- 1School of Water Resources and Environment, China University of Geosciences (Beijing), Beijing, China
- 2Chinese Academy of Geological Sciences, Beijing, China
- 3Key Laboratory of Computational Geodynamics, Chinese Academy of Sciences, Beijing, China
- 4College of Earth and Planetary Sciences, University of Chinese Academy of Sciences, Beijing, China
- 5Beijing Yanshan Earth Critical Zone National Research Station, University of Chinese Academy of Sciences, Beijing, China
- 6Beijing Institute of Geology, Beijing, China
- 7Beijing Institute of Hydrogeology and Engineering Geology, Beijing, China
Groundwater plays important roles in human community development and the urbanization process as the results of global environmental change and rapid population growth. A clear understanding of the vertical and horizontal spatial distribution characteristics of groundwater and sediment is the premise of efficient groundwater resource management and utilization in alluvial plains. To explore the evolution process of groundwater and the distribution of sediment in the Yongding River (YDR) alluvial fan, a typical profile from Mentougou to Fengheying was chosen to conduct an extensive hydrogeological investigation with multiple hydrochemical, isotopic, and age dating analyses, which represents one of the most typical hydrogeological units in the YDR alluvial fan. The analysis results show that (i) along the profile, the water’s chemistry type gradually changes from HCO3-Ca·Mg to HCO3-Na. The contents of coarse grain gradually decrease while fine particles regularly increase. Also, a similar evolution trend is found in the vertical direction. (ii) The annual renewable rate of groundwater is > 10% in the upper fan, 2%–8% nearby Daxing, and < 2% in Fengheying. (iii) In the proximal fan area, the average flow velocity of the single and double layer is about 65 m yr−1 and of approximately 18.9 m yr−1. From the mid fan to distal fan, the value of velocity and hydraulic conductivity decreases from 12.4 m yr−1 to 3.75 m yr−1 and from 8.54 m yr−1 to 0.64 m yr−1, respectively. (iv) The 14C analysis results indicate that the age of shallow and deep groundwater ranges from 5a to 60a and from 4000a to 12000a, respectively. The groundwater age increases with depth in the vertical direction and increases from northwest to southeast along in the alluvial fan in the horizontal direction. Understanding the evolution process of sediment and hydrochemistry in the YDR alluvial fan provides a valuable reference for groundwater resource development, utilization, and pollution prevention in the alluvial fan.
1 Introduction
Water shortage, extreme climate, and vulnerable eco-environment are the most pressing problems for the urbanization process. More than half of the global population relies upon groundwater for drinking purpose, and the dependency with rapid growth of urban population is expected to continuously increase (LiBianLiHuangQiLiu et al., 2021; Xiao et al., 2022a). These acute problems cause the intensive extraction and utilization of groundwater, groundwater level decline, and water quality problems (Edmunds, 2009; LiuZhouLuoWangMcClainWang et al., 2022). Groundwater in the alluvial–proluvial plain is the foremost water source. The Yongding River (YDR) is the mother river of Beijing, and several urban water supply sources and observation wells are located along the YDR. The YDR alluvial plain is one of the most important hydrogeological units in northern China. Due to the complexity of hydrogeological conditions, identifying the spatial variability of the sediment and aquifer in the alluvial fan is difficult.
Spatial variability and zonation are universal characteristics in nature, such as vegetation distribution, topography, climate, geotherm, and precipitation (Naito et al., 1995; Guillen and Palanques, 1997; Sarkar and Guha, 1997; Mallik et al., 2001). The geological phenomena also show zoning characteristics, including the geological structure, magmatic activity, weathering crust, and enrichment state of geochemical elements (Robion et al., 1997; Wogelius et al., 1997; Piao et al., 2010; Brooke et al., 2018). Several previous studies have revealed the temporal and spatial distribution characteristics of groundwater and sediment in alluvial fans (Yang, 1986; Zhou et al., 2009; Straub and Wang, 2013; Forzoni et al., 2014; Litty et al., 2017; Ezquerro et al., 2019), including the distribution characteristics of joint and fracture (Cao et al., 2018), groundwater hydrochemical characteristics (Arenas et al., 2001; Wang et al., 2011), and the interaction between tectonism, sedimentary formation, and groundwater (Benvenuti, 2003; Harvey et al., 2005; Li et al., 2008a; Mas-Pla et al., 2016). For instance, in the Chaobai River alluvial fan, a hydrochemistry analysis and an environmental isotope analysis were used to determine the hydrogeological structure, estimate groundwater renewal rates, and analyze hydrodynamical characteristics (Li et al., 2008b; Zhai et al., 2013; Guo et al., 2014; Li et al., 2014). Based on the geological conditions and hydrogeological structure of the North China Plain, the analysis of 18O, 3H, and 14C was used to reveal the horizontal and vertical hydrodynamic conditions and the zoning characteristics (Lou et al., 2006; Xiao et al., 2022b). In the Songliao Basin, the hydrodynamic intensity can be classified into strong, weak, and stagnant water zones with depth increase by analyzing groundwater dynamic field factors and chemical characteristics (Li et al., 2009). Most of the previous studies focused on a single object, groundwater or sediment, or applied a single hydrochemistry method to analyze, while a few studies applied multiple test methods to analyze the distribution characteristics of groundwater and sediment in the alluvial plain.
Alluvial aquifers of the YDR alluvial plain are the ideal representatives for identifying the spatial distribution characteristics of sediment and hydrochemicals in terms of geo-conditions. Although the hydrochemistry characteristics of the YDR alluvial fan has been reported in a number of studies (Cheng et al., 2001; Yu et al., 2007; Chen et al., 2010a; Chen et al., 2010b; Zhai et al., 2011a; Zhai et al., 2011b; Zhai et al., 2011c; Zhai et al., 2011d; Xun et al., 2011; Yu et al., 2011; Liu et al., 2012a; Liu et al., 2013a; Xiao et al., 2022c), knowledge of the evolution process of groundwater identified by multiple hydrochemical tests still remains relatively limited, especially from the two perspectives of groundwater and sediment analysis. To improve understanding of the groundwater evolution process in the YDR alluvial fan, the typical profile along the direction of Mentougou to Fengheying was chosen and analyzed by the isotopic analysis, sedimentary analysis, and age dating to identify the vertical and horizontal spatial distribution characteristics and develop a conceptual model to understand the groundwater evolution process, which is helpful to provide a scientific foundation for rational groundwater development and protection. This study analyzed the distribution characteristics of sediment and groundwater by a sedimentary test, hydrochemistry test, and isotope analysis. The aims were to (i) reveal aquifer spatial distribution characteristics in the alluvial fan based on sedimentation analysis; (ii) estimate the groundwater hydrodynamic characteristics, such as flow-renewal rate, flow velocity, and permeability-parameter distribution through water table investigation and residence-time dating (14C and 3H); (iii) evaluate groundwater quality from the hydrochemical horizontal and vertical distributions for different aquifer-unit elements and layers; and (iv) identify the concept model of groundwater evolution process in the alluvial plain. The results of the present study can improve the understanding of the groundwater evolution process and provide a valuable reference for managing groundwater resources in the alluvial plains worldwide.
2 Materials and methods
2.1 Topography and hydrological setting
The YDR alluvial–proluvial plain is located in the middle-southern part of the Beijing plain. The study area (116°50′–116°53′ E, 39°26′–40°05′ N) is approximately 2453 km2 (Figure 1). The Western Hills are situated in the western part of the study area, including Dongling Mountain (elevation 2302 m) and Baihua Mountains (elevation 1991 m). The southeast plain area is formed by the alluvial–proluvial deposition. The YDR alluvial–proluvial plain formed in the Late Pleistocene (12,000 year), and the river channels have changed several times, which cause a complex development of the alluvial fan throughout the evolution history. Shijingshan District, Beijing urban area, and Tongzhou District are the fan apex, the middle part of the fan, and marginal fan, respectively (Liu et al., 2012b; Lv, 2012; Liu et al., 2013b).
The climate characteristic of the study area is the semi-arid and semi-humid warm temperature continental monsoon climate. According to the monitoring data collected from Beijing Municipal Meteorological Station, the annual average value of air temperature is 11.54°C during the interval of 1959–2014, and the highest value of 28.2°C and the lowest value of −16.8°C occurred in July and January, respectively. The annual average values of rainfall and evaporation are 567.7 mm and 1816.49 mm, respectively. About 60–70% annual precipitation occurred between June and August.
2.2 Geological conditions
The study area is located in the upper-middle part of the sub-groundwater system of the YDR alluvial–proluvial fan (Liu et al., 2008; Liu et al., 2013b) (Figure 2A), which is in the fringe area terrain of the western uplift of Beijing. The stratigraphy of the study area includes from the Proterozoic to Cenozoic eras, except for the Upper–Middle Ordovician, Silurian, Devonian, Lower Carboniferous, and Triassic systems. The tectonics is well-developed with a series of complex folds. The direction of main faults, which are nearly perpendicular to second-order extension fractures, is consistent with that of the fold axis. Pre-quaternary strata also elongate along the north east–east (NEE) direction in the western mountains. Figure 2A briefly describes the general geological setting of the study area including the lithology distribution and water yield property. The geologic cross-section (Figure 2B) shows that the tertiary strata are mostly underlain by Cenozoic sediments in the north of the third water plant of Beijing.
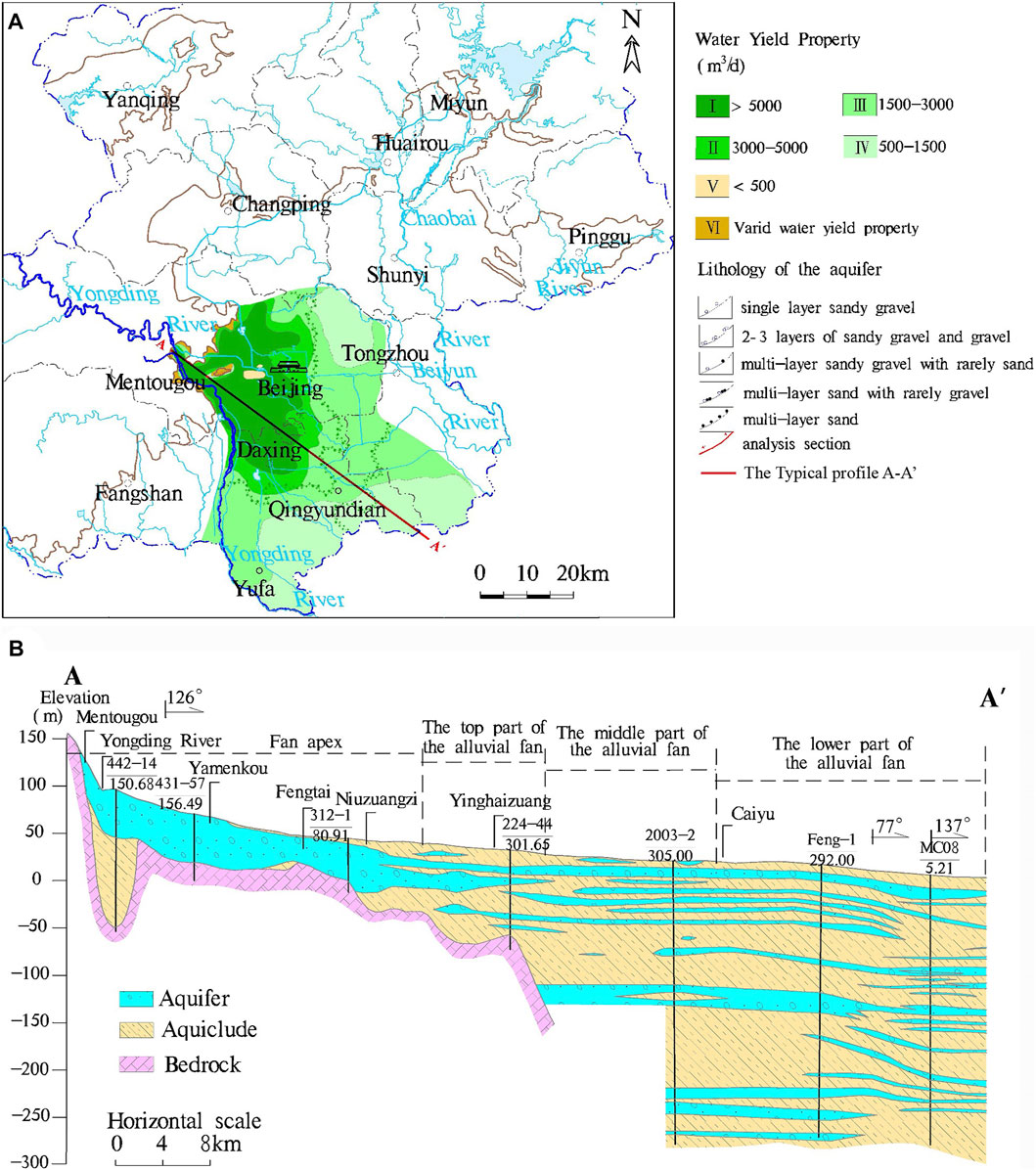
FIGURE 2. (A) Schematic map of groundwater yield property zoning and lithology of the Yongding River groundwater system in Beijing and (B) hydrogeological profile of the Yongding River alluvial fan.
94% study area is covered by the quaternary stratum layer, which is characterized by notable spatial variations in lithofacies and lithology. Under the influence of several times change of river channels, the thickness of the Quaternary stratum gradually increases from northwest to southeast and extends to more than 300 m in Fengheying in the Daxing district. The lithology in the upstream part of the study area comprises gravel land and sand pebble sedimentation. In the southern part of the alluvial fan, alluvial facies sediments lie >100–200 m below the surface, which comprises a lithology of middle-coarse and-fine sand with thickness and high porosity interspersed with lens and interbedded with sandy gravel layers in certain locations.
In the YDR alluvial fan, the loose-rock pore water occurs in the Quaternary sediments, which is mainly distributed in the mountain valley and the eastern part of the plain. The plain is composed of the modern YDR channel, and the first terrace spans from Sanjiadian to Yongdingxincheng (Zhai et al., 2011d).
In the piedmont and mountain valley, the strata are predominated by eluvial facies, and a zonal distribution with heterogeneous water-yield property exists. In the upstream fan terrain, the aquifer lithology is gravel and coarse sand, and the aquifer is a single or double sand pebble structure with considerable thickness, which has the characteristic of high hydraulic conductivity. The single aquifer thickness ranges from 10 m to 20 m, and the aggregate thickness of the aquifer is about 30 m–70 m. The hydraulic conductivity ranges from 50 m d−1 to 300 m d−1 (Geological Mineral Authority of Beijing, 2008). In the downstream, the aquifer lithology comprises thick accumulations of fine-to-coarse sand with interbedded silt–clay layers of limited lateral extent within the aquifer system. Between the Dongrancun and the Baishiqiaoxi part of the alluvial fan, the aquifer is composed of two or three layers of sand and gravel aquifer with an intercalation (interlayer) of lenticular sand and clay (Figure 2A).
From the western part to the eastern part of the alluvial fan, lithology grain size changes from coarse to fine, the number of layers increases gradually, and the aquifer property changes from unconfined to confined. The results of the hydrostratigraphical analysis indicate that groundwater types can be classified into five groups based on the following background conditions (Figure 2B): ① the fan apex includes single-layer sandy gravel and two or three layers of sandy gravel and gravel; ② the top part of the alluvial fan includes multilayer sandy gravel with rarely sand; ③ the middle part of the alluvial fan is composed of multilayer sand with rarely gravel; and ④ the distal fan comprises multilayer sand.
Groundwater is mainly recharged by rainfall, undercurrent from the river valley, lateral subsurface inflow from surrounding hills, and irrigation return flow (Geological Mineral Authority of Beijing, 2008). Vegetation absorption, evaporation, and artificial exploitation are the main ways of groundwater discharge. Except for agricultural irrigation, urban water supply pumped from groundwater is the main way of groundwater discharge in the urban district of Beijing city. Over-pumping of groundwater over the past decades caused a continuous decline in groundwater table (Geological Mineral Authority of Beijing, 2008).
2.3 Investigation and sampling
Due to the several times change of the YDR channel, the hydrogeological characteristics of the aquifer in the YDR alluvial fan gradually change from a single-pebble aquifer with considerable thickness to a sandy aquifer with thin particles and multiple layers. The typical profile A–A′ in the YDR alluvial fan was chosen to investigate. Several hydrogeological boreholes were selected to analyze the characteristics of sediment and hydrogeology along this typical profile.
Based on the analysis and investigation results published by the Geological Mineral Authority of Beijing & Beijing Institute of Hydrogeology and Engineering Geology (Lv, 2012), a supplementary investigation was carried out, which collected 200 samples in 10 monitoring wells in the proximal fan, mid-fan, and distal fan of the YDR alluvial fan. The depth of monitoring wells ranges from 8.4 m to 128 m. In order to eliminate the external disturbance, all these wells were pumped before sampling for 30 min∼4 h. We obtained 124 sets of hydrogeological test results, including physical properties and components, water level, water-quality testing, and isotopic-age data analysis.
The locations of the investigated points and testing samples were selected from three typical units of the groundwater flow system to reveal the zonality characteristics in the alluvial fan. The distribution of sampling sites along the hydrogeologic profile is shown in Figure 3.
2.4 Methods
2.4.1 Hydrochemistry and isotope testing
Geological and hydrogeological information, such as sedimentary features, vertical lithology log, and water-level distribution, was obtained by drilling and sampling. To reveal the structure of the alluvial fan, the sediment characteristics were initially analyzed by a soil-grading test along the A–A′ transect, which is representative of the YDR alluvial fan. A total of 124 samples were tested for grain-size analysis, and 10 datasets were used for calculation. In relation to major chemical compositions of groundwater for the water type analysis, 14C and 3H dating were used for groundwater dating and the flow-renewal rate, respectively. Flow velocity and permeability distribution were carried forward.
In 2006, nine samples from the shallow aquifers and five samples from the deep aquifer were collected to conduct the analysis of major ions and stable isotopes. The hydrogeochemical composition of all groundwater samples was analyzed in Beijing Institute of Hydrogeology and Engineering Geology. The filtered water samples were analyzed for cations (Na+, K+, Mg2+, and Ca2+). Cl and SO42− were determined by high-performance liquid chromatography (SHIMADZU, LC-10ADvp). The error of oxygen isotope and 13C analysis is less than ±0.1‰. The error of hydrogen isotope analysis is less than ±1‰. The 3H test was conducted by electrolytic enrichment and measured by a Quantulus-1220 (LKB) low background liquid flash recorder with 21.17% detection efficiency (background: 0.81 cpm). 14C was measured by the Quantulus-1220 (LKB) low background liquid flash meter. Chinese sugar carbon was used as the standard. The half-life was 5568 years, starting from 1950.
2.4.2 Groundwater-gradient calculation and groundwater renewal rate
According to water head and movement distance, we estimate the hydraulic gradient as follows:
where I is the hydraulic gradient, H is the water head, and L is the movement distance.
According to the tritium isotope results (Zhai et al., 2011; Lv, 2012), groundwater-flow velocity can be estimated, and the renewal rate in shallow groundwater also can be calculated to investigate zonality characteristics.
The 3H concentration in groundwater is influenced by the initial age and decay of radioactivity. The mixed model was introduced to calculate the renewal rate in shallow groundwater. Tritium’s age is calculated by using the MATLAB program. Assuming that the system was stable before 1952, i.e., the input of water is equal to the output, the calculation formula is as follows:
where Rri is the annual renewal rate, Agwi is the 3H content of groundwater, A0i is the 3H content of input water, λ is the decay constant (3H = 0.05626·yr−1), and i is the time (year) from 0 to 53 (the difference between calculation year and initial year 1952).
The 3H concentration in groundwater is calculated by the constant A0. The results were calculated by the following formulae:
In view of the changes in annual precipitation and recharge, we assume that annual recharge is proportional to annual precipitation. The annual update rate Rri can be obtained by weighted mean value Rr and annual precipitation, namely, Rri = Rr×Pi/Pm. However, the climate of the study area is semi-humid and semi-dry, and a correction factor is additionally required due to the hypothetical condition of no replenishment and minimum limit (Pt) of rainfall amount. Thus, the annual renewal rate is calculated as follows:
2.4.3 Groundwater flow velocity and hydraulic conductivity estimation
The direction of the groundwater flow can be determined based on the contour map of the groundwater table. Along the groundwater flow direction, several groundwater samples with the difference in age were collected, and then the difference in age can be calculated. This difference refers to relative age, i.e., the time between two different sampled points. After measuring the distance between the two points, groundwater flow velocity can be estimated, as shown in Eq. 5:
where L is the distance between (m), t is the age difference (a), and u is the groundwater-flow velocity (m yr−1).
2.4.4 Piper diagrams
Piper diagrams, comprised by two separate trilinear plots and a central diamond, were drawn by AqQa, which were used to illustrate major cation and anion concentrations. The points in the central diamond are from the projection of two trilinear plots. The central diamond-shaped field is used to show the hydrochemical characteristics of groundwater samples.
3 Results
3.1 Sediment characteristics
In Table 1, 83 samples were collected along the A–A′ profile and analyzed for the geotechnical test, which were used to investigate the characteristics of sediment and lithology in the alluvial–proluvial plain. For each soil sample collected from different depths in hydrogeological drilling, the constituents of different particles and mineral composition in rock and clay were tested. The zonality characteristic of the particle size was identified. The analysis of mineral composition in rocks includes quartz, potassic feldspar, plagioclase feldspar, calcite, dolomite, pyrite, hornblende, and clay minerals. The clay mineral test includes illite mixed layer, illite, kaolinite, and chlorite. In Figure 4, the left and right panels of each borehole depict the clay mineral test results and mineral composition in rock, respectively. All results are expressed in the form of pie charts.
In Table 2 and Figure 5, the particle size shows significant zoning characteristics, which gradually becomes fine from piedmont to lowland. Sediment thickness is approximately 40 m–80 m in the proximal fan, 80 m–120 m in the mid-fan, and 300 m–500 m in the distal fan. The proximal fan mainly consists of gravel and sand, which gradually divide into two or three layers of the sand gravel stratum area in the mid-fan. The grain sizes of >2 mm and <0.075 mm in sample YY account for 67.9% and 0.7%, respectively. For sample Y1, the percent of 0.5–0.25, 0.25–0.075, and 0.075–0.005 mm is 20.50%, 55.80%, and 23.70%, respectively. The Caiyuan part of the distal fan is dominated by fine clay and interbedded sand and gravel. The 0.25–0.075 mm, 0.075–0.005 mm, and <0.005 mm of sample YZ are about 5.13%, 86.65%, and 8.22%, respectively.
The sedimentation characteristic and landform lithology of the YDR alluvial–proluvial fan show significant zonal characteristics. The sediment characteristic of the YDR profile is that the contents of coarse grain regularly decrease, whereas those of the fine particles increase. The closer to the proximal fan, the thicker the sediment is. The closer to the distal fan, the finer the particle size is. The characteristic of sedimentary dynamics is rapid torrent accumulation with drag-flow properties (Forzoni et al., 2014). In the Mentougou part of the proximal fan, the migration of the gravel pebble is dominated by bed load and supplemented by rare saltation and suspension. Transport relies mainly on saltation, whereas the bed-load subsidiary in mid-fan is predominated by sand. In the Caiyu and Fengheying part of the distal fan, the migration depends on the suspension and kinetic energy of water flow decline, which tends to stabilize static water deposition. The material migration also reveals the zonality characteristics of hydrodynamic condition from the proximal fan to distal fan in the YDR alluvial fan. Thus, the sedimentation of the YDR alluvial–proluvial fan is based on horizontal zonation characteristics.
3.2 Hydrodynamic characteristic
The monitored data of the groundwater level are collected from 20 observed wells. The detailed information of the groundwater level is summarized in Table 3. The depth of the groundwater level is about 35 m–40 m in the Mentougou—Shijingshan part of the proximal fan area, and the groundwater level shows significant annual variation. From Lugou Bridge to Taixinggong, the depth of the groundwater level decreases to 20 m–25 m (Jiang et al., 2010; Liu et al., 2010). The depth gradually decreases from 20 m–25 m to 5 m–10 m in the Yinghai and Qingyundian part. In the Qingyundian part of the distal fan, the depth of groundwater is < 4 m, where the water table is stable. Thus, the depth of groundwater gradually decreases from the proximal fan and mid-fan to the distal fan. Figure 6 illustrates the isoline map of the water level.
The long-term monitored data of the groundwater level were used to calculate the hydraulic conductivity and hydraulic gradient and estimate the flow rate and the renewable rate in the YDR profile, which provide a good way to understand the zoning characteristic of the alluvial fan.
According to Eq. 1, the hydraulic gradient affected by topography is inversely proportional to the movement distance. Thus, the hydraulic gradient is about 0.55%–1.92% in the proximal fan, while it decreases to 0.22%–0.55% in the distal fan. If the groundwater-runoff condition is relatively bad, the hydraulic gradient ranges between 0.09% and 0.22% in the marginal fan area.
According to Eqs. 2–4, the results show that the renewable rate gradually decreases from the proximal fan to distal fan in the YDR (Table 4). In the proximal fan with a single layer of sand and gravel, the annual renewable rate is ≥ 10%. The annual renewal rate of groundwater in the two-to-three-layer area is about 8%–10%. The hydrodynamic renewal rate of multilayer sandy gravel with occasionally sandy composition ranges from 4% to 8% nearby Yinghaizhuang in Daxing. The value of the hydrodynamic renewable rate is < 2% in the multilayer sand of marginal zone from Caiyu to Fengheying in Tongzhou.
According to the 14C analysis results, the age of shallow and deep groundwater ranges from 5a to 60a and from 4000a to 12000a, respectively. Vertically, the age of groundwater increases with depth. Horizontally, the age of the groundwater also increases from northwest to southeast along the direction of the groundwater flow in the alluvial–proluvial fan. The distribution characteristic of different age groundwater in the horizonal direction indicates that the condition of horizontal runoff is different. According to the hydrostratigraphical analysis, it can be divided into three different parts. The first part is single- or double-layer cobble and gravel with high permeability in the upper fan area. The average velocity from YD1–YD2 is approximately 64.9 m yr−1. The second part is a multilayer with sand in the mid–lower fan. The average velocity from YD2 to YD4 is approximately 12.4 m yr−1. The third part is fine sand with an intercalation (interlayer) of lenticular sand and clay in the proximal fan. The average velocity from YD5 to YD6 is approximately 3.75 m yr−1 (Table 5), which is consistent with the calculated results from Wang and Zhou (2006).
Based on Darcy’s law, hydraulic conductivity can be estimated as follows:
where V is seepage velocity (m yr−1), K is the hydraulic conductivity of the aquifer (m yr−1), I is hydraulic gradient, n is effective porosity, and u is the actual velocity of groundwater (m yr−1).
In the upper fan area (YD1 and YD2), the permeability of single-layer or double-layer cobble and gravel with is high. The average hydraulic conductivity is approximately 18.9 m yr−1. In the mid–lower fan area (YD2, YD3, and YD4), the average hydraulic conductivity of multilayer sand is approximately 8.54 m yr−1. In the distal fan (YD5 and YD6), the average permeability of fine sand with an intercalation (interlayer) of lenticular sand and clay is approximately 0.64 m yr−1. The results of 14C analysis reveals that the direction of groundwater flow is from YD6 to YD5, which is consistent with the distribution of groundwater level (Figure 7).
3.3 Hydrochemistry characteristics
The aquifer type of the YDR alluvial–proluvial fan can be classified into four different aquifers. The first aquifer is a phreatic aquifer. The depth of the phreatic aquifer is < 50 m. The second aquifer is an artesian aquifer, which is widely distributed in the YDR alluvial fan. The depth of this aquifer ranges from 80 m to 100 m. It is consisted of multilayer sand gravel, sand, and clay. The aquifer lithology is the multilayer of sand gravel or sand in the west side of Beihai–Nanyuan, while the aquifer lithology changes from multilayer sand gravel with rarely sand to multilayer sand in the eastern side. For the third confined aquifer system, the depth varies from 150 m to 180 m, which exists in the mid-lower part of the alluvial fan. The aquifer lithology is multilayer sand. The depth of the fourth confined aquifer is approximately 300 m This aquifer is in the quaternary sediment, which is mainly distributed in Tongzhou Kuaizidian, Daxing, Caiyu, and Fengheying.
Groundwater was sampled along the typical profile for hydrochemical analysis, including K+, Na+, Ca2+, Mg2+, HCO3−, CO32−, Cl−, and SO42− (Table 6). The groundwater age-dating data are summarized in Tables 7, 8. Due to the limitations of individual tracers, the combination of 14C and 3H tracer was applied to analyze by synthesis (Figure 8). In the YDR alluvial–proluvial fan, the groundwater hydrochemical type is different along the A–A’ profile, which shows a significant change from a single type to a complex characteristic from the proximal fan to distal fan. The ion concentration of Ca2+, Mg2+, and HCO3− in groundwater gradually decreases with the increase of SO42−, Cl−, and Na + concentration from the piedmont to plain areas. However, in the central areas of the alluvial fan, the ion concentrations of SO42−, Cl−, and Na+ are higher than those in other areas due to intense human activities (Figure 9).
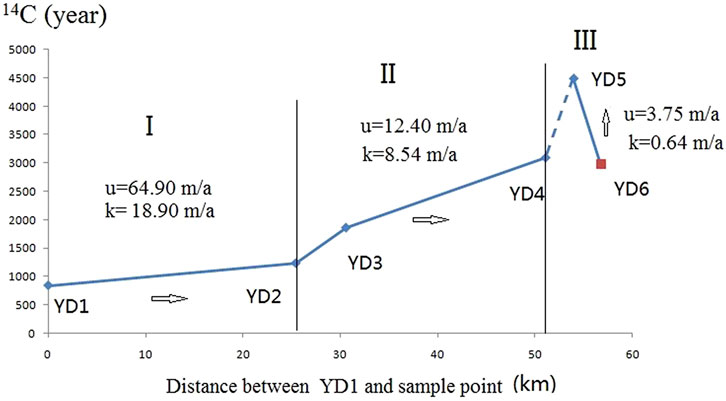
FIGURE 8. Zonality characteristics of groundwater flow velocity and aquifer permeability along the typical profile (I, II, and III indicates the upper part, middle part, and bottom part of the pluvial–alluvial fan, respectively; u is the water-flow velocity, and k is the permeability estimation).
3.3.1 Horizontal zonation of hydrochemical characteristics
The fan apex of the YDR alluvial fan is a single phreatic aquifer, and its hydrochemical component is predominated by the HCO3-Ca-Mg type. Taking the first layer as an example to analysis, under the influence of industry, urban domestic sewage discharge, reclaimed water irrigation, and other human factors, the groundwater hydrochemical type has significant difference from the proximal part to distal part of the alluvial fan (Shijingshan District—the urban area of Beijing—Daxing District), which mainly includes HCO3·Cl·SO4-Ca·Mg, HCO3·Cl-Ca·Mg, HCO3-Mg·Na·Ca, and Cl·HCO3-Ca·Mg. The groundwater hydrogeochemical type in other aquifers of the YDR alluvial fan also show significant horizontal zonation characteristics.
3.3.2 Vertical zonation of hydrochemical characteristics
In the Yongding River Basin, the distribution characteristics of sedimentation and lithology also show vertical zonality. Meanwhile, the groundwater hydrochemical type of shallow and deep aquifers is different in the vertical direction. In the marginal area of the alluvial–proluvial fan, HCO3− is the dominated anion in groundwater. For the groundwater from different depth aquifers, the ion concentration of Ca2+ and Mg2+ gradually decreases with the increase in depth, while the K+ and Na + concentration increases. The hydrochemical type shows obvious differences in different depth aquifers, changing from HCO3-Ca·Mg·Na to HCO3-Na·Ca and HCO3-Na·Ca·Mg. In the middle part of the alluvial fan, the multilayer aquifer can be classified into four aquifers. The first aquifer is an unconfined aquifer, which is dominated by the HCO3-Ca·Mg. The hydrochemical characteristic of the second aquifer is the same as that of the first aquifer. For the third or fourth aquifers near the apex fan, the hydrochemical type is predominated by HCO3-Ca·Mg, which gradually changes from HCO3-Ca·Mg·Na to HCO3-Na·Ca·Mg and HCO3-Na in the marginal area of the alluvial–proluvial fan (Figure 10).
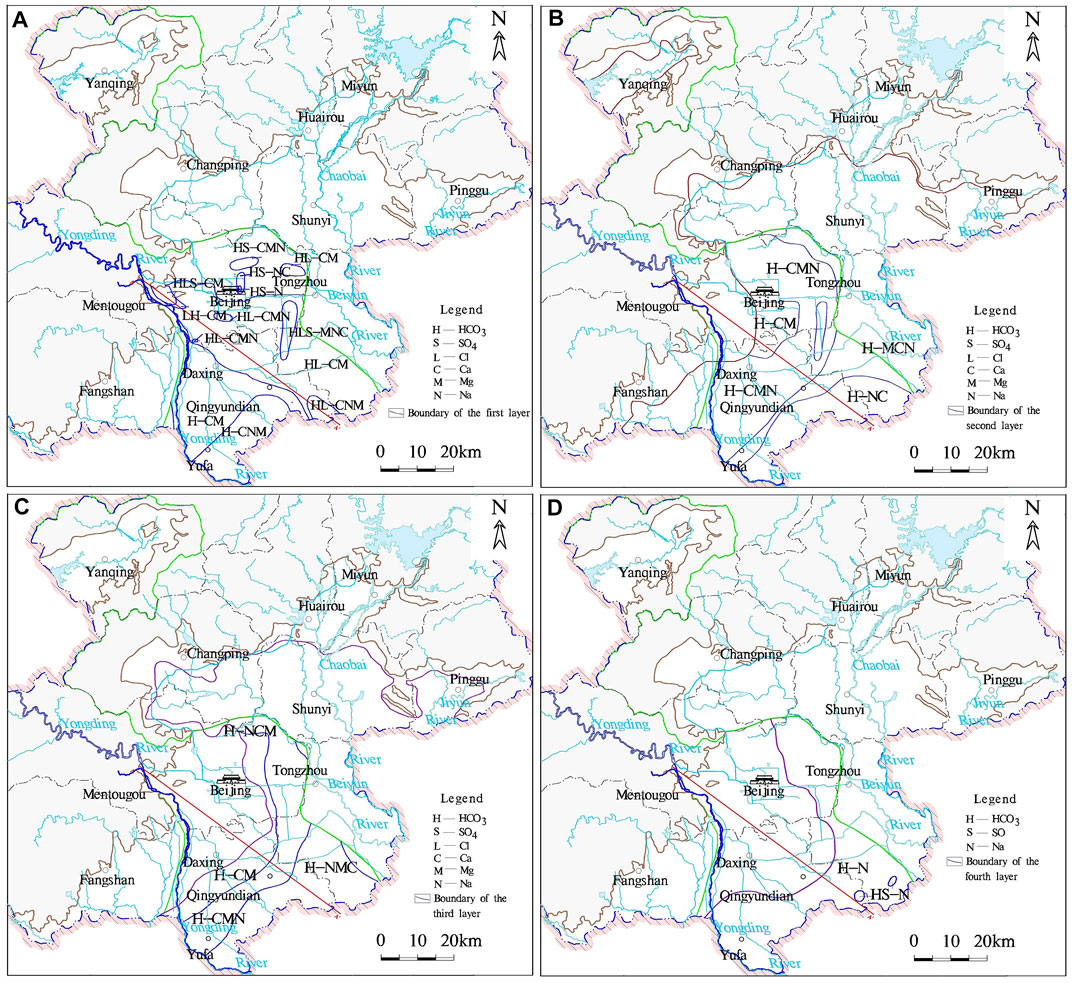
FIGURE 10. Distribution of hydrochemical types in the plain area in Beijing. (A) First aquifer, (B) second aquifer, (C) third aquifer, and (D) fourth aquifer.
4 Discussion
4.1 Hydrochemistry evolution
The hydrochemical characteristic is affected by several external influence factors, including dissolution filtration, cation exchange adsorption, mixing action, de-carbonic acid, de-sulfuric acid, concentration (inspissation), and human activities (Zhang et al., 1997; Wang et al., 1998; Shen and Wang, 2002; Song et al., 2007; Zhang et al., 2010; Zhao et al., 2012; Zheng et al., 2012).
In the upper parts of the alluvial–proluvial fan (the Mentougou and Haidian part), due to the thick sand gravel stratum, good runoff conditions, and deep buried depth, the function of evaporation, dissolution, and filtration in groundwater is extremely weak. The groundwater hydrochemical type is HCO3-Ca·Mg, and the value of total dissolved solids (TDS) is < 0.5 g L−1 (e.g., SY15). In the middle part of the alluvial fan (the Daxing YinghaiZhuang part), the aquifer permeability decreases, and the phreatic level gradually increases with weakened runoff conditions. The function of cation exchange occurs in clays, and the hydrochemical type gradually changes to HCO3-Na·Ca. TDS is approximately 1.0 g L−1 (e.g., SY17). In the marginal part of the alluvial fan (the Qingyundian–Fengyinghe part), the groundwater circulation rate is slow and the buried depth of phreatic level is < 5 m. Phreatic water is mainly affected by evaporation and gradually evolves into the function of inspissation. Due to the confluence of two rivers, Yongding River and Chaobai River, the mixing function also happens in groundwater. The hydrochemical type gradually changes to HCO3·Cl-Na·CaMg and HCO3·Cl-Na. The value of TDS ranges from 1.3 g L−1 to 2.6 g L−1 (e.g., SY18 and SY19). Thus, the evolution process of groundwater hydrochemical shows distinct zonality.
The groundwater buried depth nearby Daxing, Qingyundian (SY20, SY21, and SY22 in Table 2) is < 4 m. Due to the function of vadose soil capillarity and evapotranspiration, the shallow groundwater is intensely evaporated toward the atmosphere. In the vertical direction, TDS concentration slightly decreases with depth increase. Groundwater quality shows significant change from saline water in the upper part to the fresh water in the bottom part, which indicates that groundwater quality also shows vertical zonation.
4.2 Conceptual model for groundwater evolution
The distribution characteristic of sediment and lithology is zonality in the YDR alluvial–proluvial fan. From the proximal fan to the distal fan, the thickness of the sedimentary layer increases, the size of the sediment particle decreases, and the single layer gradually changes to multiple layers. The water-level data and the results of hydrochemical analysis and age-dating in groundwater were used to develop a conceptual model, which reveals the groundwater flow pattern and the evolution process of hydrochemical along the typical profile (Figure 11).
The groundwater level data reveal that the direction of groundwater flow is from west to east. (i) In the upper part of the alluvial fan (Mentougou), the annual renewable rate is > 10%. The renewable rate decreases to 4%–8% nearby the Daxing Yinghaizhuang part, where it is composed of multilayer sandy gravel with occasional sand. In the distal fan part, the values of the renewable rate are 2%–4% and <2% in Yinghaizhuang and Fengheying, respectively. (ii) Based on the result of 14C dating, the average value of groundwater flow velocity decreases from 65 m yr−1 to 3.75 m yr−1∼12.4 m yr−1 from the upper part to the margin part of the alluvial fan. The value of hydraulic conductivity also shows the same change tendency as groundwater flow velocity along the typical profile. The hydraulic conductivity is about 18.9 m yr−1 in the upper part of the alluvial fan, while it decreases to the 0.64 m yr−1 in the distal fan.
Combining the results of hydrochemical and isotopic analysis together with geology, topography, and hydrogeological conditions can improve the understanding of hydrogeologic processes and the reliability of groundwater-flow models. Typical zonality characteristics along the A–A′ profile (from Mentougou to Fengheying) reveal the conceptual model of the groundwater flow system in the YDR alluvial fan. The zoning characteristic of sediment and landform lithology is affected by the hydrodynamic conditions.
5 Conclusion
We provide a systematic analysis of groundwater evolution in the YDR alluvial fan. The analytic results are listed, as follows:
• The distribution characteristic of sediment and landform lithology is affected by the hydrodynamic conditions of the YRD alluvial fan. From the proximal fan to the distal fan in the typical profile, the sedimentary thickness increases from 40m–80 m in Mentougou to 300m–500 m in Caiyu and Fengheying. Coarse grains gradually decrease and fine particles gradually increase. The similar change trend is also found in the vertical direction.
• Zonality characteristics influence hydrodynamic behavior. The annual renewable rate of groundwater decreases from > 10% in the upper fan to 2%–8% nearby Daxing, which is < 2% in the distal fan. For the flow velocity and aquifer permeability, the average value of flow velocity in the single and double layer is 65 m yr−1 and 18.9 m yr−1 in the upper fan, respectively. From the mid fan to the distal fan, velocity decreases from 12.4 m yr−1 to 3.75 m yr−1 and permeability decreases from 8.54 m yr−1 to 0.64 m yr−1.
• The hydrochemical characteristics of the second, third, and fourth aquifers show horizontal and vertical zonation. From the proximal fan to distal fan along the YDR, the hydrochemical type gradually changes from HCO3-Ca·MgNa to HCO3-Na. In the vertical direction, the ion concentration of Ca2+ and Mg2+ decreases, but K+ and Na + increase with depth increase.
• The hydrochemical evolution process is obvious different in the different part of the alluvial fan. The function of dissolution and infiltration is the dominated hydrochemical process in the proximal fan area. From the mid fan to the distal fan, hydrochemical process changes to the function of cation-exchange absorption. In the area of the distal fan, the main hydrochemical process is the function of mixing and inspissation.
This study provides the essential and systematic analysis for identifying hydrochemical characteristics, hydrochemical evolution, and groundwater dynamics in the YDR alluvial fan, which is helpful to protect and manage the mother river of Beijing.
Data availability statement
The datasets presented in this study can be found in online repositories. The names of the repository/repositories and accession number(s) can be found in the article/Supplementary Material.
Author contributions
Conceptualization: KL and XQ; methodology: KL; validation: KL, XQ, and SW; data curation: YS; writing—original draft preparation: KL; writing—review and editing: KL and XQ.
Funding
This study was funded by the China Geological Survey (No. DD20201165, DD20221677), CGS Research (No. JKY202004), National Key Research and Development Program of China (No. 2020YFC1807104) and Fundamental Research Funds for the Central Universities (E1E40411X2 and Y95401PXX2).
Acknowledgments
We would like to thank the professional reviewers and editors.
Conflict of interest
The authors declare that the research was conducted in the absence of any commercial or financial relationships that could be construed as a potential conflict of interest.
Publisher’s note
All claims expressed in this article are solely those of the authors and do not necessarily represent those of their affiliated organizations, or those of the publisher, the editors, and the reviewers. Any product that may be evaluated in this article, or claim that may be made by its manufacturer, is not guaranteed or endorsed by the publisher.
References
Arenas, C., Millán, H., Pardo, G., and Pocoví, A. (2001). Ebro Basin continental sedimentation associated with late compressional pyrenean tectonics (north-eastern Iberia): Controls on basin margin fans and fluvial systems. Basin Res. 13, 65–89. doi:10.1046/j.1365-2117.2001.00141.x
Benvenuti, M. (2003). Facies analysis and tectonic significance of lacustrine fan-deltaic successions in the Pliocene-Pleistocene Mugello Basin, Central Italy. Sediment. Geol. 157, 197–234. doi:10.1016/s0037-0738(02)00234-8
Brooke, S. A. S., Whittaker, A. C., Armitage, J. J., D'Arcy, M., and Watkins, S. E. (2018). Quantifying sediment Transport dynamics on alluvial fans from spatial and temporal changes in grain size, Death Valley, California. JGR. Earth Surf. 123, 2039–2067. doi:10.1029/2018jf004622
Cao, W. G., Yang, H. F., Liu, C. L., Li, Y. J., and Bai, H. (2018). Hydrogeochemical characteristics and evolution of the aquifer systems of Gonghe Basin, Northern China. Geosci. Front. 9 (3), 907–916. doi:10.1016/j.gsf.2017.06.003
Chen, Z. Y., Liu, J., Yang, X. K., Chen, J., and Wang, Y. (2010). The environmental isotope markers of groundwater flow patterns of the Song-Nen Plain. Earth Sci. Front. 17 (6), 94–101. (in Chinese with English abstract).
Chen, Z. Y., Wang, Y., Liu, J., and Wei, W. (2010). Groundwater changes of selected groundwater systems in Northern China in recent fifty years. Quat. Sci. 30 (1), 115–126. (in Chinese with English abstract). doi:10.1016/j.proeps.2013.03.182
Cheng, D. Z., Chen, M., Shi, S. P., and Ma, Z. Z. (2001). Impact of anthropogenic modifications on the rainfall runoff in the upstream of Yongding River. Des. Water Resour. Hydroelectr. Eng. 20 (2), 19–21. (in Chinese with English abstract).
Edmunds, W. M. (2009). Geochemistry's vital contribution to solving water resource problems. Appl. Geochem. 24, 1058–1073. doi:10.1016/j.apgeochem.2009.02.021
Ezquerro, L., Luzón, A., Simón, J. L., and Liesa, C. L. (2019). Alluvial sedimentation and tectono-stratigraphic evolution in a narrow extensional zigzag basin margin (northern Teruel Basin, Spain). J. Palaeogeogr. 8, 29. doi:10.1186/s42501-019-0044-4
Forzoni, A., Storms, J., Whittaker, A., and de Jager, G. (2014). Delayed delivery from the sediment factory: Modeling the impact of catchment response time to tectonics on sediment flux and fluvio-deltaic stratigraphy. Earth Surf. Process. Landf. 39 (5), 689–704. doi:10.1002/esp.3538
Geological Mineral Authority of Beijing & Beijing Institute of Hydrogeology and Engineering Geology, (2008), Groundwater of beijing. China Land Press, Beijing.
Guillen, J., and Palanques, A. (1997). A shoreface zonation in the Ebro Delta based on grain size distribution. J. Coast. Res. 13, 867–878. doi:10.1016/S0924-2716(97)00008-7
Guo, G. X., Hou, Q. L., Xu, L., Liu, J. R., and Xin, B. D. (2014). Delamination and zoning characteristics of quaternary groundwater in Chaobai alluvial-proluvial fan, beijing, based on hydrochemical analysis. Acta Geosci. Sin. 35 (2), 204–210. (in Chinese with English abstract). doi:10.3975/cagsb.2014.02.12
Harvey, A. M., Mather, A. E., and Stokes, M. (2005). “Alluvial fans: Geomorphology, sedimentology, dinamics - introduction. A review of alluvial-fan research,”. Alluv. fans Geomorphol. Sedimentol. Din. Geological Society of London, special publication., 251, 1–7. doi:10.1144/GSL.SP.2005.251.01.01
Jiang, T. S., Yang, Z. S., Huang, Z. F., Shi, J. J., and Cai, L. (2010). Tendency and mechanism analysis of total hardness in shallow groundwater in the suburb of Beijing. Hydrogeology Eng. Geol. 37 (4), 33–37. doi:10.16030/j.cnki.issn.1000-3665.2010.04.007
Li, F. D., Pan, G. Y., Tang, C. Y., Zhang, Q. Y., and Yu, J. J. (2008). Recharge source and hydrogeochemical evolution of shallow groundwater in a complex alluvial fan system, southwest of North China Plain. Environ. Geol. 55, 1109–1122. doi:10.1007/s00254-007-1059-1
Li, Q., Zhou, J. L., Zhou, Y. Z., Bai, C. Y., Tao, H. F., Jia, R. L., et al. (2014). Variation of groundwater hydrochemical characteristics in the plain area of the Tarim Basin, Xinjiang Region, China. Environ. Earth Sci. 72, 4249–4263. doi:10.1007/s12665-014-3320-8
Li, X. Q., Zhang, L., and Hou, X. W. (2008). Use of hydrogeochemistry and environmental isotopes for evaluation of groundwater in Qingshuihe Basin, northwestern China. Hydrogeol. J. 16, 335–348. doi:10.1007/s10040-007-0269-7
Li, Y. H., Deng, Y. E., and Jia, S. Y. (2009). Hydrochemistry characteristics and hydrodynamic zone of groundwater of Tunnel in Wuzhi mountain. Res. Soil & Water Conservation 16 (1), 172–174. (in Chinese with English abstract). doi:10.1042/BSR20080061
LiBianLiHuangQiLiu, S. R. B. J. W. H., Li, B., and Qi, W. (2021). Hyporheic zone geochemistry of a multi-aquifer system used for managed aquifer recharge in Beijing, China. Appl. Geochem. 131, 105032. doi:10.1016/j.apgeochem.2021.105032
Litty, C., Schlunegger, F., and Viveen, W. (2017). Possible threshold controls on sediment grain properties of Peruvian coastal river basins. Earth Surf. Dynam. 5 (3), 571–583. doi:10.5194/esurf-5-571-2017
Liu, F., Li, Y. H., and Lin, J. (2008). A hydrogen and oxygen isotope study of groundwater in the Yongding River drainage of beijing and its environmental significance. Acta Geosci. Sin. 29 (2), 161–166. (in Chinese with English abstract). doi:10.3321/j.issn:1006-3021.2008.02.005
Liu, J. L., Pang, Z. H., Wang, S. F., Kong, Y. L., and Zhou, J. (2010). Impact of precipitation change and human activities on groundwter regime in the Chaobaihe alluvial and diluvial fan in Beijing in the last 30 years. Quat. Sci. 30 (1), 138–144. (in Chinese with English abstract). doi:10.3969/j.issn.1001-7410.2010.01.13
Liu, J. R., Lin, P., and Sun, Y. (2012). Study on groundwater infiltration influence of the Yongding River. Beijing: Beijing Institute of Hydrogeology and Engineering Geology. (in Chinese with English abstract).
Liu, K., Liu, J. R., Sun, Y., Liu, Y. C., Zhang, Y., and Wang, L. Y. (2013). River discharge by using regressive model in gorge of the Yongding River in Beijing. Earth Sci. - J. China Univ. Geosciences 38, 58–64. (in Chinesee with English abstract). doi:10.3799/dqkx.2013.S2.010
Liu, Y. Z., Wu, Q., Gao, Z. H., Lin, P., and Han, Z. (2013). Re-understanding of the variation laws of Quaternary groundwater in Beijing Plain. Geotechnical Investigation Surv. (5), 43–47. (in Chinese with English abstract).
Liu, Y. Z., Wu, Q., Lin, P., Liu, J. R., Xing, L. T., and Gao, Z. H. (2012). Restudy of the storage and migration model of the Quaternary groundwater in Beijing Plain area. Sci. China Earth Sci. 55 (7), 1147–1158. (in Chinese with English abstract). doi:10.1007/s11430-012-4417-0
LiuZhouLuoWangMcClainWang, S. Y. W. F. M. X., Luo, W., and McClain, M. E. (2022). A numerical assessment on the managed aquifer recharge to achieve sustainable groundwater development in Chaobai River area, Beijing, China. J. Hydrology 613, 128392. doi:10.1016/j.jhydrol.2022.128392
Lou, Z. H., Cheng, J. R., and Jin, A. M. (2006). Origin and evolution of the hydrodynamics in sedimentary basins-A case study of the Songliao basin. Acta Sedimentol. Sin. 24 (2), 193–201. (in Chinese with English abstract).
Lv, J. B. (2012). Geological background along the Banks of theYongding river. Urban Geol. 7 (1), 4–7. (in Chinese with English abstract).
Mallik, A. U., Lamb, E. G., and Rasid, H. (2001). Vegetation zonation among the microhabitats in a lacustrine environment: Analysis and application of belowground species trait patterns. Ecol. Eng. 18, 135–146. doi:10.1016/S0925-8574(01)00069-6
Mas-Pla, J., Menció, A., Bach, J., Soler, D., Zamorano, M., and Brusi, D. (2016). Trace element groundwater pollution hazard in regional hydrogeological systems (Empordà basin, NE Spain). Water Air Soil Pollut. 227, 218–240. doi:10.1007/s11270-016-2891-2
Naito, K., Fukahori, Y., He, P. M., Sakurai, W., Shimazaki, H., and Matsuhisa, Y. (1995). Oxygen and carbon isotope zonations of wall rocks around the Kamioka Pb-Zn skarn deposits, central Japan: Application to prospecting. J. Geochem. Explor. 54, 199–211. doi:10.1016/0375-6742(95)00044-5
Piao, S. L., Ciais, P., Huang, Y., Shen, Z. H., Peng, S. S., Li, J. S., et al. (2010). The impacts of climate change on water resources and agriculture in China. Nature 467, 43–51. doi:10.1038/nature09364
Robion, P., Kissel, C., de Lamotte, D. F., Lorand, J. P., and Guezou, J. C. (1997). Magnetic mineralogy and metamorphic zonation in the Ardennes Massif (France-Belgium). Tectonophysics 271, 231–248. doi:10.1016/S0040-1951(96)00268-5
Sarkar, A., and Guha, A. K. (1997). Pleistocene paleoclimatic zonation in northern Indian ocean as revealed from Globorotalia menardii abundance. Indian J. Geo-Marine Sci. 26, 84–87. doi:10.1007/s12594-012-0207-8
Shen, Z. L., and Wang, Y. X. (2002). Review and Outlook of water-rock interaction studies. Earth Science-Journal China Univ. Geosciences 27 (2), 127–133. (in Chinese with English abstract).
Song, X. F., Li, F. D., Yu, J. J., Tang, C. Y., Yang, C., Yang, X. C., et al. (2007). Characteristics of groundwater cycle using deuterium, oxygen-18 and hydrochemistry in Chaobai RIver Basin. Geogr. Res. 26 (1), 11–21. (in Chinese with English abstract). doi:10.3321/j.issn:1000-0585.2007.01.002
Straub, K. M., and Wang, Y. N. (2013). Influence of water and sediment supply on the long term evolution of alluvial fans and deltas: Statistical characterization of basin filling sedimentation patterns. J. Geophys. Res. Earth Surf. 118, 1602–1616. doi:10.1002/jgrf.20095
Wang, S. W., Kuo, Y. M., Kao, Y. H., Jang, C. S., Maji, S. K., Chang, F. J., et al. (2011). Influence of hydrological and hydrogeochemical parameters on arsenic variation in shallow groundwater of southwestern Taiwan. J. Hydrology 408, 286–295. doi:10.1016/j.jhydrol.2011.08.017
Wang, X. J., and Zhou, X. (2006). A preliminary analysis of the 14C age of groundwater in the Yongdinghe River Plain in beijing. Geol. Rev. 52 (2), 283–288. (in Chinese with English abstract). doi:10.3321/j.issn:0371-5736.2006.02.019
Wang, Y. X., Ma, T., and Li, Y. M. (1998). Geochemical modeling of water-rock interaction in the Liulin karst system, Shanxi province. Earth science-Journal China Univ. Geosciences 23 (5), 519–522. doi:10.1016/S0022-1694(01)00376-6(in Chinese with English abstract)
Wogelius, R. A., Fraser, D. G., Wall, G. R. T., and Grime, G. W. (1997). Trace element and isotopic zonation in vein calcite from the Mendip Hills, UK, with spatial-process correlation analysis. Geochimica Cosmochimica Acta 61, 2037–2051. doi:10.1016/S0016-7037(97)00065-3
Xiao, Y., Hao, Q. C., Zhang, Y. H., Zhu, Y. C., Yin, S. Y., Qin, L. M., et al. (2022). Investigating sources, driving forces and potential health risks of nitrate and fluoride in groundwater of a typical alluvial fan plain. Sci. Total Environ. 802, , 149909. doi:10.1016/j.scitotenv.2021.149909
Xiao, Y., Liu, K., Hao, Q. C., Li, Y. S., Xiao, D., and Zhang, Y. J. (2022). Occurrence, controlling factors and health hazards of fluoride-enriched groundwater in the lower Flood Plain of Yellow River, northern China. Expo. Health 14, 345–358. doi:10.1007/s12403-021-00452-2
Xiao, Y., Liu, K., Hao, Q. C., Xiao, D., Zhang, Y. C., Yin, S. Y., et al. (2022). Hydrogeochemical insights into the signatures, Genesis and sustainable perspective of nitrate enriched groundwater in the piedmont of Hutuo watershed, China. Catena 212, 106020. doi:10.1016/j.catena.2022.106020
Xun, H., Wang, J. S., Zhai, Y. Z., and Zheng, J. Q. (2011). Chemical characteristics and evolution of groundwater in the Yongding River alluvial fan of Beijing plain. Acta Geosci. Sin. 32 (3), 357–366. (in Chinese with English abstract). doi:10.1007/s12182-011-0118-0
Yang, W. C. (1986). The sedimentary characteristics and the environmental changes of the Taizhi River alluvial fan. Land & Resour. (2), 117–128. (in Chinese with English abstract).
Yu, J. J., Song, X. F., Liu, X. C., Yang, C., Tang, C. Y., Li, F. D., et al. (2007). A study of groundwater cycle in Yongding River Basin by using δD, δ18O and hydrochemical data. J. Nat. Resour. 22 (3), 415–423. (in Chinese with English abstract).
Yu, M., Wei, Y. S., Liu, J. G., Liu, P. F., Zhang, Z. M., Wei, W., et al. (2011). Impact of socioeconomic development on water resource and water enviroment of Yongding River in Beijing. Acta Sci. Circumstantiae 31 (9), 1817–1825. (in Chinese with English abstract). doi:10.13671/j.hjkxxb.2011.09.007
Zhai, Y. Z., Wang, J. S., Teng, Y. G., and Rui, Z. (2011). Variations of δD and δ18O in water in beijing and their Implications for the local water cycle. Resour. Sci. 31 (1), 92–97. doi:10.1007/s40333-015-0048-6(in Chinese with English abstract)
Zhai, Y. Z., Wang, J. S., Teng, Y. G., and Zuo, R. (2011). Chemical and isotopic characteristics of groundwater in the Yongding River groundwater system of Beijing, Plain. Acta Geosci. Sin. 32 (1), 101–106. doi:10.1007/s40333-015-0048-6(in Chinese with English abstract)
Zhai, Y. Z., Wang, J. S., Zheng, J. Q., and Huan, H. (2011). Evolution and driving forces of water consumed structure in beijing during the past 30 years. J. Nat. Resour. 26 (4), 635–643. doi:10.3390/su14159596(in Chinese with English abstract)
Zhai, Y. Z., Wang, J. S., and Zhou, (2013). J. Hydrochemical and isotopic markers of flow patterns and renewal Mode of groundwater in Chaobai RIver alluvial fan in beijing. J. Basic Sci. Eng. 21 (1), 32–44. (in Chinese with English abstract). doi:10.3969/j.issn.1005-0930.2013.01.004
Zhai, Y. Z., Wang, J. S., Zheng, J. Q., and Huan, H. (2011). Evolution and driving forces of water consumed structure in beijing during the past 30 years. J. Nat. Res. 26 (4), 635–643.
Zhai, Y. Z., Wang, J. S., Zuo, R., and Teng, Y. G. (2011). Progress in applications of groundwater ages in groundwater research. Earth Environ. 39 (1), 113–120. (in Chinese with English abstract).
Zhang, G. H., Liu, Z. P., Fei, Y. H., Lian, Y. L., Yan, M. J., and Wang, J. Z. (2010). The relationship between the distribution of irrigated Crops and the supply capability of regional water resources in north China plain. Acta Geosci. Sin. 31 (1), 17–22. (in Chinese with English abstract). doi:10.3724/SP.J.1231.2010.06586
Zhang, Z. H., Shi, D. H., Shen, Z. L., Zhong, Z. S., and Xue, Y. Q. (1997). Evolution and development of groundwater environment in north China Plain under human activities. Acta Geosci. Sin. 18 (4), 337–344. (in Chinese with English abstract).
Zhao, W., Lin, J., Guo, G. X., and Liu, Z. M. (2012). Development of layered monitoring and specialized inspection system of groundwater environment in beijing. South-to-North Water Divers. Water Sci. Technol. 10 (2), 83–87. (in Chinese with English abstract). doi:10.1186/gb-2010-11-s1-o10
Zheng, Y. J., Li, W. P., Wang, R. J., Liu, J. R., and Li, Y. M. (2012). Groundwater circulation and evolution characteristics in alluvial fan area of Chaobai River. Yangtze River 43 (15), 43–46. (in Chinese with English abstract). doi:10.1186/1744-859X-5-S1-S193
Keywords: age dating, conceptual model, groundwater renewability, hydrogeochemistry, Yongding River alluvial fan
Citation: Liu K, Qiao X, Wang S and Sun Y (2022) Understanding of groundwater evolution through chemical and age dating information in the Yongding River alluvial fan in Beijing. Front. Environ. Sci. 10:1056607. doi: 10.3389/fenvs.2022.1056607
Received: 29 September 2022; Accepted: 07 November 2022;
Published: 23 November 2022.
Edited by:
Yong Xiao, Southwest Jiaotong University, ChinaReviewed by:
Xuan Yu, Sun Yat-sen University, Zhuhai Campus, ChinaLinxian Huang, University of Jinan, China
Narsimha Adimalla, Chang’an University, China
Copyright © 2022 Liu, Qiao, Wang and Sun. This is an open-access article distributed under the terms of the Creative Commons Attribution License (CC BY). The use, distribution or reproduction in other forums is permitted, provided the original author(s) and the copyright owner(s) are credited and that the original publication in this journal is cited, in accordance with accepted academic practice. No use, distribution or reproduction is permitted which does not comply with these terms.
*Correspondence: Xiaojuan Qiao, cWlhb3hqMjAxMEAxNjMuY29t