- 1Tropical Forests and People Research Centre, University of the Sunshine Coast, Maroochydore, QLD, Australia
- 2Department of Earth and Environment, Florida International University, Miami, FL, United States
- 3Department of Soil Science, College of Agriculture and Food Sciences, Visayas State University, Baybay, Leyte, Philippines
- 4College of Marine and Environmental Sciences, James Cook University, Townsville, QLD, Australia
Shifting cultivation is a dominant land-use in the tropical forest-agriculture frontier in Southeast Asia and is blamed for much of the environmental degradation in the region. We examined the distribution and availability of four soil macronutrients—i.e., soil organic carbon (SOC), total nitrogen (N), phosphorus (P) and potassium (K), in secondary forests regenerating after shifting cultivation abandonment. Soil samples were collected along an upland fallow gradient on Leyte Island in the Philippines. The effect of site environmental attributes on the availability of SOC and nutrients was investigated using linear mixed-effect models. We found relatively higher concentrations of SOC and P in the oldest fallows and higher N concentration in the youngest fallow secondary forest. There was no significant difference in SOC and other macronutrients within sites of different fallow categories and soil depths, except in the case of soil K, which was highest in our control old-growth forest. Patch size together with slope of the site and fallow age were the most influential factors in explaining the variability in SOC and nutrients availability in secondary forests recovering after shifting cultivation abandonment. Our study suggests that shifting cultivation may not be detrimental to soil quality, at least on the soil parameters and soil type we studied in the Philippines upland.
Introduction
Shifting cultivation, swidden or slash-and-burn agriculture, is a common land-use in the tropical forest-agriculture Frontier and considered as a major source of forest degradation (Ziegler et al., 2011; Curtis et al., 2018). Generally, shifting cultivation involves slashing and burning of forest vegetation before, or at the onset, of the monsoon to release nutrients locked in plant biomass; the sites are then cultivated and harvested over several years before they are left fallow to allow secondary forest development (Fox et al., 2000). Much of the forests in the tropics have been subjected to shifting cultivation, and this is a major contribution to the livelihood and food security of smallholder rural farmers living in upland rural areas (Mertz et al., 2009a; van Vliet et al., 2012; Heinimann et al., 2017).
Although a reliable estimate of the extent of land under shifting cultivation is not available, according to Mertz et al. (2009b) at least 14–34 million people are dependent on shifting cultivation alone in Asia. In the last few decades, a rapid transformation of shifting cultivation landscapes, however, has taken place in much of the Southeast Asia, mainly driven by urbanization, and changing land-use policies and focus of the governments (Fox et al., 2009; van Vliet et al., 2012). Another threat is posed by large-scale plantations of commercial and perennial crops including rubber and oil palm in lands where shifting cultivation was prevalent (Ziegler et al., 2012; Ahrends et al., 2015; Terefe and Kim 2020). Yet shifting cultivation is regarded as one of the major drivers of deforestation and forest degradation in this region and hence is discouraged in local land-use policies (Hett et al., 2012; Mukul et al., 2016a).
Soil quality is one of the key environmental attributes that is largely influenced by shifting cultivation, and other human use and local practices in the humid tropics (Kotto-Same et al., 1998; Hughe et al., 1999; Bruun et al., 2009; Lawrence et al., 2010; Filho et al., 2015; Zhang et al., 2019). Soil quality is the capacity of the soil to support forest growth without causing further degradation of the soil or to the environment (Lal 1997). Soil quality is closely linked to soil resilience which refers to the ability of the soil to restore its functions following major disturbances (Guo and Gifford 2002; Filho et al., 2015). The environmental aspects of shifting cultivation, however, are more complex than is often presented in the literature, and the transformations of shifting cultivation landscapes to other land-uses may have a wide range of environmental consequences, both at local and global levels (Bruun et al., 2009; van Vliet et al., 2012; Mukul and Herbohn 2016). The transition of shifting cultivation landscapes to sedentary agriculture, for instance, will bring a substantial reduction in the above and below ground carbon stocks compared to the transition of shifting cultivation landscapes to secondary forests (Ziegler et al., 2012; van Straaten et al., 2015).
The effect of anthropogenic forest disturbances, including shifting cultivation, on soil carbon and other nutrients are still unclear, and characterized by data scarcity and inconclusiveness (see—Richards et al., 2007; de Neergaard et al., 2008; Bruun et al., 2009; Sang et al., 2013; Paudel et al., 2015; de Blecourt et al., 2017; Teixeira et al., 2020; Mukul et al., 2021). Consequently, we investigated whether shifting cultivation has major impacts on key soil macronutrients and soil quality indicators namely—soil organic carbon (SOC), total nitrogen (N), phosphorus (P) and potassium (K) in secondary forests regenerating after shifting cultivation abandonment. In the Philippines, shifting cultivation, locally known as kaingin, represents a dominant land-use (Saurej and Sajise 2010). After logged forest, post-kaingin forest constitutes the largest group of secondary forests in the country (Chokkalingam and Perera 2001).
The specific objectives of our study were—1) to determine the status of SOC, total N, P, and K in regenerating forests recovering after shifting cultivation abandonment in the Philippines uplands, and 2) to identify the effect of site environmental attributes on soil carbon and nutrient recovery. Understanding soil C and nutrients dynamics after shifting cultivation abandonment also have wider implications for forest management and restoration, not only in the Philippines, but in other parts of the humid tropics where large areas of land are now recovering from anthropogenic and other disturbances (see—Bruun et al., 2009; Sang et al., 2013; Smith et al., 2015; Thomaz 2017; Ota et al., 2020; Mukul et al., 2020, 2022).
Materials and methods
The study area
About 23 percent of the total land area in the Philippines is classified as uplands with elevation ranging between 100 and 500 m asl (Carating et al., 2014). Our study was conducted in an upland area situated on the western side of the island of Leyte (Figure 1). Leyte is the eighth largest island in the country with an area of about 800,000 ha and located between 124°17/ and 125°18/ East longitude and between 9°55/ and 11°48/ North latitude. Forests cover only about 10 percent area in Leyte. The once dipterocarp rich rainforests of the island are now dominated by patches of old-growth forest, secondary forest, coconut plantations, Abaca (Musa textilis, a fiber yielding species from the Musaceae) and fast-growing timber plantations (Asio et al., 1998; Bonner et al., 2019).
According to the Coronas Climate Classification, Leyte has a “type IV” climate with two distinct seasons—monsoon and dry. The annual rainfall is about 4,000 mm with a mean annual temperature of about 28°C that varies little throughout the year (Mukul et al., 2016a). The relative humidity of the island ranges from 75 to 80 percent (Kolb 2003). The soil on Leyte island is mostly Andisol which possesses markedly higher concentrations of organic carbon than other parts of the country (Navarrete et al., 2013).
Site selection and characteristics
We purposively selected Barangay (administrative entity, similar to a village) Gaas (hereafter Gaas only) on Leyte Island because the area has a relatively low population density and forests are relatively undisturbed there, which is one of the prerequisites for the development of second-growth forests (Chazdon 2014). Kaingin in the Philippines can be categorized into three distinct types based on the sites where it is practiced: the tubigan, the katihan and the dahilig systems (Olofson 1980). The tubigan and katihan systems are practiced in lower elevation areas or on gently sloping land with limited irrigation facilities. In contrast, the dahilig system is widely practiced in heavily forested areas and on steeper slopes (Olofson 1980). For our study, we focused, only on the dahilig system because it represents the shifting cultivation system widespread in most parts of Southeast Asia (Chokkalingam and Perera 2001).
Altogether 25 sites (5 categories x 5 replicates) were established in the area. We took samples from four different fallow age categories, i.e. less than (or equal to) 5 years old fallow (SA0-5, also referred to as new fallows), 6–10 years old fallow (SA6-10, also referred to as young fallows), 11–20 years old fallow (SA11-20, referred to as middle-aged fallows), and 21–30 years old fallow (SA21-30, also referred to as oldest fallows), and from old-growth forests (OF) as our control. Our old-growth forests are similar to the primary forests in terms of structure and floristic composition, never been used for kaingin and/or logging, however, may have experienced limited levels of anthropogenic disturbances, like fuelwood and fodder collection. We took samples only from sites that were 1 ha or more in size. The fallow age was confirmed by asking the kaingin farmer engaged in shifting cultivation at each site. A vegetation survey was conducted at each site and all trees ≥5 cm dbh were identified and measured for tree species richness, abundance and basal area (Mukul 2016). To measure site elevation, geographic position, and distance from the nearest old-growth control forest, we used a hand-held global positioning system (Model: Garmin eTrex). A digital plant canopy imager (Model: CID Bio-Science, CI-110/120) was used to measure the leaf area index of each site. Table 1 presents the key environmental attributes of our study sites.
Soil sample collection
We established four transects of 50 m × 5 m in each of the 25 sites. Within each site, transects were spaced at least 5 m apart, parallel to each other and running from the boundary to the center of the site. Soil samples were collected from the beginning, middle, and end of each transect. Samples from the top 30 cm of topsoil were obtained. We collected soil samples from three different depths, i.e., 0-5 cm (also referred to as the top layer), 6–15 cm (the middle layer) and 16–30 cm (the bottom layer) using a standard soil auger (Manufacturer: AMS Inc., United States). The topsoil profile is widely reported to be most affected by land-use/cover change (Bruun et al., 2009; Sang et al., 2013); and is also the standard depth recognized by the Food and Agriculture Organization of the United Nations (FAO) and approved by Intergovernmental Panel on Climate Change (IPCC) for global voluntary carbon markets (FAO 2006). Altogether 900 soil samples were collected from 100 transects distributed across 25 sites. Samples were placed in plastic bags in the field immediately after collection and labeled before further processing in the laboratory.
Soil sample processing
All analyses were performed in the Philippines in the Visayas State University (VSU) Analytical Chemistry Laboratory. Samples were air-dried at room temperature before being passed through a 2 mm sieve to remove rocks, pebbles and plant materials (e.g., roots, coarse woody debris, etc.). We used a homogenized composite sample (n = 3) pooled for each soil depth (i.e., top, middle, and bottom layer) for each transect. Consequently, we had 300 composite soil samples for laboratory analysis to determine SOC, N, P, and K. Subsamples were oven dried (105°C for 48 h), and all calculations are reported on an oven-dry weight basis.
For SOC (% C) we oxidized the composite soil samples for each transect by heating with H2SO4 in the presence of dichromate as described in Heanes (1984). Unlike the traditional Walkley-Black method, external heating ensures complete oxidation and a more reliable estimate of SOC (Krishan et al., 2009; Congdon 2012). Soil N and P were determined colorimetrically following the single digestion method (Anderson and Ingram 1989), while soil total K was quantified using an atomic absorption spectrophotometer. Soil carbon and nutrient turnover are expressed as the percentage of respective soil parameters in our control old-growth forest. Soil physical and chemical properties, like bulk density, moisture content and pH were also analyzed following standardized procedures and protocols (Table 2).
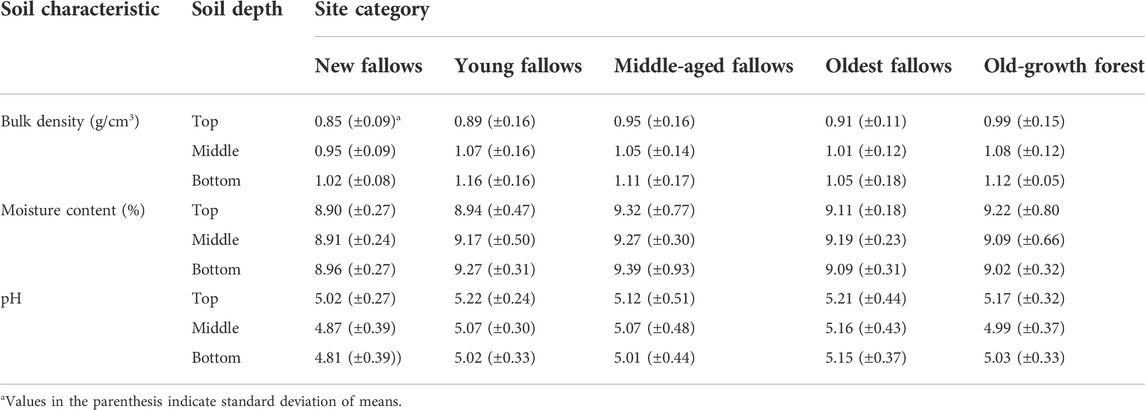
TABLE 2. Soil physical and chemical characteristics of our study sites on Leyte Island, the Philippines.
Statistical analysis
We performed an analysis of variance (ANOVA) and Tukey’s post-hoc analysis to test for significant differences among variables. We developed linear mixed-effect models (hereafter refer to as LMEM) to identify the effect of selected site environmental attributes on the SOC, N, P and K concentration in relation to our control old-growth forests using the package “nlme” (Pinheiro et al., 2011). For LMEM along with fallow age (FA), we first considered eight environmental variables as our explanatory variables (i.e. fixed factors)—elevation (EL), slope (SL), patch size (PS), distance from the nearest control old-growth forest (DIS), tree species richness (N), tree species abundance (A), basal area (BA), and leaf area index (LAI). Tree species richness, abundance, basal area, and leaf area index were expressed per site (0.1 ha area) basis. After performing the Pearson’s correlation test only five site environmental variables (i.e., fallow age, elevation, slope, patch size, and leaf area index) were, however, found suitable for our final LMEM (Supplementary Table S1). We used sites nested in fallow categories as the random effect in our LMEM with SOC, N, P, and K at different soil depths as the response variable. All statistical analyses were performed using the ‘R’ Statistical Package (version 3.0.1; R Development Core Team 2019). We considered the Akaike Information Criterion corrected for small sample sizes (AICc) for the selection of our best models. In our study, only models within four AICc units were considered as competing models (Grueber et al., 2011). We used the package “MuMin” for model selections and to evaluate the contribution that different fixed effects had on explaining the variation in the response variables.
Results
Soil carbon and nutrients in regenerating tropical secondary forests
Soil organic carbon and the other macronutrients were consistently high in the top (i.e., 0-5 cm) soil layer of our fallow sites and control old-growth forest. We found higher SOC concentrations in our oldest fallows followed by young fallows and in new fallows (Table 3; Supplementary Figure S1). The differences in soil C distribution was not significant in the case of the top layer of soil, whilst in the middle (6–15 cm) and bottom (16–30 cm) layers it was significantly (F4, 24 = 2.74, p < 0.05) different across the sites. Tukey’s post-hoc analysis also revealed significantly (p < 0.05) higher SOC concentrations in our middle and bottom soil layers in the oldest fallows followed by young and new fallows. The relative contribution of the SOC at different soil depths to soil C pool was consistently higher in the bottom layer of soil. In the topsoil layer, SOC concentration was relatively higher in our control old-growth forest (26.5%), followed by the middle-aged sites (25.5%) (Figure 2). In the case of the oldest fallows, the relative contribution of SOC in the bottom layer was comparatively higher (46.2%) than all other fallow age categories.
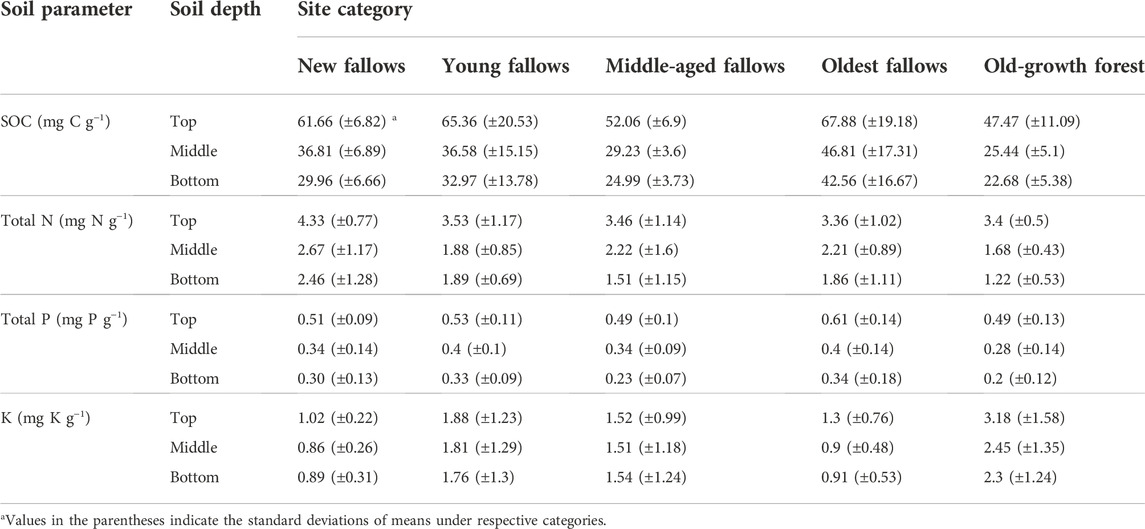
TABLE 3. Concentrations of soil organic carbon and nutrients across the sites of different fallow categories and old-growth forest on Leyte Island, the Philippines.
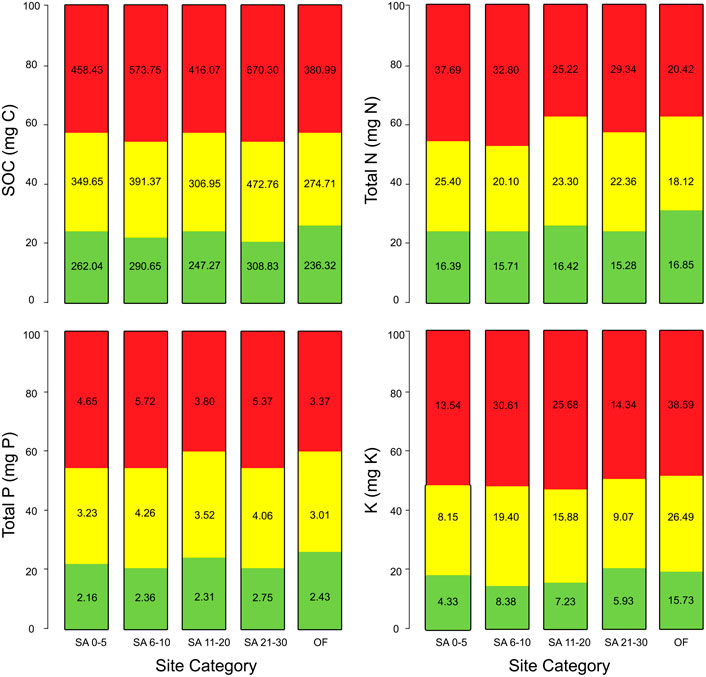
FIGURE 2. The relative contribution of soil C and other nutrients at different soil depths in our sites on Leyte Island, the Philippines. The Y-axis here indicates the percentage (%) contribution to the total soil carbon and nutrients pools at the three soil depths studied. The values within the bars are soil macronutrients (mg) in different site categories adjusted after respective bulk density and soil depths.
Interestingly soil total N concentrations were highest in the new fallow sites and in the top layer of the soil that we investigated (see Table 3). We found no significant difference in the soil N distribution between different site categories and soil depths as indicated by ANOVA. The relative contribution of soil N present in different soil depths to total N pool in our control old-growth forest was comparable (30.4%, 32.7%, and 36.9%). Among all fallow age categories, the relative contribution of soil N in the bottom layer (47.8%) was higher in our young fallow sites.
Similar to SOC and N there was no significant difference in total P concentrations in our study sites distributed across different fallow age categories and old-growth forests without any kaingin history (Supplementary Figure S1). The concentrations of soil P were, however, higher in the oldest fallows and in the topsoil layer of all fallow age categories although the differences were not statistically significant using ANOVA (Table 3). The relative contribution of soil P at the top and bottom layers varied substantially across our sites. Similar to other soil macronutrients, the contribution of soil P in the bottom layer to total soil P pool was consistently higher in our sites of different fallow age and old-growth forest (Figure 2).
Soil total K concentration was highest in the old-growth forest at all soil depths followed by young fallows and middle-aged fallows (Table 2). We found significantly higher (F4, 24 = 3.16, p < 0.05) soil K concentrations in the top layer of soil of our old-growth forest using ANOVA and Tukey’s post-hoc analysis. The difference was, however, not significant in the middle (F4, 24 = 2.15, p = 0.11) and bottom (F4, 24 = 1.73, p = 0.18) soil layers.
Recovery of soil carbon and nutrients in regenerating tropical secondary forests
We found high SOC, soil N and soil P in our fallow sites when compared with control old-growth forest (Figure 3). Interestingly, the recovery of soil macronutrients was higher in our young fallow and oldest fallow secondary forest sites. Among the soil nutrients, soil K recovery was lowest across all fallow age categories. The pattern of soil K recovery was different to soil C and N and P, with relatively higher recovery in middle-aged fallows. Soil K recovery was lowest in the oldest fallows. Despite relatively higher variability within the sites in soil carbon and nutrient turnover, there was no significant difference between sites (of different fallow age) in the recovery of selected soil parameters.
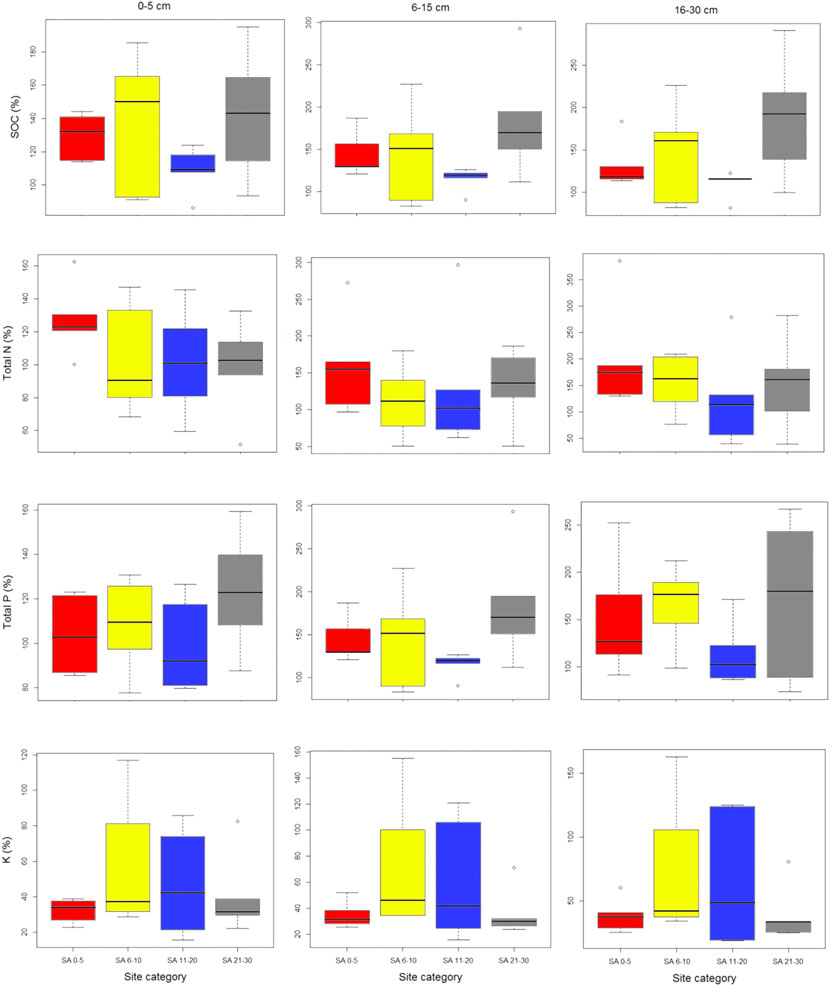
FIGURE 3. Recovery of soil carbon and nutrients at our study sites on Leyte Island, the Philippines. Note that different scales are used on the Y axes.
Environmental controls on the recovery of soil carbon and nutrients in regenerating tropical secondary forests.
Patch size (PS) explained the highest amount of variation in soil C and nutrients recovery in regenerating secondary forests (Table 4). Other site factors important in explaining the variations were slope (SL) and fallow age (FA). There was no influence of the distance from nearby old-growth forest (DIS) to the recovery of the soil C and nutrients across our sites of different fallow age categories. Patch size was found to be consistently important in explaining the variation in recovery for all the soil parameters and soil depths we examined (Table 5). In the case of soil P recovery, we also found that the slope of the sites was equally important in explaining the variation as the patch size.
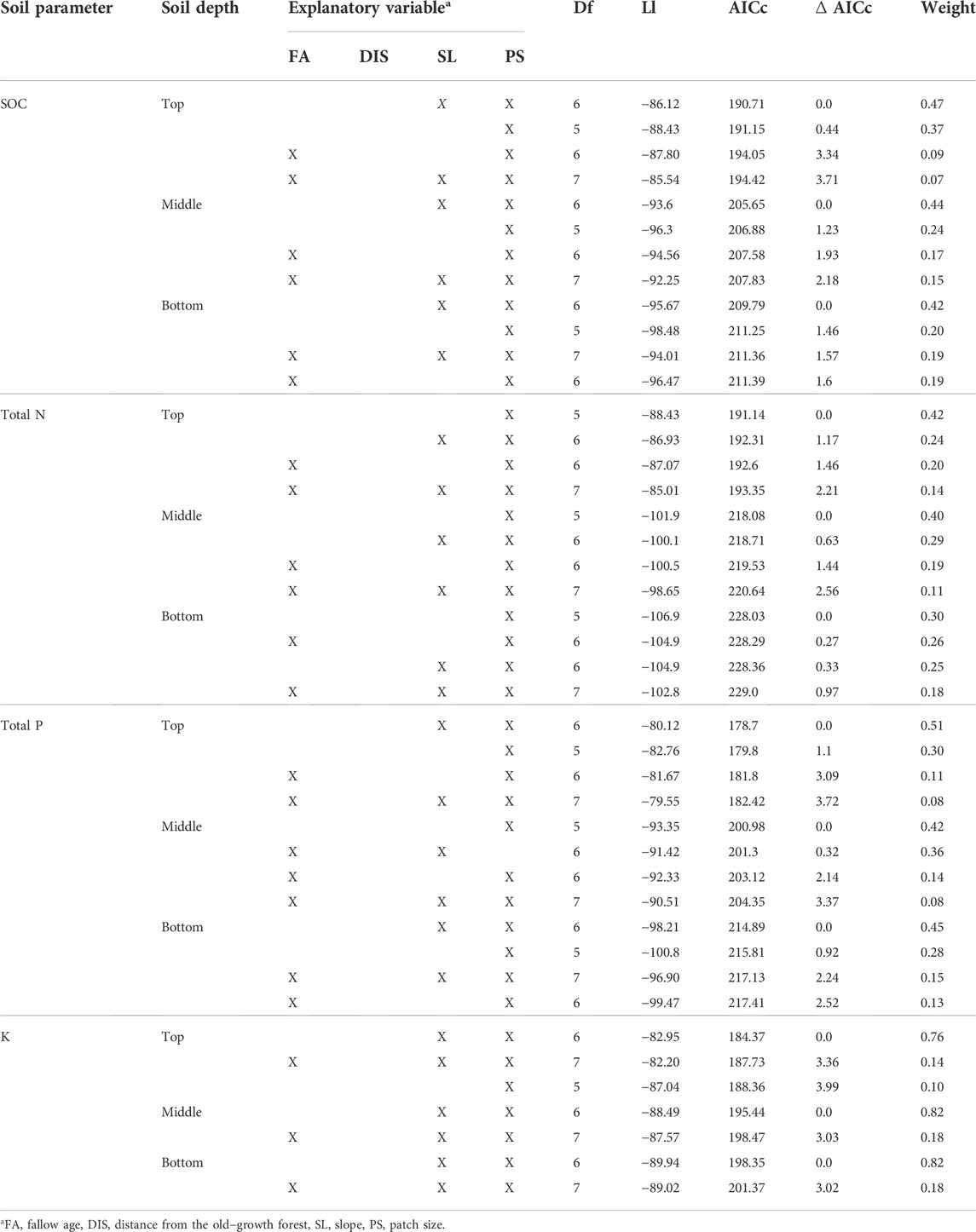
TABLE 4. Summary of LMEM between soil C and nutrient recovery with environmental attributes obtained using the package MuMin. Where, DF—Degree of Freedom, LL—Log Likelihood, AICc—Akaike Information Criterion corrected for small sample size.
Discussion
Variability in soil carbon and nutrients in regenerating tropical secondary forests
We did not find a clear or consistent effect of shifting cultivation on SOC, N, and P in our secondary forest sites in the upland Philippines. However, we did find a relatively greater influence of shifting cultivation on soil K concentration in our study sites in upland Philippines. The higher levels of soil C and nutrients (i.e., N and P) in our young fallows could be due to the initial release of carbon and nutrients from plant materials to soil following the burning (Giardina et al., 2000; Tanaka et al., 2001). Interestingly, we find a high variability of SOC within our sites of different fallow categories, which may be due to the past kaingin history attributed to previous fallow cycles that we could not capture. It may also be due to litterfall dynamics or soil below−ground activity, such as microbial presence or fine root biomass in specific sites (see—Sayer et al., 2011; Langee et al., 2014; Filho et al., 2015; Sarai et al., 2022). Heating of soil during burning acts as an important mechanism of nutrient release and the initial burning associated with shifting cultivation is reported to increase SOC by the addition of organic matter in soil via ash (Tanaka et al., 2001; Thomaz 2017). Burning also results in losses of soil available N due to increased volatilization, although, the losses of soil P to the atmosphere due to volatilization is relatively low (Giardina et al., 2000; Romanyá et al., 2001; Taylor et al., 2017). In shifting cultivation landscapes, burning also results in a decrease in soil microbial activity and associated biomass at the initial stage that may negatively affect soil carbon (Tanaka et al., 2001; Mukul and Herbohn 2016; Hattori et al., 2019). In regenerating forests, N deposition is also coupled with enhanced productivity, thus greater C in soil and living biomass (Thomaz 2017).
The relatively lower concentration of soil C and P in our middle−aged fallows could be attributed to the increasing nutrient uptake by regenerating forests during successional development (Filho et al., 2015). Similarly, relatively greater concentrations of soil C, N, and P in oldest fallows could be due to higher litterfall, decomposition and microbial activity in regenerating forests recovering after shifting cultivation (see—Sayer et al., 2011; Lange et al., 2015; Paudel et al., 2015; Teegalapalli et al., 2018; Jones et al., 2019). A positive effect of shifting cultivation on soil available P and K in secondary forests has also been reported by Brand and Pfund (1998). Temjen et al. (2022) found a relatively higher concentration of SOC, available N, P, and K with increase in fallow period in Nagaland, India. In contrast to our findings, Garcia−Oliva et al. (1999), however, have found a 32% initial decrease in soil organic C stock due to fire and combustion in Mexico, and Yang et al. (2003) found a reduction in the soil available N and P due to shifting cultivation in China. Soil K was consistently low at all our fallow sites, although Yang et al. (2003) reported an increase in soil K after fire and shifting cultivation. Other than combustion during the burning of vegetation, soil runoff associated with forest clearing during shifting cultivation has also been reported to cause nutrient losses (Brand and Pfund 1998; Rodenburg et al., 2003; Thomaz 2017; Hattori et al., 2019). Such generalizations, however, require careful consideration because in the tropics smallholder farmers usually prefer sites with greater soil fertility for their shifting cultivation practice (Mertz et al., 2008).
Influence of site environmental factors on the recovery of soil carbon and nutrients
We found that site environmental parameters, mainly patch size, have the greatest influence on the recovery of SOC, total N, and P; and we have found that the same factors influence the aboveground biomass and carbon recovery in regenerating forests after shifting cultivation in Philippines uplands (see Mukul et al., 2016b). As mentioned earlier, the higher levels of soil organic carbon and nutrients in our fallow sites could also be due to the initial release of carbon and nutrients following the burning of biomass and may not portray the actual recovery in young fallows. In the case of soil K, both the patch size and slope were found to be equally important for its recovery. Patch size, however, was found to be the most important factor as models within ∆AICc = 4 are considered as equivalent and explain the same amount of variation as other more complex models incorporating more interactions of variables (Bartoń 2011). Due to the lack of reliable information about past fallow cycles, we did not take account of this in our analysis. It is thus unclear what impact past fallow cycles may have had on the soil C and nutrients in our fallow sites. In an Indonesian study, the number of fallow cycles did not influence soil nutrients, like soil P, although the presence of deep and fine root systems in soil was found to be more important than fire history in the recovery of soil P (Lawrence and Schlesinger 2001). A similar observation was also made by Bruun et al. (2009) where they found higher growth of pioneer species in fallows due to their shallow root systems and the ability to take up nutrients from the top layer. The effect of land−use and site environmental attributes on soil C and nutrients is evident from many other studies (see—Neill et al., 1997; van Noordwijk et al., 1997; Hatter et al., 2010; Parras−Alcantara et al., 2013; Fernandez−Romero 2014). A long fallow period is critical to restoring essential soil nutrients, and it may take as long as 20 years to restore soil nutrients to a level similar to undisturbed forests (Funakawa et al., 2009; Rahamiralala et al., 2010). Forest structure and species composition may also influence the dynamics of soil C and nutrients, and vice versa (Lawrence et al., 2005; Firn et al., 2007; Paoli et al., 2008; Lange et al., 2014; Raich et al., 2014). Furthermore, spatial heterogeneity in soil in terms of texture, clay mineralogy and site topography and climate may vary and can also override the effects of other environmental factors (Bruun et al., 2006; Nottingham et al., 2015).
Conclusion
Our study finds that shifting cultivation may not be as detrimental to soil quality at least on the soil type and climate we studied, and that geographic and site−specific conditions may be important in determining the impact that shifting cultivation has on soil properties. We also found greater availability in the soil C, N and P in regenerating tropical secondary forests compared to old−growth forests after shifting cultivation abandonment. In young fallows, this may be attributed to the initial release of carbon and nutrients from slashing and burning of plant biomass available in the sites. In our old−growth control forests, there might be much more C, N, P, and K present in the biomass (above and below ground). In older fallow sites, repeated clearing, burning and run−off from the cleared landscape presumably led to the loss of much of the stored nutrients. In addition, over time, more of these nutrients are expected to be sequestered in the developing vegetation, leading concentrations of soil macronutrients to change over time due to plant uptake and recycling through litter fall and decomposition. We find, patch size together with the slope and fallow age significant explanatory factors associated with the recovery process of soil macronutrients.
In tropical regions shifting cultivation is still a dominant land−use and likely to contribute to the food security and livelihoods of smallholder farmers for many years ahead. The traditional view of shifting cultivation as environmentally detrimental in terms of its impacts on soil is not well established (Murty et al., 2002). A better understanding of this issue, with systematic and long−term studies, is crucial for the restoration and management of tropical forests recovering after shifting cultivation and other anthropogenic disturbances.
Data availability statement
The raw data supporting the conclusion of this article will be made available by the authors, without undue reservation.
Author contributions
SM: conceptualization. SM and JH: project administration. SM, JH, AF, and RC: methodology. SM, AF, and RC: investigation. SM: data curation, formal analysis, and writing. JH, AF, and RC: review and editing. JH: supervision and funding. All authors contributed to the article and approved the submitted version.
Funding
This work was made possible by a scholarship (International Postgraduate Research Scholarship) to SM from the Australian Government while pursuing his doctoral study at The University of Queensland, and support to JH from the Australian Centre for International Agricultural Research (Projects: ASEM/2010/50 and ASEM/2016/103).
Acknowledgments
We would like to thank Drs Nestor Gregorio, Arturo Pasa and VSU−ACIAR field staff especially Nayar Adanarg, Val Solano, Jun Ray, and Cris Solano for their support during the fieldwork. Thanks are also due to Ofelia Maranguit, Jertz Escala, Jun Zhang and Mayet Avela for their assistance in the laboratory analysis, and to Professors Jennifer Firn, Susanne Schmidt, David Lamb and L. A. Bruinjeel for their valuable feedback at different stages of this work.
Conflict of interest
The authors declare that the research was conducted in the absence of any commercial or financial relationships that could be construed as a potential conflict of interest.
Publisher’s note
All claims expressed in this article are solely those of the authors and do not necessarily represent those of their affiliated organizations, or those of the publisher, the editors and the reviewers. Any product that may be evaluated in this article, or claim that may be made by its manufacturer, is not guaranteed or endorsed by the publisher.
Supplementary material
The Supplementary Material for this article can be found online at: https://www.frontiersin.org/articles/10.3389/fenvs.2022.1076506/full#supplementary-material
References
Ahrends, A., Hollingsworth, P. M., Ziegler, A. D., Fox, J. M., Chen, H., Su, Y., et al. (2015). Current trends of rubber plantation expansion may threaten biodiversity and livelihoods. Glob. Environ. Change 34, 48–58. doi:10.1016/j.gloenvcha.2015.06.002
Anderson, S. E., and Ingram, J. S. I. (1989). Tropical soil biology and fertility: A handbook of methods. Aberystwyth: C.A.B. International.
Asio, V. B., Jahn, R., Stahr, K., and Margraf, J. (1998). “Soils of the tropical forests of Leyte, Philippines II: Impact of different land uses on status of organic matter and nutrient availability,” in Soils of tropical forest ecosystems: Characteristics, ecology and management. Editors A. Schulte, and D. Ruhiyat (New York, NY, USA: Springer), 37–44.
Bartoń, K. (2011). Package ‘MuMIn. Available at: http://cran.r−project.org/web/packages/MuMIn/MuMIn.pdf.
Bonner, M. T. L., Herbohn, J., Gregorio, N., Pasa, A., Avela, M. S., Solano, C., et al. (2019). Soil organic carbon recovery in tropical tree plantations may depend on restoration of soil microbial composition and function. Geoderma 353, 70–80. doi:10.1016/j.geoderma.2019.06.017
Brand, J., and Pfund, J. L. (1998). Site and watershed level assessment of nutrient dynamics under shifting cultivation in eastern Madagascar. Agric. Ecosyst. Environ. 71, 169–183. doi:10.1016/s0167−8809(98)00139−x
Bruun, T. B., de Neergaard, A., Lawrence, D., and Ziegler, A. D. (2009). Environmental consequences of the demise in swidden cultivation in Southeast Asia: Carbon storage and soil quality. Hum. Ecol. 37, 375–388. doi:10.1007/s10745−009−9257−y
Bruun, T. B., Mertz, O., and Elberling, B. (2006). Linking yields of upland rice in shifting cultivation to fallow length and soil properties. Agric. Ecosyst. Environ. 113, 139–149. doi:10.1016/j.agee.2005.09.012
Carating, R. B., Galanta, R. G., and Bacatio, C. D. (2014). The soils of the Philippines. New York, NY, USA: Springer.
Chazdon, R. L. (2014). Second growth: The promise of tropical forest regeneration in an age of deforestation. Chicago, IL, USA: University of Chicago Press.
Chokkalingam, U. W. U., and Perera, G. A. D. (2001). The evolution of swidden fallow secondary forests in Asia. J. Trop. For. Sci. 13, 800–815.
Congdon, R. A. (2012). Basic methods for the collection, preparation and analysis of plant tissue, water and soil samples. Townsville: James Cook University.
Curtis, P. G., Slay, C. M., Harris, N. L., Tyukavina, A., and Hansen, M. C. (2018). Classifying drivers of global forest loss. Science 361, 1108–1111. doi:10.1126/science.aau3445
de Blécourt, M., Corre, M. D., Paudel, E., Harrison, R. D., Brumme, R., and Veldkamp, E. (2017). Spatial variability in soil organic carbon in a tropical montane landscape: Associations between soil organic carbon and land use, soil properties, vegetation, and topography vary across plot to landscape scales. Soil 3, 123–137. doi:10.5194/soil−3−123−2017
de Neergaard, A., Magid, J., and Mertz, O. (2008). Soil erosion from shifting cultivation and other smallholder land use in Sarawak, Malaysia. Agric. Ecosyst. Environ. 125, 182–190. doi:10.1016/j.agee.2007.12.013
FAO, (2006). Guidelines for soil description. 4th edition. Rome, Italy: Food and Agriculture Organization of the United Nations FAO.
Fernández−Romero, M. L., Lozano−García, B., and Parras−Alcántara, L. (2014). Topography and land use change effects on the soil organic carbon stock of forest soils in Mediterranean natural areas. Agric. Ecosyst. Environ. 195, 1–9. doi:10.1016/j.agee.2014.05.015
Filho, A. A. R., Adams, C., Manfredini, S., Aguilar, R., and Neves, W. A. (2015). Dynamics of soil chemical properties in shifting cultivation systems in the tropics: A meta−analysis. Soil Use Manag. 31, 474–482. doi:10.1111/sum.12224
Firn, J., Erskine, P. D., and Lamb, D. (2007). Woody species diversity influences productivity and soil nutrient availability in tropical plantations. Oecologia 154, 521–533. doi:10.1007/s00442−007−0850−8
Fox, J., Fujita, Y., Ngidang, D., Peluso, N., Potter, N., Sakuntaladewi, N., et al. (2009). Policies, political−economy, and swidden in Southeast Asia. Hum. Ecol. 37, 305–322. doi:10.1007/s10745−009−9240−7
Fox, J., Truong, D. M., Rambo, T. A., Tuyen, N. P., Cuc, L. T., and Leisz, S. (2000). Shifting cultivation: A new old paradigm for managing tropical forests. BioScience 50, 521–528. doi:10.1641/0006−3568(2000)050[0521:scanop]2.0.co;2
Funakawa, S., Makhrawie, M., and Pulunggono, H. B. (2009). Soil fertility status under shifting cultivation in East Kalimantan with special reference to mineralization patterns of labile organic matter. Plant Soil 319, 57–66. doi:10.1007/s11104−008−9849−0
Garcı́a−Oliva, F., Sanford, R. L. J., and Kelly, E. (1999). Effects of slash−and−burn management on soil aggregate organic C and N in a tropical deciduous forest. Geoderma 88, 1–12. doi:10.1016/s0016−7061(98)00063−9
Giardina, C. P., Sanford, R. L. J., Døckersmith, I. C., and Jaramillo, V. J. (2000). The effects of slash burning on ecosystem nutrients during the land preparation phase of shifting cultivation. Plant Soil 220, 247–260. doi:10.1023/a:1004741125636
Grueber, C. E., Nakagawa, S., Laws, R. J., and Jamieson, I. G. (2011). Multimodel inference in ecology and evolution: Challenges and solutions. J. Evol. Biol. 24, 699–711. doi:10.1111/j.1420−9101.2010.02210.x
Guo, L. B., and Gifford, R. M. (2002). Soil carbon stocks and land−use change: A meta analysis. Glob. Change Biol. 8, 345–360. doi:10.1046/j.1354−1013.2002.00486.x
Hattar, B. I., Taimeh, A. Y., and Ziadat, F. M. (2010). Variation in soil chemical properties along toposequences in an arid region of the Levant. Catena 83, 34–45. doi:10.1016/j.catena.2010.07.002
Hattori, D., Kenzo, T., Shirahama, T., Harada, Y., Kendawang, J. J., Ninomiya, I., et al. (2019). Degradation of soil nutrients and slow recovery of biomass following shifting cultivation in the heath forests of Sarawak, Malaysia. For. Ecol. Manag. 432, 467–477. doi:10.1016/j.foreco.2018.09.051
Heanes, D. L. (1984). Determination of total organic−C in soils by an improved chromic acid digestion and spectrophotometric procedure. Commun. Soil Sci. Plant Anal. 15, 1191–1213. doi:10.1080/00103628409367551
Heinimann, A., Mertz, O., Frolking, S., Christensen, A. E., Hurni, K., Sedano, F., et al. (2017). A global view of shifting cultivation: Recent, current, and future extent. PLoS ONE 12, e0184479. doi:10.1371/journal.pone.0184479
Hett, C., Castella, J., Heinimann, A., Messerli, P., and Pfund, J. (2012). A landscape mosaics approach for characterizing swidden systems from a REDD+ perspective. Appl. Geogr. 32, 608–618. doi:10.1016/j.apgeog.2011.07.011
Hughes, R. F., Kauffman, J. B., and Jaramillo, V. J. (1999). Biomass, carbon, and nutrient dynamics of secondary forests in a humid tropical region of Mexico. Ecology 80, 1892–1907. doi:10.2307/176667
Jones, I. L., DeWalt, S. J., Lopez, O. R., Bunnefeld, L., Pattison, Z., and Dent, D. H. (2019). Above−and belowground carbon stocks are decoupled in secondary tropical forests and are positively related to forest age and soil nutrients respectively. Sci. Total Environ. 697, 133987. doi:10.1016/j.scitotenv.2019.133987
Kolb, M. (2003). “Silvicultural analysis of “rainforestation farming” area on Leyte island, Philippines,”. PhD thesis (Germany: , University of Göttingen).
Kotto−Same, J., Woomer, P. L., Appolinaire, M., and Louis, Z. (1998). Carbon dynamics in slash−and−burn agriculture and land use alternatives of the humid forest zone in Cameroon. Agric. Ecosyst. Environ. 65, 245–256. doi:10.1016/s0167−8809(97)00060−1
Krishan, G., Srivastav, S. K., Kumar, S., Saha, S. K., and Dadhwal, V. K. (2009). Quantifying the underestimation of soil organic carbon by the Walkley and Black technique − examples from Himalayan and Central Indian soils. Curr. Sci. 96, 1133–1136.
Lal, R. (1997). Degradation and resilience of soils. Phil. Trans. R. Soc. Lond. B 352, 997–1010. doi:10.1098/rstb.1997.0078
Lange, M., Eisenhauer, N., Sierra, C. A., Bessler, H., Engels, C., Griffiths, R. I., et al. (2015). Plant diversity increases soil microbial activity and soil carbon storage. Nat. Commun. 6, 6707. doi:10.1038/ncomms7707
Lawrence, D., Radel, C., Tully, K., Scmook, B., and Schneider, L. (2010). Untangling a decline in tropical forest resilience: Constraints on the sustainability of shifting cultivation across the globe. Biotropica 42, 21–30. doi:10.1111/j.1744−7429.2009.00599.x
Lawrence, D., and Schlesinger, W. H. (2001). Changes in soil phosphorus during 200 years of shifting cultivation in Indonesia. Ecology 82, 2769–2780. doi:10.1890/0012−9658(2001)082[2769:cispdy]2.0.co;2
Lawrence, D., Suma, V., and Mogea, J. P. (2005). Change is species composition with repeated shifting cultivation: Limited role of soil nutrients. Ecol. Appl. 15, 1952–1967. doi:10.1890/04−0841
Mertz, O., Leisz, S. J., Heinimann, A., Rerkasem, K., Thiha, Dressler W., Pham, V. C., et al. (2009b). Who counts? Demography of swidden cultivators in Southeast Asia. Hum. Ecol. 37, 281–289. doi:10.1007/s10745−009−9249−y
Mertz, O., Padoch, C., Fox, J., Cramb, R. A., Leisz, S. J., Nguyen, T. L., et al. (2009a). Swidden change in Southeast Asia: Understanding causes and consequences. Hum. Ecol. 37, 259–264. doi:10.1007/s10745−009−9245−2
Mertz, O., Wadley, R. L., Nielsen, U., Bruun, T. B., Colfer, C. J. P., de Neergaard, A., et al. (2008). A fresh look at shifting cultivation: Fallow length an uncertain indicator of productivity. Agric. Syst. 96, 75–84. doi:10.1016/j.agsy.2007.06.002
Mukul, S. A., Herbohn, J., Firn, J., and Gregorio, N. (2022). “Carbon and biodiversity outcomes under divergent management scenarios − lessons from upland Philippines shifting cultivation landscapes,” in Farmer innovations and best practices by shifting cultivators in Asia-Pacific. Editor M. Cairns, 408–420.
Mukul, S. A., Halim, M. A., and Herbohn, J. (2021). “Forest carbon stock and fluxes: Distribution, biogeochemical cycles, and measurement techniques,” in Life on land, encyclopedia of the UN sustainable development goals. Editors W. Leal Filho, A. Azul, L. Brandli, A. Lange Salvia, and T. Wall (Switzerland: Springer), 361–376.
Mukul, S. A., Herbohn, J., and Firn, J. (2020). Rapid recovery of tropical forest diversity and structure after shifting cultivation in the Philippines upland. Ecol. Evol. 10, 7189–7211. doi:10.1002/ece3.6419
Mukul, S. A., Herbohn, J., and Firn, J. (2016a). Co−Benefits of biodiversity and carbon sequestration from regenerating secondary forests in the Philippine uplands: Implications for forest landscape restoration. Biotropica 48, 882–889. doi:10.1111/btp.12389
Mukul, S. A., Herbohn, J., and Firn, J. (2016b). Tropical secondary forests regenerating after shifting cultivation in the Philippines uplands are important carbon sinks. Sci. Rep. 6, 22483. doi:10.1038/srep22483
Mukul, S. A., and Herbohn, J. (2016). The impacts of shifting cultivation on secondary forests dynamics in tropics: A synthesis of the key findings and spatio temporal distribution of research. Environ. Sci. Policy 55, 167–177. doi:10.1016/j.envsci.2015.10.005
Mukul, S. A. (2016). “Shifting cultivation in the upland secondary forests of the Philippines: Biodiversity and carbon stock assessment, and ecosystem services trade−offs in land−use decisions,”. PhD Thesis (Australia: The University of Queensland).
Murty, D., Kirschbaum, M. U. F., Mcmurtrie, R. E., and Mcgilvray, H. (2002). Does conversion of forest to agricultural land change soil carbon and nitrogen? A review of the literature. Glob. Change Biol. 8, 105–123. doi:10.1046/j.1354−1013.2001.00459.x
Navarrete, I. A., Tsutsuki, K., and Asio, V. B. (2013). Characteristics and fertility constraints of degraded soils in Leyte, Philippines. Archives Agron. Soil Sci. 59, 625–639. doi:10.1080/03650340.2012.663908
Neill, C., Melillo, J. M., Steudler, P. A., Cerri, C. C., Moraes, J. F. L. D., Piccolo, M. C., et al. (1997). Soil carbon and nitrogen stocks following forest clearing for pasture in the southwestern Brazilian Amazon. Ecol. Appl. 7, 1216–1225. doi:10.1890/1051−0761(1997)007[1216:scansf]2.0.co;2
Nottingham, A. T., Whitaker, J., Turner, B. L., Salinas, N., Zimmermann, M., Malhi, Y., et al. (2015). Climate warming and soil carbon in tropical forests: Insights from an elevation gradient in the Peruvian Andes. BioScience 65, 906–921. doi:10.1093/biosci/biv109
Olofson, H. (1980). Swidden and kaingin among the southern Tagalog: A problem in philippine upland ethno−agriculture. Philipp. Q. Cult. Soc. 8, 168–180.
Ota, L., Chazdon, R. L., Herbohn, J., Gregorio, N., Mukul, S. A., and Wilson, S. J. (2020). Achieving quality forest and landscape restoration in the tropics. Forests 11, 820. doi:10.3390/f11080820
Paoli, G. D., Curran, L. M., and Slik, J. W. F. (2008). Soil nutrients affect spatial patterns of aboveground biomass and emergent tree density in southwestern Borneo. Oecologia 155, 287–299. doi:10.1007/s00442−007−0906−9
Parras−Alcántara, L., Martín−Carrillo, M., and Lozano−García, B. (2013). Impacts of land use change in soil carbon and nitrogen in a Mediterranean agricultural area (Southern Spain). Solid earth. 4, 167–177. doi:10.5194/se−4−167−2013
Paudel, E., Dossa, G. G. O., and XuHarrison, J. R. D. (2015). Litterfall and nutrient return along a disturbance gradient in a tropical montane forest. For. Ecol. Manag. 353, 97–106. doi:10.1016/j.foreco.2015.05.028
Pinheiro, J., Bates, D., Roy, S. D., and Sarkar, D. (2011). Package ‘nlme. Available at: http://cran.r−project.org/web/packages/nlme/nlme.pdf.
R Development Core Team, (2019). R: A language and environment for statistical computing. Vienna: R Foundation for Statistical Computing.
Raharimalala, O., Buttler, A., Ramohavelo, C. D., Razanaka, S., Sorge, J. P., and Gobat, J. M. (2010). Soil−vegetation patterns in secondary slash and burn successions in Central Menabe, Madagascar. Agric. Ecosyst. Environ. 139, 150–158. doi:10.1016/j.agee.2010.07.013
Raich, J. W., Clark, D. A., Schwendenmann, L., and Wood, T. E. (2014). Aboveground tree growth varies with belowground carbon allocation in a tropical rainforest environment. PLoS ONE 9, e100275. doi:10.1371/journal.pone.0100275
Richards, A. E., Dalal, R. C., and Schmidt, S. (2007). Soil carbon turnover and sequestration in native subtropical tree plantations. Soil Biol. Biochem. 39, 2078–2090. doi:10.1016/j.soilbio.2007.03.012
Rodenburg, J., Stein, A., Noordwijk, M., and Ketterings, Q. M. (2003). Spatial variability of soil pH and phosphorus in relation to soil run−off following slash−and−burn land clearing in Sumatra, Indonesia. Soil Tillage Res. 71, 1–14. doi:10.1016/s0167−1987(02)00141−1
Romanyá, J., Casals, P., and Vallejo, V. R. (2001). Short term effects of fire on soil nitrogen availability in Mediterranean grasslands and shrublands growing in old fields. For. Ecol. Manag. 147, 39–53. doi:10.1016/s0378−1127(00)00433−3
Sang, P. M., Lamb, D., Bonner, M., and Schmidt, S. (2013). Carbon sequestration and soil fertility of tropical tree plantations and secondary forest established on degraded land. Plant Soil 362, 187–200. doi:10.1007/s11104−012−1281−9
Sarai, S. S., De Jong, B. H., Esperanza, H. L., Jorge, M. V., Danilo, M. R., and Aryal, D. R. (2022). Fine root biomass stocks but not the production and turnover rates vary with the age of tropical successional forests in Southern Mexico. Rhizosphere 21, 100474. doi:10.1016/j.rhisph.2022.100474
Sayer, E. J., Heard, M. S., Grant, H. K., Marthews, T. R., and Tanner, E. V. J. (2011). Soil carbon release enhanced by increased tropical forest litterfall. Nat. Clim. Chang. 1, 304–307. doi:10.1038/nclimate1190
Smith, P., House, J. I., Bustamante, M., Sobocká, J., Harper, R., Pan, G., et al. (2015). Global change pressures on soils from land use and management. Glob. Change Biol. 22, 1008–1028. doi:10.1111/gcb.13068
Suarez, R. K., and Sajise, P. E. (2010). Deforestation, swidden agriculture and Philippine biodiversity. Philipp. Sci. Lett. 3, 91–99.
Tanaka, S., Andoa, T., Funakawa, S., Sukhrun, C., Kaewkhongkha, T., and Sakurai, K. (2001). Effect of burning on soil organic matter content and N mineralization under shifting cultivation system of Karen people in Northern Thailand. Soil Sci. Plant Nutr. 47, 547–558. doi:10.1080/00380768.2001.10408418
Taylor, B. N., Chazdon, R. L., Bachelot, B., and Menge, D. N. L. (2017). Nitrogen−fixing trees inhibit growth of regenerating Costa Rican rainforests. Proc. Natl. Acad. Sci. U. S. A. 114, 8817–8822. doi:10.1073/pnas.1707094114
Teegalapalli, K., Mailappa, A. S., Lyngdoh, N., and Lawrence, D. (2018). Recovery of soil macronutrients following shifting cultivation and ethnopedology of the Adi community in the Eastern Himalaya. Soil Use Manag. 34, 249–257. doi:10.1111/sum.12420
Teixeira, H. M., Cardoso, I. M., Bianchi, F. J. J. A., Silva, A. C., Jamme, D., and Peña−Claros, M. (2020). Linking vegetation and soil functions during secondary forest succession in the Atlantic forest. For. Ecol. Manag. 457, 117696. doi:10.1016/j.foreco.2019.117696
Temjen, W., Singh, M. R., and Ajungla, T. (2022). Effect of shifting cultivation and fallow on soil quality index in Mokokchung district, Nagaland, India. Ecol. Process. 11, 42. doi:10.1186/s13717−022−00386−w
Terefe, B., and Kim, D. G. (2020). Shifting cultivation maintains but its conversion to mono−cropping decreases soil carbon and nitrogen stocks compared to natural forest in Western Ethiopia. Plant Soil 453, 105–117. doi:10.1007/s11104−019−03942−0
Thomaz, E. L. (2017). High fire temperature changes soil aggregate stability in slash−and−burn agricultural systems. Sci. Agric. 74, 157–162. doi:10.1590/1678−992x−2015−0495
van Noordwijk, M., Cerri, C., Woomer, P. L., Nugroho, K., and Bernoux, M. (1997). Soil carbon dynamics in the humid tropical forest zone. Geoderma 79, 187–225. doi:10.1016/s0016−7061(97)00042−6
van Straaten, O., Corre, M. D., Wolf, K., Tchienkoua, M., Cuellar, E., Matthews, R. B., et al. (2015). Conversion of lowland tropical forests to tree cash crop plantations loses up to one−half of stored soil organic carbon. Proc. Natl. Acad. Sci. U. S. A. 112, 9956–9960. doi:10.1073/pnas.1504628112
van Vliet, N., Mertz, O., Heinimann, A., Langanke, T., Pascual, U., Schmook, B., et al. (2012). Trends, drivers and impacts of changes in swidden cultivation in tropical forest−agriculture frontiers: A global assessment. Glob. Environ. Change 22, 418–429. doi:10.1016/j.gloenvcha.2011.10.009
Yang, Y., Guo, J. F., Chen, G. S., He, Z. M., and Xie, J. S. (2003). Effect of slash burning on nutrient removal and soil fertility in Chinese fir and evergreen broadleaved forests of mid−subtropical China. Pedosphere 13, 87–96.
Zhang, J., Bruijnzeel, L. A., Quiñones, C. M., Tripoli, R., Asio, V. B., and van Meerveld, H. J. (2019). Soil physical characteristics of a degraded tropical grassland and a ‘reforest’: Implications for runoff generation. Geoderma 333, 163–177. doi:10.1016/j.geoderma.2018.07.022
Ziegler, A. D., Fox, J. M., Webb, E. L., Padoch, C., Leisz, S. J., Cramb, R. A., et al. (2011). Recognizing contemporary roles of swidden agriculture in transforming landscapes of Southeast Asia. Conserv. Biol. 25, 846–848. doi:10.1111/j.1523−1739.2011.01664.x
Keywords: soil macronutrients, succession, forest restoration, Philippines, Southeast Asia
Citation: Mukul SA, Herbohn J, Ferraren A and Congdon R (2022) Limited role of shifting cultivation in soil carbon and nutrients recovery in regenerating tropical secondary forests. Front. Environ. Sci. 10:1076506. doi: 10.3389/fenvs.2022.1076506
Received: 21 October 2022; Accepted: 07 November 2022;
Published: 01 December 2022.
Edited by:
Junhong Bai, Beijing Normal University, ChinaReviewed by:
Gaurav Mishra, Indian Council of Forestry Research and Education (ICFRE), IndiaDeb Raj Aryal, National Council of Science and Technology (CONACYT), Mexico
Copyright © 2022 Mukul, Herbohn, Ferraren and Congdon. This is an open-access article distributed under the terms of the Creative Commons Attribution License (CC BY). The use, distribution or reproduction in other forums is permitted, provided the original author(s) and the copyright owner(s) are credited and that the original publication in this journal is cited, in accordance with accepted academic practice. No use, distribution or reproduction is permitted which does not comply with these terms.
*Correspondence: Sharif A. Mukul, c211a3VsQHVzYy5lZHUuYXU=