- 1Department of Environmental Engineering, Faculty of Civil Engineering, Istanbul Technical University, Istanbul, Türkiye
- 2Department of Environmental Engineering, Corlu Faculty of Engineering, Namik Kemal University, Tekirdag, Türkiye
This study presents the payback periods of applying rainwater harvesting (RWH) and/or graywater reuse (GWR) systems as alternative water resources in different building typologies, such as a hospital, shopping mall, and hotel. These buildings are under operation in the Antalya Province of Türkiye, which is a large city having the densest tourism activities. The significance of the work performed through the cost-benefit analyses for the selected case studies basically lies on the water savings while serving to four of the sustainable development goals, namely, clean water and sanitation, sustainable cities and communities, responsible consumption and production, and climate action. These efforts may be considered valuable urban-based solutions toward climate change effects. Thorough surveys on the existing selected typologies are conducted regarding their water consumption and probable water savings via reuse activities. As-built plumbing projects and plans are also investigated during the accomplishment of the comprehensive design work leading to the calculation of the total investment and operation costs of the rainwater harvesting and graywater reuse practices. The up-to-date prices are used in monetary terms, and euro currency is used to make the results more meaningful by the interested parties. All the selected typologies undergo cost-benefit analysis for both of the alternative water reuse systems. The payback periods are calculated as 6, 2, and 9 years for RWH and as 5, 6, and 9 years for GWR for the hospital, shopping mall, and the hotel, respectively. The water savings for RWH varied between 20% and 50% whereas for GWR, the range was 48%–99%. Both of the systems are performed for the shopping mall simultaneously, and the resulting payback period is found to be 5 years, and water saving reached 72%. Recent information on the amortization periods in the literature states that less than a decade demonstrates achievable and highly acceptable applications. As such, the design attempts in this study also correlated with these findings. However, feasibility of these practices may be increased by encouraging the public on their utility and benefit of water savings. As is the case in many of the developed countries, incentives like tax reductions and even exemptions may be realized to achieve better applicability of these alternative technologies.
1 Introduction
As water resources are becoming limited due to increase in population, urbanization efforts, mass housing, and decrease in clean receiving water bodies in parallel to the effect of climate change, penetrating into alternative water resources, have accelerated all around the world, especially in countries/regions experiencing water scarcity and shortage. The common alternatives of concern in adaptation to climate change are mainly rainwater harvesting (Ghaffarian Hoseini et al., 2016; Merville-Screeve et al., 2016; Campisano et al., 2017; Musayev et al., 2018), graywater reuse (Vuppaladadiyam et al., 2019; Elhegazy and Eid, 2020; Anuja et al., 2021; Khajvand et al., 2022), reuse of treated wastewater (Angelakis and Bontoux, 2001; Mizyed, 2013; Ofori et al., 2021), and desalination of seawater (Navarro, 2018; Zhu et al., 2019; Pistocchi et al., 2020), which are all regarded satisfactory applications as urban nature-based solutions and green infrastructure as strategies for climate change adaptation. In the transition to the “Circular Economy,” which is defined as the most important strategy of adaptation to climate change within the EU Green Deal Policy, it has become imperative to ensure “water circularity” (EC, 2019).
The subject of concern is highly important, especially regarded as an urban-based solution within the context of climate change adaptation exertions. It seems that this topic will even receive more interest than before as water shortage and scarcity will be among the leading environmental problems in the coming years. Sustainable development goal numbers 6 (clean water and sanitation), 11 (sustainable cities and communities), 12 (responsible consumption and production), and 13 (climate action) focus on this issue; therefore, it will gain more interest among the public as sustainability lies at the center of our lives.
Water reuse is not a new technology; however, it has gained interest in line with the lessening of freshwater resources all around the world as underlined by Angelakis et al. (2018). The oldest technology known among these alternatives is rainwater harvesting and storing rainfall in cisterns and later using it for any domestic needs including drinking and cooking (Okhravi et al., 2016; Poff et al., 2016). Graywater is the domestic wastewater excluding the septic/toilet water, forming the weakly polluted part of domestic discharges. This water resource can easily be treated within the building where it is being collected and reused, especially for flushing water which almost constitutes 25%–30% of domestic water uses (Jorgensen et al., 2009; Muthukumaran et al., 2011; Gross et al., 2015; Bergel et al., 2016; Ghunmi and Eslamian, 2016). Moreover, this water resource may even be used in cleaning the indoor environment, as laundry water, or for garden irrigation and car washing as an outdoor application. Domestic consumption may decrease by around 50% through graywater reuse (GWR) and rainwater harvesting (RWH), leading to high water savings (EPA, 2017; Vieira et al., 2017). These alternative uses have even become sounder due to the negative impacts of climate change on the existing water resources, as underlined by Novotny (2013) and Eslamian (2016).
Rapid urbanization has presented many complications, especially in developing countries, over time. In the future, due to the effects of climate change, urbanization is projected to increase necessitating the acceleration of sustainable management strategies including water uses. The Turkish Ministry of Environment, Urbanization, and Climate Change organized a “Climate Council” in February 2022 to determine the country’s roadmap to combat climate change. Two of the important actions stated in the final declaration are as follows (URL1, 2022):
* Legislation on water efficiency should be established in buildings, the use of graywater should be encouraged, and the use of rainwater and the establishment of a zero-waste system should be made mandatory.
* Rainwater harvesting and the use of graywater should be expanded, and guiding legislation should be developed for this purpose. Wastewater treated in wastewater treatment plants should be reused.
As a reliance on these significant tutorials, we decided to initially mention RWH and GWR alternatives in this article based on their advantages and disadvantages by means of a search throughout the world. It is then that the situation in Turkey is explained briefly, and emphasis is given to Antalya Province in which a hospital, a shopping mall, and a hotel under operation are selected as representative building typologies. Cost and benefit analyses of RWH and GWR are conducted separately and of the combined use of both alternatives in the shopping mall in detail, forming preceding examples on their design to initiate such reuse activities in Türkiye that characterizes a developing country facing adverse climate change effects on its water resources (CCIWR, 2016). Due to confidentially agreements signed with the owners of these specific typologies, their names will not be stated in the article and they will be referred as typologies A, B, and C on behalf of the selected hospital, shopping mall, and hotel, respectively.
The topic of concern is among the leading and outstanding environmental problems of the world due to adverse climate change effects, especially on water resources. Therefore, this study aims to present the details of the design work on three different case studies in which RWH and GWR collection, treatment, storage, and reuse applications are proposed. The results are put forth in terms of payback periods, while annual % water savings and their monetary terms are mentioned.
1.1 Rainwater harvesting (RWH)
RWH is a simple method that has been practiced for thousands of years in the arid lands of the world, especially in areas where other water resources are scarce and/or difficult to access. Since early civilizations, collecting precipitation and storing water have been very important for people living in arid and semi-arid regions (Angelakis et al., 2018; Al-Batsh et al., 2019). Archaeological findings in many parts of the world confirm that since the prehistory period, people met various water needs such as domestic needs, agricultural irrigation, and livestock activities by collecting and storing rainwater in low permeable lands or cavities in rocks (Yannopoulos et al., 2019). It is known that storage of rainwater for use dates back to the Neolithic Age (Mays et al., 2013). Harvesting methods range from small and simple, such as connecting a water barrel to a rainwater downpipe, to large and complex ones that collect water from acres of lands and serve large numbers of people. However, with the management of large quantities of water supply achieved by the development of engineering skills in the industrial era, the application of this method has lost its reputation more recently (Yannopoulos et al., 2019).
In RWH applications, the water falling on the roof of the building can be accessed without any carrying costs. The roof is a surface-free environment from human intervention. Therefore, there are not many sources of pollution outside the roof surface. It is easy to manage and treat at a relatively low cost. For these reasons, RWH is highly efficient as part of an integrated water management approach for seasonal water security (Smit, 2019). There are large-scale RWH system applications in many parts of the world. These applications are specifically common in hotels, university campuses, airports, and complex buildings.
Strategic management of rainwater is a form of water management that can reduce disaster risk for countries facing water scarcity, drought, or flood risks (Borja-Vega, 2020a; Borja-Vega, 2020b). Known as the driest continent in the world, Australia is the region where RWH studies started (Rahman et al., 2012). There are different practices in different states in the United States of America. For example, there are many harvesting and green roof applications in New York (Sel, 2017). Governments play an important role in promoting and improving RWH practices. Government subsidies can encourage the establishment of RWH systems and increase the number of users, especially among low-income households. For example, there are large incentive projects in Brazil and Singapore. Since the beginning of the 2000s, the Chinese people’s perspective on a sustainable society and the importance of water have been developed with the help of awareness studies created by non-governmental organizations in the country (Fu, 2018).
Germany is a country with abundant water resources compared to other countries. However, the country has strict laws and regulations on both the development and use of water resources and the management of wastewater in order to maintain a good water status and ensure the sustainable use of water resources. In addition, significant budgets are allocated to research and development studies in the fields of water resource management technology. Programs being implemented by governments or local governments to promote RWH systems are mainly tax deductions, cost-based grants, obligations for new constructions, penalties, tax liabilities related to stormwater discharge fees, and restrictions on the water use in applications such as landscape irrigation. RWH systems are common in countries with a lack and irregularity of precipitation and/or past significant droughts (Celik et al., 2017). In addition, reasons such as the protection of existing water resources and the concern of pioneering RWH technologies give priority to the widespread use of these practices (Schuetze, 2013; Campisano et al., 2017).
1.2 Graywater reuse (GWR)
Graywater is a large volume of wastewater with a high potential for reuse and application. It is domestic wastewater originating from showers, handwash basins, laundries, washing machines, and kitchen waters, excluding toilet waste, which is considered as black water, and food waste from garbage disposals (Wilderer, 2004). The origin and composition of graywater depends on the lifestyle, population structure, social and cultural habits, the number of people living in the house, the chemicals used in the house, and the availability of water and climatic conditions, which differ from one country to another (Filali et al., 2022).
The characteristic of graywater is that it contains high concentrations of easily degradable organic matter. The pathogen rate in graywater is relatively low. It generally contains less suspended solids (SS) and nitrogen (N) and more phosphorus (P) than a typical domestic sewer. In general, ammonia and total Kjeldahl nitrogen (TKN) concentrations in greywater are approximately 10 times lower than that in domestic wastewater (Lazarova et al., 2003). The content of metals and organic pollutants in graywater is generally low and at lower levels for zinc and mercury than for mixed wastewater (Potivichayanon et al., 2021; Shaikh and Ahammed, 2022). Graywater sources are divided into two: low-load sources (tubs, showers, and hand sinks) and high-load sources (laundry and kitchen). The typical graywater volume varies between 60 L and 120 L/person/day, although it differs depending on user behavior, population structure, customs and habits, plumbing, and water availability (Zavala et al., 2016). The use of treated graywater includes indoor reuse such as flush toilets and outdoor reuse such as garden irrigation and car washing. Treated graywater is also used in agriculture when it complies with the different health and environmental standards established for this purpose (Rodda et al., 2011).
Characterization of graywater reveals that it must be treated to a higher standard before it is reused to avoid health risks and adverse esthetic and environmental effects. The main goal of graywater treatment is to reduce SS, organic resistance, and microorganisms through direct legal requirements and its relationship with the esthetic and health properties of the recovered water. Technologies applied for graywater treatment include physical, chemical, and biological systems. GWR systems are implemented in many countries. However, policies, regulations, participation, and socio-economic and technical complications vary from country to country (Mizyed, 2013; Thaher et al., 2020; Nawamed, 2021; Al-Khatib et al., 2022). Therefore, it is important to develop policies and regulations that are compatible with local needs and requirements. Internationally, GWR is often optional rather than mandatory. In some settlements, voluntary practices are encouraged through incentives such as tax breaks, grants, and permit exemptions (Bell, 2018; SF, 2022). Some authorities may require contractors to install dual-plumbing systems to accommodate future GWR (Yu et al., 2016).
Looking at the European Union (EU) regulations, it is seen that there is currently no directive regulating the reuse of water. In the Assessment of the Water Framework Directive, Europe’s Plan for Conservation of Water Resources (2012) highlights the importance of water reuse in irrigation and industries, but there is no clear directive (Stein, 2015). EU-level instruments on water reuse have been prepared in 2016 (EU IA, 2016), and the key idea behind these explanations and efforts is the sustainable management of water as water resources are becoming scarce in time due to climate change impacts. The Water Reuse–Legislative Framework in EU Regions has been proposed in 2018 by the Commission for the Environment, Climate Change, and Energy; however, this suggestion basically relies on the reuse of treated wastewater in irrigation within the context of river basin management plans (EU, 2018). To help alleviate demands for drinking water in water-limited Australia, for example, water recycling is increasingly being incorporated into the policy framework and development of guidelines. Implementation rules for the use of graywater were established in the country in 2010. Various incentives are offered to owners of GWR systems in various states in the United States. However, this practice varies on the basis of country administration, as in rainwater, and differs on the basis of states and even cities (Yu et al., 2013).
1.3 Current situation of the water sector in Antalya Province, Türkiye
Of the overall 81 provinces in Türkiye, 30 are managed through greater metropolitan municipalities among which Istanbul represents one of the top ranked crowded cities of the world, Ankara, the capital town of Turkey, Izmir, the third-most crowded city located along the Aegean Coast, and Antalya, the most favored and internationally well-known tourist city of the country on the Mediterranean Coast can be addressed. In its present state, the average amount of usable water in our country is around 1,519 m3/person/year (MoFA, 2022). Therefore, it can be stated that Türkiye is experiencing water scarcity according to this amount. As it is surrounded by seas on the three sides, desalinization of seawater is a feasible option but it rather supports the water demand in local areas rather than the highly urbanized cities. The potential of treated water use, especially in agricultural irrigation, is an appealing option in the country as Türkiye is still known as an agricultural country; however, such activities are not frequent in urbanized areas. As such, the rest of the two options, RWH and GWR, will be emphasized as nature-based solutions of water reuse for the urbanized tourism provinces, and Antalya Province, in that sense, is selected as the case study metropolis subject to this study.
Figure 1 shows the map of Turkey on which 81 provinces are shaded, and only the names of the greater metropolitan cities are plotted. Table 1 presents population, population share, and socio-economic development ranking of Antalya, whereas Table 2 depicts some of the water-related data.

TABLE 2. Water-related data for Antalya—2021 values (EI, 2021).
The daily amount of water transferred to the drinking and utility water network by the municipalities in Antalya as of 2021 is 329 L per person, which is above the country’s average of 224 L. In the climate projections studied until 2,100, it is seen that Antalya will inevitably be affected by climate change, and decrease in precipitation is expected in addition to temperature increases (CCIWR, 2016). There are 29 rivers in Antalya, and 7.6% of the water potential of Turkey is in this city. Among the 24 dams in the province, drinking water is provided from just four of them. The province is also rich in terms of groundwater resources. However, since the province is one of the most important tourism centers in the country, its population, which reaches very high levels, especially in the summer months, causes problems related to the city’s water resources. Antalya, one of the most important centers of Turkish tourism, hosted a total of 9,094,051 domestic and foreign tourists in 2021 (MoEU, 2021). In line with this foresight, it is important that these two alternative water resources need to be widely used, especially in hotels and shopping centers, considering the high level of water use, as it is one of the most important tourism centers of the country. In the context of RWH, since the province receives more precipitation than Türkiye’s average, significant amounts of water can be saved via this resume activity. Some examples on both RWH and GWR exist in the city, especially in hotels; however, these applications are not widespread in the city as is the case in the other metropolitan cities of the country. More recently, there seems to be high awareness of climate change in Türkiye, particularly in the highly urbanized regions with the huge efforts of the central government and the local municipalities. Therefore, with this study, it is aimed to at least realize the design experience and calculate the payback periods through the cost-benefit analyses of the selected building typologies to present their economic feasibility/acceptability. Moreover, this work intends to form an example to other similar cities not only in the country but also for the interest of other cities/countries within the context of climate change adaptation efforts.
It is important to highlight the issue that neither the RWH nor the GWR applications in Turkey are based on any related national regulations and standards regarding the quality aspects. However, the state policy emphasizes on the water reuse practices without interrupting the wellbeing of humans. Regulation on rainwater collection, storage, and discharge systems has been put into force in 2017 through publication in the official newspaper dated 23 June 2017 with the number 30,105 as an initial effort for encouraging RWH. With the amendment made by the MoEU in the Planned Areas Zoning Regulation, it became obligatory to install a rainwater collection system in new buildings in order to ensure that the rainwater on the roof is collected in a tank under the garden floor as of 23 January 2021.
2 Data and methodology
A hospital (typology A), shopping mall (typology B), and hotel (typology C) under operation are selected as representative building typologies on which cost and benefit analyses of RWH and GWR systems separately and of the combined form of both alternatives in typology B are studied in detail. These buildings do not benefit from any of the so-called water resources for the time-being. Characteristics of each building and their current water consumptions and plumbing projects provided by the related owners create the significant data on the study. Moreover, monthly 9 years’ average precipitation data provided from the Antalya International Airport Meteorological Station constitute another data file in the design and analysis.
Characteristics of each of the building types are given in Table 3, and the overall 9-year monthly average precipitation values are given in Table 4.
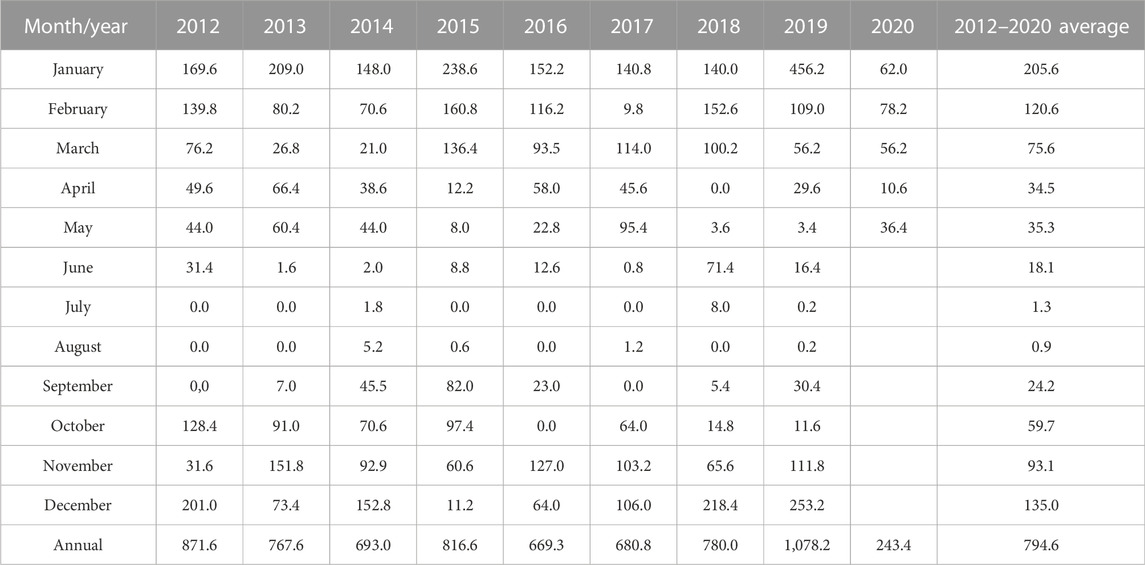
TABLE 4. Nine-year monthly average precipitation values (mm) of the Antalya Airport Meteorological Station.
Eq. 1 expresses the total amount of RWH (Dogangonul and Dogangonul, 2009):
where
Vr = amount of collectable rainfall, m3
A = area of the rainfall collection area, m2
P = average amount of precipitation, mm
e = flow coefficient of the drainage surface.
The runoff coefficient (e) is taken as 0.8 as all catchment areas are the deck (terrace) floor according to Farreny et al. (2011).
Harvested rainwater and/or graywater resources are assumed to be initially used as flushing water in the toilets, and the excess/remaining portion is to be utilized for garden/green area irrigation if there is such an availability or else may be used as cleaning water for indoors and car washing. Table 5 refers to the unit water consumption values per activity. The values given in Table 5 are collected from the Turkish Statistical Institute (TSI) as of 2021. The related standard takes place in TS EN16941-2 for non-potable water systems— Part 2: Systems for the use of treated graywater (sink wastewater) and published in February 2021.
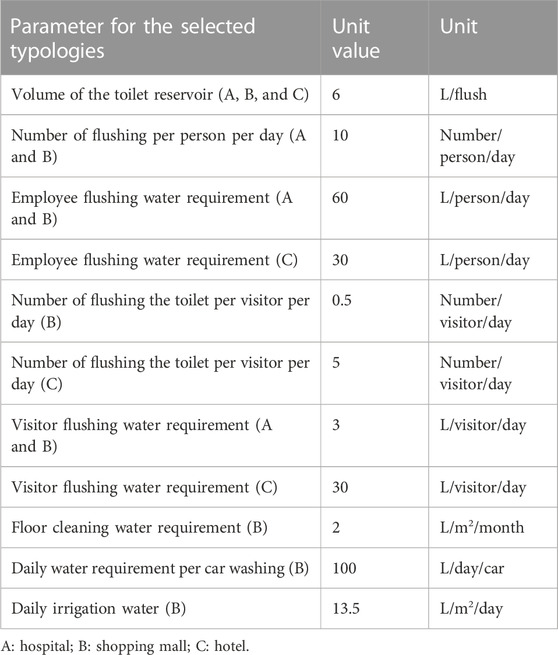
TABLE 5. Unit water consumption values considered in the calculations for each activity (TSI, 2021).
Eq. 2 expresses the total amount of GW generated from domestic water consumption:
GW collection is possible from handwash sinks and showers in these building typologies. As such, the unit water to be collected per building typology is given in Table 6. However, this water resource requires treatment prior to use even as toilet flushing water, and package-type membrane bioreactors (MBRs) are preferred to be installed at the basement of the buildings. These pre-engineered systems are easily established and put into operation (Atanasova et al., 2017). An appropriate MBR and its appurtenances together with pumping facilities constitute the investment cost of GWR systems.
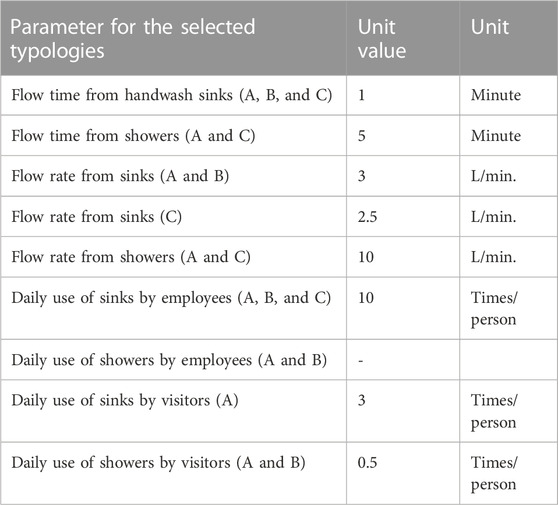
TABLE 6. Unit amount of GW to be collected for each activity (TSI, 2021).
For RWH, the collected water has to be stored prior to use for any of the reuse activities. As such, storage tank volume(s) is/are calculated according to the following methodology. Initially, the daily precipitation amount is evaluated in the month with the highest rainfall, and the amount of precipitation collected in the storage tank is considered. It is appropriate to choose a tank with expert opinion to store the maximum possible precipitation for at least 3 days. Furthermore, for attaining more suitable solutions, it is advised to select two or more similar tanks rather than one. Table 7 refers to the details of the storage design work of the RWH systems for each of the building typology.
When deciding on the treatment system capacity in the GWR system, the amount of required water and generated GW are evaluated together. If the amount of GW generated is more than the amount of the required water, the GW treatment system capacity is determined according to the water requirement. In scenarios, the treatment system capacity is selected according to the amount of GW generated. When the system is being set up, it is accepted that domestic wastewater such as from sinks, showers, bathtubs, and washings will collect in the GW treatment plant. The wastewater from the kitchen and black water that will cause inhibition (garden drainage and pool water) will not be given to the GW treatment plant.
As GW is different from freshwater, it necessitates some guidelines for reuse. The following points are usually considered:
• GW should not be kept for more than 24 h.
• The system should be designed to prevent water from penetrating the ground and be used for drinking purposes by humans or animals.
• GW should not be allowed to seep into the soil, accumulate, or flow.
• The system should be kept as simple as possible, and systems that require less maintenance should be preferred.
• There should be 3-way valves and cross-linking systems for easy transfer between the graywater system and the sewer line.
• Prior to use, labels must be inserted into the piping system so as to indicate their origin.
In the benefit determinations, the amount of water that is saved through the reuse application is converted to cost equivalence whereas the cost part includes the investment (pumping station, pumps, booster system, filter units and its appurtenances, piping and instrumentation, and storage tank for RWH, and in addition to such units, inclusion of a treatment system for GWR, crimping, and line laying for the installation of these systems to already existing buildings) and operational costs (electricity, maintenance and routine operation inspections, and repairs).
Some assumptions are made during the comprehensive design work of both reuse applications. These are summarized as follows:
• Prismatic galvanized modular water storage tanks are preferred for RWH.
• MBR package treatment systems are chosen as the GW treatment system.
• It is thought that the amount of water remaining in the storage tank is used in the coming month for RWH.
• Financial analysis was conducted in 10-year periods for 50 years in GWR systems and 100 years in RWH systems.
• For the unit price of water used in the calculations, 1 September 2022 is taken as the basis for Antalya Province. It is important to note here that due to high inflation fluctuations faced in the country, the unit cost of water changes quite frequently in the provinces; these cost values vary among the province and are set by the municipalities. Wastewater removal cost is also considered in the unit cost of water.
• The date 1 September 2022 is taken as the basis for the exchange rate used in the accounts.
• Inflation-based price increases are ignored.
• Value-added tax (VAT) and other taxes are not included in the calculations.
• A real discount rate of 5% is used.
3 Results and discussion
Detailed design criteria and calculations leading to the final cost benefit analyses are separately presented in Supplementary Materials (SM). SM-1 refers to RWH systems, whereas SM-2 denotes the GWR systems. The information provided in SM covers the calculation of the water savings, investment and operation costs, and cost-benefit analyses for the three selected typologies.
The cost and benefit analyses are conducted separately for each of the selected building typologies, and the final results are given as payback periods in years. The discussions will be based on these calculated periods. The feasible rate of return for both of the alternative water resources is accepted as less than a decade.
Table 8 summarizes the payback periods calculated for the selected building typologies resulting from the detailed cost-benefit analyses.
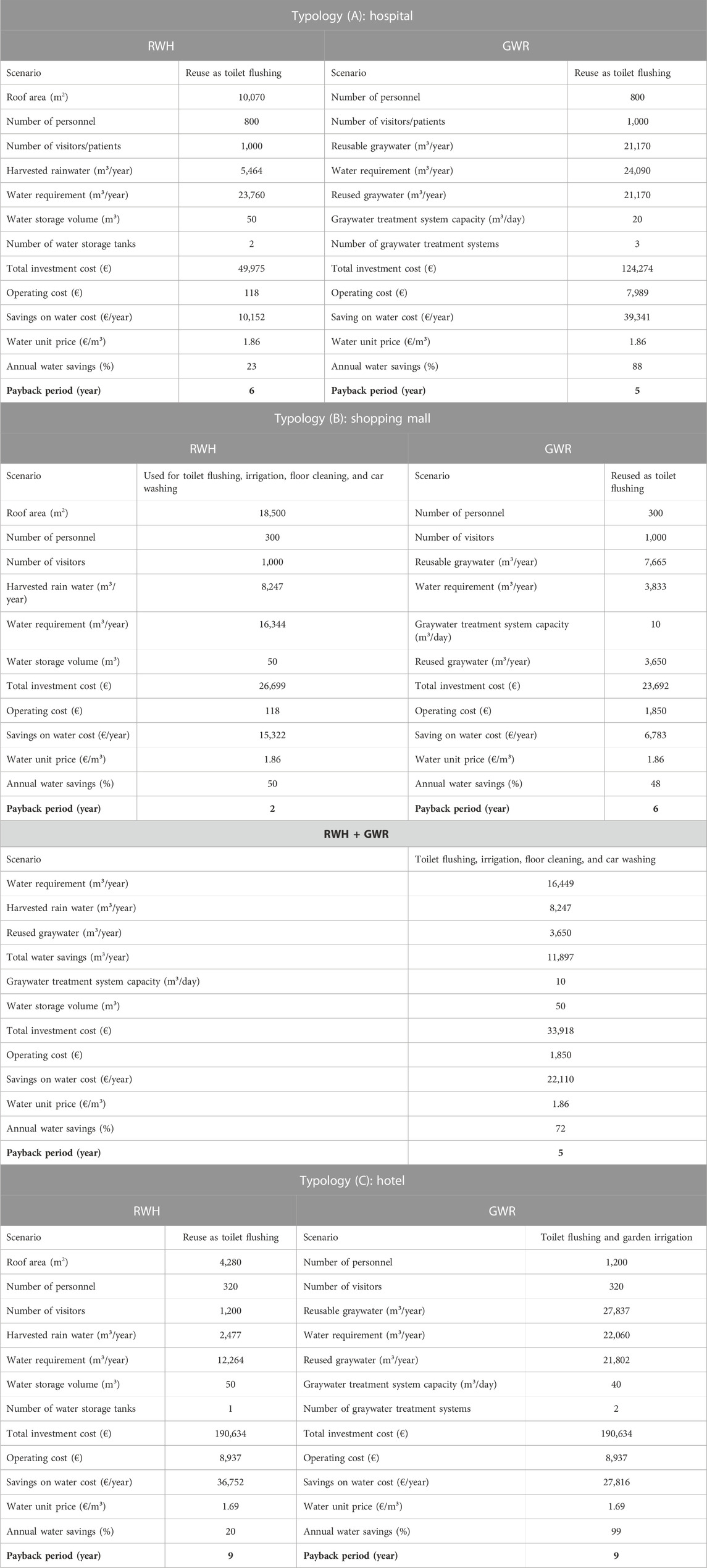
TABLE 8. Results of payback periods for different building typologies via water savings through RWH and GWR systems.
The water savings in different building typologies as expected and calculated in this study are different from each other, and this circumstance affects the payback periods. However, it is seen that both reuse strategies, applied separately or combined, will denote economic feasibility if the investments are amortized in shorter periods like 10 years or less. In a university campus in Northeastern Mexico where both strategies are practiced, the amortization period is found to be 6 years (Zavala et al., 2016). India is a country that experiences water shortage on the contrary to its rapidly growing population. A recent study on the design of the RWH system from this country has been conducted in the campus of a South Indian university where water is collected from 19 selected roofs. The result was appealing, and the payback period was calculated to be 13 years (Anchan and Shiva Prasad, 2021). Aybuga and Isıldar (2017) calculated the payback period of both RWH and GWR systems in Ankara, Türkiye, for around 5 years at the household level. A practical case study of a single-family house in Durban, Kwa-Zulu Natal Province of South Africa, was conducted on both systems, and the corresponding payback period was found to be 4.39 years (Zhang et al., 2021).
Another interesting study on cost-effectiveness of RWH and GWR systems has recently been conducted in single-family houses located in eight different countries. The results highly fluctuated based on the accessibility to freshwater, price of water, quality of water, climate change, and precipitation regime of the city. Moreover, the general outcome of the work put forth the commonly accepted reality that such new technologies and their financial indicators will bring benefits in the long run no matter the location of the building (Stec and Slys, 2022). In another preceding outcome of the surveys made within the same study in eight different European cities, it is seen that RWH is comparatively preferred than GWR systems. This finding emphasizes a social problem on the questionable hygienic conditions of GWR. This is also seen in the study conducted by Yu et al. (2016) that underlines the requirement of providing some financial incentives on the GWR systems to encourage the utility of GW. Most recently, Al-Saidi (2021) and Al-Khatib et al. (2022) outlined the knowledge about the social acceptance of reuse applications. It provides a methodical aspect on the overall reuse trials and social insolences toward water reuse, considering the elements of water source, technology, and end use. As such, inspiration on the reuse practices is not solely a technological and financial feature; it also governs a socio-economic insight necessitating the dissemination activities to be carried out at the nation-wide scale.
In addition to the payback periods, water savings (%) with the scenarios of RWH and GWR through referring to the applications, namely, in toilet flushing, irrigation, floor cleaning, and car washing, are also important, and the outcomes of this study are given in Table 9.
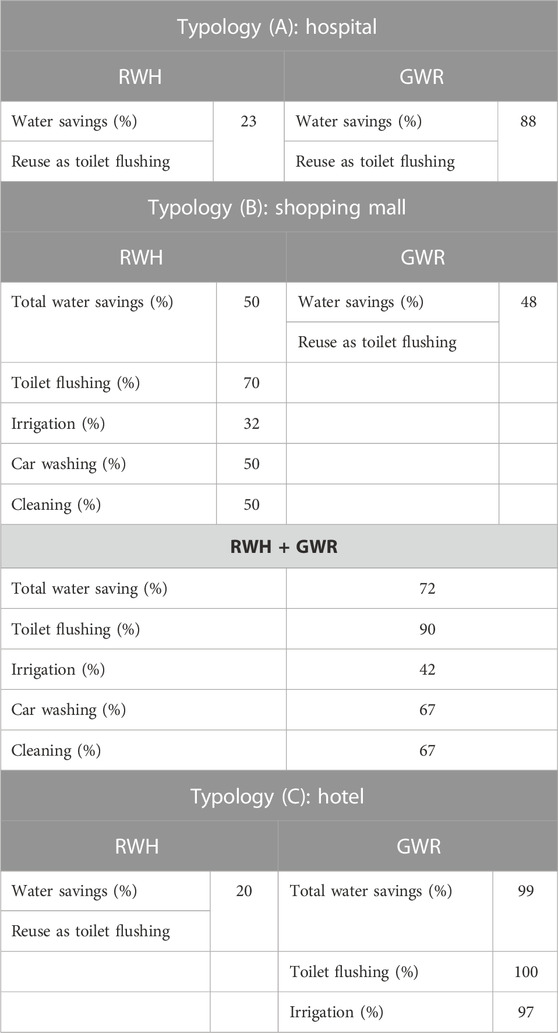
TABLE 9. Distribution of annual water savings for different building typologies through RWH and GWR systems.
4 Conclusion
Cost-benefit analyses of RWH and GWR systems for three different building typologies, a hospital, a shopping mall, and a hotel, existing in the Antalya Province of Türkiye constitute the main body of the article. Basic design criteria are considered for both of the alternative water resources during the design calculations, and the results are presented in the form of payback periods and water savings. Literature reports on the use of these alternative water resources state that the payback periods less than a decade seem to be highly satisfactory, and this finding has also been verified through the three typologies selected from the Antalya Province of Türkiye. Calculations based on the design criteria and corresponding assumptions based on the plumbing plans of the buildings covered the detailed preparation of the investment and operation costs together with the amortization times. This work carried out on real cases under operation put forth comprehensive examples that may be followed by other scientists and practitioners from different parts of the world dealing with the fulfillment of similar water reuse attempts and efforts.
The lessons learned from the studied cases may be evaluated according to their strengths and weaknesses. Both systems seem to be a convenient solution to water scarcity issues, especially in tourism sector-dominating provinces and cities which face vast population fluctuations over months of a year. The realization is that RWH is a comparatively easier water gaining system regarding its installation and operation. It does not necessarily need high technical expertise and expensive equipment and appurtenances. However, the harvested amount of water relies on the largeness of the effective roof surface area and the amount of precipitation that the region receives. In that sense, the methodology is easy to apply but is regarded as a system whose water flow rate changes spatially and temporally. It is again a comparatively safer system in terms of its quality rather than quantity. Total water savings through RWH were calculated to be 23% for typology A (hospital), 50% for typology B (shopping mall), and 20% for typology C (hotel). On the other hand, GWR requires more technical work than RWH as it needs a treatment facility. Nowadays, the package MBR modules are simply supplied by the manufacturers, and they also provide technical assistance during the operation of the overall system. For sure, there exists no water flow rate problem as GW is continuously generated from a building and it may safely be used for flushing the toilets after disinfection. As such, the utility of both water resources will lead to undeniable water savings. Water gain will in turn protect the receiving water bodies and diminish the pollution load of the wastewater treatment plants. Moreover, there will also be a financial benefit as the water bills will be highly reduced. Total water savings through GWR were calculated to be 88% for typology A (hospital), 48% for typology B (shopping mall), and 99% for typology C (hotel). One example was studied for both of the reuse alternatives for typology B where total water saving reached 72%. As seen from the saving values, high benefit can be obtained through these applications, and as such, freshwater resources may be more effectively used, particularly in the urbanized areas.
Last but not least, public awareness and raising public interest on the great benefit of these alternative systems is another issue that should be equally emphasized like their application by the governmental and local authorities. Furthermore, their practice may even be encouraged more by applying certain tax assistances similar to the undertaking of some countries as an important adaptation action toward climate change.
Data availability statement
The original contributions presented in the study are included in the article/Supplementary Material; further inquiries can be directed to the corresponding author.
Author contributions
AT and AH designed the study. AT, AH, and EG generated the methodology used in the study. EAK performed all the analyses and data processing as part of her master thesis. AH and EG prepared the details of Supplementary Materials. EAK, AH, and AT prepared the manuscript. All authors listed made a substantial, direct, and intellectual contribution to the manuscript and approved it for publication.
Conflict of interest
The authors declare that the research was conducted in the absence of any commercial or financial relationships that could be construed as a potential conflict of interest.
Publisher’s note
All claims expressed in this article are solely those of the authors and do not necessarily represent those of their affiliated organizations, or those of the publisher, the editors, and the reviewers. Any product that may be evaluated in this article, or claim that may be made by its manufacturer, is not guaranteed or endorsed by the publisher.
Supplementary material
The Supplementary Material for this article can be found online at: https://www.frontiersin.org/articles/10.3389/fenvs.2022.1080092/full#supplementary-material
References
Al-Batsh, N., Al-Khatib, I. A., Ghannam, S., Anayah, F., Jodeh, S., Hanbaly, G., et al. (2019). Assessment of rainwater harvesting systems in poor rural communities: A case study from yatta area, Palestine. Water 11, 585. doi:10.3390/w11030585
Al-Khatib, I. A., Al Shami, A. A. H. U., Garcia, G. R., and Celik, I. (2022). Social acceptance of greywater reuse in rural areas. J. Environ. Public Health 2022, 1–11. doi:10.1155/2022/6603348
Al-Saidi, M. (2021). From acceptance snapshots to the social acceptability process: Structuring knowledge on attitudes towards water reuse. Front. Environ. Sci. 9, 633841. doi:10.3389/fenvs.2021.633841
Anchan, S. S., and Shiva Prasad, H. C. (2021). Feasibility of roof top rainwater harvesting potential-a case study of South Indian University. Clean. Eng. Technol. 4, 100206. doi:10.1016/j.clet.2021.100206
Angelakis, A. N., Asano, T., Bahri, A., Jimenez, B. E., and Tchobanoglous, G. (2018). Water reuse: From ancient to modern times and the future. Front. Environ. Sci. 6, 26. doi:10.3389/fenvs.2018.00026
Angelakis, A. N., and Bontoux, L. (2001). Wastewater reclamation and reuse in Eureau countries. Water Policy 3 (1), 47–59. doi:10.1016/S1366-7017(00)00028-3
Anuja, J., Darshan, B., and Meyyappan, N. (2021). Study on reuse of grey water- a review. J. Phys. Conf. Ser. 1979, 012004. IOP Publishing. doi:10.1088/1742-6596/1979/1/012004
Atanasova, N., Dalmau, M., Comas, J., Poch, M., Rodriquez-Roda, I., and Buttiglieri, G. (2017). Optimized MBR for greywater reuse systems in hotel facilities. J. Environ. Manag. 193, 503–511. doi:10.1016/j.jenvman.2017.02.041
Aybuga, K., and Isıldar, G. Y. (2017). An evaluation of rain water harvesting and grey water reuse potential for Ankara. Sigma J. Eng. Nat. Sci. 8 (3), 209–216. Available at: https://sigma.yildiz.edu.tr/article/553.
Bell, L. M. (2018). “Examining the user experience in climate-adaptive policies: Tucson Arizona’s residential gray water recycling,”. MSc. Thesis (Faculty of the Graduate School of Cornell University), 121. In partial fulfillment of the requirements for the degree of master of science Available at: https://ecommons.cornell.edu/bitstream/handle/1813/64837/Bell_cornell_0058O_10406.pdf?sequence=1.
Bergel, T., Kotowski, T., and Woyciechowska, O. (2016). Daily water consumption for Household purposes and its variability in a rural household. J. Ecol. Eng. 17 (3), 47–52. doi:10.12911/22998993/63312
Borja-Vega, C. (2020a). What makes rural water systems sustainable? Meta-analysis, determinants, and the empirical impacts of a large-scale WASH program in Nicaragua. UK: Faculty of the Graduate School of Civil Engineering, 301. Available at: https://etheses.whiterose.ac.uk/27508/1/Borja-Vega%20Thesis200984810_Leeds%20CLEAN.pdf.
Borja-Vega, C. (2020b). What makes rural water systems sustainable? Meta-analysis, determinants, and the empirical impacts of a large-scale WASH program in Nicaragua. PhD. Thesis (Sheffield & York, United Kingdom: White Rose Libraries, Universities of Leeds).
Campisano, A., Butler, D., Ward, S., Burns Friedler, E., DeBusk, K., Fisher-Jeffes, L. N., et al. (2017). Urban rainwater harvesting systems research: Implementation and future perspectives. Water Res. 115, 195–209. doi:10.1016/j.watres.2017.02.056
CCIWR (2016). Climate change impacts on water resources of Turkey project (2013-2016) of the general directorate of water management under the Ministry of agriculture and forestry. Available at: http://iklim.tarimorman.gov.tr/Eng/.
Celik, I., Tamimi, L. M. A., Al-Khatib, I. A., and Apul, D. S. (2017). Management of rainwater harvesting and its impact on the health of people in the Middle East: Case study from yatta town, Palestine. Environ. Monit. Assess. 189 (6), 271. doi:10.1007/s10661-017-5970-y
Dogangonul, C., and Dogangonul, O. (2009). Small and medium stormwater use. 1st edition. Ankara, Türkiye: Ankara Teknik Publications. (in Turkish).
EC (2019). A European Green Deal, striving to be the first climate-neutral continent. Priorities 2019-2024. Available at: https://ec.europa.eu/info/strategy/priorities-2019-2024/european-green-deal_en.
EI (2021). Environmental indicators, general directorate of environmental impact assessment, permit and inspection, republic of Türkiye, Ministry of environment, urbanization and climate change. Ankara 234 Pages Available at: https://webdosya.csb.gov.tr/db/environmentalindicators/duyurular/csidb_cevresel_gostergeler_2022en_31agustos-20221007154853.pdf.
Elhegazy, H., and Eid, M. M. M. (2020). A state-of-the-art-review on grey water management: A survey from 2000 to 2020s. Water Sci. Technol. 82 (12), 2786–2797. doi:10.2166/wst.2020.549
EPA (2017). Water recycling and reuse: The environmental benefits. Available at: http://www.epa.gov/region9/water/recycling/(accessed October 05, 2022).
Eu, I. A. (2016). EU-Level instruments on water reuse final report to support the commission’s impact assessment, prepared by amec foster wheeler environment & infrastructure UK ltd, IEEP, ACTeon, IMDEA and NTUA. Available at: https://ec.europa.eu/environment/water/blueprint/pdf/EU_level_instruments_on_water-2nd-IA_support-study_AMEC.pdf.
EU (2018). Water reuse – legislative framework in EU regions, commission for the environment, climate change and Energy. European Committee of the Regions. Available at: https://cor.europa.eu/en/engage/studies/Documents/Water-reuse.pdf. doi:10.2863/846239
Farreny, R., Morales-Pinzo´n, T., Guisasola, A., Taya, T., Rieradevall, J., and Gabarrell, X. (2011). Roof selection for rainwater harvesting: Quantity and quality assessments in Spain. Water Res. 45, 3245–3254. doi:10.1016/j.watres.2011.03.036
Filali, H., Barsan, N., Souguir, D., Nedeff, V., Tomozei, C., and Hachicha, M. (2022). Greywater as an alternative solution for a sustainable management of water resources—a review. Sustainability 14 (2), 665. doi:10.3390/su14020665
Fu, L. (2018). Advancing rainwater harvesting systems to help mitigate the urban flooding problems in China. Athens, Georgia, USA: Master of Landscape Architecture. Available at: http://getd.libs.uga.edu/pdfs/fu_li_201805_mla.pdf.
Ghaffarian Hoseini, A., Tookey, J., Ghaffarian Hoseini, A., Yusoff, S. M., and Hassan, N. B. (2016). State of the art of rainwater harvesting systems towards promoting green built environments: A review. Desalination Water Treat. 57 (1), 1–10. doi:10.1080/19443994.2015.1021097
Ghunmi, L. A., and Eslamian, S. (2016). “Greywater,” in Urban water reuse handbook. Editor S. Eslamian (USA: CRC Press, Taylor and Francis Group), 405–420.
Gross, A., Maimon, A., Alfiya, Y., and Friedler, E. (2015). in Greywater reuse (USA: CRC Press, Taylor and Francis Group), 301.
Jorgensen, B., Graymore, M., and O’Toole, K. (2009). Household water use behavior: An integrated model. J. Environ. Manag. 91 (1), 227–236. doi:10.1016/j.jenvman.2009.08.009
Khajvand, M., Mostafazadeh, A. K., Drogui, P., Tyagi, R. D., and Brien, E. (2022). Greywater characteristics, impacts, treatment, and reclamation using adsorption processes towards the circular economy. Environ. Sci. Pollut. Res. 29, 10966–11003. doi:10.1007/s11356-021-16480-z
Lazarova, V., Hills, S., and Birks, R. (2003). Using recycled water for non-potable, urban uses: A review with particular reference to toilet flushing. Water Sci. Technol. Water Supply 3 (4), 69–77. doi:10.2166/ws.2003.0047
Mays, L., Antoniou, G. P., and Angelakis, A. N. (2013). History of water cisterns: Legacies and lessons. Water 5 (4), 1916–1940. doi:10.3390/w5041916
Merville-Screeve, R., Ward, S., and Butler, D. (2016). Rainwater harvesting typologies for UK houses: A multi criteria analysis of system configurations. Water 8, 129. doi:10.3390/w8040129
Mizyed, N. R. (2013). Challenges to treated wastewater reuse in arid and semi-arid areas. Environ. Sci. Policy 25, 186–195. doi:10.1016/j.envsci.2012.10.016
MoEU (2021). Antalya provincial environmental status report. Ankara: Ministry of Environment and Urbanization. Available at: https://webdosya.csb.gov.tr/db/ced/icerikler/antalya_2020_-cdr-20210812095503.pdf.
MoFA (2022). Turkey's policy on water issues, enterprising and humanitarian foreign policy, republic of Türkiye. Ankara: Ministry of Foreign Affairs. Available at: https://www.mfa.gov.tr/turkey_s-policy-on-water-issues.en.mfa.
Musayev, S., Burgess, E., and Mellor, J. (2018). A global performance assessment of rainwater harvesting under climate change. Resour. Conservation Recycl. 132, 62–70. doi:10.1016/j.resconrec.2018.01.023
Muthukumaran, S., Baskaran, K., and Sexton, N. (2011). Quantification of potable water savings by residential water conservation and reuse – a case study. Resour. Conservation Recycl. 55 (11), 945–952. doi:10.1016/j.resconrec.2011.04.013
Navarro, T. (2018). Water reuse and desalination in Spain – challenges and opportunities. J. Water Reuse Desalination 8 (2), 153–168. doi:10.2166/wrd.2018.043
Nawamed (2021). Nature based solutions for domestic water reuse in Mediterranean countries, European Union under the ENI CBC Mediterranean Sea Basin Programme (2014-2020). Available at: https://www.enicbcmed.eu/sites/default/files/2021-05/Output_3.1_Greywater%20reuse%20in%20NAWAMED%20countries.pdf.
Novotny, V. (2013). Water-energy nexus: Retrofitting urban areas to achieve zero pollution. Build. Res. Inf. 41 (5), 589–604. doi:10.1080/09613218.2013.804764
Ofori, S., Puškáčová, A., Růžičková, I., and Wanner, J. (2021). Treated wastewater reuse for irrigation: Pros and cons. Sci. Total Environ. 760, 144026. doi:10.1016/j.scitotenv.2020.144026
Okhravi, S., Eslemian, S., and Adamowski, J. (2016). “Water reuse in rainwater harvesting,” in Urban water reuse handbook. Editor S. Eslemian (USA: CRC Press, Taylor and Francis Group), 787–804.
Pistocchi, A., Bleninger, T., Breyer, C., Caldera, U., Dorati, C., Ganora, D., et al. (2020). Can seawater desalination be a win-win fix to our water cycle? Water Res. 182 (1), 115906. doi:10.1016/j.watres.2020.115906
Poff, N. L., Brown, C. M., Grantham, T. E., Matthews, J. H., Palmer, M. A., Spence, C. M., et al. (2016). Sustainable water management under future uncertainty with eco-engineering decision scaling. Nat. Clim. Change 6 (1), 25–34. doi:10.1038/nclimate2765
Potivichayanon, S., Sittitoon, N., and Vinneras, B. (2021). Exposure assessment of treated greywater reused for irrigation. Water Sci. Technol. Water Supply 21 (8), 4404–4417. doi:10.2166/ws.2021.191
Rahman, A., Keane, J., and Imteaz, M. A. (2012). Rainwater harvesting in Greater Sydney: Water savings, reliability and economic benefits. Resour. Conservation Recycl. 61, 16–21. doi:10.1016/j.resconrec.2011.12.002
Rodda, N., Salukazanaa, L., Jackson, S. A. F., and Smith, M. T. (2011). Use of domestic greywater for small-scale irrigation of food crops: Effects on plants and soil. Phys. Chem. Earth Parts A/B/C 36, 1051–1062. doi:10.1016/j.pce.2011.08.002
Schuetze, T. (2013). Rainwater harvesting and management – policy and regulations in Germany. Water Supply 13 (2), 376–385. doi:10.2166/ws.2013.035
SEGE (2017). Socio-economic development ranking research of provinces and regions. Ankara: General Directorate of Development Agencies under the Ministry of Industry and Technology. (in Turkish) Available at: https://www.sanayi.gov.tr/merkez-birimi/b94224510b7b/sege/il-sege-raporlari.
Sel, A. K. (2017). Investigation of rainwater over residential building scope in the context of sustainable design. MSc. Thesis. Istanbul, Türkiye: Istanbul Halic University Graduate School of Natural and Applied Sciences Architecture Program, 88. (in Turkish) Available at: https://acikbilim.yok.gov.tr/handle/20.500.12812/89021.
SF (2022). Onsite water recycling: An innovative approach to solving an old problem, san francisco water power sewer services. E-Book Available at: https://sfpuc.org/sites/default/files/construction-and-contracts/design-guidelines/Onsite%20Water%20Recycling%20E-book_2022_Final.pdf.
Shaikh, I. N., and Ahammed, M. M. (2022). Granular media filtration for on-site treatment of greywater: A review. Water Sci. Technol. 86 (5), 992–1016. doi:10.2166/wst.2022.269
Smit, M. (2019). “Rainwater harvesting in the 21st century – an Australian perspective,” in 3rd world irrigation forum (WIF3), 1-7 september 2019 (Bali, Indonesia. ST-1.1 W.1.1.31 Available at: https://www.icid.org/wif3_bali_2019/wif3_1-1_31-min.pdf.
Stec, A., and Slys, D. (2022). Financial and social factors influencing the use of unconventional water systems in single-family houses in eight European countries. Resources 11, 16. doi:10.3390/resources11020016
Stein, U. (2015). Water reuse and water quality aspects in Europe, CAPACITIE Training Course. Berlin: Ecologic Institute. Available at: https://www.ecologic.eu/sites/default/files/event/2015/22_steinwaterreusewaterquality_0.pdf.
Thaher, R. T., Mahmoud, N., Al-Khatib, I. A., and Hung, Y-T. (2020). Reasons of acceptance and barriers of house onsite greywater treatment and reuse in Palestinian rural areas. Water 12, 1679. doi:10.3390/w12061679
TSI (2021). Turkish Standards Institute, TS EN16941-2 Non-potable water systems - Part 2: Systems for the use of treated grey water (sink wastewater). Ankara: TSI Standards Services.
URL1 (2022). Turkey's first climate Council convened in konya. Available at: https://ab.csb.gov.tr/en/turkeys-first-climate-council-convened-in-konya-news-267433 (accessed on October 05, 2022).
Vieira, P., Jorge, C., and Covas, D. (2017). Assessment of household water use efficiency using performance indices. Resour. Conservation Recycl. 116, 94–106. doi:10.1016/j.resconrec.2016.09.007
Vuppaladadiyam, A. K., Merayo, N., Prinsen, P., Luque, R., Blanco, A., and Zhao, M. (2019). A review on greywater reuse: Quality, risks, barriers and global scenarios. Rev. Environ. Sci. Bio/Technology 18, 77–99. doi:10.1007/s11157-018-9487-9
Wilderer, P. A. (2004). Applying sustainable water management concepts in rural and urban areas: Some thoughts about reasons, means and needs. Water Sci. Technol. 4, 7–16. doi:10.2166/wst.2004.0403
Yannopoulos, S., Giannopoulou, I., and Kaiafa-Saropoulou, M. (2019). Investigation of the current situation and prospects for the development of rainwater harvesting as a tool to confront water scarcity worldwide. Water 11 (10), 2168. doi:10.3390/w11102168
Yu, Z. L. T., DeShazo, J. R., Stenstrom, M. K., and Cohen, Y. (2016). Cost–benefit analysis of onsite residential graywater recycling: A case study on the city of los angeles. J. Am. Water Works Assoc. 108, E436–E444. doi:10.5942/jawwa.2015.107.0124
Yu, Z. L. T., Rahardianto, A., DeShazo, J. R., Stenstrom, M. K., and Cohen, Y. (2013). Critical review: Regulatory incentives and impediments for onsite graywater reuse in the United States. Water Environ. Res. 85 (7), 650–662. doi:10.2175/106143013X13698672321580
Zavala, M. A. L., Vega, R. C., and Miranda, R. A. L. (2016). Potential of rainwater harvesting and greywater reuse for water consumption reduction and wastewater minimization. Water 8, 264. doi:10.3390/w8060264
Zhang, L., Njepu, A., and Xia, X. (2021). Minimum cost solution to residential energy-water nexus through rainwater harvesting and greywater recycling. J. Clean. Prod. 298, 126742. doi:10.1016/j.jclepro.2021.126742
Keywords: Antalya, alternative water resources, graywater reuse, rainwater harvesting, payback period, Türkiye
Citation: Kilinc EA, Tanik A, Hanedar A and Gorgun E (2023) Climate change adaptation exertions on the use of alternative water resources in Antalya, Türkiye. Front. Environ. Sci. 10:1080092. doi: 10.3389/fenvs.2022.1080092
Received: 25 October 2022; Accepted: 12 December 2022;
Published: 04 January 2023.
Edited by:
Irene Voukkali, Open University of Cyprus, CyprusReviewed by:
Issam A. Al-Khatib, Birzeit University, PalestineFlora Silva, Polytechnic Institute of Bragança (IPB), Portugal
Copyright © 2023 Kilinc, Tanik, Hanedar and Gorgun. This is an open-access article distributed under the terms of the Creative Commons Attribution License (CC BY). The use, distribution or reproduction in other forums is permitted, provided the original author(s) and the copyright owner(s) are credited and that the original publication in this journal is cited, in accordance with accepted academic practice. No use, distribution or reproduction is permitted which does not comply with these terms.
*Correspondence: Aysegul Tanik, tanika@itu.edu.tr