- 1Laboratório de Ecologia do Ictioplâncton e Pesca em Águas Interiores, Universidade Federal do Oeste do Pará, Santarém, Brazil
- 2Programa de Pós-Graduação em Ecologia Aquática e Pesca, Núcleo de Ecologia Aquática e Pesca da Amazônia, Universidade Federal do Pará, Belém, Brazil
- 3Programa de Pós-Graduação em Ecologia, Universidade Federal do Pará, Instituto de Ciências Biológicas-ICB, Belém, Brazil
- 4Centro de Ciências Humanas, Naturais, Saúde e Tecnologia, Universidade Federal do Maranhão, Pinheiro, Brazil
The reproduction of neotropical fish is linked to environmental stimuli that act as triggers in this process. Thus, it is possible to affirm that specific environmental changes can delimit most fish’s period and reproductive success. El Niño Southern Oscillation phenomena (ENSO) influence rainfall and, consequently, hydrological dynamics, affecting several fish population aspects, especially reproductive aspects. However, in the Amazon, empirical evidence of the effect of anomalous climatic events on fish reproduction is incipient. In this sense, we investigated the taxonomic and functional structure of parental fish larvae stocks and how species modulate their reproductive activities before (2013 and 2014) and during La Niña (2018) and El Niño (2019) events. The data evidence that the larval assemblages suffered alterations in the taxonomic and functional composition between the three analyzed periods and a pattern of temporal distribution with a high influence of environmental variables. Flow, pH, and electrical conductivity were the most important variables to modulate fish reproductive intensity between periods. The species seem to respond to combinations of these variables that reflect environmental conditions conducive to biological recruitment according to their ecological characteristics, allowing the perpetuation or decline of their abundance in each period. All species of commercial interest associated with the neutral period showed a sharp decline in the subsequent moments. These results suggest that the incidence of anomalous climatic events added to regional fisheries exploitation can negatively affect the phenology and demographic patterns of larvae assemblages on a short temporal scale. During the neutral period, the community was dominated by larvae of periodic large, herbivores, piscivores, epibenthic maneuverable, and nektonic burst swimmers. The decrease in the abundance of certain functional groups at the neutral and La Niña period favored an accelerated ecological succession during the El Niño period, due to the increase of species from basal trophic levels (planktivores), generalists (omnivores), highly resilient (intermediate strategists), sedentary, short-distance migrants and little commercially exploited. Thus, the results contribute to the understanding of the interactions between anomalous climatic events and the reproduction of fish with different ecological characteristics in the Amazon River.
1 Introduction
El Niño Southern Oscillation (ENSO) events are associated with abnormal surface water warming that occurs at irregular intervals in the tropical Pacific and can affect global climate (Marengo et al., 2012). Severe ENSO seasons affect not only the Pacific Ocean but the inland waters of the entire planet (Ward et al., 2014). In the northern region of Brazil, El Niño episodes cause drastic changes in rainfall patterns and consequently promote more extreme droughts than normal, while La Niña episodes have the opposite effect (Reboita et al., 2021). In Amazonian river systems, ENSO effects are reflected by changes in flow conditions, limnological variables, lateral connectivity, and seasonal biogeochemical cycles (Towner et al., 2021). During El Niño, the absence of rain causes, among others, severe droughts, decreased lateral connectivity, intense forest fires, and reduced floodplain areas. Meanwhile, the La Niña seasons are marked by high hydrological and flow levels, large amounts of rainfall, increased biological productivity, and a diversity of habitats suitable for the development of associated organisms (Towner et al., 2021). Additionally, La Niña seasons are related to abundant fishing harvests in the Amazon (Isaac et al., 2016).
Although the Amazon ichthyofauna is very adapted to the intense hydrological dynamics that naturally occur in this system, due to the periodicity of the flood pulse, studies indicate that extreme climatic events can cause significant and lasting changes in the taxonomic composition and functional diversity of the fish community in floodplain environments (Freitas et al., 2013; Röpke et al., 2017). The way the ichthyofauna responds to the existing environmental conditions during anomalous hydrological events is related to the ecological characteristics of each species and that often allow them to support or not such conditions (Chessman, 2013; Chea et al., 2020). The climatic anomalies associated with ENSO impact several population aspects of fish, especially reproductive ones. For example, prolonged droughts and mild floods can cause high mortality of larvae and juveniles, lower proportions of females with mature gonads, and decreased size at first maturity (Castello et al., 2019; Röpke et al., 2022). On the other hand, some species of periodic and migratory fish benefit from larger nursery areas, feeding areas, and the connectivity between the main channel and the floodplains during La Niña events when the flood is intense (Oliveira et al., 2014; Camacho Guerreiro et al., 2021; Cataldo et al., 2022).
Although studies indicate the existence of the effect of La Niña and El Niño on the reproductive success of ichthyofauna, few investigations have been carried out on this topic, especially in the Amazon. Empirical information from the perspective of the ichthyoplankton community stands out as a fundamental tool to assess the effect of changes in environmental gradients on fish reproduction (Cajado et al., 2020; Tyler et al., 2021). Ichthyoplankton dynamics reflect the status of commercially and ecologically important fish populations and provide predictive answers about potential future recruitment (Zacardi et al., 2017a; Humphries et al., 2020).
Understanding the response of the fish community to climatic anomalies through a functional face applied to ichthyoplankton becomes fundamental because it makes it possible to identify a more predictable response of biological communities to environmental changes (Arantes et al., 2019a; Gogola et al., 2021). Information of this nature would contribute to the understanding of the effect of climate change on the reproductive aspects of Neotropical fish. This fact is aggravated because, in recent decades, events such as ENSO have become increasingly intense and frequent in the tropics due to increasing changes in the global climate (Espinoza et al., 2019); and thus, studies of their effects on ichthyoplankton communities can help in decision-making for effective management measures for the conservation of fisheries resources.
In this study, we evaluated the possible influences of ENSO on the taxonomic and functional structure of the fish larvae community in the surroundings of a Lower Amazon River floodplain. Environmental variables with potential influence on the structure of ichthyoplankton assemblages were also included in our analyses. We collected ichthyoplankton in three hydrological periods: neutral, La Niña, and El Niño during the rising of river water, the reproductive phase of most fish species in the Lower Amazon (Zacardi et al., 2017b), to test the hypothesis that anomalous climatic events significantly affect fish reproduction, with changes in demographic aspects and in the taxonomic and functional structure of fish larvae assemblages. We expect that the response of species to climate change will be influenced by their functional traits. For example, pelagic fish larvae must be associated with different periods than benthic species, because the former may be more sensitive to changes in water masses than the latter, which have more geographically fixed, near-bottom habitat requirements (Duarte et al., 2020, 2022; Silva et al., 2020). In addition, periodic species, as well as medium-distance migrators, should be more abundant during La Niña, due to favorable conditions for the reproduction of these fish, such as greater amounts of rainfall and river flow (Zacardi et al., 2017b; Humphries et al., 2020). Finally, we believe that commercially exploited species will have their reproduction more affected, given that previous studies have observed the effect of climatic events on the reproductive parameters of these fish (Röpke et al., 2022).
2 Material and methods
2.1 Study area
This study was conducted in Lower Amazon River near the city of Santarém, state of Pará, Brazil. Specifically, the study was developed in the main channel of the river along marginal areas of a complex of alluvial islands locally known as “Ilha das Marrecas” (2°12′41″ S and 2°18′52″ S, 54° 45′42″ W and 54° 43′11″ W) (Figure 1). Amazon River, responsible for almost 95% of all discharge in the Amazon basin, with an average flow of 209,000 (m−³. s), is classified as a whitewater river, having high dissolved oxygen concentrations and conductivity electrical, almost neutral pH, and large concentrations of nutrient-rich sediments, making it a highly dynamic and productive river (Barthem and Fabré, 2004; Junk et al., 2011). Due to the local characteristics of the river’s hydrodynamics, erosion processes, transport, and sediment deposition on the archipelago, this environment has annual changes in its structure. These changes generate a mosaic of habitats in the floodplain (i.e., channels, marginal lakes, extensive aquatic macrophytes stands) that favor success in the life cycle of many regional species (Zacardi et al., 2017b; Oliveira et al., 2020). It should be noted that the floodplain in the Amazon River is locally called “várzea” which represents regions of the fluvial plain flooded by white water rivers rich in sediments, coming from the Andes, which fertilize the soil favoring the growth and establishment of extensive areas of aquatic vegetation (Piedade et al., 2020), colonized by an association of invertebrates (entomofauna) and periphyton in the root system serving as food sources for many aquatic biota organisms (Sánchez-Botero et al., 2007; Magalhães et al., 2015).
The local climate is classified as the Am type in the Köppen system, that is, rainy tropical, with a restricted annual temperature range and mean monthly precipitation of over 60 mm. Mean air temperature is approximately 27.7°C and related humidity, is 86%, varying little during the year, while annual rainfall is approximately 2,000 mm (Costa et al., 2013).
The archipelago of the Ilha das Marrecas is an important fluvial and lake fishing area in the Lower Amazon, with the city of Santarém being the main fish market for landing and marketing fish (Pereira et al., 2019). Fisheries in the study area sustain per capita fish consumption rates of 40–94 kg yr−1, well above the global average of 16 kg yr−1 (Isaac et al., 2016). The fishing activity occurs intensively for eight months throughout the year and the composition of the species caught varies between the hydrological phases, but basically focuses on medium and large migratory species, representatives of the families Serrasalmidae (e.g., Mylossoma spp) Anostomidae (e.g., Schizodon spp.), Pimelodidae (e.g., Brachyplatystoma spp., Pseudoplatystoma spp.), Pristigasteridae (e.g., Pellona spp.), Sciaenidae (e.g., Plagioscion spp.) e Curimatidae (e.g., Psectrogaster spp.) (Isaac et al., 2016). Fisheries productivity is associated with several factors, but in general, the highest catches per unit of effort are associated with lower values of flow, river level, rainfall, and higher temperatures (Pinaya et al., 2016).
2.2 Data sampling
Ichthyoplankton were collected monthly during four reproductive periods between January and April 2013, 2014, 2018, and 2019. Sampling was carried out over four stations randomly distributed in the river channel at the edge of the archipelago. The collections were carried out during the daytime (3 pm–6 pm) and nighttime (8 pm–11 pm, UTC -3) aboard a local vessel at reduced speed, through horizontal trawls carried out in the subsurface (0–1 m) of the water column for 5 minutes using a conical plankton net with 300 μm mesh size and coupled mechanical flowmeter to obtain the volume of filtered water. The net was always held against the flow to increase capture efficiency. Fish larvae were euthanized with benzocaine (250 mg L−1) and then preserved in formalin (10%) and stored in polyethylene bottles labeled to be transported to the laboratory. The collection effort totaled 128 samples at the end of the study (16 months of collection x 4 sampling stations x 2 sampling times—day and night), and all samples contained fish larvae.
Concomitantly with the collection of ichthyoplankton, limnological variables such as water temperature (°C), dissolved oxygen (mg L−1), electrical conductivity (μS cm−1) and potential hydrogen (pH) were recorded using a multiparameter. Hydrological data (rainfall index, flow, and water levels) for each month of collection were obtained from the website of Brazilian Water Agency—ANA (http://hidroweb.ana.gov.br). The ENSO phenomena data were obtained by the National Oceanic and Atmospheric Administration—NOAA (www.psl.noaa.gov/data/climateindices/list/). The ENSO values were quantitatively expressed by the Oceanic Niño Index (ONI), where values greater than or equal to 0.5 represent El Niño events and values less than or equal to -0.5 La Niña events (values between -0.5 and 0.5 represent neutral periods when none of the events occur). Based on this index, the reproductive periods (months from January to April) of 2013 and 2014 were considered neutral, while 2018 was considered La Niña and 2019 El Niño. The license for the collection of biological material was granted by SISBIO/ICMBio/MMA through authorization number 72.330 issued based on Normative Instruction no 154/2007.
2.3 Sample processing
In the laboratory, samples were screened by seizing the fish larvae from the suspended material, debris, and total plankton, under stereoscopic microscope. Subsequently, they were identified at the lowest possible taxonomic level, based on the regressive development sequence technique (Nakatani et al., 2001) and morphological, meristic, and morphometric characteristics, using specialized bibliographies. The larvae classified as “unidentified” or at the order level corresponded to individuals with damaged structures and/or at a very early stage of development and made up less than 10% of the collected material. Thus, the specimens identified at the level of families belonging to Engraulidae, Doradidae and Auchenipteridae were included in the analyses as taxonomic units (e.g., Engraulidae gen. sp.) because larvae of these groups were relatively abundant in the samples, and their exclusion from the analyses could influence ordination results. The taxonomic status of the species followed (Fricke et al., 2022)
2.4 Functional classification
We assumed that captures of fish larvae in plankton reveal the adults individuals of the species are in reproductive activity in the region at a certain phase of the hydrological cycle. This assumption is reasonable because the structure of ichthyoplankton communities results from patterns in the reproductive behavior and gene flow of adults (Zacardi et al., 2020; Mariac et al., 2022). Thus, functional traits we used here were selected according to the biological aspects of adult fish and their relationship with ecosystem and community functions (Rosado et al., 2013; Winemiller et al., 2015; Gogola et al., 2021).
Each taxonomic unit was classified into four functional groups defined by 1) life history strategy, 2) feeding habits, 3) type of migration and 4) swimming-performance/microhabitat-use strategies according to (Arantes et al., 2018; 2019b; Silva et al., 2020). Individuals were classified based on species-level information and in cases of unavailable data available information on genera was used. Therefore, for Engraulidae gen. sp., Doradidae gen. sp. and Auchenipteridae gen. sp. functional characteristics were obtained considering traits of typical species for each of these families, based on the fish fauna inventories developed by (Imbiriba et al., 2020; Oliveira et al., 2020) in the study area. For Engraulidae gen. sp. the functional characteristics of the species of the genus Anchoviella Fowler 1911 were considered, which are local migratory fish, periodic strategists with maturation at small size, planktivores and nektonic maneuverable (Silva et al., 2020). While for Doradidae gen. sp., the species Nemadoras humeralis (Kner 1855), Ossancora punctata (Kner 1855) and Platydoras costatus (Linnaeus 1758) were considered, these species have convergent ecological characteristics, because they are sedentary, periodic strategists, omnivores and benthic-slow (Birindelli and Sousa, 2018). For Auchenipteridae gen. sp., the species Auchenipterichthys longimanus (Günther 1864) and Centromochlus heckelii (De Filippi 1853) were considered, both are sedentary of intermediate strategists, omnivores and benthic-slow (Freitas et al., 2011; Birindelli and Akama, 2018).
The species were classified according to four life history strategies taking into account the maximum body size, size at first maturation, fecundity and parental investment by individual offspring, as previously described by (Winemiller and Rose, 1992; Röpke et al., 2017; Arantes et al., 2018; 2019b): Intermediate strategists - have fecundity between 1,000 and 9,000 relatively large oocytes (diameter 1.4–2.0 mm) and intermediate degree of parental care (e.g., internal fertilization, so that oocytes are fertilized before spawn); Opportunistic - correspond to fish of small size (26–113 mm standard length (SL), early maturation (<60 mm SL), high and sustained reproductive effort, but low fecundity and no parental care; Periodic strategists with maturation at small size (63–148 mm SL) - have fecundity between 6,760 and 74,220 small oocytes (diameter of 0.5–1.3 mm), maximum size between 137 and 410 mm SL and no parental care; Periodic strategists with maturation at large sized (>164 mm SL)—characterized by having high fecundity between 1,000 and 202,960 small oocytes (diameter 0.7–1.6 mm), without parental care and maximum size >253 mm SL (Supplementary Material S1). Species of life history strategy in equilibrium were not captured due to the ecological characteristics of this group that form couples, usually build nests, take care of the offspring, and do not have planktonic eggs and larvae. Hence, the fishing gear employed was not able to capture them, since it was directed to the capture of planktonic organisms.
The species were classified according to six feeding strategies based on dietary information available in the scientific literature in: Herbivores—which feed predominantly on plant material (seeds, fruits or leaves) and filamentous algae; Omnivores - species that have a generalist diet without predominance of plant or animal tissue; Detritivores—which predominantly ingest fine particulate organic matter and periphytic algae; Planktivores - species that ingest phytoplankton, zooplankton and occasionally small amounts of plant material and debris; Carnivores—which feed on fish and numerous aquatic and terrestrial invertebrates (e.g., Ephemeroptera, Chironomidae, Coleoptera, Crustacea etc.); Piscivores—which feed on fish at any stage of ontogenetic development, whole or in pieces, including scales and fins.
In addition, migratory strategies were assigned based on dispersion behavior and distances of migrations performed by species. This classification was based on information previously described by García-Dávila et al. (2018) and Arantes et al. (2019b) in: Sedentary—resident species that spend their entire life cycle in lowland (from floodplain) habitats, including lakes, eventually performing short-distance movements. These species generally have small body or territorial behavior, and are often associated with substrates or structured habitats (e.g., tree branches and aquatic vegetation); Short-distance or lateral migrators (between 100 and 499 km)—fish migrating between river floodplain habitats, including major river channels and lakes, connecting channels and various vegetated habitats such as flooded forests; Medium-distance migrators (between 500 and 1,500 km)—comprise species that perform lateral migrations to floodplain habitats flooded during high water, but also perform longitudinal migrations (often hundreds of kilometers) along river channels during the receding water, to reproduce at the time of rising water; Long-distance or interborder migrators (>1,500 km)—comprise species that migrate thousands of kilometers along the river channels for trophic, dispersive and reproductive purposes, with the life cycle basically associated with the main channel of the river.
Finally, species were allocated according to five strategies of swimming-performance/microhabitat-use based on morphological characteristics previously described by (Arantes et al., 2019b) in: Nektonic maneuverable—fishes have laterally compressed body and upper mouth position. The morphological traits associated with efficient swimming performance are based on a hydrodynamic body and feeding within the water column; Nektonic burst swimmers—have fusiform body and mouth in terminal position. The morphological traits associated with efficient swimming performance are based on a hydrodynamic body and feeding within the water column; Epibenthic maneuverable—relatively deep body and less hydrodynamic than nektonic maneuverable fish, but efficient in making lateral and vertical curves. Eyes positioned more dorsally than laterally; Benthic-slow—relatively wide body, dorsally located eyes and inferior mouth, which are characteristic of the inhabitants of the river bottom. Low muscle mass and little area in pectoral and caudal fins. Benthic-fast—have relatively wide body, dorsally located eyes and inferior mouth, characteristic of the inhabitants of the river bottom. Higher muscle mass and caudal fin proportion—characteristics associated with a more efficient sustained swim compared to benthic-slow fish; Surface dwellers—partially compressed body, usually superior mouth, short or fusiform body. Eyes positioned more dorsally than laterally.
2.5 Data analyses
The abundances of fish larvae were standardized as volume of 10 m−3 of filtered water (number of organisms per 10 m−3) following Nakatani et al. (2001): Y = (X/V) × 10, where Y represents the density of larvae at 10 m−3, X represents the number of captured larvae and V represents the volume of filtered water. The final statistical analysis consisted of matrices of 128 samples and 55 species, four life history strategy groups, six trophic strategy groups, four migration groups and six swimming/microhabitat use groups. Samples were aggregated by climatic period (neutral, La Niña and El Niño), sampling season and time of day (day/night). For the ordination analyses (RDA and PCA) the environmental data were submitted to z-score transformation to standardize variables scales (Gotelli and Ellison, 2013). Dispersion plots and simple Pearson correlation tests were used to evaluate potential collinearity between explanatory variables before analysis. Subsequently, variance inflation factor (VIF) also evaluated the presence of multicollinearity between the variables, considering values up to 3.0 as the limit of multicollinearity (Bocard et al., 2011). Was metrics water level with the flow were highly collinear (r2 = 0.92), and therefore, the water level was excluded from the analysis as it contained the highest VIF (9.94). After removing water level, all variables showed low correlations (<5) and VIF lower than three.
The Principal Component Analysis (PCA) was used to reduce the dimensions of variables environmental and examine the existence of environmental differences between periods. Axes with eigenvalues greater than 1.0 were used for interpretation, according to the Kaiser-Guttman criterion. Only variables with score coefficients >0.4 were considered biologically important (Gotelli and Ellison, 2013).
Differences in temporal distribution patterns (neutral, La Niña, and El Niño - predictor variables) in the taxonomic and functional structure of fish larvae assemblages (response variables) were evaluated using abundance data (larvae density.10 m−³) and analyzed using a model-based multivariate approach (Warton et al., 2015) with the R package mvabund (version 4.1.3). The mvabund package uses simultaneous generalized linear models (GLMs), which specify the mean-variance relationship in the data (manyglm), which for the larval density data was a negative binomial distribution with a log-link function (confirmed by examining residual plots during model validation) (Warton et al., 2015). In addition, a distance-based approach, multivariate and univariate GLMs, using the same linkage function as in the previous model, was fitted to test the effect of ONI and environmental variables (electrical conductivity, pH, dissolved oxygen, rainfall, and flow) on the assemblage of fish larvae. The contributions of taxa to the sum of logarithmic odds ratios (or sum of multivariate deviations) for period effects (neutral, La Niña and El Niño), ONI, and environmental variables significant were investigated (with a correction applied to p-values to account for multiple tests). The nature of the significant contributions of taxa to the inter-period differences in the multivariate model was also investigated by fitting univariate models for each significant taxon in the mvabund.
A non-metric multidimensional scaling ordination (nMDS) was used to visualize the variations in the taxonomic composition of the larval assemblage between periods. To visualize the effects of variables from the best-fit GLM model variables on fish larvae assemblages the variables were plotted using Partial Redundancy Analysis (pRDAs) with emphasis on ONI, whose significance is evaluated by a test with 999 permutations. For the ordering for pRDA, the density values of the biological data matrix were log-chord transformed. This technique combines the logarithmic transformation that makes the distribution of species more symmetrical, reducing the effects of bias caused by abundant species with the chord transformation that removes the double zeros effect from the analysis (Legendre and Borcard, 2018). The pRDA was performed using the sampling stations, years, months, and time (day/night) as covariates to reduce the influences of spatial structure, interannual, monthly and sampling time, respectively. The relationships between larval density (10 m−³) of taxonomic species, and functional groups (life history strategy, feeding habits, type of migration and swimming-performance/microhabitat-use) with the Oceanic Niño Index and local environmental variables (flow, rainfall index, electrical conductivity, dissolved oxygen, pH and temperature) was evaluated. For the composition graph in RDA, only the species that showed significant differences between the three periods using the GLM model were used, precisely to facilitate the interpretation of the results graphically. These statistical analyses were performed in the Software R version 4.1.1 using the package Vegan (Oksanen et al., 2018).
3 Results
Environmental variables differed between the periods studied. The first two axes of PCA explained approximately 55% of the data variability. The La Niña period presented higher values of electrical conductivity, pH, and rainfall that were positively associated with PC1, while El Niño presented higher values of temperature, ONI, dissolved oxygen, and flow, variables associated more with PC2, except for flow that was negatively more associated with PC1 (Figure 2; Table 1). The neutral period recorded a gradient associated with the intermediate conditions environmental between the two periods of ENSO.
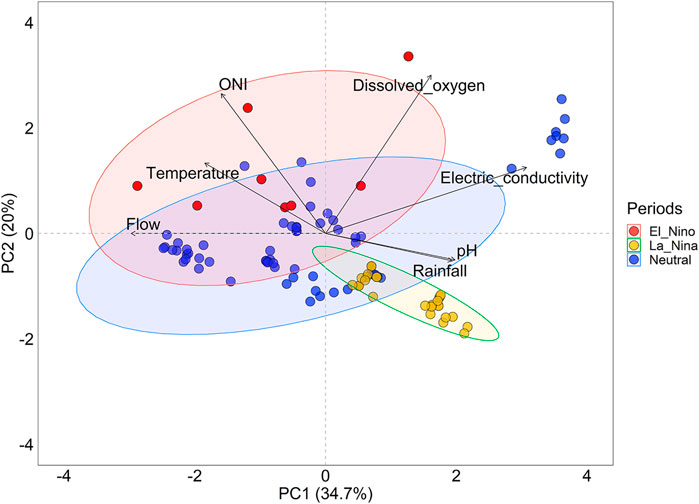
FIGURE 2. PCA analysis among variables environmental in the three sampled periods (neutral, La Niña, and El Niño) during the rising water in the Lower Amazon.
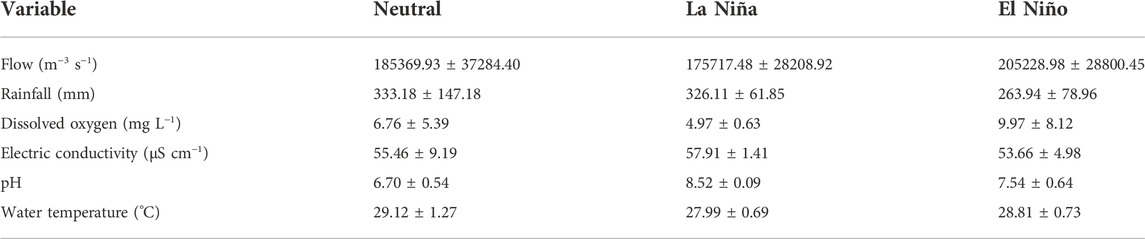
TABLE 1. Mean and standard deviation values of the flow and limnological variables of the lower Amazon River between three periods (neutral, La Niña, and El Niño).
A total of 50,484 fish larvae classified in nine orders, 27 families, 40 genera and 55 species were captured (Supplementary Material S1). The composition and abundance of larval assemblages differed significantly between the neutral (2013 and 2014), La Niña (2018) and El Niño (2019) periods (LR = 763.5, p-value = 0.001) (Figure 3). The assemblage differences between each period were driven by the significant contributions of 20 species (Figure 4). The mean density of larvae captured in the neutral period (123.05 larvae 10 m−3) was 13 times greater than the La Niña period (17.88 larvae.10 m−3) and about six times greater than the El Niño period (39.88 larvae.10 m−3).
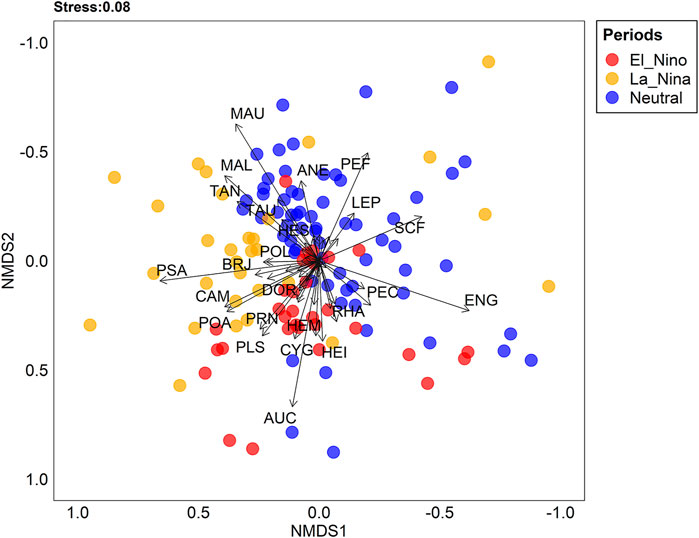
FIGURE 3. Non-metric multidimensional scaling ordinations (nMDS) visualizing period effects on fish assemblage composition. MAL—Mylossoma albiscopum, MAU—M. aureum, SCF—Schizodon fasciatus, PSA—Psectrogaster amazonica, POA—Potamorhina altamazonica, POL- P. latior, AUC—Auchenipteridae gen. sp., TAN—Triportheus angulatus, TAU–T. auritus, ENG—Engraulidae gen. sp., LES—Leporinus sp., PLF—Pellona flavipinnis, PEC—P. castelnaeana, PMB—Pimelodus blochii, PSP—Pseudoplatystoma punctifer, ANE—Anodus elongatus, CAM—Calophysus cf. macropterus, HEM—Hemiodus microlepis, PRN—Prochilodus nigricans, CYG—Cynodon gibbus, HEI—Hemiodus immaculatus, HES—Hemiodus sp., RHA—Rhinosardinia amazonica, BRJ—Brachyplatystoma juruense, PLS—Plagioscion squamosissimus and DOR—Doradidae gen. sp.
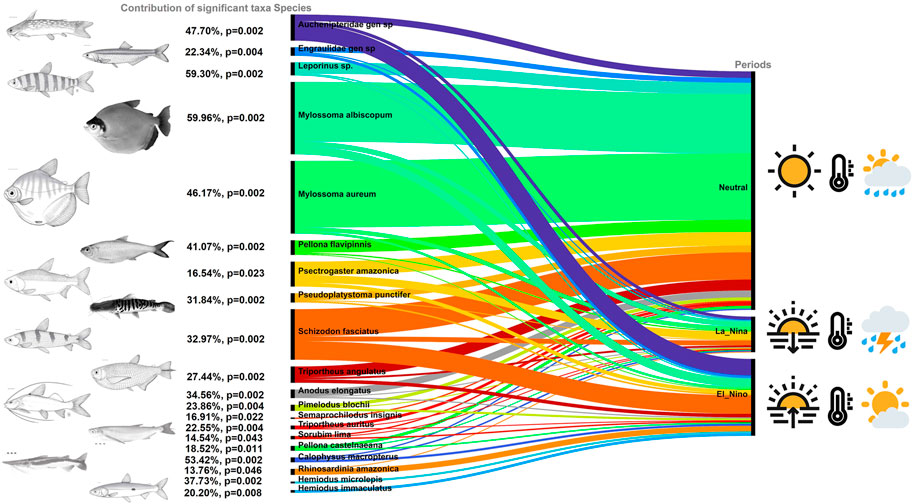
FIGURE 4. Summary of results from individual multivariate GLM for period effects on larval assemblage composition, the percent contribution of univariate model deviance of significant taxa to the parameter deviance in the multivariate model, and the associated p-value. Figures of the fish were adapted from Galvis et al. (2006) and Santos et al. (2006).
ONI and local environmental variables explained 9.66% of the change in species composition and had, except temperature and dissolved oxygen, significant effect on fish larval assemblages (RDA: permutation tests, df = 7, F = 3.55, p-value < 0.001). The first two axes of the RDA explained 13.44% of the restricted variation. The taxonomic structure of the larval assemblage was strongly associated with the environmental conditions arranged along the temporal gradient (Table 2; Figure 5). The larvae of the Characiformes Mylossoma aureum, M. albiscopum, Schizodon fasciatus, Leporinus sp., Anodus elongatus, Siluriformes Pseudoplatystoma punctifer, Sorubim lima, and Clupeiformes Pellona flavipinnis, P. castelnaeana and Engraulidae gen. sp. were more representative in the neutral period and showed a sharp decline in their abundances in subsequent periods (LR: p-value < 0.05) (Figure 4). Interestingly, all these species, except Engraulidae gen. sp., are highly commercially exploited in the Lower Amazon region. The curimatid Psectrogaster amazonica we associated with the La Niña period and were positively associated with high gradients of electrical conductivity (LR = 42.78, p-value = 0.001) and pH (LR = 12.43, p-value = 0.04). While Auchenipteridae gen. sp., Pimelodus blochii, and Hemiodus microlepis increased in abundance during the El Niño period, a fact evidenced by the association of these species with high ONI (Figure 5). Furthermore, the abundance of these species decreased with higher pH values (LR: p-value < 005).
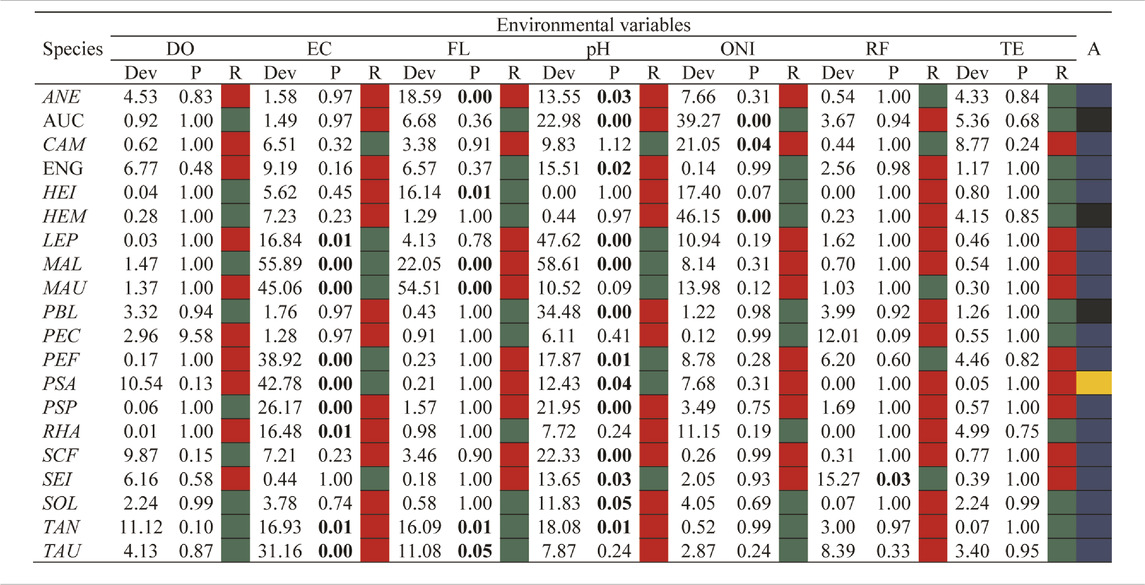
TABLE 2. Summary of GLM for the effects of environmental variables and periods on the taxonomic structure of fish larvae assemblages in the Lower Amazon. DO = Dissolved Oxygen, EC = Electrical Conductivity, FL = Flow, pH = Hydrogenonic Potential, ONI = Oceanic Niño Index, RF = Rainfall, TE = Temperature, A = association with the neutral period (color blue), El Niño (color black) and La Niña (color yellow). R = type of positive (color green) or negative (color red) relationship with the environmental variable. Values in bold indicate significance (p < 0.05). The abbreviation of the species names can be found in the legend of Figure 3.
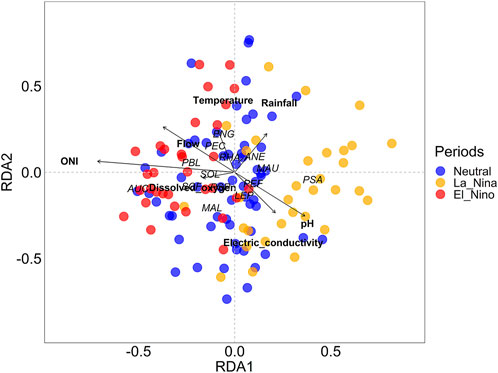
FIGURE 5. Redundancy analysis (RDA) showing the association between environmental variables and the taxonomic structure of fish larvae assemblages. Only the most representative species were displayed in the graph. A detailed list of species is available in Supplementary Material S1 and their abbreviations are in the legend of Figure 2.
The functional structures of the assemblages also changed between periods (LR = 739.4, p-value = 0.001) and were strongly modulated by ONI and local environmental variables (RDA: permutation tests, df = 7, F = 4.53, p-value < 0.001) that explain 11.61% of the change in the functional structure of the larval assemblage. Larvae of fish large periodicals, herbivores, piscivores, epibenthic maneuverable, and nektonic burst swimmers were more abundant during the neutral epoch and were negatively associated with electrical conductivity and pH and positively to the flow (p-value < 0.05) (Table 3). Larvae of fish migratory medium-distance, with a life history strategy periodic of small-sized, and maneuverable nektonic predominated during the La Niña season (2018), in addition, they were positively related to the high gradients of pH, electrical conductivity, and negatively to the flow. While larvae of species with intermediate life history strategy, sedentary, short-distance migratory, planktivores, omnivores as well as benthic slow and benthic fast species, were associated with El Niño (2019), a fact observed by the positive correlations of these groups with high ONI, in addition to being positively related to higher values of flow and temperature and negatively to rainfall, electrical conductivity, and pH (p-value < 0.05) (Figures 6A,B). Larvae of fish opportunistic life-history, as well as long-distance migrators, carnivores, and Surface dwellers, were not affected by the periods. However, they were negatively influenced by pH and electrical conductivity. Only long-distance migrants and Surface dwellers were not influenced by the variables included in our models.
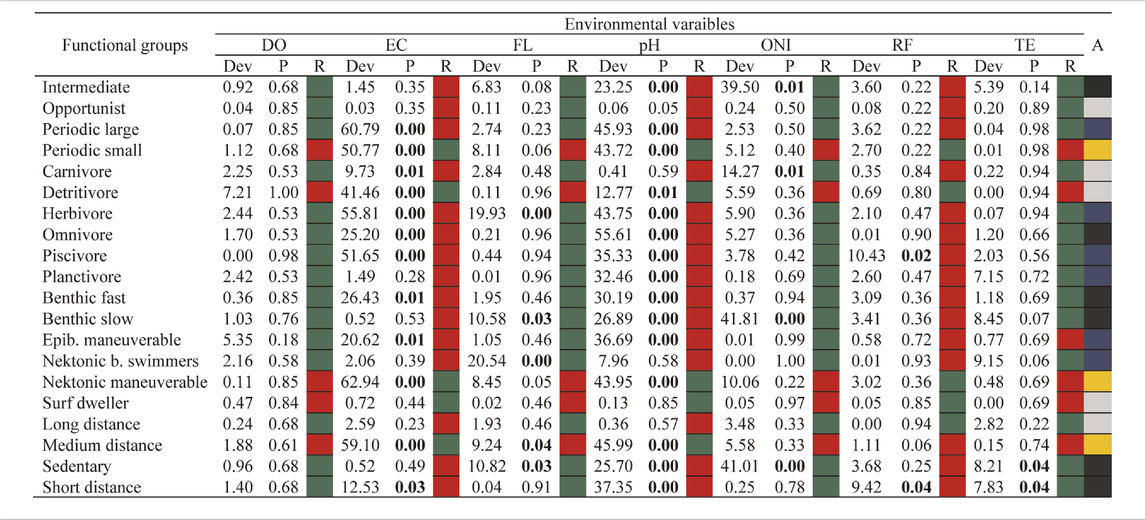
TABLE 3. Summary of GLM for the effects of environmental variables and periods on the functional structure of fish larvae assemblages in the Lower Amazon. DO = Dissolved Oxygen, EC = Electrical Conductivity, FL = Flow, pH = Hydrogenonic Potential, ONI = Oceanic Niño Index, RF = Rainfall, TE = Temperature, A = association with the neutral period (color blue), El Niño (color black) and La Niña (color yellow), and absence of association significative with periods (color gray). R = type of positive (color green) or negative (color red) relationship with the environmental variable. Values in bold indicate significance (p < 0.05).
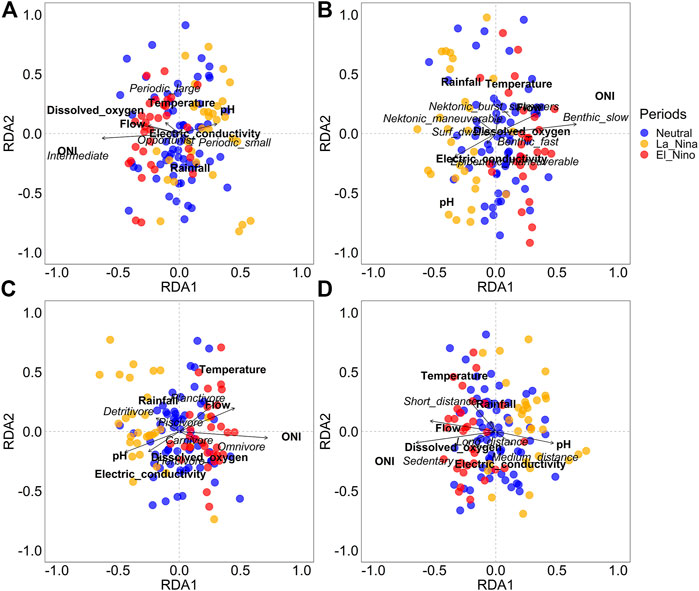
FIGURE 6. Redundancy analysis (RDA) showing the association between environmental variables and the functional structure of larval assemblages. (A) life history strategy, (B) feeding habits, (C) swimming/microhabitat use, and (D) migration.
4 Discussion
The patterns of association of larval community to hydrological periods are dependent on the ecological strategies of each species and show that climatic events modulate the structure of larval fish assemblages, corroborating our hypothesis. These findings contribute to an understanding of interactions between anomalous climatic events and fish reproduction in the Amazon River. Larvae of large periodic fish, piscivores, herbivores, maneuverable epibenthic, and nektonic burst swimmers predominated in the neutral period. On other hand, larvae of small periodicals and medium-distance migrators were more abundant in the La Niña period. In addition, larvae of fish with basal feeding habits (planktivores), generalists (omnivores), intermediate strategists, short-distance, and sedentary migrators, as well as benthic and commercially unexploited species, dominated assemblages during El Niño.
The environmental conditions were characteristic of each period, but the flow and precipitation values diverged from the proposed models for Amazon region throughout the seasonal cycle (Towner et al., 2021). Higher flows were observed during El Niño despite lower amounts of rainfall. Recent studies have documented abnormal precipitation in Andean regions caused by Pacific Decadal Oscillation (PDO), and ENSO in positive phases (Rodríguez-Morata et al., 2019; Mohammadi et al., 2020). This may favor higher temperatures in this period and, consequently, rapid melting of glaciers that contribute as one of the sources of water for the Amazon River, as observed by Rocha et al., 2019. It is known that the Andean thaw acts as a regulator of the hydrological regime and strongly affects geomorphology, biochemistry, and ecology of headwater tributaries of the Amazon basin (Filizola and Guyot, 2011; Vauchel et al., 2017), exporting a source of sediments, organic matter, and nutrients to the lower sectors of this basin.
The higher values of pH and electrical conductivity during La Niña possibly associated with high rainfall recorded during this period. The rainfall regime is a main regulatory agent of water courses, being responsible for the distribution of nutrients and ions in the water column, affecting concentrations of physicochemical variables in rivers (Esteves, 1998; Zuliani et al., 2016). According to Carvalho et al. (2000), during increased precipitation, pH tends to rise and approach neutrality, as there is a greater dilution of dissolved compounds and faster flow. This is caused by the increase in the volume of water which causes the acidity of the water to decrease. However, rainfall is responsible for leaching the soil from adjacent regions, influencing the periodic supply of suspended solids, particulate inorganic matter, and natural calcium carbonate in the region, and increasing turbidity (Silva et al., 2008). Thus, the electrical conductivity that depends on the ionic composition of water bodies is also influenced by the volume of local rainfall.
Our results showed that in the neutral period the mean density of larvae was much higher than in the La Niña and El Niño periods. This high variability in abundance between different periods has also been recorded in other river systems (Bednarski et al., 2008; Marschall et al., 2021). Changes in the flow and amount of rainfall have been suggested as plausible explanations for the variation in fish reproductive success in rivers with floodplain areas (King et al., 2009; Andrews et al., 2020; Stoffers et al., 2022). In fact, most species associated with the neutral period showed a positive relationship to flow, pH, and electrical conductivity, but precipitation was less important. In Amazonian rivers, increased flow, which is correlated at river level, is the main mechanism for transporting eggs and larvae to floodplain areas (Oliveira et al., 2020; Zacardi et al., 2020). It is noteworthy that the early stages of fish are critical due to their high susceptibility to predation and the need for readily available food resources for growth (Grenouillet and Pont, 2001; Montenegro et al., 2020). Therefore, the floodplain is considered an excellent nursery and natural breeding ground during the initial cycle of fish development (Bittencourt et al., 2020; Zacardi et al., 2020).
The studies by Baumgartner et al. (2008) and Tondato et al. (2010) also recorded the association of pH and electrical conductivity with the abundance of fish larvae, however, the effect of these variables on fish reproduction is not known for sure, but somehow, they induce migration and spawning for some species. Additionally, the decrease in larvae of fish of commercial interest after the neutral period may indicate some connection with fishing carried out in the region. Zacardi et al. (2018) verified the relationship between the production of landed fish and the abundance of larvae in the lower stretch of the Amazon River and observed that the fishing effort undertaken on adults compromises the spawning stock and, consequently, the annual recruitment of resources. The reproductive parameters of commercially exploited species are more susceptible to the effects of climatic events (Röpke et al., 2022), suggesting that environmental factors and fishing intensity can act simultaneously for the failure or success of fish recruitment in each period. Therefore, relatively minor changes in the reproductive success of large periodic can lead to poor recruitment for at least 2 years (Bayley et al., 2018; Castello et al., 2019; Cataldo et al., 2022).
The inferred effects of climatic events on the biodiversity of fish larvae indicate that the environmental conditions associated with ENSOs act as a filter in the functional structure of local assemblages, as observed in studies involving adult fish in the Amazon (Freitas et al., 2013; Röpke et al., 2017). Several functional groups had reproduction strongly associated with periods of high flux values, as they exploit favorable conditions for the transport of larvae to locations conducive to development. The large-size periodics decreased in abundance during ENSOs. These fish are more exploited by fisheries, in addition, they have a slow life cycle, being more sensitive to environmental changes (e.g., decreased flow) and anthropogenic pressures (Castello et al., 2019; Camacho Guerreiro et al., 2021). Larvae of many fish epibenthic maneuverable, herbivorous, and piscivorous benefit from increased flows as they are carried by rising water levels into recent floodplains to explore structurally complex habitats. For example, herbivore larvae (e.g., Mylossoma spp.) tend to colonize aquatic vegetation in search of refuge and food (Bittencourt et al., 2020; Oliveira et al., 2020), and piscivorous larvae maximize their survival by capturing abundant prey in the various habitats and micro-habitat of the floodplain (Tondato et al., 2010; Nunn et al., 2012; Grzybkowska et al., 2018).
On the other hand, although the lower flows during La Niña may indicate some disadvantages in dispersal mechanisms, the offspring of small periodic fish can take advantage of the low abundances of predatory fish larvae, such as piscivores and large periodic, to maximize recruitment. This strategy guarantees good ecological conditions and advantages for the survival of small periodic since predation is one of the main mortality factors during the initial ontogeny of fish (Nunn et al., 2012; Cajado et al., 2018; Humphries et al., 2020).
The El Niño period, in turn, was less favorable to the reproduction of some previously abundant functional groups (e.g., periodicals, medium-distance migrators, herbivores, and maneuverable epibenthic), suggesting that the combination of environmental conditions favorable to the reproduction of most of these groups did not occur effectively. This result corroborates the view that, regardless of anomalous hydrological periods, there is scaling at the time of spawning season between species (Shuai et al., 2016; Barthem et al., 2017; Tyler et al., 2021) and functional similarity reflects the preference between reproductive periods. For example, the associations of slow benthic and fast benthic fish larvae, as well as sedentary ones with the high fluxes in El Niño corroborate previous studies that demonstrate that species of these functional groups require minimum hydrological levels to trigger their reproduction (for example, greater than 5 m) (Cañas and Waylen, 2012; Hermann et al., 2021). This allows the larvae to enter the floodplain areas and explore the spatial patterns and nature of resources essential for successful recruitment, as reported by Duarte et al. (2020, 2022) and Zacardi and Ponte (2021). Thus, the period and extent of the flooding over the river plain significantly contribute to the generation and maintenance of the biodiversity of the Amazon ichthyofauna (Röpke et al., 2017; Frederico et al., 2021).
These examples further indicate that changes in environmental conditions associated with ENSOs favor some functional groups but are detrimental to others. However, a deeper understanding of how climatic events influence the structure of fish descendants can be obtained from the expansion of the sampling effort in spatial and temporal scales, inclusion of interactions between factors such as seasonal periods, water level elevation intervals, river, prey availability, lateral connectivity, and forest cover.
5 Conclusion
The variation in the taxonomic and functional structure of the fish larvae community between periods highlights the potential for extreme weather events as a factor affecting fish reproduction in the Amazon River. Despite this evidence, further studies are needed to better understand the processes that shape the reproductive dynamics of fish under anomalous hydrological conditions in the Amazon basin. Although we can only assume, the interaction between hydrological variations and factors such as changes in local environmental variables, ecological relationships, and fisheries seem to enable the success of certain functional groups in each period. The findings of this study can serve as a baseline to assist the implementation of protective measures on reproductive fish stocks, for example, in times of ENSO under atypical flow conditions and changes in environmental characteristics, where the intensity of reproduction and the biological recruitment is predictable, and thus adjust the duration of fish reproduction protection periods. An alternative widely used in the region as an effective action for the conservation of species and the sustainable use of fisheries resources.
Data availability statement
The raw data supporting the conclusion of this article will be made available by the authors, without undue reservation.
Ethics statement
Ethical review and approval was not required for the animal study because the license for the collection of biological material was granted by SISBIO/ICMBio/MMA through authorization number 72.330 issued based on Normative Instruction no 154/2007.
Author contributions
Conceptualisation: RC, DZ, and MA. Developing methods: RC, DZ, LO, and FS. Conducting the research: RC, LO, and FS. Data analysis: RC. Preparation of figures and tables: RC. Conducting the research, data interpretation, writing: RC, DZ, MA, LO, and FS. All authors contributed to the article and approved the submitted version.
Funding
This study is part of the Master Thesis of the first author (RC) at the Graduate Program on Aquatic Ecology and Fishing, Universidade Federal do Pará. RC was funded by Coordenação de Aperfeiçoamento de Pessoal de Nível Superior (CAPES) and by UFPA (PROPESP/UFPA #02/2022).
Acknowledgments
The authors thank the colleagues from the Universidade Federal do Oeste do Pará, represented by the Laboratório de Ecologia do Ictioplâncton e Pesca em Águas Interiores (https://leipaiufopa.com/) for the assistance in the collection, sorting and identification of the biological material used in this study.
Conflict of interest
The authors declare that the research was conducted in the absence of any commercial or financial relationships that could be construed as a potential conflict of interest.
Publisher’s note
All claims expressed in this article are solely those of the authors and do not necessarily represent those of their affiliated organizations, or those of the publisher, the editors and the reviewers. Any product that may be evaluated in this article, or claim that may be made by its manufacturer, is not guaranteed or endorsed by the publisher.
Supplementary material
The Supplementary Material for this article can be found online at: https://www.frontiersin.org/articles/10.3389/fenvs.2022.1064170/full#supplementary-material
References
Andrews, S. N., Linnansaari, T., Leblanc, N., Pavey, S. A., and Curry, R. A. (2020). Interannual variation in spawning success of striped bass ( Morone saxatilis ) in the saint john river, new brunswick. River Res. Appl. 36, 13–24. doi:10.1002/rra.3545
Arantes, C. C., Fitzgerald, D. B., Hoeinghaus, D., and Winemiller, K. (2019a). Impacts of hydroelectric dams on fishes and fisheries in tropical rivers through the lens of functional traits. Curr. Opin. Environ. Sustain. 37, 28–40. doi:10.1016/j.cosust.2019.04.009
Arantes, C. C., Winemiller, K. O., Asher, A., Castello, L., Hess, L. L., Petrere, M., et al. (2019b). Floodplain land cover affects biomass distribution of fish functional diversity in the Amazon River. Sci. Rep. 9, 16684. doi:10.1038/s41598-019-52243-0
Arantes, C. C., Winemiller, K., Petrere, M., Castello, L., Hess, L., and Freitas, C. E. de C. (2018). Relationships between forest cover and fish diversity in the Amazon River floodplain. J. Appl. Ecol. 55, 386–395. doi:10.1111/1365-2664.12967
Barthem, R. B., and Fabré, N. N. (2004). “Biologia e diversidade dos recursos pesqueiros da Amazônia,” in A pesca e os recursos pesqueiros na Amazônia brasileira. Editor M. L. Ruffino (Brasília: Ibama), 17–62.
Barthem, R. B., Goulding, M., Leite, R. G., Cañas, C., Forsberg, B., Venticinque, E., et al. (2017). Goliath catfish spawning in the far Western Amazon confirmed by the distribution of mature adults, drifting larvae and migrating juveniles. Sci. Rep. 7, 41784–41813. doi:10.1038/srep41784
Baumgartner, G., Nakatani, K., Gomes, C., Bialetzki, A., Sanches, P. V., and Makrakis, M. C. (2008). Fish larvae from the upper Paraná River: Do abiotic factors affect larval density? Neotrop. Ichthyol. 6, 551–558. doi:10.1590/S1679-62252008000400002
Bayley, P., Castello, L., Batista, V. S., and Fabré, N. N. (2018). Response of Prochilodus nigricans to flood pulse variation in the central Amazon. R. Soc. Open Sci. 5, 172232. doi:10.1098/rsos.172232
Bednarski, J., Miller, S. E., and Scarnecchia, D. L. (2008). Larval fish catches in the lower Milk River, Montana in relation to timing and magnitude of spring discharge. River Res. Appl. 24, 844–851. doi:10.1002/rra.1098
Birindelli, J. L. O., and Akama, A. (2018). “Family Auchenipteridae-driftwood catfishes,” in Field guide to the fishes of the Amazon, orinoco, and guianas. Editors P. van der Sleen, and J. Albert (New Jersey: Princeton University Press), 465.
Bittencourt, S. C. da S., Zacardi, D. M., Monteiro, T., Nakayama, L., and Queiroz, H. L. (2020). Juvenis de peixes associados a macrófitas aquáticas em ambientes de várzea na Amazônia Central, Brasil. Biota Amaz. 10, 43–52. doi:10.18561/2179-5746/biotaamazonia.v10n2p38-45
Cajado, R. A., de Oliveira, L. S., da Silva, F. K. S., and Zacardi, D. M. (2020). Ontogenetic structure and distribution patterns of ichthyoplankton in the confluence zone of two river systems in the Eastern Amazon. J. Appl. Ichthyol. 36, 801–810. doi:10.1111/jai.14111
Cajado, R. A., Oliveira, L. S. de, Oliveira, C. C., Ponte, S. C. S., Bittencourt, S. C. da S., Queiroz, H. L. de, et al. (2018). Distribuição de larvas de Brycon amazonicus (PISCES: BRYCONIDAE) no entorno da reserva de Desenvolvimento sustentável mamirauá: Base ecológica para manejo. Rev. Ibero-Americana Ciências Ambient. 9, 78–87. doi:10.6008/cbpc2179-6858.2018.006.0010
Camacho Guerreiro, A. I., Amadio, S. A., Fabré, N. N., and da Silva Batista, V. (2021). Exploring the effect of strong hydrological droughts and floods on populational parameters of Semaprochilodus insignis (Actinopterygii: Prochilodontidae) from the Central Amazonia. Environ. Dev. Sustain. 23, 3338–3348. doi:10.1007/s10668-020-00721-1
Cañas, C., and Waylen, P. (2012). Modelling production of migratory catfish larvae (Pimelodidae) on the basis of regional hydro- Climatology features of the Madre de Dios Basin in southeastern Peru. Hydrol. Process. 26, 996–1007. doi:10.1002/hyp.8192
Carvalho, A. R., Mingante, H. F. S., and Tornisielo, V. L. (2000). Relações da atividade agropecuária com parâmetros físicos químicos da água. Quim. Nova 23, 618–622. doi:10.1590/s0100-40422000000500009
Castello, L., Bayley, P., Fabré, N. N., and Batista, V. S. (2019). Flooding effects on abundance of an exploited, long-lived fish population in river-floodplains of the Amazon. Rev. Fish. Biol. Fish. 29, 487–500. doi:10.1007/s11160-019-09559-x
Cataldo, D., Leites, V., Bordet, F., and Paolucci, E. (2022). Effects of El Niño-Southern Oscillation (ENSO) on the reproduction of migratory fishes in a large South American reservoir. Hydrobiologia 849, 3259–3274. doi:10.1007/s10750-022-04941-6
Chea, R., Pool, T. K., Chevalier, M., Ngor, P., So, N., Winemiller, K. O., et al. (2020). Impact of seasonal hydrological variation on tropical fish assemblages: Abrupt shift following an extreme flood event. Ecosphere 11, 3303. doi:10.1002/ecs2.3303
Chessman, B. C. (2013). Identifying species at risk from climate change: Traits predict the drought vulnerability of freshwater fishes. Biol. Conserv. 160, 40–49. doi:10.1016/j.biocon.2012.12.032
Costa, A. C. L., Junior, J. de A. S., Cunha, A. C. da, Feitosa, J. R. P., Portela, B. T. T., Silva, G. G. C., et al. (2013). Revista Brasileira de Geografia Física Índices de conforto térmico e suas variações sazonais em cidades de diferentes dimensões na Região Amazônica. Rev. Bras. Geogr. Física 6, 478–487. doi:10.26848/rbgf.v6.3.p478-487
Duarte, C., Espírito-Santo, H. M. V., Zuanon, J., Rapp Py-Daniel, L. H., and Deus, C. P. (2020). What happens in the darkness? Seasonal variations in tropical benthic fish assemblages. Mar. Freshw. Res. 71, 419–431. doi:10.1071/MF19038
Duarte, C., Farago, T. L. B., dos Anjos, C. S., dos Santos, N. R., do Nascimento, L. M., Cella-Ribeiro, A., et al. (2022). Spatial and seasonal variation of benthic fish assemblages in whitewater rivers of Central Amazon. Biota Neotrop. 22. doi:10.1590/1676-0611-bn-2021-1312
Espinoza, J. C., Sörensson, A. A., Ronchail, J., Molina-Carpio, J., Segura, H., Gutierrez-Cori, O., et al. (2019). Regional hydro-climatic changes in the southern Amazon basin (upper madeira basin) during the 1982–2017 period. J. Hydrology Regional Stud. 26, 100637. doi:10.1016/j.ejrh.2019.100637
Esteves, F. A. (1998). in Fundamentos de Limnologia. Editor F. de A. Esteves. 2nd ed. (Rio de Janeiro: Interciência).
Filizola, N., and Guyot, J. L. (2011). Fluxo de sedimentos em suspensão nos rios da Amazônia. Rev. Bras. Geociências 41, 566–576. doi:10.25249/0375-7536.2011414566576
Frederico, R. G., Dias, M. S., Jézéquel, C., Tedesco, P. A., Hugueny, B., Zuanon, J., et al. (2021). The representativeness of protected areas for Amazonian fish diversity under climate change. Aquat. Conserv. 31, 1158–1166. doi:10.1002/aqc.3528
Freitas, C. E. C., Siqueira-Souza, F. K., Humston, R., and Hurd, L. E. (2013). An initial assessment of drought sensitivity in Amazonian fish communities. Hydrobiologia 705, 159–171. doi:10.1007/s10750-012-1394-4
Freitas, T. M. S., Almeida, V. H. D. C., Valente, R. de M., and Montag, L. A. F. (2011). Feeding ecology of Auchenipterichthys longimanus (Siluriformes: Auchenipteridae) in a riparian flooded forest of eastern amazonia, Brazil. Neotrop. Ichthyol. 9, 629–636. doi:10.1590/S1679-62252011005000032
Fricke, R., Eschmeyer, W. N., and van der Laan, R. (2022). Eschmeyer’s catalog of fishes: Genera species. references [Internet]. 2022. Available at: https://researcharchive.calacademy.org/research/ichthyology/catalog/fishcatmain.asp.
Galvis, G., Mojica, J. I., Duque, S., Castellanos, C., Sánchez-Duarte, P., Arce, M., et al. (2006). “Peces del medio Amazonas. Región de Leticia. Serie de Guías Tropicales de Campo,”. Editor R. D. C. Mittermeier and W. Konstant Bogotá. 5th ed. (Colombia: Editorial Panamericana).
García-Dávila, C. R., Riveiro, H., Flores, M. A., Mejia de Loayza, J. E., Angulo, C. A. C., Castro, D., et al. (2018). Peces de consumo de la amazonía peruana. Available at: http://repositorio.iiap.gob.pe/handle/IIAP/368.
Gogola, T. M., Piana, P. A., da Silva, P. R. L., Topan, D. A., and Sanches, P. V. (2021). Fish reproductive activity reveals temporal variations predominating spatial heterogeneity in maintaining high functional diversity of a Neotropical reservoir. Ecol. Freshw. Fish. 31, 154–163. doi:10.1111/eff.12621
Grenouillet, G., and Pont, D. (2001). Juvenile fishes in macrophyte beds: Influence of food resources, habitat structure and body size. J. Fish. Biol. 59, 939–959. doi:10.1111/j.1095-8649.2001.tb00163.x
Grzybkowska, M., Dukowska, M., Leszczyńska, J., Lik, J., Szczerkowska-Majchrzak, E., and Przybylski, M. (2018). The food resources exploitation by small-sized fish in a riverine macrophyte habitat. Ecol. Indic. 90, 206–214. doi:10.1016/j.ecolind.2018.02.021
Hermann, T. W., Stewart, D. J., Barriga Salazar, R. E., and Coghlan, S. M. (2021). Spatial and temporal patterns of pelagic catfish larvae drifting in lowland rivers of eastern Ecuador (pisces: Siluriformes). Ichthyol. Herpetol. 109, 978–990. doi:10.1643/i2020019
Humphries, P., King, A., McCasker, N., Kopf, R. K., Stoffels, R., Zampatti, B., et al. (2020). Riverscape recruitment: A conceptual synthesis of drivers of fish recruitment in rivers. Can. J. Fish. Aquat. Sci. 77, 213–225. doi:10.1139/cjfas-2018-0138
Imbiriba, L. C., Silva, Y. K. C., Serrão, E. M., and Zacardi, D. M. (2020). Ictiofauna acompanhante associada a pesca do camarão-da-amazônia Macrobrachium amazonicum (heller, 1862) (Decapoda, palaeomonidae): Subsídios para gestão ambiental e ordenamento da pesca. G. Sci. 14, 52–73. doi:10.22478/ufpb.1981-1268.2020v14n4.52766
Isaac, V. J., Castello, L., Santos, P. R. B., and Ruffino, M. L. (2016). Seasonal and interannual dynamics of river-floodplain multispecies fisheries in relation to flood pulses in the Lower Amazon. Fish. Res. 183, 352–359. doi:10.1016/j.fishres.2016.06.017
Junk, W. J., Piedade, M. T. F., Schöngart, J., Cohn-Haft, M., Adeney, J. M., and Wittmann, F. (2011). A classification of major naturally-occurring amazonian lowland wetlands. Wetlands 31, 623–640. doi:10.1007/s13157-011-0190-7
King, A. J., Tonkin, Z., and Mahoney, J. (2009). Environmental flow enhances native fish spawning and recruitment in the Murray River, Australia. River Res. Appl. 25, 1205–1218. doi:10.1002/rra.1209
Legendre, P., and Borcard, D. (2018). Box–Cox-chord transformations for community composition data prior to beta diversity analysis. Ecography 41, 1820–1824. doi:10.1111/ecog.03498
Magalhães, E. R. S., Yamamoto, K., Beltão, H., and Loebens, S. C. (2015). Bancos de macrófitas aquáticas em lago de várzea: Alimentação de duas espécies de peixes na região de Manaus, amazonas, brasil. Acta Pesca 3, 25–40. doi:10.2312/ActaFish.2015.3.1.25-40
Marengo, J. A., Liebmann, B., Grimm, A. M., Misra, V., Silva Dias, P. L., Cavalcanti, I. F. A., et al. (2012). Recent developments on the South American monsoon system. Int. J. Climatol. 32, 1–21. doi:10.1002/joc.2254
Mariac, C., Id, O., Vigouroux, Y., Id, O., Nu, J., Rodriguez, E. Z., et al. (2022). Species-level ichthyoplankton dynamics for 97 fishes in two major river basins of the Amazon using quantitative metabarcoding. Mol. Ecol. 31, 1627–1648. doi:10.1111/mec.15944
Marschall, E. A., Glover, D. C., Mather, M. E., and Parrish, D. L. (2021). Modeling larval American shad recruitment in a large river. N. Am. J. Fish. Manag. 41, 939–954. doi:10.1002/nafm.10460
Mohammadi, B., Vaheddoost, B., and Danandeh Mehr, A. (2020). A spatiotemporal teleconnection study between Peruvian precipitation and oceanic oscillations. Quat. Int. 565, 1–11. doi:10.1016/j.quaint.2020.09.042
Montenegro, A. K. A., Cardoso, M. M. L., Silva, M. C. B. C. da, and Tataje, D. A. R. (2020). Role of the rain and macrophytes on temporal and spatial pattern of ichthyoplankton in the Caatinga Biome, Brazil/Papel da chuva e das macrófitas no padrão temporal e espacial do ictioplâncton no Bioma Caatinga, Brasil. Braz. J. Animal Environ. Res. 3, 3963–3980. doi:10.34188/bjaerv3n4-094
Nakatani, K., Agostinho, A. A., Baumgartner, G., Bialetzki, A., Sanches, P. V., Makrakis, M. C., et al. (2001). Ovos e larvas de peixes de água doce: Desenvolvimento e manual de identificação. Mar. Eduem 1, 378.
Nunn, A. D., Tewson, L. H., and Cowx, I. G. (2012). The foraging ecology of larval and juvenile fishes. Rev. Fish. Biol. Fish. 22, 377–408. doi:10.1007/s11160-011-9240-8
Oksanen, A. J., Blanchet, F. G., Kindt, R., Legen-, P., Minchin, P. R., Hara, R. B. O., et al. (2018). Community ecology package. Ecol. Package, 263.
Oliveira, A. G., Suzuki, H. I., Gomes, L. C., and Agostinho, A. A. (2014). Interspecific variation in migratory fish recruitment in the Upper Paraná River: Effects of the duration and timing of floods. Environ. Biol. Fishes 98, 1327–1337. doi:10.1007/s10641-014-0361-5
Oliveira, L. S., Cajado, R. A., Santos, L. R. B., Suzuki, M. A. de L., and Zacardi, D. M. (2020). Bancos de macrófitas aquáticas como locais de desenvolvimento das fases iniciais de peixes em várzea do Baixo Amazonas. Oecol. Aust. 24, 644–660. doi:10.4257/oeco.2020.2403.09
Pereira, D. V., Silva, L. F. S., and Sousa, K. N. S. (2019). Distribuição espacial dos sítios de captura registrados nos polos de desembarque pesqueiro no município de Santarém (Pará – brasil). Biota Amaz. 9, 43–47. doi:10.18561/2179-5746/biotaamazonia.v9n1p43-47
Piedade, M. T. F., Lopes, A., Demarchi, O. L., Cruz, J., and Junk, W. J. (2020). “Os campos naturais e as plantas herbáceas na planície de inundação amazônica e sua utilização,” in Várzeas amazônicas: Desafios para um manejo sustentável. Editors W. J. Junk, M. T. F. Piedade, F. Wittmann, and J. Schöngart (India: NPA), 86–105.
Pinaya, W. H. D., Lobon-Cervia, F. J., Pita, P., Buss de Souza, R., Freire, J., and Isaac, V. J. (2016). Multispecies fisheries in the lower Amazon River and its relationship with the regional and global climate variability. PLoS One 11, e0157050. doi:10.1371/journal.pone.0157050
Reboita, M. S., Oliveira, K. R., Corrêa, P. Y. C., and Rodrigues, R. (2021). Influência dos Diferentes Tipos do Fenômeno El Niño na Precipitação da América do Sul. Available at: https://periodicos.ufpe.br/revistas/rbgfe.
Rocha, N. S., Veettil, B. K., Grondona, A., and Rolim, S. (2019). The influence of ENSO and PDO on tropical Andean glaciers and their impact on the hydrology of the Amazon Basin. Singap. J. Trop. Geogr. 40, 346–360. doi:10.1111/sjtg.12290
Rodríguez-Morata, C., Díaz, H. F., Ballesteros-Canovas, J. A., Rohrer, M., and Stoffel, M. (2019). The anomalous 2017 coastal El Niño event in Peru. Clim. Dyn. 52, 5605–5622. doi:10.1007/s00382-018-4466-y
Röpke, C. P., Amadio, S., Zuanon, J., Ferreira, E. J. G., de Deus, C. P., Pires, T. H. S., et al. (2017). Simultaneous abrupt shifts in hydrology and fish assemblage structure in a floodplain lake in the central Amazon. Sci. Rep. 7, 40170. doi:10.1038/srep40170
Röpke, C., Pires, T. H. S., Zuchi, N., Zuanon, J., and Amadio, S. (2022). Effects of climate-driven hydrological changes in the reproduction of Amazonian floodplain fishes. J. Appl. Ecol. 59, 1134–1145. doi:10.1111/1365-2664.14126
Rosado, B., Dias, A., and Mattos, E. (2013). Going back to basics: Importance of ecophysiology when choosing functional traits for studying communities and ecosystems. NatCon. 11, 15–22. doi:10.4322/natcon.2013.002
Sánchez-Botero, J., Leitão, R. P., Caramaschi, É. P., and Sequeira Garcez, D. (2007). The aquatic macrophytes as refuge, nursery and feeding habitats forfresh water fish from Cabiúnas Lagoon, Restinga de Jurubatiba National Park, Rio de Janeiro, Brazil. Acta Limnol. Bras. 19, 143–153.
Santos, G. M., Ferreira, E. J. G., and Zuanon, J. A. S. (2006). Peixes comerciais de Manaus. Karnataka: Edições Ibama.
Shuai, F., Li, X., Li, Y., Li, J., Yang, J., and Lek, S. (2016). Temporal patterns of larval fish occurrence in a large subtropical river. PLoS One 11, 01464411–e146520. doi:10.1371/journal.pone.0146441
Silva, A. E. P., Angelis, C. F., Machado, L. A. T., and Waichaman, A. V. (2008). Influência da precipitação na qualidade da água do Rio Purus. Acta Amaz. 38, 733–742. doi:10.1590/s0044-59672008000400017
Silva, P. B., Arantes, C. C., Freitas, C. E., Petrere, M., and Ribeiro, F. R. V. (2020). Seasonal hydrology and fish assemblage structure in the floodplain of the lower Amazon River. Ecol. Freshw. Fish. 00, 162–173. doi:10.1111/eff.12572
Stoffers, T., Buijse, A. D., Geerling, G. W., Jans, L. H., Schoor, M. M., Poos, J. J., et al. (2022). Freshwater fish biodiversity restoration in floodplain rivers requires connectivity and habitat heterogeneity at multiple spatial scales. Sci. Total Environ. 838, 156509. doi:10.1016/j.scitotenv.2022.156509
Tondato, K. K., Aparecida De Fátima Mateus, L., and Ziober, S. R. (2010). Spatial and temporal distribution of fish larvae in marginal lagoons of Pantanal, Mato Grosso State, Brazil. Neotrop. Ichthyol. 8, 123–134. doi:10.1590/S1679-62252010005000002
Towner, J., Ficchí, A., Cloke, H. L., Bazo, J., Coughlan De Perez, E., and Stephens, E. M. (2021). Influence of ENSO and tropical Atlantic climate variability on flood characteristics in the Amazon basin. Hydrol. Earth Syst. Sci. 25, 3875–3895. doi:10.5194/hess-25-3875-2021
Tyler, K. J., Wedd, D., Crook, D. A., Kennard, M. J., and King, A. J. (2021). Hydrology drives variation in spawning phenologies and diversity of larval assemblages of Australian wet–dry tropical fish. Freshw. Biol. 66, 1949–1967. doi:10.1111/fwb.13802
Vauchel, P., Santini, W., Guyot, J. L., Moquet, J. S., Martinez, J. M., Espinoza, J. C., et al. (2017). A reassessment of the suspended sediment load in the Madeira River basin from the Andes of Peru and Bolivia to the Amazon River in Brazil, based on 10 years of data from the HYBAM monitoring programme. J. Hydrol. X. 553, 35–48. doi:10.1016/j.jhydrol.2017.07.018
Ward, P. J., Jongman, B., Kummu, M., Dettinger, M. D., Weiland, F. C. S., and Winsemius, H. C. (2014). Strong influence of El Niño Southern Oscillation on flood risk around the world. Proc. Natl. Acad. Sci. U. S. A. 111, 15659–15664. doi:10.1073/pnas.1409822111
Warton, D. I., Foster, S. D., De’ath, G., Stoklosa, J., and Dunstan, P. K. (2015). Model-based thinking for community ecology. Plant Ecol. 216, 669–682. doi:10.1007/s11258-014-0366-3
Winemiller, K., Fitzgerald, D. B., Bower, L., and Pianka, E. (2015). Functional traits, convergent evolution, and periodic tables of niches. Ecol. Lett. 18, 737–751. doi:10.1111/ele.12462
Winemiller, K. O., and Rose, K. A. (1992). Patterns of life-history diversification in north American fishes: Implications for population regulation. Can. J. Fish. Aquat. Sci. 49, 2196–2218. doi:10.1139/f92-242
Zacardi, D. M., Bittencourt, S. C. da S., Nakayama, L., and Queiroz, H. L. (2017a). Distribution of economically important fish larvae (Characiformes, prochilodontidae) in the central amazonia, Brazil. Fish. Manag. Ecol. 24, 283–291. doi:10.1111/fme.12222
Zacardi, D. M., Bittencourt, S. C. da S., and Queiroz, H. L. (2020). Recruitment of migratory Characiforms in the different wetland habitats of Central Amazonia: Subsidies for sustainable fisheries management. J. Appl. Ichthyol. 36, 431–438. doi:10.1111/jai.14040
Zacardi, D. M., Ponte, S. C. S., Chaves, C. S., Oliveira, L. S., and Cajado, R. A. (2018). Interannual variation at the recruitment of larval of Mylossoma (characidae; Characiformes) in lower Amazon, Pará. Acta Fish. Aquatic Resour. 6, 17–28. doi:10.2312/Actafish.2018.6.1.17-28
Zacardi, D. M., Ponte, S. C. S., Ferreira, L. C., Silva, M. A. S., Silva, S. J. Á., and Chaves, C. S. (2017b). Diversity and spatio-temporal distribution of the ichthyoplankton in the lower Amazon River , Brazil. Biota Amaz. 7, 12–20. doi:10.18561/2179-5746/biotaamazonia.v7n2p12-20
Zacardi, D. M., and Ponte, S. C. S. (2021). Seasonality determines patterns of composition and abundance of ichtyoplankton in Maiká lake, Eastern Amazon. Panam. J. Aquat. Sci. 16, 37–51.
Keywords: El Niño Southern Oscillation, reproductive dynamics, ichthyoplankton, functional diversity, Lower Amazon
Citation: Cajado RA, Oliveira LS, Silva FKS, Zacardi DM and Andrade MC (2022) Effects of anomalous climatic events on the structure of fish larvae assemblages in the eastern Amazon. Front. Environ. Sci. 10:1064170. doi: 10.3389/fenvs.2022.1064170
Received: 07 October 2022; Accepted: 21 November 2022;
Published: 08 December 2022.
Edited by:
Josie South, University of Leeds, United KingdomReviewed by:
Carlos Rodrigues-Filho, Minas Gerais State University, BrazilSeiji Miyazono, Yamaguchi University, Japan
Ali Serhan Tarkan, Muğla University, Turkey
Copyright © 2022 Cajado, Oliveira, Silva, Zacardi and Andrade. This is an open-access article distributed under the terms of the Creative Commons Attribution License (CC BY). The use, distribution or reproduction in other forums is permitted, provided the original author(s) and the copyright owner(s) are credited and that the original publication in this journal is cited, in accordance with accepted academic practice. No use, distribution or reproduction is permitted which does not comply with these terms.
*Correspondence: Ruineris Almada Cajado, ruineris.cajado@gmail.com