- 1Department of Oceanography and Coastal Sciences, Louisiana State University, Baton Rouge, LA, United States
- 2Environment Department, Micro, Small and Medium Enterprises Development Agency (MSMEDA), Giza, Egypt
- 3Department of Planning and Natural Resources, Division of Coastal Zone Management, St. Thomas, VI, United States
Microplastics are ubiquitous in Earth’s ecosystems and many efforts have begun to understand their distributions. Large rivers, like the Mississippi River, provide a unique system in which to look at large-scale patterns of microplastic distribution. In this study, we sampled four species of widely-distributed fishes from five sites along the mainstem Mississippi River, from Minnesota to Louisiana, United States. Microplastics were found in all fish species and at all sites; however, microplastics increased in occurrence in the Lower Mississippi River. Fragments were the most common morphologies and polypropylene was the most common polymer detected. We also examined the hypothesis that microplastic loads in fishes increased downstream, but found support for this hypothesis only when examining Largemouth Bass; Flathead Catfish, Shortnose Gar, and Bluegill were all found to have similar microplastic loads along the mainstem Mississippi River. It is clear that microplastics are heterogeneously distributed throughout ecosystems, and further understanding of microplastic distributional patterns and varying species burdens are needed to fully understand threats that microplastics present.
1 Introduction
Since the mid-20th century, an estimated 8,300 million metric tons of plastic have been produced (Geyer et al., 2017), which is consistent with the prevalence of plastics in aquatic ecosystems (Cole et al., 2011; Rochman et al., 2013). Plastic debris in marine ecosystems was first discovered decades ago (Carpenter and Smith, 1972) and has since been dominated by research focused on marine environments (Cole et al., 2011). However, in more recent years, there has been a growing interest in understanding the dynamics of plastics in freshwater environments and the subsequent effects on the fish in freshwater habitats (Eerkes-Medrano et al., 2015).
Ecosystems and their inhabitants may be particularly vulnerable to microplastics, which are typically defined as plastics that are less than 5 mm in size in any dimension (Duis and Coors, 2016; van Wezel et al., 2016). Microplastics can further be divided into two categories: primary and secondary microplastics. Primary microplastics are raw resin pellets (nurdles) used in the plastic manufacturing process and are purposely manufactured to be small in size (e.g., Fendall and Sewell, 2009). Primary microplastics are also found in facial cleansers and used as bead-blasting media (Fendall and Sewell, 2009; Thompson et al., 2009). Secondary microplastics originate from larger plastics breaking apart over time. These chemical and physical breakdowns are results of photo-oxidation, mechanical stress, and wave-actions (Barnes et al., 2009; Cole et al., 2011; Van Wezel et al., 2016).
Microplastics can span the size ranges of phytoplankton and zooplankton, which form the base of aquatic food webs. When small plastics enter aquatic environments, they become bioavailable to the organisms that ingest them, along with any absorbed contaminants (Jabeen et al., 2017). Once ingested, microplastics can lead to both physical and chemical hazards including clogged digestive tracts, false satiation (Hoss and Settle, 1990; Derraik, 2002), lower feeding activity (Anderson et al., 2016), reduced reproductive fitness, and increased immune response (Von Moos et al., 2012).
Microplastics have been reported in oceans and coastal environments around the world, and as such there are likely different ways that microplastics enter and pass-through aquatic environments (Cole et al., 2011) and biota. However, the occurrence of microplastics in freshwater fish species has varied greatly among studies. A study by Park et al. (2020) investigated microplastic pollution in six species of fish from the Han River and its tributaries in South Korea and found all species of fish contained microplastics (between 4 and 48 particles). A study of Gizzard Shad (Dorosoma cepedianum) and Largemouth Bass (Micropterus salmoides) within two hypereutrophic drinking water reservoirs in Illinois reported 100% of the sampled fish to be contaminated with microplastics (Hurt et al., 2020). A study including Bluegill (Lepomis macrochirus) and Longear Sunfish (Lepomis megalotis) reported 45% of the sampled fish containing microplastics in their stomachs (Peters and Bratton, 2016). The percent microplastic occurrence also appears to be increasing compared to earlier research (Toner and Midway, 2021); for example, only 12% of Gudgeon (Gobio gobio) in urbanized streams contained microplastics (Sanchez et al., 2014) and only 8% of 44 different species of freshwater fishes in Texas had microplastic contamination (Phillips and Bonner, 2015).
The Mississippi River is the largest river in North America and connects 32 states while discharging about 41% of the United States freshwater into the Gulf of Mexico (Goolsby and Battaglin, 2001). A recent large-scale study in the Mississippi River quantified microplastics in the water column from St. Louis, Missouri to New Orleans, Louisiana (Scircle et al., 2020) and found that microplastic concentrations generally increased downriver. The Mississippi River is also considered to be a large source of microplastics; Di Mauro et al. (2017) reported high microplastic concentrations in the northern Gulf of Mexico, which are thought to be sourced from the Mississippi River. In general, it has been reported that up to 80% of plastic pollution in marine environments is estimated to come from terrestrial sources (Cole et al., 2011). The large aquatic and terrestrial expanse of the Mississippi River not only suggests that it may hold large numbers of microplastics, but also the watershed’s biodiversity may be interacting with microplastics.
Despite the Mississippi River’s size and influence, little research exists on microplastic pollution prevalence in, or effects on, fishes in the river basin. Although it might be expected that most fish species ingest microplastics, substantial variability in microplastic consumption may exist due to differences in feeding behaviors, habitat use, and other species-specific differences. One way to understand fish and microplastics across large spatial scales is to focus on a set of common and widely distributed species. In theory, the same species should have the same (or similar) ontogeny, behavior, and feeding habits across space, and as such, differences in microplastic concentrations in the same species across a large area should be reflective of the microplastics in that location. Although the Mississippi River is home to an estimated 288 fish species (Ross and Brenneman, 2001), Bluegill (L. macrochirus), Largemouth Bass, Shortnose Gar (Lepisosteus platostomus), and Flathead Catfish (Pylodictis olivaris), are widely distributed throughout the Mississippi River and also routinely sampled by fishery monitoring programs, making them good candidate species for large-scale studies.
This study was designed to understand microplastics pollution in the Mississippi River by quantifying microplastics in common Mississippi River fishes captured at different locations. Our objective was to capture the same fish species across a spatial gradient of the upper mainstem (upstream) to lower mainstem (downstream) Mississippi River. By comparing both species and location, we are attempting to understand microplastics both in the context of fish species and the Mississippi River system.
2 Materials and methods
2.1 Study area and fish sampling
Fish were collected by existing fish sampling programs operated by United States resource agencies: Louisiana Department Wildlife and Fisheries (LDWF), Missouri Department of Conservation, Wisconsin Department of Natural Resources, and Minnesota Department of Natural Resources. Fish were sampled in the late summer and fall of 2018 and 2019 at five different sites on the mainstem of the Mississippi River: Minneapolis MN, La Crosse WI, Cape Girardeau MO, Caruthersville MO, and St. Francisville LA (Figure 1; Table 1). All fish were collected using electrofishing, except for Flathead Catfish in St. Francisville, LA and Shortnose Gar in Caruthersville, MO (Table 1), which were caught using two sizes of hoop nets (large nets with a diameter of 1.1–1.2 m and small nets with a diameter of 0.5–0.6) and 91.44 m long gillnets (full sampling and gear protocols can be found in Gutreuter et al., 1995). Due to historically high-water levels during the 2019 sampling season, samples were not collected by LDWF. Fish species included in the study were selected based on their ubiquity throughout the mainstem Mississippi River, but also because they represented different foraging guilds (including both different prey types and different habitats or parts of the water column in which they forage) that could lead to different microplastic exposures. Flathead catfish are benthic piscivores, while Largemouth Bass and Shortnose Gar are ambush predators. Bluegill are zooplanktivores and insectivores. After sampling, all frozen fishes were transferred to Louisiana State University (Baton Rouge, LA) either by direct pickup from agencies, or through shipment of freshly caught frozen samples with dry ice. Upon collection, fish were separated into labeled bags and immediately placed in ice-filled coolers. Once back at the lab, fish remained in the labeled bags and were immediately placed in a chest freezer until they were ready to be processed.
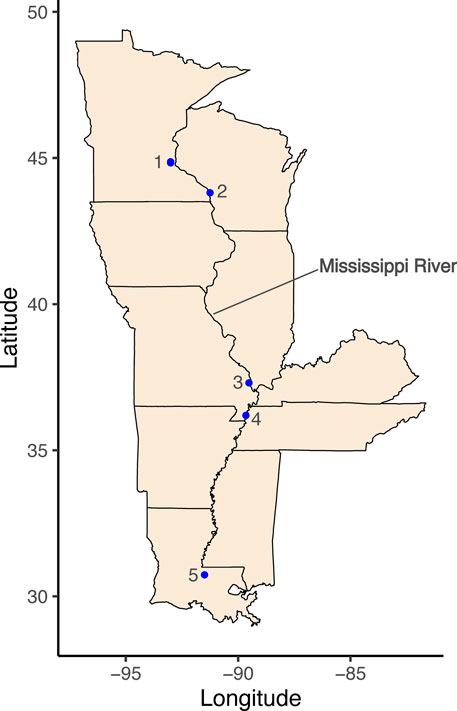
FIGURE 1. Map of the mainstem Mississippi River and the surrounding U.S. states. Fish sampling locations are represented by blue dots and numbers that correspond to descriptions in Table 1. The inset map in the bottom right shows the 48 contiguous U.S. states and the mainstem Mississippi River in blue.
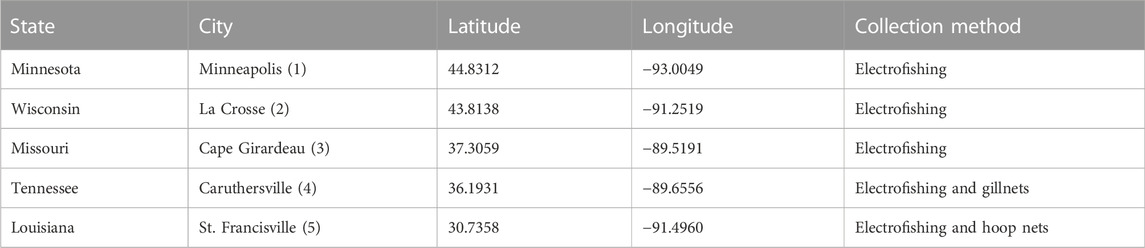
TABLE 1. Sampling locations and methods used to collect fish samples. The number in parentheses behind the city name corresponds to the location numbers on the map in Figure 1. Sampling dates varied by location, but can be found in Supplementary Data Sheet.
2.2 Sample processing
Fish were thawed for 24 h prior to dissection. Once thawed, each individual fish was given a sample ID, measured for total length (mm), and weighed (g). Individual fish were dissected by removing their stomach, which were weighed (g) and then placed in separate clean glass jars and stored in a freezer. Stomachs were liquified using a KOH digestion process by Foekema et al. (2013). 10 g of KOH flakes were measured out in a Griffin beaker and slowly poured into a flask with 100 ml of distilled and filtered water. The solution was mixed thoroughly using a magnetic stirring rod that was cleaned with filtered water. The KOH solution was then filtered before use. Sample jars were filled with a 10% potassium hydroxide (KOH) solution, sealed tightly and placed in a warm water bath set to 60°C for 24 h or until the stomach was completely liquified (e.g., Dehaut et al., 2016; Zhang et al., 2020). After the initial digestion, contents of the sample were filtered through one or more (depending on sample density) 20 μm nylon net filter paper using a vacuum filtration system. Sample jars were rinsed using filter deionized (DI) water into the filtration system. The filter paper containing the sample was placed directly into a petri dish, sealed tightly, and labeled. The petri dishes were then placed in a drying oven set at 60°C for 24 h.
2.3 Polymer analysis
Individual putative plastic particles found within each fish sample were further analyzed using Fourier transform infrared (FTIR) spectroscopy, a method that uses infrared radiation to characterize microplastics at the molecular level (Frias et al., 2014). Each filter paper was first examined visually under a dissecting microscope. If a putative plastic was visually identified, it was then removed using stainless steel forceps and processed through the FTIR spectrometer. Depending on the size of the plastic either the ThermoScientific iS5 spectrometer (equipped with an attenuated total reflection (ATR) accessory) or the ThermoScientific Nicolet iN10MX was used. In the latter, particles were placed on a gold-plated slide. To determine polymer composition of plastic particles, spectra taken from each particle were compared to a library of polymer spectra from the OMNIC (ThermoFisher) software library. Particles were classified as plastic if their spectrum had over a 70% match with a polymer spectrum from the pre-existing OMNIC spectral library; however, samples that fell between 60% and 70% were visually examined and compared to the previously identified polymers (e.g., Lusher et al., 2013). Total numbers of microplastics and their polymeric composition were summarized for each fish.
To prevent contamination, nitrile gloves were used when processing samples and ultimately none of the microplastics in the samples had a polymer match with the nitrile gloves. All equipment and work surfaces were washed with distilled water prior to use, and stomachs were placed immediately in clean glass jars once removed. After filtering the samples, papers were placed in new Petri dishes that were closed immediately to prevent any aerial contamination. Control samples, processed in the same manner as the fish samples but lacking fish tissue, were processed regularly to test the possible contamination from the liquid potassium hydroxide, air, and equipment. No microplastics were identified in the control samples.
2.4 Data analysis
Microplastic counts within fish were often low (e.g., zero), suggesting a Zero Inflated Poisson (ZIP) regression be used to test the relationship between the response of microplastics ingestion and the predictors of species and location. The use of a ZIP model allowed for the accommodation of zero-inflation, while still performing the Poisson regression. A ZIP model is composed of two linked models. The first model is a logistic regression that models 0s and 1s using a Bernoulli distribution, which effectively estimates whether an observation is a value that should be included in the Poisson model for counts, or whether it is a zero that should be further excluded. The model is written as:
where ωi is the logistic model estimate for fish i and ψ is the probability. The second model is a modified Poisson that models the counts,
Where C is the observed counts and λi is the Poisson parameter for counts. Note that the Poisson equation is very similar to a typical Poisson estimation, except the ωi parameter is modifying what observations are included in the counts, and thereby reducing the number of zeros going into the Poisson regression. The above zero-inflated model structure was then used for two models that represented different questions. Below, for simplicity, we show the linear component of the regression:
Where
3 Results
A total of n = 229 fish were sampled from the mainstem of the Mississippi River with 28 (12%) of those fish containing one or more microplastic particle. Microplastics were found in all four species of fish: 12 out of 89 Bluegills (13%), 3 out of 31 Flathead Catfish (10%), 7 out of 55 Largemouth Bass (13%), and 6 out of 54 Shortnose Gar (11%) (Table 2). Pooling species, the percentage of fish with microplastics in their stomachs was higher in downstream locations compared to upstream: only 2 out of 44 fish samples in Minnesota reported microplastics compared to 8 out of 43 fish samples in Louisiana (Figure 2).
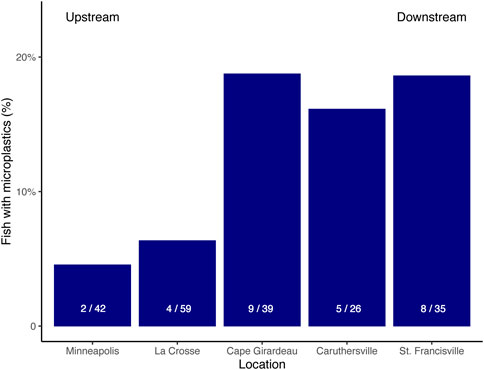
FIGURE 2. Percentage of fish samples by location that contained microplastics. Fish were considered as either containing or not containing microplastics; i.e., abundance of microplastics was not accounted for in this representation. Sites are ordered from upstream to downstream and the white fraction with each bar represents the number of fish with microplastics to the total number of fish sampled.
Microplastics detected had a mean size of 1.4 mm and ranged from 0.1 to 9.0 mm. The morphological types of plastic found within the fish were fibers, fragments, and foams (Figure 3). Bluegill had the highest overall count of fibers with 9, followed by Largemouth Bass with 6, and Shortnose Gar with 2. No fibers were found within Flathead Catfish. Microplastic fragments were found in all four species. Largemouth Bass had the highest overall count of fragments at 7, followed by Bluegill and Shortnose Gar with 5, and Flathead Catfish with an overall count of 3. Foam particles were only found in Bluegill and Largemouth Bass.
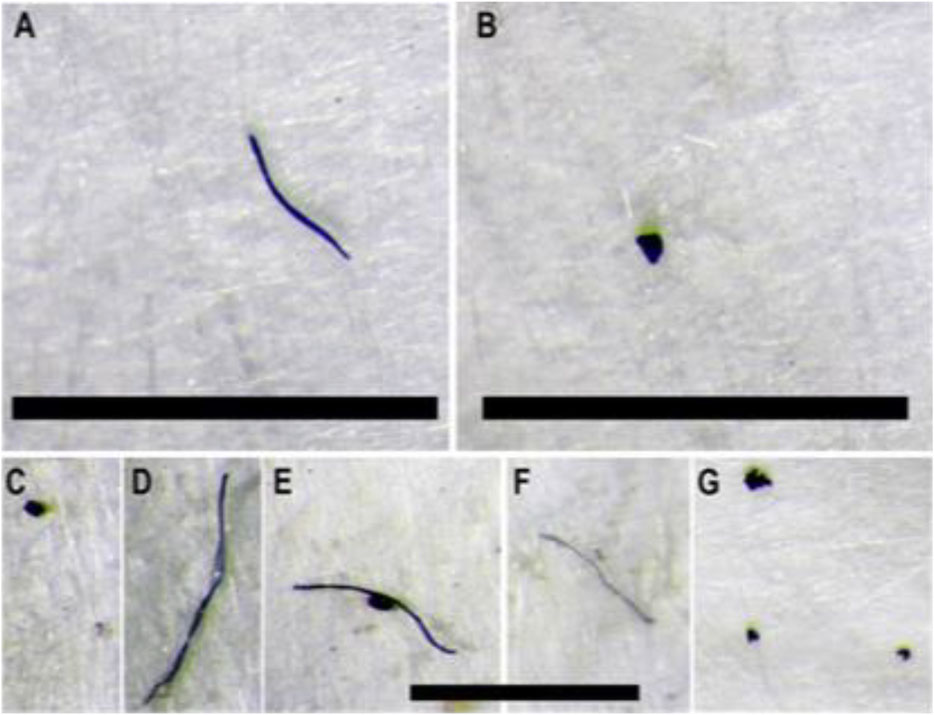
FIGURE 3. Microplastics found in fish stomachs from the Mississippi River. Fiber (A) and fragment (B) found within Shortnose Gar. Fibers and fragments (C–G) are fibers and fragments found within in Largemouth Bass. All black bars represent 1 mm scale.
All the major categories of plastic polymers from The American Chemistry Council resin identification coding system were found in a least one or more of the four fish species being studied (Table 3). Polyethylene terephthalate (PET) and polyethylene (PE, including both low- and high-density) were present in Bluegill and Flathead Catfish, respectively. Polypropylene was the only polymer found within all four species. Polyethylene was present in Bluegill, Flathead Catfish, and Largemouth Bass but absent in Shortnose Gar. Bluegill, Largemouth Bass, and Shortnose Gar all had plastics present from the polymer category labeled other. This category is a catch-all category for all other types of plastic polymers outside of the six major polymers.
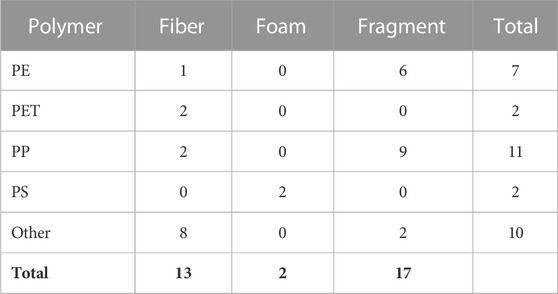
TABLE 3. Distribution of microplastic polymers by morphologies. PE, Polyethylene; PET, Polyethylene terephthalate; PP, Polypropylene; PS, Polystyrene; Other includes a range of polymer types that can be referenced in Supplementary Data Sheet S2.
A zero-inflated Poisson model was used to examine the relationship between the different locations and the microplastics counts ingested by fishes. The model reported a significant effect of zero-inflation model coefficients (i.e., the binomial model component): Psi (ψ) estimate = 0.31, p-value < 0.001. This statistically significant zero-inflation parameter estimate supports the choice of a zero-inflation model. A significant effect of the river kilometer was found for only one species, Largemouth Bass (
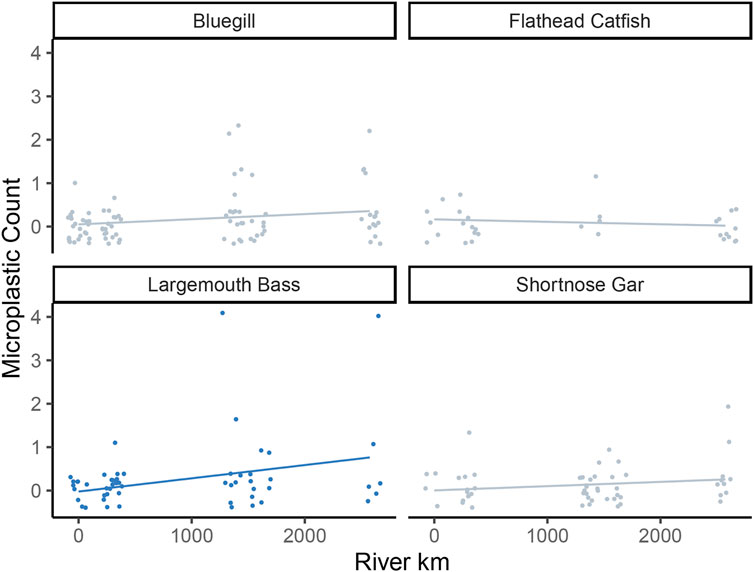
FIGURE 4. Scatterplots by species of microplastic counts per individual fish regressed against river location (km; with 0 km representing the farthest upstream location and points jittered to prevent overlap). Regression lines represent the zero-inflated Poisson model fit, with gray intervals representing model uncertainty. Gray points and lines indicate species with non-significant model fits, while blue points and lines represent significant model fits.
4 Discussion
This study is the first macroscale study in the Mississippi River looking at microplastics in freshwater fishes. The results are largely in agreement with other studies supporting the idea that freshwater fishes ingest microplastics (Silva-Cavalcanti et al., 2017; Collard et al., 2018). Plastics were found in the stomachs of all four fish species we investigated, with an average of 12% of the sampled fish containing microplastics. The number of fishes that ingested microplastics at downstream locations was generally higher than the number of fish that ingested microplastics upstream; however, only Largemouth Bass showed a significant effect of downstream location on increasing microplastic counts.
Microplastics are commonly reported in Largemouth Bass. For example, Hurt et al. (2020) studied Largemouth Bass in Lake Bloomington and Evergreen Lake in Illinois, and microplastics were present in 100% of the specimens (n = 24) with high concentrations of microplastics of approximately 25 microplastic particles per fish. Although Largemouth Bass were a focal species of our study, only 13% of Largemouth Bass we sampled contained microplastics in their stomachs. Phillips and Bonner (2015) found microplastics in Bluegill stomachs in Texas, but they only examined 12 specimens. However, Peters and Bratton (2016) also examined microplastics in Bluegill in the Brazos River Basin in Texas, and 144 out of 318 Bluegill had microplastics in their stomachs. In comparison, our study reported low numbers of Bluegill with microplastics in their stomach, 12 out of 89 Bluegills (13%). No prior documentation of microplastics in Shortnose Gar and Flathead Catfish exists, perhaps due to the overall lack of commercial and recreational importance of both species. However, our study is the first to document microplastics in Shortnose Gar and Flathead Catfish, which were found in 11% and 10% of each respective species.
We expected to find higher proportions of fish with microplastics in their stomachs. Many studies of freshwater fish and microplastics report ranges of 20%–70% of fishes containing microplastics (e.g., Peters and Bratton, 2016; McGoran et al., 2017; Hurt et al., 2020), whereas we found only 10%–13% of fish (including four species) contained microplastics. It is worth noting that while we are confident that our rates of microplastic occurrence are lower, when comparing among studies there are notable differences in species, laboratory methods, and polymer analysis that could account for some of the differences in results. Despite the relatively low occurrence rate of microplastics we observed, our findings are somewhat consistent with results from another study of a riverine fish (Sanchez et al., 2014), as well as other studies done on estuarine species (Dantas et al., 2012; Ramos et al., 2012) and some marine fishes (Possatto et al., 2011; Romeo et al., 2015). Ultimately, it appears microplastics in fish may be highly variable and not only influenced by species and location, but by season, local conditions, and a range of other possible factors (Collard et al., 2019).
It is also possible that methodological difference contributed to some of the differences we observed between our study and others. For example, Hurt et al. (2020) digested the whole gut and gills together using 10 ml of 1 mol L−1 KOH and 5 ml of sodium dodecyl sulfate [0.5% w/v (ca. 5 g L−1)] per gram of fish tissue 10% KOH at 50°C, and modified potassium hydroxide and hydrogen peroxide digestion. Phillips and Bonner (2015) did not report microplastic occurrence, nor digest the gastrointestinal tract prior to examination. Although methodological techniques for microplastics have advanced in the past decade, there still remain differences that may or may not contribute to individual study results, and such differences should be taken into consideration when comparing research.
Jabeen et al. (2017) investigated 27 fish species for microplastics and found that demersal fish species ingested more microplastics than pelagic species; however, Rummel et al. (2016) found the opposite—pelagic species ingest more microplastics than demersal species. Our findings of consistent occurrence of microplastics across different species (with different habitat and feeding modalities) is more in accordance with Lusher et al. (2013), who did not find any significant difference between different species and habitats. For the most part, the sizes of the fishes in our study were large enough that we do not expect them to be directly consuming microplastics, such as through misidentification with other small food items. Rather, we expect the consumption of microplastics in our study was a function of the microplastics in prey items or accidental ingestion from water taken in during feeding (e.g., Andrade et al., 2019). We did not find any clear species impact on microplastics loads, but we did see increases in microplastics with downstream river location (particularly increasing around Cape Girardeau, MO, which is a short distance downstream from St. Louis, MO). This downstream effect could be reflecting the fact that there are more microplastics in downstream locations—although not necessarily higher concentrations. Another lurking factor could be that as turbidity increases downstream, selectivity of prey items may decrease (Benfield and Minello, 1996; Abrahams and Kattenfeld, 1997) effectively increasing accidental ingestion of microplastics. A final consideration is trophic transfer of microplastics, which has been documented in aquatic food webs (Carbery et al., 2018). Predatory species like Largemouth Bass, Flathead Catfish, and Shortnose Gar could be expected to have higher concentrations of microplastics based on indirect consumption of microplastics in prey items. Also, Bluegill feed on zooplantkon, which are also reported to ingest microplastics (Cole et al., 2013). However, it is thought that the retention and excretion time of microplastics in fish guts varies widely, which makes microplastic consumption via trophic pathways uncertain (Okamoto et al., 2022).
Within fish stomachs we found polymers of PET, PE, PP, and PS. Although we did not have specific hypotheses about which polymers to expect, similar polymers were reported in stomachs of fish sampled from three major tributaries of Lake Michigan (McNeish et al., 2018), the Amazon River estuary (Pegado et al., 2018), and several marine fish studies (Lusher et al., 2013; Neves et al., 2015; Steer et al., 2017). The exact sources of the polymers we observed are unknown as samples were taken from multiple locations throughout the river—in addition to the fact that many polymers have multiple uses (i.e., cannot be tied to one industry) and traveled unknown distances to get to the location of consumption in the river. It is not unrealistic to think that the large urban areas located along the river could be a source of polymers, simply because polymers are associated with human population and industry. A study of microplastic pollution in the Rhine River, Germany showed sections of the river near highly populated cities having high microplastic concentrations (Mani et al., 2015). Along with surface runoff during storm events, urban areas are home to large wastewater treatment facilities that have been reported to be a source of microplastic pollution (Browne et al., 2011).
Only secondary microplastics were found within the stomachs of the fish from our study. Fish stomachs contained plastic fragments, fibers, and foams that likely broke apart from larger plastics over time. Foams have a density <1 g/cm3 and will consequently float, which is consistent with foams only occurring in Bluegill and Largemouth Bass—both species known to feed on floating prey (McNaught and Hasler, 1961; Keast, 1978). We also found fibers in Bluegill stomachs, which is consistent with other studies reporting microplastic shapes in Bluegill (Peters and Bratton, 2016; Park et al., 2020). We found no primary microplastics in fishes, even though the Mississippi River is used as a transportation system for many industries that use primary microplastics, such as raw resin pellets or nurdles. Although we do not know why we did not observe any nurdles, it may be because primary microplastics are less abundant than secondary microplastics in aquatic environments (Hale et al., 2020), or types of microplastics aggregate in different parts of the river system.
5 Study limitations
As with any study of fish, sampling gear should be considered for its effect(s) on the results. The primary gear used to sample fish was electrofishing, and there are no known direct effects of electrofishing on stomach contents or stomach fullness, compared to other common gears like traps, where fish could remain for hours without food (potentially effecting stomach fullness). Electrofishing may have a differential effect on fishes of different sizes; however, the habitats sampled are more likely to influence the sizes of fish that were captured (and which are determined by the existing sampling programs). Although we cannot quantify it, one possible effect of the sampling methodology is that fish sampling tends to take place during the day when governmental agencies work, and if any species (or individuals) feed at night their diets could be misrepresented in our work. A laboratory consideration worth noting is the temperature used for KOH digestion. We used 60°C, which is based on work by Foekema et al. (2013) and supported by Dehaut et al. (2016) and Zhang et al. (2020) to not degrade any polymers. However, other studies (e.g., Karami et al., 2017; Thiele et al., 2019) have reported polymer degradation at 60°C and recommend 40°C, which may prevent degradation and still effectively digest samples.
Another consideration that we were not able to quantify well is river discharge or sediment at exact sampling locations. Although there are point measurements of discharge for locations along the Mississippi River, a single integrated estimate of discharge would need to be applied to what are often very heterogeneous riverine habitats that are sampled. For instance, some sampling events may take place in the thalweg, but many samples are likely taken from backwaters, side channels, pools, and other habitats that are only indirectly described by a single discharge estimate. It is worth noting, however, that both years of sampling (especially 2019) saw very high river levels, and if the extreme values of discharge effectively diluted microplastics throughout the river system (Scircle et al., 2020), it could explain why we observed generally low percent occurrence of microplastics in fishes. Sediment is also constantly changing in the mainstem Mississippi River, and although sediment may be a source of microplastics (especially for benthic species) and could be informative toward understanding microplastics in fishes, no detailed sediment data was available.
6 Conclusion
This study provides the first large scale examination and confirmation of microplastic pollution in the stomach of fishes from the Mississippi River. Microplastics were present in four widely distributed fish species in the Mississippi River, but generally at low levels of occurrence. Largemouth bass were found to have increasing microplastic loads with increasing distance downstream; however, the overall counts of microplastics were not as high as reported in other studies. Microplastic effects on fish are a continued area of study, in addition to any indirect effects of fish consumption by humans. Given the extreme anthropogenic influence on the Mississippi River, further investigations should consider other fish species and consider taking concurrent water samples in order to best understand the local environments and microplastics availability to fish.
Data availability statement
The original contributions presented in the study are included in the article/Supplementary Material, further inquiries can be directed to the corresponding author.
Ethics statement
Ethical review and approval was not required for the animal study because no live organisms were handled.
Author contributions
Conceptualization, methodology, formal analysis, investigation, data curation, writing—original draft preparation, writing—review and editing, and visualization KT, AG, MB, and SM; funding acquisition, SM. All authors have read and agreed to the published version of the manuscript.
Funding
This research was funded by Louisiana Sea Grant College Program under the National Oceanic and Atmospheric Administration Award NA18OAR4170098, part of Program Project ID 2018 R/CWQ-08-PD.
Acknowledgments
This study would not have been possible without very helpful and supportive collections made by numerous individuals at the Minnesota Department of Natural Resources, the Wisconsin Department of Natural Resources, the Missouri Department of Conservation, and the Louisiana Department of Wildlife and Fisheries for providing samples. AG was supported by the Fulbright International Program and Louisiana State University. Lucas Pensinger, Kenneth Erickson, David Smith, Josef Schuster, and Emma Guidry helped with sample preparation and analysis.
Conflict of interest
The authors declare that the research was conducted in the absence of any commercial or financial relationships that could be construed as a potential conflict of interest.
Publisher’s note
All claims expressed in this article are solely those of the authors and do not necessarily represent those of their affiliated organizations, or those of the publisher, the editors and the reviewers. Any product that may be evaluated in this article, or claim that may be made by its manufacturer, is not guaranteed or endorsed by the publisher.
Supplementary material
The Supplementary Material for this article can be found online at: https://www.frontiersin.org/articles/10.3389/fenvs.2022.1065583/full#supplementary-material
References
Abrahams, M. V., and Kattenfeld, M. G. (1997). The role of turbidity as a constraint on predator-prey interactions in aquatic environments. Behav. Ecol. Sociobiol. 40, 169–174. doi:10.1007/s002650050330
Anderson, J. C., Park, B. J., and Palace, V. P. (2016). Microplastics in aquatic environments: Implications for Canadian ecosystems. Environ. Pollut. 218, 269–280. doi:10.1016/j.envpol.2016.06.074
Andrade, M. C., Winemiller, K. O., Barbosa, P. S., Fortunati, A., Chelazzi, D., Cincinelli, A., et al. (2019). First account of plastic pollution impacting freshwater fishes in the Amazon: Ingestion of plastic debris by piranhas and other serrasalmids with diverse feeding habits. Environ. Pollut. 244, 766–773. doi:10.1016/j.envpol.2018.10.088
Barnes, D. K., Galgani, F., Thompson, R. C., and Barlaz, M. (2009). Accumulation and fragmentation of plastic debris in global environments. Philosophical Trans. R. Soc. B Biol. Sci. 364 (1526), 1985–1998. doi:10.1098/rstb.2008.0205
Benfield, M. C., and Minello, T. J. (1996). Relative effects of turbidity and light intensity on reactive distance and feeding of an estuarine fish. Environ. Biol. Fishes 46, 211–216. doi:10.1007/bf00005223
Browne, M. A., Crump, P., Niven, S. J., Teuten, E., Tonkin, A., Galloway, T., et al. (2011). Accumulation of microplastic on shorelines woldwide: Sources and sinks. Environ. Sci. Technol. 45, 9175–9179. doi:10.1021/es201811s
Carbery, M., O'Connor, W., and Palanisami, T. (2018). Trophic transfer of microplastics and mixed contaminants in the marine food web and implications for human health. Environ. Int. 115, 400–409. doi:10.1016/j.envint.2018.03.007
Carpenter, E. J., and Smith, K. L. (1972). Plastics on the Sargasso Sea surface. Science 175, 1240–1241. doi:10.1126/science.175.4027.1240
Cole, M., Lindeque, P., Halsband, C., and Galloway, T. S. (2011). Microplastics as contaminants in the marine environment: A review. Mar. Pollut. Bull. 62, 2588–2597. doi:10.1016/j.marpolbul.2011.09.025
Cole, M., Lindeque, P., Fileman, E., Halsband, C., Goodhead, R., Moger, J., et al. (2013). Microplastic ingestion by zooplankton. Environ. Sci. Technol. 47, 6646–6655. doi:10.1021/es400663f
Collard, F., Gasperi, J., Gilbert, B., Eppe, G., Azimi, S., Rocher, V., et al. (2018). Anthropogenic particles in the stomach contents and liver of the freshwater fish Squalius cephalus. Sci. Total Environ. 643, 1257–1264. doi:10.1016/j.scitotenv.2018.06.313
Collard, F., Gasperi, J., Gabrielsen, G. W., and Tassin, B. (2019). Plastic particle ingestion by wild freshwater fish: A critical review. Environ. Sci. Technol. 53, 12974–12988. doi:10.1021/acs.est.9b03083
Dantas, D. V., Barletta, M., and Da Costa, M. F. (2012). The seasonal and spatial patterns of ingestion of polyfilament nylon fragments by estuarine drums (Sciaenidae). Environ. Sci. Pollut. Res. 19, 600–606. doi:10.1007/s11356-011-0579-0
Dehaut, A., Cassone, A. L., Frere, L., Hermabessiere, L., Himber, C., Rinnert, E., et al. (2016). Microplastics in seafood: Benchmark protocol for their extraction and characterization. Environ. Pollut. 215, 223–233. doi:10.1016/j.envpol.2016.05.018
Derraik, J. G. (2002). The pollution of the marine environment by plastic debris: A review. Mar. Pollut. Bull. 44 (9), 842–852. doi:10.1016/S0025-326X(02)00220-5
Di Mauro, R., Kupchik, M. J., and Benfield, M. C. (2017). Abundant plankton-sized microplastic particles in shelf waters of the northern Gulf of Mexico. Environ. Pollut. 230, 798–809. doi:10.1016/j.envpol.2017.07.030
Duis, K., and Coors, A. (2016). Microplastics in the aquatic and terrestrial environment: Sources (with a specific focus on personal care products), fate and effects. Environ. Sci. Eur. 28, 2. doi:10.1186/s12302-015-0069-y
Eerkes-Medrano, D., Thompson, R. C., and Aldridge, D. C. (2015). Microplastics in freshwater systems: A review of the emerging threats, identification of knowledge gaps and prioritisation of research needs. Water Res. 75, 63–82. doi:10.1016/j.watres.2015.02.012
Fendall, L. S., and Sewell, M. A. (2009). Contributing to marine pollution by washing your face: Microplastics in facial cleansers. Mar. Pollut. Bull. 58, 1225–1228. doi:10.1016/j.marpolbul.2009.04.025
Foekema, E. M., De Gruijter, C., Mergia, M. T., Van Franeker, J. A., Murk, A. J., and Koelmans, A. A. (2013). Plastic in North Sea fish. Environ. Sci. Technol. 47, 8818–8824. doi:10.1021/es400931b
Frias, J. P., Otero, V., and Sobral, P. (2014). Evidence of microplastics in samples of zooplankton from Portuguese coastal waters. Mar. Environ. Res. 95, 89–95. doi:10.1016/j.marenvres.2014.01.001
Geyer, R., Jambeck, J. R., and Law, K. L. (2017). Production, use, and fate of all plastics ever made. Sci. Adv. 3, e1700782. doi:10.1126/sciadv.1700782
Goolsby, D. A., and Battaglin, W. A. (2001). Long-term changes in concentrations and flux of nitrogen in the Mississippi River Basin, USA. Hydrol. Process. 15 (7), 1209–1226. doi:10.1002/hyp.210
Gutreuter, S., Burkhardt, R., and Lubinski, K. (1995). USGS science for a changing world. in Long term resource monitoring program procedures: Fish monitoring. Editor E. M. T. C. National Biological Service (Onalaska, Wisconsin: USGS).
Hale, R. C., Seeley, M. E., La Guardia, M. J., Mai, L., and Zeng, E. Y. (2020). A global perspective on microplastics. J. Geophys. Res. Oceans 125. doi:10.1029/2018jc014719
Hoss, D. E., and Settle, L. R. (1990). “Ingestion of plastics by teleost fishes,” in Proceedings of the second international conference on marine debris (Miami, FL: NOAA technical memorandum. NOAA-TM-NMFS-SWFSC-154).
Hurt, R., O'Reilly, C. M., and Perry, W. L. (2020). Microplastic prevalence in two fish species in two United State reservoirs. L&O Letters 5, 147–153. doi:10.1002/lol2.10140
Jabeen, K., Su, L., Li, J., Yang, D., Tong, C., Mu, J., et al. (2017). Microplastics and mesoplastics in fish from coastal and fresh waters of China. Environ. Pollut. 221, 141–149. doi:10.1016/j.envpol.2016.11.055
Karami, A., Golieskardi, A., Choo, C. K., Romano, N., Ho, Y. B., and Salamatinia, B. (2017). A high-performance protocol for extraction of microplastics in fish. Sci. Total Environ. 578, 485–494. doi:10.1016/j.scitotenv.2016.10.213
Keast, A. (1978). Feeding interrelations between age-groups of pumpkinseed (Lepomis gibbosus) and comparisons with Bluegill (L. macrochirus). JFRBC 35, 12–27. doi:10.1139/f78-003
Lusher, A. L., Mchugh, M., and Thompson, R. C. (2013). Occurrence of microplastics in the gastrointestinal tract of pelagic and demersal fish from the English Channel. Mar. Pollut. Bull. 67, 94–99. doi:10.1016/j.marpolbul.2012.11.028
Mani, T., Hauk, A., Walter, U., and Burkhardt-Holm, P. (2015). Microplastics profile along the Rhine River. Sci. Rep. 5, 17988. doi:10.1038/srep17988
McGoran, A. R., Clark, P. F., and Morritt, D. (2017). Presence of microplastic in the digestive tracts of European flounder, Platichthys flesus, and European smelt, Osmerus eperlanus, from the River Thames. Environ. Pollut. 220, 744–751. doi:10.1016/j.envpol.2016.09.078
McNaught, D. C., and Hasler, A. D. (1961). Surface schooling and feeding behavior in the white bass, Roccus chrysops (rafinesque), in Lake Mendota. Limnol. Oceanogr. 6, 53–60. doi:10.4319/lo.1961.6.1.0053
McNeish, R. E., Kim, L. H., Barrett, H. A., Mason, S. A., Kelly, J. J., and Hoellein, T. J. (2018). Microplastic in riverine fish is connected to species traits. Sci. Rep. 8, 11639. doi:10.1038/s41598-018-29980-9
Neves, D., Sobral, P., Ferreira, J. L., and Pereira, T. (2015). Ingestion of microplastics by commercial fish off the Portuguese coast. Mar. Pollut. Bull. 101, 119–126. doi:10.1016/j.marpolbul.2015.11.008
Okamoto, K., Nomura, M., Horie, Y., and Okamura, H. (2022). Color preferences and gastrointestinal-tract retention times of microplastics by freshwater and marine fishes. Environ. Pollut. 304, 119253. doi:10.1016/j.envpol.2022.119253
Park, T. J., Lee, S. H., Lee, M. S., Lee, J. K., Lee, S. H., and Zoh, K. D. (2020). Occurrence of microplastics in the Han River and riverine fish in South Korea. Sci. Total Environ. 708, 134535. doi:10.1016/j.scitotenv.2019.134535
Pegado, T., Schmid, K., Winemiller, K. O., Chelazzi, D., Cincinelli, A., Dei, L., et al. (2018). First evidence of microplastic ingestion by fishes from the Amazon River estuary. Mar. Pollut. Bull. 133, 814–821. doi:10.1016/j.marpolbul.2018.06.035
Peters, C. A., and Bratton, S. P. (2016). Urbanization is a major influence on microplastic ingestion by sunfish in the Brazos River Basin, Central Texas, USA. Environ. Pollut. 210, 380–387. doi:10.1016/j.envpol.2016.01.018
Phillips, M. B., and Bonner, T. H. (2015). Occurrence and amount of microplastic ingested by fishes in watersheds of the Gulf of Mexico. Mar. Pollut. Bull. 100, 264–269. doi:10.1016/j.marpolbul.2015.08.041
Possatto, F. E., Barletta, M., Costa, M. F., Do Sul, J. A., and Dantas, D. V. (2011). Plastic debris ingestion by marine catfish: An unexpected fisheries impact. Mar. Pollut. Bull. 62, 1098–1102. doi:10.1016/j.marpolbul.2011.01.036
R Core Team, (2022). R: A language and environment for statistical computing. Vienna, Austria: R Foundation for Statistical Computing.
Ramos, J. A. A., Barletta, M., and Costa, M. F. (2012). Ingestion of nylon threads by Gerreidae while using a tropical estuary as foraging grounds. Aquat. Biol. 17, 29–34. doi:10.3354/ab00461
Rochman, C. M., Hoh, E., Kurobe, T., and Teh, S. J. (2013). Ingested plastic transfers hazardous chemicals to fish and induces hepatic stress. Sci. Rep. 3, 3263. doi:10.1038/srep03263
Romeo, T., Pietro, B., Peda, C., Consoli, P., Andaloro, F., and Fossi, M. C. (2015). First evidence of presence of plastic debris in stomach of large pelagic fish in the Mediterranean Sea. Mar. Pollut. Bull. 95, 358–361. doi:10.1016/j.marpolbul.2015.04.048
Ross, S. T., and Brenneman, W. M. (2001). The inland fishes of Mississippi. America: Univ. Press of Mississippi.
Rummel, C. D., Loder, M. G., Fricke, N. F., Lang, T., Griebeler, E. M., Janke, M., et al. (2016). Plastic ingestion by pelagic and demersal fish from the North Sea and Baltic Sea. Mar. Pollut. Bull. 102, 134–141. doi:10.1016/j.marpolbul.2015.11.043
Sanchez, W., Bender, C., and Porcher, J. M. (2014). Wild gudgeons (Gobio gobio) from French rivers are contaminated by microplastics: Preliminary study and first evidence. Environ. Res. 128, 98–100. doi:10.1016/j.envres.2013.11.004
Scircle, A., Cizdziel, J. V., Missling, K., Li, L., and Vianello, A. (2020). Single-pot method for the collection and preparation of natural water for microplastic analyses: Microplastics in the Mississippi River System during and after historic flooding. Environ. Toxicol. Chem. 39, 986–995. doi:10.1002/etc.4698
Silva-Cavalcanti, J. S., Silva, J. D. B., Franca, E. J., Araujo, M. C. B., and Gusmao, F. (2017). Microplastics ingestion by a common tropical freshwater fishing resource. Environ. Pollut. 221, 218–226. doi:10.1016/j.envpol.2016.11.068
Steer, M., Cole, M., Thompson, R. C., and Lindeque, P. K. (2017). Microplastic ingestion in fish larvae in the Western English Channel. Environ. Pollut. 226, 250–259. doi:10.1016/j.envpol.2017.03.062
Thiele, C. J., Hudson, M. D., and Russell, A. E. (2019). Evaluation of existing methods to extract microplastics from bivalve tissue: Adapted KOH digestion protocol improves filtration at single-digit pore size. Mar. Pollut. Bull. 142, 384–393. doi:10.1016/j.marpolbul.2019.03.003
Thompson, R. C., Swan, S. H., Moore, C. J., and Vom Saal, F. S. (2009). Our plastic age. Philosophical Trans. R. Soc. B 364, 1971–1976. doi:10.1098/rstb.2009.0054
Toner, K., and Midway, S. R. (2021). Historic fish samples from the Southeast USA lack microplastics. Sci. Total Environ. 776. doi:10.1016/j.scitotenv.2021.145923
Van Wezel, A., Caris, I., and Kools, S. A. (2016). Release of primary microplastics from consumer products to wastewater in the Netherlands. Environ. Toxicol. Chem. 35, 1627–1631. doi:10.1002/etc.3316
Von Moos, N., Burkhardt-Holm, P., and Köhler, A. (2012). Uptake and effects of microplastics on cells and tissue of the blue mussel Mytilus edulis L. after an experimental exposure. Environ. Sci. Technol. 46 (20), 11327–11335. doi:10.1021/es302332w
Keywords: largemouth bass, flathead catfish, bluegill, polypropylene, fragment
Citation: Gad AK, Toner K, Benfield MC and Midway SR (2023) Microplastics in mainstem Mississippi River fishes. Front. Environ. Sci. 10:1065583. doi: 10.3389/fenvs.2022.1065583
Received: 09 October 2022; Accepted: 12 December 2022;
Published: 18 January 2023.
Edited by:
Albert Chakona, South African Institute for Aquatic Biodiversity, South AfricaReviewed by:
Leandro E. (Steve) Miranda, Mississippi State University, United StatesFrance Collard, Norwegian Polar Institute, Norway
Copyright © 2023 Gad, Toner, Benfield and Midway. This is an open-access article distributed under the terms of the Creative Commons Attribution License (CC BY). The use, distribution or reproduction in other forums is permitted, provided the original author(s) and the copyright owner(s) are credited and that the original publication in this journal is cited, in accordance with accepted academic practice. No use, distribution or reproduction is permitted which does not comply with these terms.
*Correspondence: Stephen R. Midway, smidway@lsu.edu
†These authors have contributed equally to this work and share first authorship