- 1Tropical Crops Genetic Resources Institute, Chinese Academy of Tropical Agricultural Sciences, Haikou, China
- 2College of Tropical Crops, Hainan University, Haikou, China
- 3College of Forestry, Hainan University, Haikou, China
Green manure intercropping is an agronomic management practice that effectively increases soil nutrients in understory and reduces weed population. However, the influence of different green manures on soil properties and soil bacterial community in litchi orchards in the tropical regions of China remains largely unknown. Here, we examined the effect of intercropping three leguminous green manure crops in litchi orchards of Hainan Province. No intercropping was used as the control. Different green manures increased the contents of different soil nutrients. For example, Desmodium ovalifolium increased the total nitrogen content by 7.93%; Grona heterocarpos increased the ammonium nitrogen content by 558.85%; and Stylosanthes guianensis increased the available phosphorus content by 1207.34%. However, intercropping with D. ovalifoliu and S. guianensis reduced the content of available potassium by 47.29% and 58.48%, respectively. The intercropping of green manure increased the abundance of several microbial genera, including Bradyrhizobium, Serratia, and Bacillus, which are known to facilitate soil nitrogen accumulation, plant growth, and phosphorus dissolution. Compared with no intercropping, the three intercropping treatments significantly improved the contents of soil ammonium nitrogen and soil available phosphorus in the litchi orchard. Therefore, intercropping with green manure crops in litchi orchards is an effective management measure; however, the choice of the green manure crop should be based on the soil conditions of litchi orchards.
Introduction
Legumes fix atmospheric nitrogen N) and are planted as green manure crops for improving ecosystem efficiency, especially in chronically N-deficient cropping systems (Zahran, 1999; Wang et al., 2022). Intercropping with green manure crops increases land productivity while decreasing N supplementation (Xu et al., 2020). Litchi (Litchi chinensis Sonn.) is a traditional fruit native to South China (Li et al., 2020; Jiang et al., 2021). Litchi fruit has an appealing colour, a refreshing flavour, and high nutritive and market value. Orchard intercropping plays an important role in improving soil quality and environmental sustainability and reducing agricultural input (Abdalla et al., 2019). Studies showed that intercropping with green manure can increase soil organic matter (SOM) and N use efficiency, reduce soil erosion, inhibit weed growth, and improve soil microbial diversity (Vicente-Vicente et al., 2016; Vukicevich et al., 2016).
Soil microorganisms stabilize the rhizosphere structure, directly regulate the nutrients acquisition ability of plants and the nutrient cycle in the soil, and are a key component of the soil ecosystem (Bunemann et al., 2018; Zhao et al., 2022). Studying the abundance of different functionally diverse soil microbial populations is an effective means to understanding the effect of intercropping on soil properties. For example, legume intercropping increases the availability various forms of nitrogen, which can be assessed by analyzing the abundance of relevant microorganisms (Abdalla et al., 2019).
Most of the studies conducted to date on intercropping have focused on crop yield and soil properties (Ma et al., 2017; Maitra et al., 2021). Previous studies showed that green manure intercropping in orchards significantly improved soil nutrients and bacteria. However, intercropping green manure in litchi orchards was rarely reported in tropical, and more excellent green manure varieties were needed. In this study, intercropping three legumes (D. ovalifolium, G. heterocarpos, or S. guianensis) in the litchi orchard, and elucidate effect of intercropping on soil properties and soil bacterial community. The purpose was to select leguminous green manure suitable for intercropping in young litchi orchards, and provide scientific theoretical guidance for future research.
Materials and methods
Plant species
This research planted litchi trees in 2019 with a plant-to-plant spacing of 4 m × 5 m. The variety was “Feizixiao”. The following legumes were used for intercropping: Desmodium ovalifolium Reyan variety No. 16 introduced from Australia in 1998 b y the Chinese Academy of Tropical Agricultural Sciences (CATA), Stylosanthes guianensis Reyan variety No. 18 introduced from the Center of International Agriculture Tropical (CIAT) in 1996 by the CATA, and Grona heterocarpos from the CATA. Green manure crops were planted at a distance of 1.5 m from the base of the litchi stem in April 2021, at a density of nine seedlings per square meter.
Field site description and soil material collection
The layout of the field was shown in Figure 1. This study was conducted at the litchi demonstration orchard station of CATA in Danzhou, Hainan Province, China (109°57′E, 19°53′N). This region is characterized by a tropical monsoon climate, with a yearly sunlight period of 1,781°h, annual average rainfall of 1,823 mm, and yearly average temperature of 23.7°C. At the study site, the maximum and lowest temperatures are 38°C and 7°C, respectively. The location is frost-free throughout the year, and the soil type is red loam. Four treatments were conducted: three intercropping treatments, each with a different green manure crop (D. ovalifolium, G. heterocarpos, or S. guianensis), and one no-intercropping treatment (grower standard; control). All legumes planted in early 2021 and pre-weeded. There was a total of 12 experimental plots, with each treatment performed in triplicate. The details of soil nutrients are summarized in Table 1.
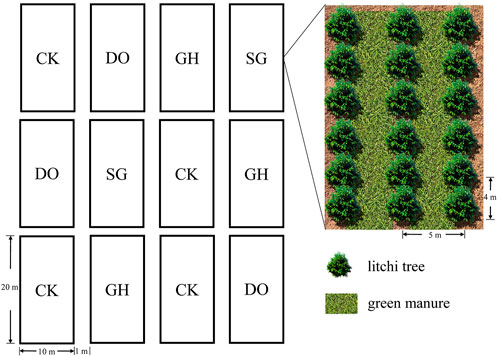
FIGURE 1. The layout of the sample plots for orchard and green manure. CK: litchi orchard with no intercropping; DO: litchi orchard intercropped with Desmodium ovalifolium; GH: litchi orchard intercropped with Grona heterocarpos; SG: litchi orchard intercropped with Stylosanthes guianensis.
In December 2021, soil samples with a depth of 0–20 cm were collected from three plots of each treatment after the removal of impurities on the surface. Soil samples were collected at five random points in each plot and mixed. Subsequently, 12 soil samples were pulverised and passed through a 1 mm mesh. Each of these samples was divided into two portions: one portion was air-dried to determine the soil chemical characteristics; another portion was frozen at −80°C for 16 S rRNA gene analysis.
Soil nutrient contents
Dichromate oxidation was used to determine the SOM content (Gao et al., 2021; Wang T. et al., 2022), and total N was determined using the semi-micro Kjeldahl digestion method (Kachurina et al., 2000). After extraction with 2 mol L−1 KCl (1:10, w/v), the concentrations of soil ammonium nitrogen (NH4+-N) and nitrate nitrogen (NO3−-N) were determined by UV spectrophotometry (Bao, 1999). Soil available potassium (AK) and available phosphorous (AP) were measured using the NH4OAc− flame photometer method and NaHCO3− molybdenum antimony colorimetric method (Bao, 1999), respectively. Soil moisture content and soil pH were measured using the ZD-1608 soil moisture meter and ZD-18 pH meter (ZD INSTRUMENT, Taizhou, China), respectively.
Soil DNA extraction
DNA extraction from soil samples for 16s RNA sequencing to analyze soil bacteral communities. Briefly, total genomic DNA was extracted from soil samples using the cetyl trimethyl ammonium bromide (CTAB) method. Then, monitor the DNA concentration and purity on 1% agarose gels. The V3-V4 region of the 16 S ribosomal RNA (rRNA) genes were amplified using the 341 F (5′-CCTAYGGGRBGCASCAG-3′) and 806R (5′-GGACTCNNGGGTATCAAT-3′) primers with the barcode. The PCR amplification products were electrophoresed on a 2.0% agarose gel after being mixed with an equal volume of 1X TAE buffer. Then, QiagenGel Extraction Kit (Qiagen, Germany) was used to purify PCR products, and used to construct the 16 S rDNA library.
Statistical analysis
PCR products were sequenced utilizing Illumina’s paired-end sequencing technology (Wekemo Tech Group Corp. Shenzhen, China). Briefly, FASTQ files containing raw data were converted to a QIIME2 compatible format by the qiime tool and process each sample. The amplicon sequence variant (ASV) feature table and taxonomy table were then generated in reverse using the QIIME2 plugin, and any contaminating mitochondrial and chloroplast DNA sequences (Callahan et al., 2016; Bokulich et al., 2018).
SPSS (v. 26) statistical software was used to conduct an analysis of variance on the relative abundances of different bacterial genera and soil properties, and significant level was set at p < .05. Linear discriminant analysis Effect Size (LEfSe), Kruskal Wallis test, and Analysis of variance (ANOVA) were employed to identify bacterial genera showed differences in abundance among samples and treatments (Segata et al., 2011; Love et al., 2014; Mandal et al., 2015). The default score screening value for Linear Discriminant Analysis effect size is LDA >4. Alpha diversity, including the Shannon index, Chao1 index, Faith’s phylogenetics diversity index, and Observed OTUs was calculated using the QIIME2 software based on the obtained ASV. Redundancy analysis (RDA) was computed with R software (vegan package).
Results
Soil nutrient contents
Table 2 showed the contents of soil nutrients in different treatments. Intercropping with S. guianensis, D. ovalifolium, and G. heterocarpos in the litchi orchard seemed to have no significant effect on soil NO3 −-N as well as pH. The SOM content of S. guianensis, D. ovalifolium, and G. heterocarpos intercropping treatments was 16.55%, 22.30%, and 10.79% lower than that of the no-intercropping (control) treatment, respectively (p < .05). Compared to the other treatments, the D. ovalifolium treatment significantly more TN content (p < .05). The G. heterocarpos treatment significantly more NH4+-N content than the other treatments and was 558.85% higher than the control (p < .05). The S. guianensis treatment significantly more available phosphorous (AP) content than the other treatments, and 1,207.34% more than the control (p < .05). The no-intercropping and G. heterocarpos treatments had significantly higher AK contents than the other treatments (p < .05). The D. ovalifolium treatment increased soil water content by 11.73% compared to the control (p < .05). The S. guianensis treatment increased aboveground biomass compared to the other treatments (p < .05).
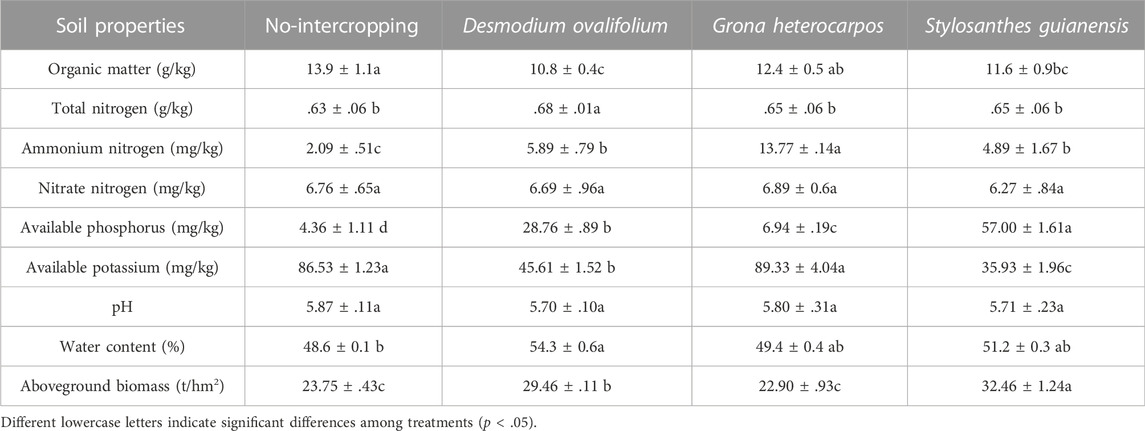
TABLE 2. Chemical properties and nutrient contents of soil in litchi orchard grown without intercropping or with Stylosanthes guianensis, Desmodium ovalifolium, and Grona heterocarpos intercropping.
Soil bacterial diversity and richness
A total of 9,544 Operational taxonomic units (OTUs) were obtained from all samples. Venn diagram showed that the D. ovalifolium treatment contained the highest number of bacterial OTUs (3,765), followed by the no-intercropping (3,623), G. heterocarpos (3,427), and S. guianensis treatments (3,067). A total of 699 OTUs were common to all treatments, and 1,978, 1,794, 1,827, and 1,475 OTUs were exclusive to the no-intercropping, S. guianensis, D. ovalifolium, and G. heterocarpos treatments, respectively (Figure 2).
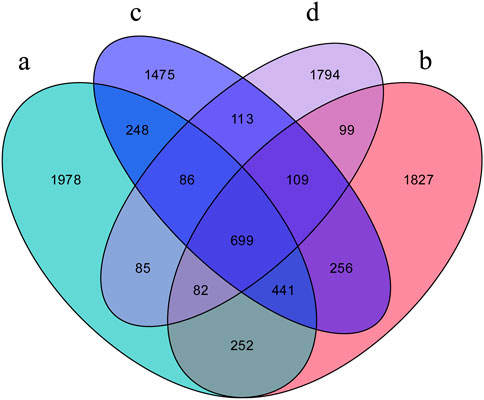
FIGURE 2. Number of observed taxonomic units (OTUs) in the soil. (A) litchi orchard with no intercropping; (B) litchi orchard intercropped with Desmodium ovalifolium; (C) litchi orchard intercropped with Grona heterocarpos; (D) litchi orchard intercropped with Stylosanthes guianensis.
Shannon and Simpson indices showed that soil bacterial diversity was significantly lower in the S. guianensis treatment than in the no-intercropping control (p > .05) (Figure 3). This result was confirmed by the Chao1 richness estimator and Observed OTUs index (Figure 3). On the other hand, D. ovalifolium and G. heterocarpos had no significant influence on bacterial richness compared with the no-intercropping control (Figure 3).
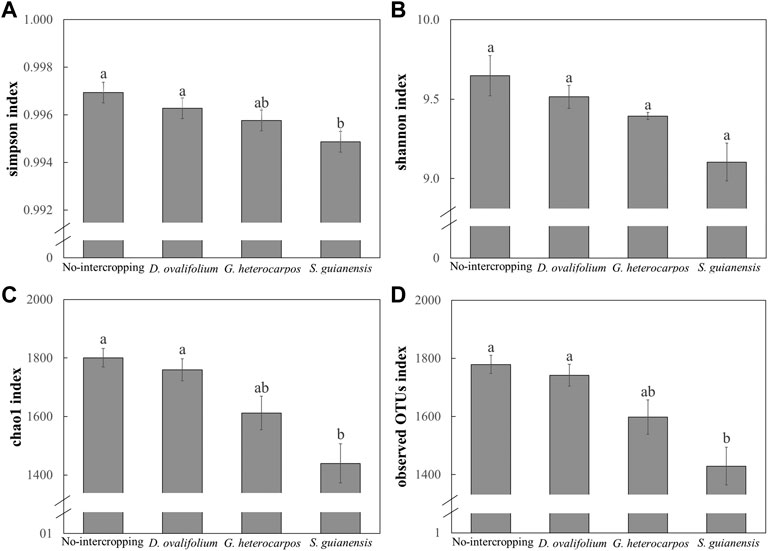
FIGURE 3. Soil bacterial diversity and richness in litchi orchards intercropped with Desmodium ovalifolium, Grona heterocarpos, and Stylosanthes guianensis. Different lowercase letters in the figure indicate significant differences between treatments (p < 0.05). (A): Simpson index; (B): Shannon index; (C): Chao1 index; (D): Observed OTUs index.
Soil bacterial community
Bacterial community composition in the no-intercropping and green manure treatments was compared at different classification levels (phylum, class, and genus). At the phylum level, Acidobacteriota, Chloroflexi, Proteobacteria, and Firmicutes were the four most abundant soil bacteria across all four treatments that were Acidobacteriota, Chloroflexi and Proteobacteria than 1%. The relative abundance of subdominant soil bacterial phylum level (Actinobacteriota, Crenarchaeota, Bacteroidota, Verrucomicrobiota, GAL15, Myxococcota) was greater than 1% in the four treatments. Acidobacteriota (relative abundance = 32.22–41.38%) was the most abundant phylum in four treatments, followed by Chloroflexi (relative abundance = 18.05% and 19.83% in D. ovalifolium and G. heterocarpos treatments, respectively), and Proteobacteria (relative abundance = 19.08% and 17.84% in no-intercropping and D. ovalifolium treatments, respectively). Except Acidobacteriota, Chloroflexi, and Firmicutes, the bacterial phyla did not differ significantly between treatments; the relative abundance of Acidobacteriota was lowest in the S. guianensis treatment (32.22%), compared to the other three treatments (relative abundance = 41.39%, 40.16%, and 40.26% in D. ovalifolium, G. heterocarpos treatments, and no-intercropping, respectively), while that of Chloroflexi (relative abundance = 18.05%, 19.83%, 11.86% and 16.57% in D. ovalifolium, G. heterocarpos, S. guianensis treatments, and no-intercropping, respectively) in D. ovalifolium and G. heterocarpos treatments and of Firmicutes (relative abundance = .82%, .61%, .66% and 16.61% in D. ovalifolium, G. heterocarpos, S. guianensis treatments, and no-intercropping, respectively) were more abundant in the S. guianensis treatment than that in the remaining treatments (Figure 4A).
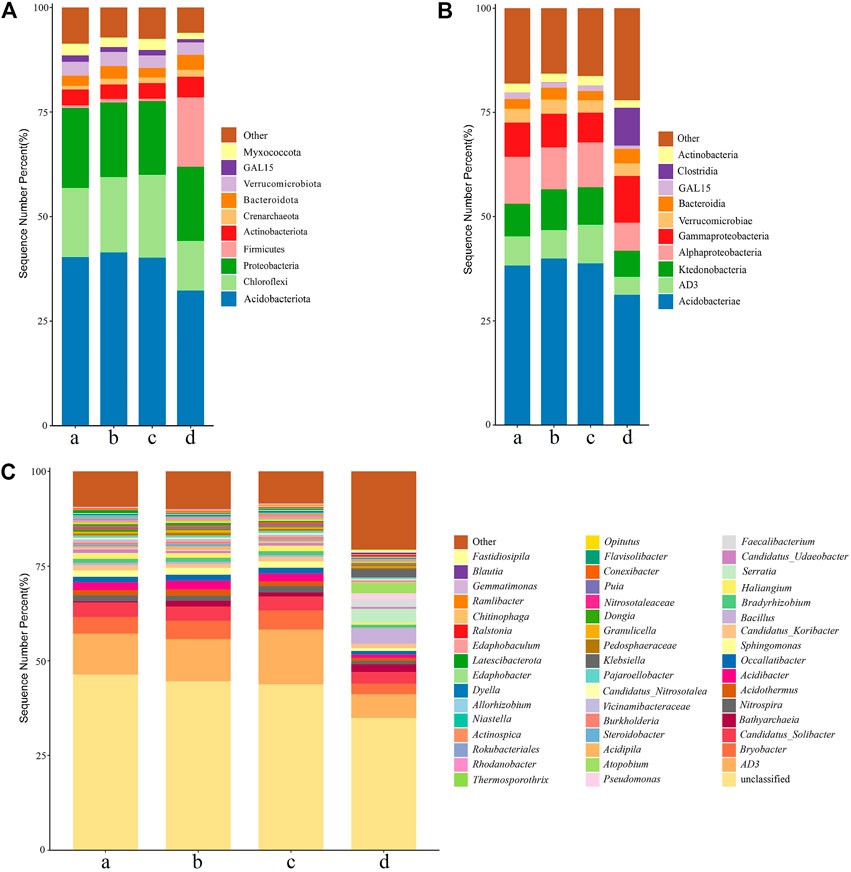
FIGURE 4. Soil bacterial community composition in different treatments at the phylum level. (A) Top 10 relatively abundant bacterial of the treatments at the class level (B) Top fifty relatively abundant bacterial of the treatments at the genus level (C). (A) litchi orchard with no intercropping; (B) litchi orchard intercropped with Desmodium ovalifolium; (C) litchi orchard intercropped with Grona heterocarpos; (D) litchi orchard intercropped with Stylosanthes guianensis.
At the class level, Acidobacteriae, AD3, Ktedonobacteria, Alphaproteobacteria, and Gammaproteobacteria were frequently found in four treatments, with relative abundance exceeding 1% (Figure 4B). Verrucomicrobiae, Bacteroidia, GAL15, Clostridia, and Actinobacteria were the top four subdominant bacterial classes. The top four soil bacterial classes in the four treatments were Acidobacteriae (31.26%–39.90%), AD3 (4.32%–9.32%), Ktedonobacteria (6.23%–9.80%), and Alphaproteobacteria (6.69%–11.23%), and the relative abundance of each of these classes in the S. guianensis treatment was decreased compared to other treatments. The relative abundance of Clostridia (.03%–9.08%) was decreased in D. ovalifolium and G. heterocarpos treatments than in the S. guianensis treatment.
Among the fifty most abundant bacterial genera, AD3 (5.57%–12.86%), Acidobacterium (2.08%–4.79%), and Bryobacter (2.47%–4.47%) were the most abundant (Figure 4C). Nitrospira, Acidothermus, Acidibacter, Occallatibacter, and Sphingomonas were also found at similarly high abundance in the no-intercropping, D. ovalifolium, and G. heterocarpos treatments. Bathyarchaeia, Candidatus Koribacter, Bacillus, Serratia, and Faecalibacterium showed similar abundance in the S. guianensis treatment.
Species diversity among treatments
The results of LEfSe revealed significant differences in the abundance of 20 bacterial species among all treatments. The most characteristic microorganisms were found in S. guianensis treatments, but the least in no intercropping (Figure 5). Different bacterial species showed dominance in different intercropping treatments: Acidobacterium in the D. ovalifolium treatment, AD3 in the G. heterocarpos treatment, and Serratia, Christensenellaceae, Atopobium, and Faecalibacterium in the S. guianensis treatment.
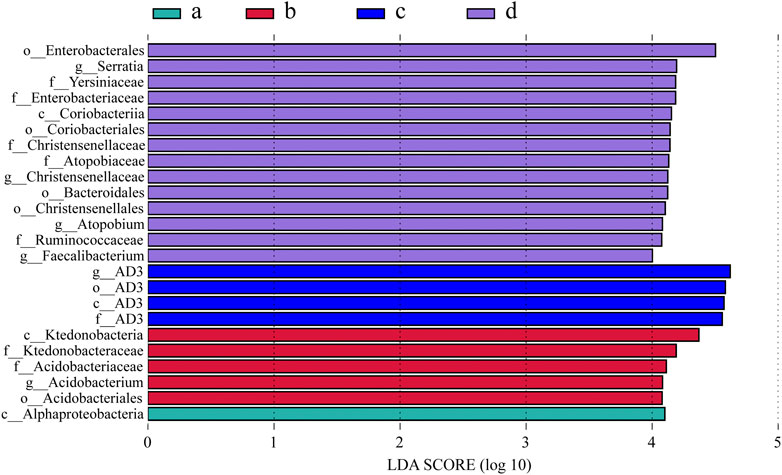
FIGURE 5. Linear discriminant analysis (LDA) score distribution histogram. (A) litchi orchard with no intercropping; (B) litchi orchard intercropped with Desmodium ovalifolium; (C) litchi orchard intercropped with Grona heterocarpos; (D) litchi orchard intercropped with Stylosanthes guianensis.
To clarify the differences in species abundance among treatments at the genus level, the top 15 genera significant differences were displayed. (Figure 6). Compared with the no-intercropping control, green manure reduced the relative abundance of Acidothermus by 15.13%–52.87% (p < .05); D. ovalifolium and G. heterocarpos increased the relative abundance of Bradyrhizobium (p < .05); D. ovalifolium increased the relative abundance of Acidobacterium, Bathyarchaeia, and Acidipila by 142.9%, 468.4%, and 172.4%, respectively (p < .05); G. heterocarpos increased the relative abundance of AD3 by 135.2% (p < .05); S. guianensis increased the relative abundance of Bacillus, Serratia, Faecalibacterium, Atopobium, and Christensenellaceae (p < .05), and reduced the relative abundance of AD3, Acidobacterium, Nitrospira, Acidothermus, Acidibacter, Haliangium, Acidipila, and Steroidobacter (p < .05).
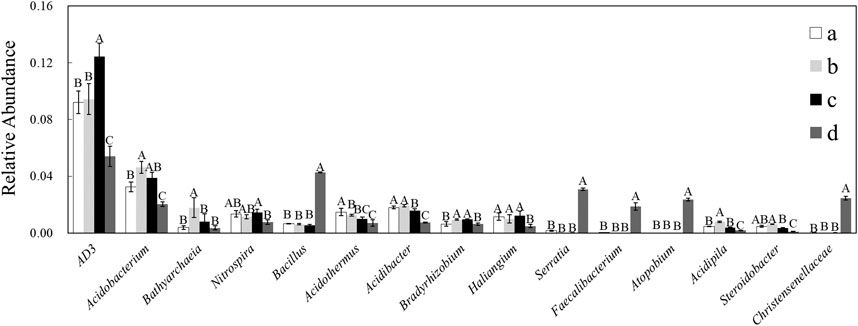
FIGURE 6. Bacterial genera with high relative abundance and significance. Different uppercase letters above bars within the same genus indicate significant differences among treatments (p < .05). (A) litchi orchard with no intercropping; (B) litchi orchard intercropped with Desmodium ovalifolium; (C) litchi orchard intercropped with Grona heterocarpos; (D) litchi orchard intercropped with Stylosanthes guianensis.
Correlation between soil bacterial communities and soil properties
Redundancy analysis (RDA) was conducted to study a correlation between soil bacterial communities and properties (Figure 7). RDA of the phylum’s ten most abundant species at the phylum showed that the first and second axes individually explained 51.98% and 41.15% of the variance, respectively. Similarly, at the genus level, the first and second axes explained 49.46% and 33.35% of the variance, respectively. The AP vector pointed toward Actinobacteriota and Firmicutes, and the soil water content (SWC) vector pointed toward Verrucomicrobiota, Bacteroidota, and Proteobacteria, indicating that the correlation between AP content as well as Actinobacteriota and Firmicutes was positive, and positive correlations were found between the SWC content as well as Verrucomicrobiota, Bacteroidota, and Proteobacteria. Gemmatimonadota and Myxococcota were positively correlated with the soil total nitrogen and SOM content. The NO3−-N and NH4+-N contents were positively correlated with Acidobacteriota and Chloroflexi. At the genus level, the dominating genera, AD3, Acidothermus, Acidobacterium, Occallatibacter, Bradyrhizobium, Acidibacter, GAL15, Candidatus_Solibacter, and Sphingomonas, were negatively correlated with AP and SWC but positively correlated with NO3−-N and AK. In addition, Serratia, Christensenellaceae, Faecalibacterium, Candidatus_Udaeobacter, and Candidatus_Koribacter were negatively correlated with NO3−-N and AK but positively correlated with AP and SWC. Haliangium and Nitrospira were negatively correlated with AP and NH4+-N but positively correlated with TN and SOM, whereas Bacillus showed the opposite trend.
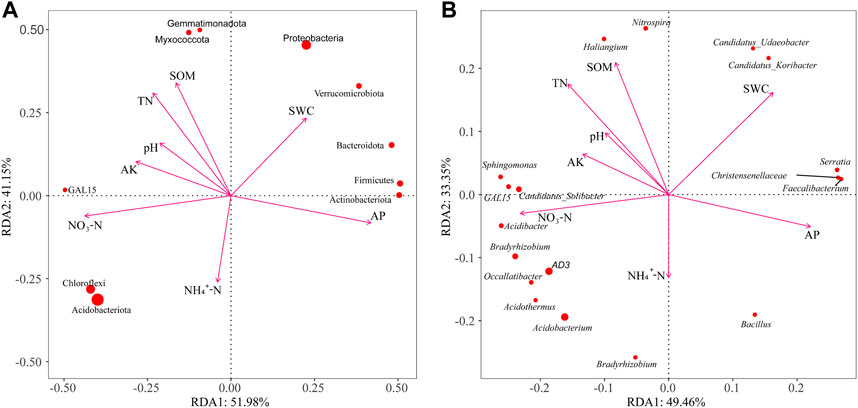
FIGURE 7. Redundancy analysis (RDA) of soil bacteria at the phylum and genus levels. Results of the RDA of bacteria at the phylum (A) and genus (B) levels. SWC, soil water content; TN, soil total nitrogen content; NH4+-N, ammonium nitrogen content; NO3−-N, nitrate nitrogen content; AP, available phosphorus content; AK, available potassium content; SOM, soil organic matter content.
Discussion
In this study, high-throughput sequencing was used to reveal the effects of three green manure crops (D. ovalifolium, G. heterocarpos, and S. guianensis) on soil microbial community diversity and composition in litchi orchards grown in the tropical monsoon climate of Hainan, China. The results showed that three legumes were planted with altered soil nutrients and bacterial diversity. Among them, it can be known from the OTUs statistics that each treatment has many unique OTUs. Bacterial phyla Acidobacteriota, Chloroflexi, Proteobacteria, and Firmicutes showed higher abundance in all soil treatments.
Effect of intercropping on soil nutrient contents
The input-output balance is a determinant of the soil nutrient content (Christodoulou et al., 2019). Long-term growth of green manure crops improves SOM in Mediterranean region (Castellano-Hinojosa and Strauss, 2020; Repullo-Ruiberriz de Torres et al., 2021). Hu et al. (2022) and Haruna (2019) also reported similar results in the tropics and subtropics. The above studies showed that long-term cultivation of green manure can significantly improve SOM, but this study did not significantly improve the SOM. In the no intercropping treatment, various species of weeds could continuously produce litter on the soil surface, which contributes SOM to the soil. Before the green manure was planted, we clear the weeds on the soil surface, and the green manure has no litter before the mature period. This may be one of the reasons. Legumes are well-known for their ability to establish symbiotic associations with N-fixing rhizobia, contributing to the soil N budget via biological N fixation. Studies have shown that legumes increase soil TN and total carbon (TC) contents and decrease the carbon to nitrogen ratio (Leite et al., 2021). In the present study, the TN content was significantly increased after intercropping of D. ovalifolium, thus showed that short-term green manure application can increase the contents of some soil nutrients and change the soil carbon to nitrogen ratio. The balance between N input and root N uptake forms soil available N. The NH4+-N content was significantly increased after intercropping of the three green manure crops, consistent with a previous study (Zhong et al., 2018). This may be related to nitrogen fixation by leguminous rhizobia. In this study, the three intercropping treatments can provide available nitrogen beyond their own growth needs, and all of them have improvement in soil nitrogen content. In South China, among the macronutrients essential for plant growth, p is the most limiting element, owing to its immobilization by high levels of aluminum (Al) and iron (Fe) (Zhang R. Z. et al., 2020). In this study, the three green manure crops had different effects on the soil available phosphorus (AP) content. A previous study showed that S. guianensis promotes soil phosphatase activity, which may be the higher soil available phosphorus content was enhanced by intercropping (Zhou et al., 2019). Moreover, the promotion of p release by legumes may be related to the secretion of organic acids from roots. This may explain the increase in soil AP content observed in the current study. In previous studies, intercropping plants improved phosphorus use efficiency under low soil phosphorus stress (Wang et al., 2017; Zhu et al., 2023). In this experiment, phosphorus was the main limiting factor in soil nutrients, and intercropping increased soil available phosphorus content, which may be method to improve soil phosphorus use efficiency. During the growth period, green manure crops and fruit trees absorb potassium, thus decreasing the soil available potassium content. In the present study, intercropping with D. ovalifoliu and S. guianensis significantly reduced the soil available potassium content, possibly due to the greater demand for potassium than G. heterocarpos, similar to the results of Ding et al. (2021). However, no-intercropping showed opposite results, which may be related to different planting methods and growth stages.
In previous studies, the cultivation of green manure was an effective method to improve soil surface water content in orchard (Wang et al., 2022). In the present study, the soil water content was not significant affected by G. heterocarpos and S. guianensis, while D. ovalifolium significantly increased the soil water content. This phenomenon may be attributed to the morphology and biology differences of the three plants. For example, G. heterocarpos and S. guianensis plants have erect stems, whereas D. ovalifolium plants produce a great many of branches and stolons from the base of the stem, which are more conducive to minimizing soil water loss through evaporation. Green manure aboveground biomass is an important factor to consider when evaluating alternative chemical fertilizers. In the current study, the aboveground biomass of G. heterocarpos and S. guianensis were greater than those of the other treatments, indicating that they were better adapted to the low-phosphorus orchard environment than the other treatments. By contrast, G. heterocarpos and S. guianensis may be good choices for lychee orchard intercropping.
Effect of intercropping on soil microbiota diversity
Bacteria play highly diverse roles in nature, ranging from pathogenicity to plant growth promotion. Plant species alter soil bacterial communities (Wu et al., 2021; Ablimit et al., 2022). In our study, unlike D. ovalifolium and G. heterocarpos, S. guianensis significantly decreased soil bacterial diversity in litchi orchards. This may be related to the more significant influence of S. guianensis on soil bacteria than other treatments. Some studies have shown that S. guianensis affect soil bacteria function, which may be related to root exudates and soil chemical property (Zhou et al., 2019). Intercropping S. guianensis affects soil bacteria and needs further observation. Most previous studies showed that legume intercropping significantly increases bacterial community diversity (Castellano-Hinojosa and Strauss, 2020; Ding et al., 2021; Zhu et al., 2022). These previous results from long-term trials of orchard intercropping are inconsistent with our findings. These studies are different from ours and may be related to different planted regions and plant species, and may also be related to the period of planted. Zhong et al. (2018) studied 20-year-old legume–persimmons intercropping systems, and found that legumes decreased diversity but did not affect microbial richness. These studies suggest that the duration of legume cover may affect soil bacterial community diversity. Our study was in the tropics; warmer temperatures, more rainfall, and vigorous plant growth may affect soil bacterial communities. Changes in soil bacterial diversity in long-term legume green manure cultivation may require further observations.
Effect of soil bacterial communities
Plants can affect bacterial communities and function through many aspects, such as root exudates, soil nutrients, soil temperature, and soil moisture (Wang X. et al., 2021). At the phylum level, the relative abundance of Firmicutes increased more than others. Firmicutes are one of the most common among the different bacterial clades found in soil. Multiple genera within the phylum Firmicutes are associated with properties related to plant growth promotion, biocontrol, and bioremediation (Chauhan et al., 2017; Naureen et al., 2017). S. guianensis significantly increased the relative abundance of Firmicutes, which has been shown to promote plant growth and inhibit soil pathogens, which may be one of the reasons for the significant increase in soil available phosphorus (Hashmi et al., 2020; Wu et al., 2020). The above discussion results showed that intercropping S. guianensis may be way to improve plant growth and soil phosphorus content, but to consider that it reduces soil bacterial diversity, which requires further observation.
In the no intercropping treatment, significantly increased the abundance of Acidothermus. Acidothermus promotes the breakdown of cellulose and facilitates soil C cycling (Nie et al., 2021), which may be responsible for the increase in SOM content in the no-intercropping control. Bradyrhizobium, a beneficial bacterial genus, promotes nodulation, plant growth, and N fixation in legumes (Favero et al., 2022). Intercropping with D. ovalifolium and G. heterocarpos significantly increased the abundance of Bradyrhizobium, which promotes N accumulation in the soil. Bacillus can dissolve insoluble phosphates and produced phytohormones and antifungal compounds in the soil, while being able to promote plant growth. (Omeiri et al., 2022; James et al., 2023). Serratia exhibits significant plant-beneficial potential in dissolving inorganic phosphates (Shahid et al., 2019). Intercropping with S. guianensis significantly increased the abundance of Bacillus and Serratia, which may boost plant growth by enhancing nutrient acquisition. Taking the above results into consideration, intercropping with different plant species has different effects on soil nutrient contents and microbial communities, consistent with previous findings (Wang et al., 2022). The intercropping of the three legumes can improve some soil nutrients and the abundance of beneficial bacterial communities, but the selection of orchards may need to consider the actual soil conditions.
Interactions between green manure, soil nutrients and soil bacteria
In previous studies, soil N and pH were reported as the most important factors affecting plant growth and soil microbiota (Zhang J. et al., 2020; Ding et al., 2021). In this experiment, the content of soil available phosphorus was very low, and became a key factor affected plant growth. AP showed a negative correlation with Acidobacteria, which is an indicator of nutrient-poor soil environment (Kim et al., 2021). This showed that soil nutrients and soil bacteria have mutual influence. AP showed a strong positive correlation with Serratia, Christensenellaceae, Faecalibacterium, and Bacillus. The relative abundance of these bacteria was higher in the intercropping S. guianensis treatment, which may be one of the reasons for the increase of soil available phosphorus (Shahid et al., 2019). In addition, TN, and soil water content positively correlated with Candidatus_Solibacter, Nitrospira, and Haliangium. The relative abundance of these bacteria was higher in the intercropped D. ovalifolium treatments, which may be the result of soil environment and nutrient interactions. Overall, it appeared that soil bacterial communities were sensitive to variations in plant and environmental factors. The effects of different intercropping treatments on the bacterial community were different, which may be related to the root exudates, morphology and biology of the plants. The specific influencing factors in this experiment need our further study.
Intercropping green manures is a way to sustainably develop agriculture. In this experiment, Different treatments had different effects on soil nutrient and bacterial abundance. For example, D. ovalifolium and G. heterocarpos increased the abundance of N-fixing bacteria such as Bradyrhizobium, which enhance soil N accumulation and plant growth, while S. guianensis increased the abundance of multiple beneficial bacteria such as Serratia and Bacillus, which increased soil phosphorus content. Short-term intercropping of all three legumes reduced bacterial diversity, which requires further observation. From the results, Intercropping D. ovalifolium and S. guianensis treatments improved soil nitrogen and phosphorus content in litchi orchards and increased the abundance of beneficial soil bacteria. Intercropping D. ovalifolium and S. guianensis may be a choice for intercropping in litchi orchards, but long-term observation is required.
Data availability statement
The datasets presented in this study can be found in onlinerepositories. The names of the repository/repositories andaccession number(s) can be found below: https://doi.org/10.6084/m9.figshare.21258363.v1.
Author contributions
DY, BY, YW, YS, and CL performed the experiments. DY, BY, and AH designed the experiments and wrote the manuscript. All authors have read and agreed to the published version of the manuscript.
Funding
This work was supported by Agricultural science and technology innovation service in Hainan Province (HNNYKJY201807), Hainan Province Natural Science Foundation of China (322QN394), the Central Public-interest Scientific Institution Basal Research Fund for Chinese Academy of Tropical Agricultural Science (No. 1630032022025) and Scientific and Technological Transformative Project of Tropical Crops Genetic Resources Institute, Chinese Academy of Tropical Agricultural Sciences (PZS2022001).
Conflict of interest
The authors declare that the research was conducted in the absence of any commercial or financial relationships that could be construed as a potential conflict of interest.
Publisher’s note
All claims expressed in this article are solely those of the authors and do not necessarily represent those of their affiliated organizations, or those of the publisher, the editors and the reviewers. Any product that may be evaluated in this article, or claim that may be made by its manufacturer, is not guaranteed or endorsed by the publisher.
References
Abdalla, M., Hastings, A., Cheng, K., Yue, Q., Chadwick, D., Espenberg, M., et al. (2019). A critical review of the impacts of cover crops on nitrogen leaching, net greenhouse gas balance and crop productivity. Glob. Chang. Biol. 25 (8), 2530–2543. doi:10.1111/gcb.14644
Ablimit, R., Li, W., Zhang, J., Gao, H., Zhao, Y., Cheng, M., et al. (2022). Altering microbial community for improving soil properties and agricultural sustainability during a 10-year maize-green manure intercropping in Northwest China. J. Environ. Manage. 321, 115859. doi:10.1016/j.jenvman.2022.115859
Bao, S. D. (1999). Analysis of soil agriculture chemistry. Beijing: China Agriculture Press. in Chinese.
Bokulich, N. A., Kaehler, B. D., Rideout, J. R., Dillon, M., Bolyen, E., Knight, R., et al. (2018). Optimizing taxonomic classification of marker-gene amplicon sequences with QIIME 2 ' s q2-feature-classifier plugin. Microbiome 6 (1), 90. doi:10.1186/s40168-018-0470-z
Bunemann, E. K., Bongiorno, G., Bai, Z., Creamer, R. E., De Deyn, G., de Goede, R., et al. (2018). Soil quality - a critical review. Soil Biol. biochem. 120, 105–125. doi:10.1016/j.soilbio.2018.01.030
Callahan, B. J., McMurdie, P. J., Rosen, M. J., Han, A. W., Johnson, A. J. A., and Holmes, S. P. (2016). DADA2: High-resolution sample inference from Illumina amplicon data. Nat. Methods 13 (7), 581–583. doi:10.1038/NMETH.3869
Castellano-Hinojosa, A., and Strauss, S. L. (2020). Impact of cover crops on the soil microbiome of tree crops. Microorganisms 8 (3), 328. doi:10.3390/microorganisms8030328
Chauhan, A., Guleria, S., Balgir, P. P., Walia, A., Mahajan, R., Mehta, P., et al. (2017). Tricalcium phosphate solubilization and nitrogen fixation by newly isolated Aneurinibacillus aneurinilyticus CKMV1 from rhizosphere of Valeriana jatamansi and its growth promotional effect. Braz. J. Microbiol. 48 (2), 294–304. doi:10.1016/j.bjm.2016.12.001
Christodoulou, E., Agapiou, A., Anastopoulos, I., Omirou, M., and Ioannides, I. M. (2019). The effects of different soil nutrient management schemes in nitrogen cycling. J. Environ. Manag. 243, 168–176. doi:10.1016/j.jenvman.2019.04.115
Ding, T. T., Yan, Z. C., Zhang, W. Z., and Duan, T. Y. (2021). Green manure crops affected soil chemical properties and fungal diversity and community of apple orchard in the loess plateau of China. J. Soil Sci. Plant Nutr. 21 (2), 1089–1102. doi:10.1007/s42729-021-00424-0
Favero, V. O., Carvalho, R. H., Leite, A. B. C., Teles dos Santos, D. M., Freitas, K. M., Boddey, R. M., et al. (2022). Bradyrhizobium strains from Brazilian tropical soils promote increases in nodulation, growth and nitrogen fixation in mung bean. Appl. Soil Ecol. 175, 104461. doi:10.1016/j.apsoil.2022.104461
Gao, W. H., Gao, K., Guo, Z. H., Liu, Y., Jiang, L., Liu, C., et al. (2021). Different responses of soil bacterial and fungal communities to 3 years of biochar amendment in an alkaline soybean soil. Front. Microbiol. 12, 630418. doi:10.3389/fmicb.2021.630418
Haruna, S. I. (2019). Influence of winter wheat on soil thermal properties of a Paleudalf. Int. Agrophys 33 (3), 389–395. doi:10.31545/intagr/110850
Hashmi, I., Bindschedler, S., and Junier, P. (2020). “Chapter 18 – Firmicutes,” in Beneficial microbes in agro-ecology. Editors N. Amaresan, M. Senthil Kumar, K. Annapurna, K. Kumar, and A. Sankaranarayanan (Massachusetts, United States: Academic Press), 363–396.
Hu, A., Huang, R., Liu, G. D., Huang, D. F., and Huan, H. F. (2022). Effects of green manure combined with phosphate fertilizer on movement of soil organic carbon fractions in tropical sown pasture. Agronomy 12, 1101. doi:10.3390/agronomy12051101
James, N., Umesh, M., Sarojini, S., Shanmugam, S., Nasif, O., Alharbi, S. A., et al. (2023). Unravelling the potential plant growth activity of halotolerant Bacillus licheniformis NJ04 isolated from soil and its possible use as a green bioinoculant on Solanum lycopersicum L. Environ. Res. 216, 114620. doi:10.1016/j.envres.2022.114620
Jiang, N. H., Zhu, H. L., Liu, W., Fan, C., Jin, F., and Xiang, X. (2021). Metabolite differences of polyphenols in different litchi cultivars (Litchi chinensis sonn.) based on extensive targeted metabonomics. Molecules 26 (4), 1181. doi:10.3390/molecules26041181
Kim, H-S., Lee, S-H., Jo, H. Y., Finneran, K. T., and Kwon, M. J. (2021). Diversity and composition of soil Acidobacteria and Proteobacteria communities as a bacterial indicator of past land-use change from forest to farmland. Sci. Total Environ. 797, 148944. doi:10.1016/j.scitotenv.2021.148944
Kachurina, O. M., Zhang, H., Raun, W. R., and Krenzer, E. G. (2000). Simultaneous determination of soil aluminum, ammonium-and nitrate-nitrogen using 1 M potassium chloride extraction. Commun. Soil Sci. Plant Anal. 31, 893–903. doi:10.1080/00103620009370485
Leite, H. M. F., Calonego, J. C., Rosolem, C. A., Mendes, L. W., Moraes, L. N., Grotto, R. M. T., et al. (2021). Cover crops shape the soil bacterial community in a tropical soil under no-till. Appl. Soil Ecol. 168, 104166. doi:10.1016/j.apsoil.2021.104166
Li, W. C., Tian, X. L., Sheng, H. Y., Ekawati, D., Zhou, Y., and Zhang, R. (2020). Response of bacterial compositions to soil biochemical properties under mulching-intensive management in a Phyllostachys edulis forest. Appl. Soil Ecol. 150, 103436. doi:10.1016/j.apsoil.2019.103436
Love, M. I., Huber, W., and Anders, S. (2014). Moderated estimation of fold change and dispersion for RNA-seq data with DESeq2. Genome Biol. 15 (12), 550. doi:10.1186/s13059-014-0550-8
Ma, Y. H., Fu, S. L., Zhang, X. P., Zhao, K., and Chen, H. Y. H. (2017). Intercropping improves soil nutrient availability, soil enzyme activity and tea quantity and quality. Appl. Soil Ecol. 119, 171–178. doi:10.1016/j.apsoil.2017.06.028
Maitra, S., Hossain, A., Brestic, M., Skalicky, M., Ondrisik, P., Gitari, H., et al. (2021). Intercropping-a low input agricultural strategy for food and environmental security. Agronomy 11 (2), 343. doi:10.3390/agronomy11020343
Mandal, S., Van Treuren, W., White, R. A., Eggesbo, M., Knight, R., and Peddada, S. D. (2015). Analysis of composition of microbiomes: A novel method for studying microbial composition. Microb. Ecol. Health Dis. 26, 27663. doi:10.3402/mehd.v26.27663
Naureen, Z., Rehman, N. U., Hussain, H., Hussain, J., Gilani, S. A., Al Housni, S. K., et al. (2017). Exploring the potentials of lysinibacillus sphaericus za9 for plant growth promotion and biocontrol activities against phytopathogenic fungi. Front. Microbiol. 8, 1477. doi:10.3389/fmicb.2017.01477
Nie, H., Qin, T., Yan, D., Lv, X., Wang, J., Huang, Y., et al. (2021). How do tree species characteristics affect the bacterial community structure of subtropical natural mixed forests? Sci. Total Environ. 764, 144633. doi:10.1016/j.scitotenv.2020.144633
Omeiri, M., Khnayzer, R., Yusef, H., Tokajian, S., Salloum, T., and Mokh, S. (2022). Bacillus spp. isolated from soil in Lebanon can simultaneously degrade methomyl in contaminated soils and enhance plant growth. Biocatal. Agric. Biotechnol. 39, 102280. doi:10.1016/j.bcab.2022.102280
Repullo-Ruiberriz de Torres, M. A., Carbonell-Bojollo, R. M., Moreno-Garcia, M., Ordonez-Fernandez, R., and Rodriguez-Lizana, A. (2021). Soil organic matter and nutrient improvement through cover crops in a Mediterranean olive orchard. Soil Tillage Res. 210, 104977. doi:10.1016/j.still.2021.104977
Segata, N., Izard, J., Waldron, L., Gevers, D., Miropolsky, L., Garrett, W. S., et al. (2011). Metagenomic biomarker discovery and explanation. Genome Biol. 12 (6), R60. doi:10.1186/gb-2011-12-6-r60
Shahid, M., Javed, M. T., Masood, S., Akram, M. S., Azeem, M., Ali, Q., et al. (2019). Serratia sp. CP-13 augments the growth of cadmium (Cd)-stressed Linum usitatissimum L. by limited Cd uptake, enhanced nutrient acquisition and antioxidative potential. J. Appl. Microbiol. 126 (6), 1708–1721. doi:10.1111/jam.14252
Vicente-Vicente, J. L., Garcia-Ruiz, R., Francaviglia, R., Aguilera, E., and Smith, P. (2016). Soil carbon sequestration rates under mediterranean woody crops using recommended management practices: A meta-analysis. Agric. Ecosyst. Environ. 235, 204–214. doi:10.1016/j.agee.2016.10.024
Vukicevich, E., Lowery, T., Bowen, P., Urbez-Torres, J. R., and Hart, M. (2016). Cover crops to increase soil microbial diversity and mitigate decline in perennial agriculture. A review. Agron. Sustain. Dev. 36 (3), 48. doi:10.1007/s13593-016-0385-7
Wang, J. C., Ren, C. Q., Cheng, H. T., Zou, Y. K., Bughio, M. A., and Li, Q. F. (2017). Conversion of rainforest into agroforestry and monoculture plantation in China: Consequences for soil phosphorus forms and microbial community. Sci. Total Environ. 595, 769–778. doi:10.1016/j.scitotenv.2017.04.012
Wang, T., Duan, Y., Liu, G., Shang, X. W., Liu, L. F., Zhang, K. X., et al. (2022). Tea plantation intercropping green manure enhances soil functional microbial abundance and multifunctionality resistance to drying-rewetting cycles. Sci. Total Environ. 810, 151282. doi:10.1016/j.scitotenv.2021.151282
Wang, X., Fang, L. C., Beiyuan, J. Z., Cui, Y. X., Peng, Q., Zhu, S. L., et al. (2021). Improvement of alfalfa resistance against Cd stress through rhizobia and arbuscular mycorrhiza fungi co-inoculation in Cd-contaminated soil. Environ. Pollut. 277, 116758. doi:10.1016/j.envpol.2021.116758
Wu, T. T., Liu, W. J., Wang, D. G., Zou, Y. K., Lin, R., Yang, Q., et al. (2020). Organic management improves soil phosphorus availability and microbial properties in a tea plantation after land conversion from longan (Dimocarpus longan). Appl. Soil Ecol. 154, 103642. doi:10.1016/j.apsoil.2020.103642
Wu, Y. P., Wang, X., Hu, R. G., Zhao, J. S., and Jiang, Y. B. (2021). Responses of soil microbial traits to ground cover in citrus orchards in central China. Microorganisms 9 (12), 2507. doi:10.3390/microorganisms9122507
Xu, Z., Li, C. J., Zhang, C. C., Yu, Y., van der Werf, W., and Zhang, F. S. (2020). Intercropping maize and soybean increases efficiency of land and fertilizer nitrogen use; A meta-analysis. Field Crops Res. 246, 107661. doi:10.1016/j.fcr.2019.107661
Zahran, H. H. (1999). Rhizobium-legume symbiosis and nitrogen fixation under severe conditions and in an arid climate. Microbiol. Mol. Biol. Rev. 63 (4), 968–989. doi:10.1128/mmbr.63.4.968-989.1999
Zhang, J., Feng, L., Ouyang, Y., Hu, R., Xu, H., and Wang, J. (2020). Phosphate-solubilizing bacteria and fungi in relation to phosphorus availability under different land uses for some latosols from Guangdong, China. Catena 195, 104686. doi:10.1016/j.catena.2020.104686
Zhang, R. Z., Mu, Y., Li, X. R., Li, S. M., Sang, P., Wang, X. R., et al. (2020). Response of the arbuscular mycorrhizal fungi diversity and community in maize and soybean rhizosphere soil and roots to intercropping systems with different nitrogen application rates. Sci. Total Environ. 740, 139810. doi:10.1016/j.scitotenv.2020.139810
Zhao, X. H., Dong, Q. Q., Han, Y., Zhang, K. Z., Shi, X. L., Yang, X., et al. (2022). Maize/peanut intercropping improves nutrient uptake of side-row maize and system microbial community diversity. BMC Microbiol. 22 (1), 14. doi:10.1186/s12866-021-02425-6
Zhong, Z. M., Huang, X. S., Feng, D. Q., Xing, S. H., and Weng, B. Q. (2018). Long-term effects of legume mulching on soil chemical properties and bacterial community composition and structure. Agric. Ecosyst. Environ. 268, 24–33. doi:10.1016/j.agee.2018.09.001
Zhou, Y., Qin, Y., Liu, X., Feng, Z., Zhu, H., and Yao, Q. (2019). Soil bacterial function associated with stylo (legume) and bahiagrass (grass) is affected more strongly by soil chemical property than by bacterial community composition. Front. Microbiol. 10, 798. doi:10.3389/fmicb.2019.00798
Zhu, L. Z., He, J., Tian, Y., Li, X. Y., Li, Y. H., Wang, F., et al. (2022). Intercropping wolfberry with gramineae plants improves productivity and soil quality. Sci. Hortic. 292, 110632. doi:10.1016/j.scienta.2021.110632
Keywords: green manure, high-throughput sequencing, soil properties, soil bacterial community structure, soil bacterial diversity
Citation: Yuan B, Yu D, Hu A, Wang Y, Sun Y and Li C (2023) Effects of green manure intercropping on soil nutrient content and bacterial community structure in litchi orchards in China. Front. Environ. Sci. 10:1059800. doi: 10.3389/fenvs.2022.1059800
Received: 02 October 2022; Accepted: 14 December 2022;
Published: 09 January 2023.
Edited by:
Fujiang Hou, Lanzhou University, ChinaReviewed by:
Meng Wu, Institute of Soil Science, Chinese Academy of SciencesSushanta Naik, Indian Council of Agricultural Research (ICAR), India
Copyright © 2023 Yuan, Yu, Hu, Wang, Sun and Li. This is an open-access article distributed under the terms of the Creative Commons Attribution License (CC BY). The use, distribution or reproduction in other forums is permitted, provided the original author(s) and the copyright owner(s) are credited and that the original publication in this journal is cited, in accordance with accepted academic practice. No use, distribution or reproduction is permitted which does not comply with these terms.
*Correspondence: Daogeng Yu, geng0209@126.com
†These authors contributed equally to this work