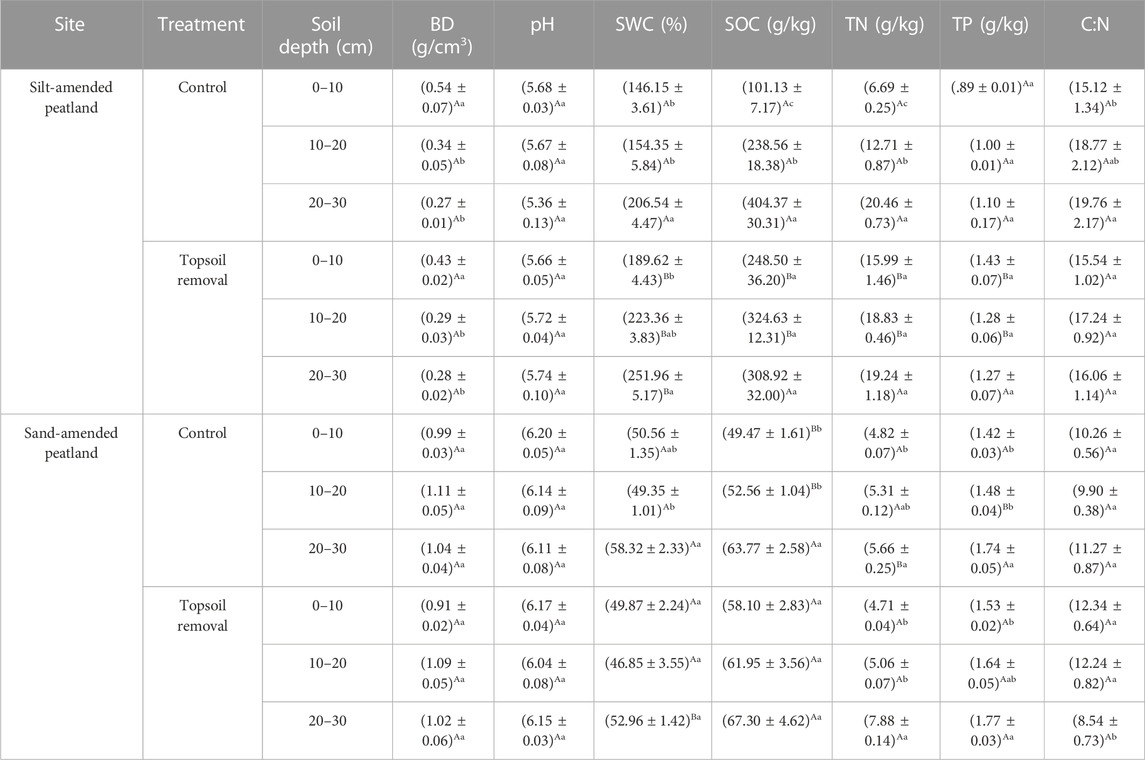
TABLE 1. Soil properties in silt-amended and sand-amended peatlands. Different capital letters represent significant differences between the two treatments within the same soil layer and the same peatland site. Different lowercase letters represent significant differences between the three soil layers within the same treatment. (p < .05). BD, soil bulk density; SWC, soil water content; SOC, soil organic carbon; TN, soil total nitrogen; TP, soil total phosphorus; C:N, soil carbon to nitrogen ratio.
4.2 Topsoil removal changed vegetation structure during peatland restoration
Because of long-term agricultural cultivation, many non-peatland species such as Echinochloa crus-galli, Murdannia keisak, and A. aequalis were observed in the restored peatlands, particularly in the control plots at the sand-amended peatland site (Table 2). These annual weed species reproduced and grew rapidly through the “R” reproductive response (Ma et al., 2019). However, the species richness and important indexes of these non-peatland species were lower in the topsoil-removed plots than those in the control plots (Table 2). This indicated that topsoil removal was effective in removing propagules of weed species from the soil.
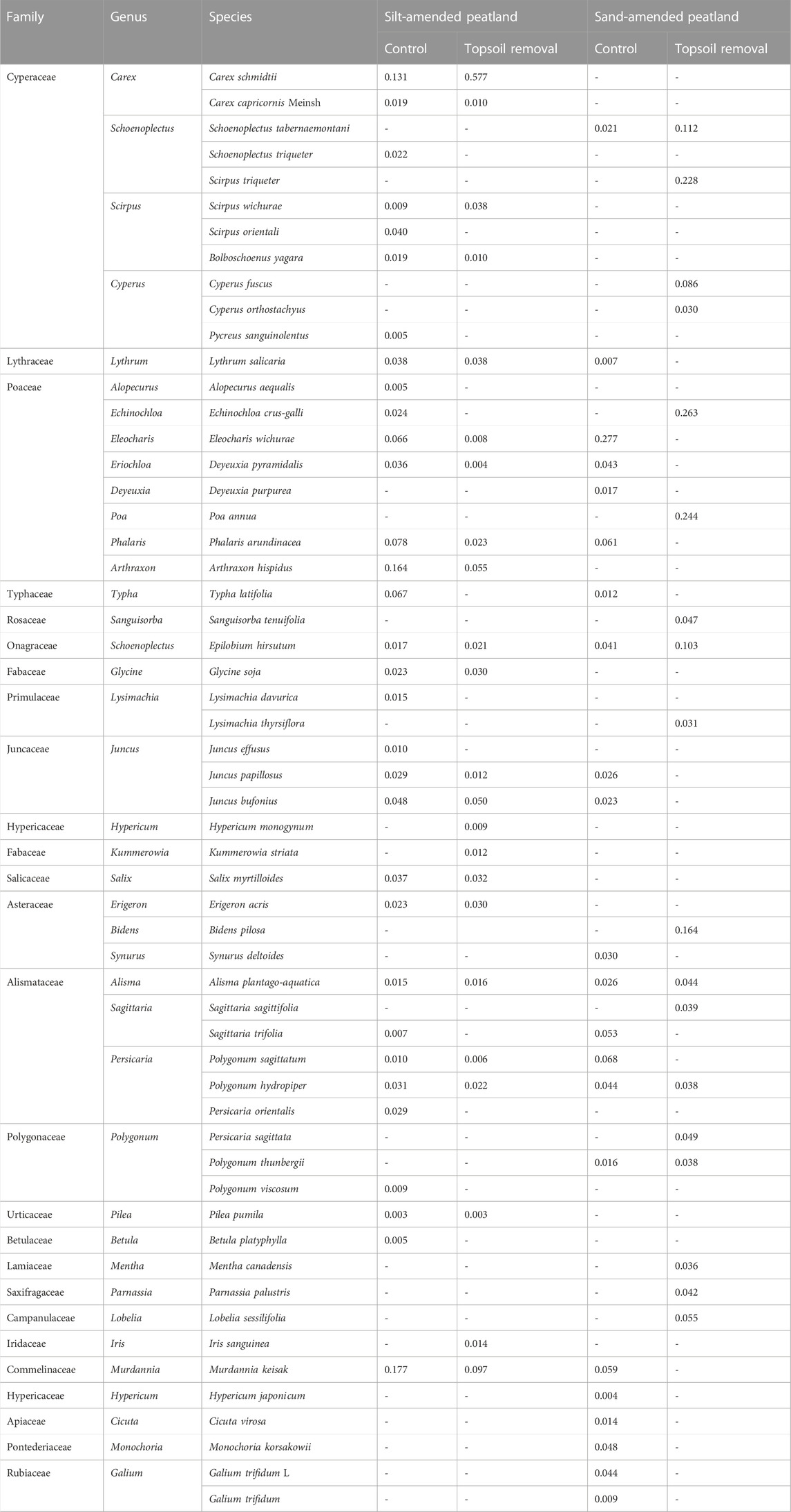
TABLE 2. Species that emerged in the vegetation plots and their importance values in the silt-amended and sand-amended peatlands.
More peatland species were observed in the topsoil-removed plots at the silt-amended peatland site (Table 2). This indicated that these peatland species were retained in the soil seed banks at the silt-amended peatland site, even in the deeper soil layer (10–20 cm) under the mineral soil. Former seed bank studies mainly focused on the upper 10-cm layer of wetland soils (Wang M et al., 2017; Davies et al., 2018; Zhao et al., 2021). However, cool, wet, and anaerobic conditions with slow microbial activity are beneficial for seed survival in peatlands; the seeds could remain viable at soil depth up to 50 cm (McGraw, 1987). In the silt-amended peatland, the surface silt soil may protect the seeds of peatland species in the layers below from physical damage and aerobic decomposition. Meanwhile, the removal of the top mineral soil layer eliminated the physical barriers that inhibited seed germination and plant smothering and exposed the peat layer below for seed germination of the retained peatland species.
Successful settlement and establishment of seedlings of target species are the most important aspects of vegetation restoration (Wang et al., 2020), where interspecific competition is a major constraint on seedling germination, growth, and survival (Kotorová and Leps, 1999). At the silt-amended peatland site, topsoil removal significantly increased the occurrence of Carex species; the coverage, density, and biomass of Carex species were 2.4, 3.0, and 5.7 times higher in the topsoil-removed plots than those in the control plots (Figure 4). By removing the topsoil, the number of weed seeds significantly declined, which provided a regenerative niche for seed germination and seedling establishment for Carex species. Therefore, the topsoil removal enhanced seedling recruitment of Carex species by reducing the interspecific competition. However, at the sand-amended peatland site, Carex species was absent in both control and topsoil-removed plots, whereas non-peatland species such as Echinochloa crus-galli and Poa annua were the dominant species. The coverage and aboveground biomass of plant community and plant diversity indexes exhibited no significant difference between the control and topsoil-removed plots. This suggests that topsoil removal did not significantly affect vegetation restoration at the sand-amended peatland site. Soil seed banks have been destroyed in sand-amended peatlands, which is a challenge to restoring the native vegetation.
RDA revealed that soil environmental factors explained more than 60% of variations in species composition in both silt- and sand-amended peatlands (Figure 6), which indicated that the change in the soil environment influenced seed bank germination. Former studies reported that agricultural practices reduced SOC, which affected the soil moisture and water holding capacity and prevented seed germination (Kettenring and Galatowitsch, 2011). In addition, seed viability in wetlands is affected by storage conditions; dry storage conditions reduced the seed viability of Carex species by 95% (Leck and Schütz, 2005). Similarly, Carex species disappeared, and few peatland species were present in the sand-amended peatland site in this study. This is because the mixing of sand into soil deteriorated soil environmental conditions, which damaged the seed banks and led to the absence of Carex species at the sand-amended peatland site.
Inconsistent with previous studies (Emsens et al., 2015; Harpenslager et al., 2015), topsoil removal did not reduce soil nutrient levels at the silt-amended peatland site in this study. On the contrary, topsoil-removed plots exhibited lower BD and higher SWC and soil nutrient (SOC, TN, and TP) levels (Table 1). However, soil properties of the topsoil-removed plots at the silt-amended peatland site were close to those of the natural peatlands (Wang et al., 2021). This can explain the strong relationships between peatland species (e.g., C. schmidtii and Lysimachia davurica), soil nutrient, and SWC at the silt-amended peatland site (Figure 6A). Therefore, topsoil removal at the silt-amended peatland site improved soil nutrient levels and created suitable abiotic conditions for the establishment of Carex species, leading to high biomass of Carex species in the topsoil-removed plots.
4.3 Implications for vegetation restoration in farmed peatlands
Our study illustrated that silt soil covering at the farmed peatlands provided suitable storage conditions for Carex seeds. The mixing of sands created the aerobic environment and led to an increase in organic matter decomposition and a significant change in physical and chemical properties of soil (Zaidel′man et al., 2001). Therefore, despite using for farming for a shorter period, the soil environment in sand-amended peatlands had dramatically changed. The result illustrated that the soil amendment type significantly affected peatland revegetation, and the silt-amended peatland exhibited a higher revegetation ability than the sand-amended peatland. Moreover, our findings confirmed that topsoil removal is an effective method for vegetation restoration in silt-amended peatlands because it not only improved soil properties but also promoted the growth of peatland species including C. schmidtii. However, topsoil removal is not a suitable way to restore all degraded peatlands, such as sand-amended peatlands. A detailed examination of soil environmental conditions and soil seed-bank distribution is necessary for successful peatland restoration.
5 Conclusion
We demonstrated that the effects of topsoil removal on vegetation restoration differed significantly between the silt- and sand-amended peatlands. The soil amendment type affected the restorability of peatlands by changing the conditions of the soil environment and soil seed bank. Topsoil removal exhibited great success in vegetation restoration in the silt-amended peatland. The addition of fine-textured material effectively protected peat at the deeper level from the aerobic environment and retained seeds in the peatlands in a favorable storage condition. After removing the topsoil material, peatland species could regenerate through the soil seed bank. On the contrary, target species disappeared from the sand-amended peatlands, and topsoil removal could not help in restoring peatland vegetation. Our study suggested that topsoil removal is an effective method for vegetation restoration in silt-amended peatlands but not in sand-amended peatlands.
Data availability statement
The original contributions presented in the study are included in the article/Supplementary Material; further inquiries can be directed to the corresponding author.
Author contributions
MW and SW conceived and designed the experiments. WC and YW were responsible for the implementation of the restoration project and management of the water level in the field. MW and YW analyzed the data and wrote the manuscript. All authors contributed to the article and approved the submitted version.
Funding
This study was supported by the National Natural Science Foundation of China (U19A2042) and the National Science and Technology Fundamental Resources Investigation Program of China (2019FY100600).
Acknowledgments
The authors would like to thank Guodong Wang for his comments on this manuscript.
Conflict of interest
The authors declare that the research was conducted in the absence of any commercial or financial relationships that could be construed as a potential conflict of interest.
Publisher’s note
All claims expressed in this article are solely those of the authors and do not necessarily represent those of their affiliated organizations, or those of the publisher, the editors, and the reviewers. Any product that may be evaluated in this article, or claim that may be made by its manufacturer, is not guaranteed or endorsed by the publisher.
Supplementary material
The Supplementary Material for this article can be found online at: https://www.frontiersin.org/articles/10.3389/fenvs.2022.1110057/full#supplementary-material
References
Beas, B. J., Smith, L. M., Hickman, K. R., Lagrange, T. G., and Stutheit, R. (2013). Seed bank responses to wetland restoration: Do restored wetlands resemble reference conditions following sediment removal? Aquat. Bot. 108, 7–15. doi:10.1016/j.aquabot.2013.02.002
Berglund, Ö., and Berglund, K. (2010). Distribution and cultivation intensity of agricultural peat and gyttja soils in Sweden and estimation of greenhouse gas emissions from cultivated peat soils. Geoderma 154 (3-4), 173–180. doi:10.1016/j.geoderma.2008.11.035
Davies, R. J. P., Mackay, D. A., Whalen, M. A., and Smyth, A. K. (2018). Vegetation and seed banks of arid ephemeral gilgai wetlands subject to contrasting grazing regimes. J. Arid. Environ. 154, 51–60. doi:10.1016/j.jaridenv.2018.03.006
Emsens, W. J., Aggenbach, C. J. S., Smolders, A. J. P., and van Diggelen, R. (2015). Topsoil removal in degraded rich fens: Can we force an ecosystem reset? Ecol. Eng. 77, 225–232. doi:10.1016/j.ecoleng.2015.01.029
Foley, J. A., Defries, R., Asner, G. P., Barford, C., Bonan, G., Carpenter, S. R., et al. (2005). Global consequences of land use. Science 309, 570–574. doi:10.1126/science.1111772
Frolking, S., Talbot, J., Jones, M. C., Treat, C. C., Kauffman, J. B., Tuittila, E. S., et al. (2011). Peatlands in the Earth's 21st century climate system. Environ. Rev. 19, 371–396. doi:10.1139/A11-014
Giannini, V., Bertacchi, A., Bonari, E., and Silvestri, N. (2019). Recolonisation by spontaneous vegetation of a rewetted peatland after topsoil removal: A focus on biomass production and nutrient uptake. Wetlands 39, 1079–1087. doi:10.1007/s13157-019-01141-2
Hallema, D. W., Lafond, J. A., Périard, Y., Gumiere, S. J., Sun, G., and Caron, J. (2015). Long-term effects of peatland cultivation on soil physical and hydraulic properties: Case study in Canada. Vadose Zone J. 14 (6), vzj2014.10.0147–12. doi:10.2136/vzj2014.10.0147
Harpenslager, S. F., van den Elzen, E., Kox, M. A. R., Smolders, A. J. P., Ettwig, K. F., and Lamers, L. P. M. (2015). Rewetting former agricultural peatlands: Topsoil removal as a prerequisite to avoid strong nutrient and greenhouse gas emissions. Ecol. Eng. 84, 159–168. doi:10.1016/j.ecoleng.2015.08.002
Heller, C., and Zeitz, J. (2012). Stability of soil organic matter in two northeastern German fen soils: The influence of site and soil development. J. Soils Sediment. 12 (8), 1231–1240. doi:10.1007/s11368-012-0500-6
Henriksson, N. D., Hydbom, S., Schmid, B. C., and Olsson, P. A. (2019). Topsoil removal enhances plant target species occurrence in sandy calcareous grassland. Flora 256, 7–15. doi:10.1016/j.flora.2019.03.014
Jackson, R. B., Anderson, L. J., and Pockman, W. T. (2000). “Measuring water availability and uptake in ecosystem studies,” in Methods in ecosystem science. Editors O. E. Sala, R. B. Jackson, H. A. Mooney, and R. W. Howarth (New York, NY, USA: Springer), 199–214.
Kalembasa, S. J., and Jenkinson, D. S. (1973). A comparative study of titrimetric and gravimetric methods for the determination of organic carbon in Soil. J. Sci. Food Agr. 24, 1085–1090. doi:10.1002/jsfa.2740240910
Kalisz, B., Urbanowicz, P., Smólczyński, S., and Orzechowski, M. (2021). Impact of siltation on the stability of organic matter in drained peatlands. Ecol. Indic. 130, 108149. doi:10.1016/j.ecolind.2021.108149
Kettenring, K. M., and Galatowitsch, S. M. (2011). Carex seedling emergence in restored and natural prairie wetlands. Wetlands 31, 273–281. doi:10.1007/S13157-011-0160-0
Klimkowska, A., Kotowski, W., Van Diggelen, R., Grootjans, A. P., Dzierza, P., and Brzezinska, K. (2010). Vegetation re-development after fen meadow restoration by topsoil removal and hay transfer. Restor. Ecol. 18, 924–933. doi:10.1111/j.1526-100X.2009.00554.x
Kløve, B., Sveistrup, T. E., and Hauge, A. (2010). Leaching of nutrients and emission of greenhouse gases from peatland cultivation at Bodin, Northern Norway. Geoderma 154 (3-4), 219–232. doi:10.1016/j.geoderma.2009.08.022
Kotorová, I., and Leps, J. (1999). Comparative ecology of seedling recruitment in an oligotrophic wet meadow. J. Veg. Sci. 10 (2), 175–186. doi:10.2307/3237139
Leck, M. A., and Schütz, W. (2005). Regeneration of Cyperaceae, with particular reference to seed ecology and seed banks. Perspect. Plant Ecol. Evol. Syst. 7, 95–133. doi:10.1016/j.ppees.2005.05.001
Leifeld, J., Wüst-Galley, C., and Page, S. (2019). Intact and managed peatland soils as a source and sink of GHGs from 1850 to 2100. Nat. Clim. Chang. 9 (12), 945–947. doi:10.1038/s41558-019-0615-5
Ma, M. J., Baskin, C. C., Li, W. J., Zhao, Y. P., Zhao, Y., Zhao, L., et al. (2019). Seed banks trigger ecological resilience in subalpine meadows abandoned after arable farming on the Tibetan Plateau. Ecol. Appl. 29 (7), e01959. doi:10.1002/eap.1959
Ma, X. H., Yin, S. C., Wen, B. L., Wang, M., Wang, G. D., Wang, D. X., et al. (2013). The carbon reserves and emissions of peatlands in China. Beijing: Chinese Forestry Press. (in Chinese).
McGraw, J. B. (1987). Seed-bank properties of an Appalachian sphagnum bog and a model of the depth distribution of viable seeds. Can. J. Bot. 65 (10), 2028–2035. doi:10.1139/b87-277
Řehounková, K., Jongepierová, I., Šebelíková, L., Vítovcová, K., and Prach, K. (2020). Topsoil removal in degraded open sandy grasslands: Can we restore threatened vegetation fast? Restor. Ecol. 29 (1), e13188. doi:10.1111/rec.13188
Resch, M. C., Schütz, M., Graf, U., Wagenaar, R., Putten, W. H., Risch, A. C., et al. (2019). Does topsoil removal in grassland restoration benefit both soil nematode and plant communities? J. Appl. Ecol. 56, 1782–1793. doi:10.1111/1365-2664.13400
Smolczynski, S., Kalisz, B., Urbanowicz, P., and Orzechowski, M. (2021). Effect of peatland siltation on total and labile C, N, P and K. Sustainability 13 (15), 8240. doi:10.3390/su13158240
Soto, D. P., and Puettmann, K. J. (2018). Topsoil removal through scarification improves natural regeneration in high-graded Nothofagus old-growth forests. J. Appl. Ecol. 55, 967–976. doi:10.1111/1365-2664.12989
Verhagen, R., Klooker, J., Bakker, J. P., and van Diggelen, R. (2001). Restoration success of low-production plant communities on former agricultural soils after top-soil removal. Appl. Veg. Sci. 4, 75–82. doi:10.1111/j.1654-109X.2001.tb00236.x
Verhoeven, J. T. A. (2014). Wetlands in Europe: Perspectives for restoration of a lost paradise. Ecol. Eng. 66, 6–9. doi:10.1016/j.ecoleng.2013.03.006
Wang, G. D., Wang, M., Lu, X. G., and Jiang, M. (2017). Duration of farming is an indicator of natural restoration potential of sedge meadows. Sci. Rep. 7 (1), 10692. doi:10.1038/s41598-017-11429-0
Wang, M., Wang, G. D., Lu, X. G., Jiang, M., and Wang, S. Z. (2017). Soil seed banks and their implications for wetland restoration along the Nongjiang River, Northeastern China. Ecol. Eng. 96, 26–33. doi:10.1016/j.ecoleng.2016.05.075
Wang, M., Wang, S. Z., Cao, Y. W., Jiang, M., Wang, G. D., and Dong, Y. M. (2021). The effects of hummock-hollow microtopography on soil organic carbon stocks and soil labile organic carbon fractions in a sedge peatland in Changbai Mountain, China. Catena 201, 105204. doi:10.1016/j.catena.2021.105204
Wang, M., Wang, S. Z., Wang, G. D., and Jiang, M. (2019). Importance of tussocks in supporting plant diversity in Carex schmidtii Meinsh wetlands. Mar. Freshw. Res. 70 (6), 807–815. doi:10.1071/MF18237
Wang, M., Wang, S. Z., Wang, G. D., and Jiang, M. (2020). Soil seed banks and restoration potential of tussock sedge meadows after farming in Changbai Mountain, China. Mar. Freshw. Res. 71 (9), 1099–1106. doi:10.1071/MF19025
Wang, Y. Q., Paul, S. M., Jocher, M., Alewell, C., and Leifeld, J. (2022). Reduced nitrous oxide emissions from drained temperate agricultural peatland after coverage with mineral soil. Front. Environ. Sci. 10, 856599. doi:10.3389/fenvs.2022.856599
Yu, Z. C. (2011). Holocene carbon flux histories of the world’s peatlands: Global carbon-cycle implications. Holocene 21, 761–774. doi:10.1177/0959683610386982
Zaidel′man, F. R., Kozhevin, P. A., Shvarov, A. P., Pavlova, E. B., and Gorlenko, M. V. (2001). The effect of different sanding methods on the biological activity and gas regime of drained peat soils. Eurasian Soil Sci. 34, 207–216.
Zakharova, O. A., Musaev, F. A., Kucher, D. E., Vinogradov, D. V., and Ushakov, R. N. (2020). Sanding of drained peatlands. BIO Web Conf. 17, 00089. doi:10.1051/bioconf/20201700089
Zhang, D. J., Xia, J. B., Sun, J. K., Dong, K. K., Shao, P. S., Wang, X. H., et al. (2022). Effect of wetland restoration and degradation on nutrient trade-off of Carex schmidtii. Front. Ecol. Evol. 9, 801608. doi:10.3389/fevo.2021.801608
Keywords: topsoil removal, regeneration, peatland restoration, soil amendment, Carex
Citation: Wang Y, Wang Y, Wang S, Wang M and Chai W (2023) Assessment of topsoil removal as an effective method for vegetation restoration in farmed peatlands. Front. Environ. Sci. 10:1110057. doi: 10.3389/fenvs.2022.1110057
Received: 28 November 2022; Accepted: 15 December 2022;
Published: 05 January 2023.
Edited by:
Xiaoyu Li, Northeast Institute of Geography and Agroecology (CAS), ChinaCopyright © 2023 Wang, Wang, Wang, Wang and Chai. This is an open-access article distributed under the terms of the Creative Commons Attribution License (CC BY). The use, distribution or reproduction in other forums is permitted, provided the original author(s) and the copyright owner(s) are credited and that the original publication in this journal is cited, in accordance with accepted academic practice. No use, distribution or reproduction is permitted which does not comply with these terms.
*Correspondence: Ming Wang, wangm100@nenu.edu.cn