- Department of Environmental Sciences, Faculty of Science, Alexandria University, Alexandria, Egypt
Indoor air pollution has been associated with adverse health effects. Sensitive people such as children spend much time at home. It is therefore important to know whether our children breath clean air inside their residences or not. Monitoring of PM10, PM2.5, TVOCs, CO, and CO2 concentrations was conducted for 24 h in 36 urban residences in Alexandria—Egypt, simultaneously outdoors and indoors (living rooms, bedrooms, and kitchens), during the summer season. During the COVID-19 pandemic, children spend 98% of their time at home (49% in living rooms, 47% in bedrooms, and 2% in kitchens). Results indicated that children are particularly exposed to high concentrations of PM10 (85.4 ± 18.7 μg/m3) and PM2.5 (57.2 ± 16.4 μg/m3) exceeding the WHO guidelines, which can be dangerous for their health. Outdoor sources were found to be a main contributor to the indoor levels of PM10, PM2.5, and CO in bedrooms and living rooms of the surveyed residences. Therefore, effective control measures to reduce outdoor air pollution can result in improved indoor air quality (IAQ). Living rooms showed the highest impact on the daily average children exposure to PM2.5, PM10, TVOCs, and CO with average contributions of 59%, 56%, 57%, and 61%, respectively. For CO2, bedrooms showed the largest contribution to the children’s average exposure with an average value of 49%, pointing to inadequate ventilation and small size of this type of room. Indoor occupants’ activities were found to considerably affect IAQ in the bedrooms and living rooms. The study also highlighted the importance of other determinants, such as occupancy and air exchange rate, on IAQ in these two microenvironments. This research provides the first data on children’s exposure to indoor air pollutants in urban residences in Egypt.
1 Introduction
Indoor air quality (IAQ) contributes greatly to the health and comfort of the occupants as people spend around 90% of their time in various indoor environments, especially in residential buildings (Schweizer et al., 2007; Odeh and Hussein, 2016). Vulnerable individuals such as children and elderly people can spend entire days in their dwellings and are at great risk as they may be exposed to different indoor pollutants released from various emission sources (World Health Organization, 2010; Mentese et al., 2020). Furthermore, indoor exposure can cause more harmful health effects and may constitute a greater threat than exposure to outdoor (ambient) air, as indoor air can be more polluted than that encountered outdoors (Faria et al., 2020; Gonzalo et al., 2022). Indoor airborne pollutants can be released inside residences from various occupants’ activities (e.g., smoking, cleaning, and cooking), use of electronic machines and consumer products (e.g., cleaning agents, cosmetics, adhesives, pesticides, and personal care products), or emission from building materials and furniture (Rivas et al., 2019). Outdoor air quality also has a great influence on residential IAQ as air pollutants can be transferred from outdoors to the indoor environment through ventilation (natural or mechanical) and infiltration (Chen and Zhao, 2011). IAQ may be largely affected by outdoor pollution level, indoor sources and activities, building characteristics, outdoor conditions, and human behavior (Vardoulakis et al., 2020; Abdel-Salam, 2021).
Numerous studies have assessed IAQ through monitoring multiple indoor air pollutants such as volatile organic compounds (VOCs), carbon dioxide (CO2), particulate matter (PM), ozone (O3), nitrogen dioxide (NO2), carbon monoxide (CO), sulfur dioxide (SO2), and radon (Rn) in residential buildings as these pollutants can pose a great risk to the inhabitants (Kozielska et al., 2020; Vardoulakis et al., 2020; Abdel-Salam, 2021). Exposure to indoor air pollution may lead to harmful outcomes, ranging from discomfort symptoms to the prevalence of cardiopulmonary disorders or even more serious health effects, such as cancers and premature deaths (Amoatey et al., 2020; Van Tran et al., 2020; World Health Organization, 2022a). This has led to the establishment of air quality standards to indoor environments by both national (governmental) and international organizations to protect human health by ensuring a better IAQ.
Children are among the most vulnerable and sensitive groups for the adverse health effects of indoor air pollution with the respiratory tract being the primary target for a wide range of chronic and acute effects (Nandasena et al., 2013). Children have a higher susceptibility to the potential health effects induced by air pollution than adults because they have higher metabolic rates and their immune and respiratory systems are immature and still developing (Schwartz, 2004; World Health Organization, 2018). Exposure to air pollutants during the process of early growth and development can greatly increase the risk of chronic diseases to the respiratory system such as asthma and allergic effects (Mendell, 2007; Clark et al., 2010). Also, children are more active than adults and breathe higher volumes of air relative to their body weight, thus exposing their lungs to more air pollutants (World Health Organization, 2005). In addition, adults typically use nasal breathing to filter out harmful particles from entering the respiratory tract, while children frequently use oral breathing with their breathing zone is much closer to the ground, resulting in more exposure and accumulation of air pollutants in the respiratory system (Bateson and Schwartz, 2008; Selgrade et al., 2008).
IAQ is critical for children’s health since they spend much of their time indoors, especially at home (from 55% to 69% of their time) and in schools (most of the daytime and up to 30% of their time) (Kawahara et al., 2012; Faria et al., 2020; Lizana et al., 2020). Therefore, many researchers focused their work on studying IAQ and children’s exposure in these indoor environments (Madureira et al., 2015; Canha et al., 2016; Abdel-Salam, 2019; Stamatelopoulou et al., 2019). Results of many recent exposure assessment studies have showed that homes and schools contribute most to the daily exposure of children to air pollutants (Faria et al., 2020; Lizana et al., 2020; Faria et al., 2022). Children’s exposure to air pollutants can be assessed through considering the time they spend in each indoor microenvironment as well as outdoors, the pollutants’ concentrations in each microenvironment, and the activities conducted by the children. High indoor pollution levels, especially in homes and schools, can cause negative effects on children’s health, cognitive development, growth and learning performance (Mendell et al., 2013; Shah et al., 2020). During the COVID-19 pandemic, stay-at-home orders were widely implemented by governments to minimize the risk of disease transmission and protect the public’s health (World Health Organization, 2021a). Mandatory stay-at-home orders and social distancing significantly reduced activities associated with the outbreak of coronavirus, such as population movement and direct contact with people outside the household (CDC, 2020). This has led to increased time spent at home, and hence longer exposure time of children to indoor air pollutants in their dwellings.
Although children spend most of their day indoors, information regarding their exposure to indoor air pollutants is very limited in developing countries. Only scarce data on IAQ are available in Egypt and therefore there are no national standards or health guidelines currently exist to assess IAQ in residential buildings. The current study aims to investigate IAQ in private urban residences located in Alexandria during the critical period of COVID-19 and to evaluate the daily average exposure of children (ranged from 5 to 10 years) to several indoor air pollutants including PM10 (particulate matter with aerodynamic diameter <10 μm), PM2.5 (particulate matter with aerodynamic diameter <2.5 μm), total volatile organic compounds (TVOCs), CO, and CO2 in the microenvironments frequented by them. The study also aims to determine the contribution of each microenvironment to the daily exposure of children as well as to identify the most potential factors affecting indoor air pollutants’ levels in the microenvironments of the largest contribution. This is the first study in Egypt attempting to assess children’s exposure to indoor air pollutants. This study can also replenish the lack of available data on IAQ in urban homes in Egypt and can provide useful data to stakeholders and regulatory authorities to develop Egyptian IAQ standards.
2 Materials and methods
2.1 Study design
The current study was conducted in the city of Alexandria which is located in the northern part of Egypt on the Mediterranean Sea. The area that Alexandria covers is about 2,679 km2 and it has a population of about 5.4 million (CAPMAS, 2020). Alexandria has a temperate Mediterranean climate with a warm humid, but not rainy, summer and a rainy cool winter. The ambient temperature varies from 12°C to 18°C with an average value of 14°C in the winter, while it varies from 25°C to 35°C with an average value of 28°C during the summer season. Outdoor and indoor monitoring of multiple air pollutants (PM2.5, PM10, TVOCs, CO and CO2) were performed in the summer season of 2021 (from early July till end of September) in 36 residences located in an urban residential area called Miami (31°16′06"N 29°59′50"E), which is situated in El-Montazah district. The location of the monitoring sites is shown in Figure 1. Eligible residences were selected based on the presence of a child in the age from 5 to 10 years of any gender. The recruitment process began early before conducting the study and it was performed through personal contacts with the householders of 80 residences that were randomly selected and distributed in the study area. Of these, only 36 approved to participate due to the coronavirus outbreak and its restrictions, knowing that no honoraria were paid for participation. The surveyed residences are private urban flats located in different floors of multi-storey residential buildings that had no attached garages. Distances of the selected residences to major traffic roads varied from 10 to 500 m. It is necessary to note that traffic data for major roads surrounding the selected residences were not available in the traffic department.
Indoor measurements of target air pollutants were carried out for 24 h in the bedrooms, living rooms, and kitchens of all selected households, whereas outdoor air measurements of the studies pollutants were collected concurrently and continuously from the balconies of the monitored rooms. The monitoring period for each residence was 7 days, where air measurements in the studied residences were collected along 12 weeks, with 3 homes being sampled simultaneously. The investigated residences were ventilated only naturally in living rooms and bedrooms to introduce outside air by opening windows. For domestic kitchens, the ventilation during cooking activity was either mechanically through applying kitchen exhaust systems or naturally by opening doors and windows. Open-plan kitchens were not available in the selected homes that relied on natural gas for domestic cooking. Gas stoves were used in all domestic kitchens for cooking which was performed 3 times per day. In all selected residences, the wooden or tile floors of the monitored bedrooms and living rooms were all covered with carpets. In addition, no pets were found in the surveyed households.
2.2 Questionnaire and children’s time-activity diary
In the present study, the surveyed households were all occupied during the measurement campaign, and the occupants were asked to keep their usual activities and typical habits to obtain measurements that reflect real-life conditions. The parents in each surveyed residence were also requested to complete a questionnaire to collect the required information related to household characteristics, human occupancy, and indoor occupants’ activities. The major characteristics of the surveyed households as well as occupants’ information and activities are given in Table 1. Additionally, a daily activity diary was developed and applied to 36 children (20 boys and 16 girls) to identify the microenvironments frequented by them, daily activities undertaken by the children in these microenvironments, and to quantify the time spent in each microenvironment at a time interval of 30 min. The daily activities of the participated children in the residential microenvironments were mainly eating, watching TV, sleeping, playing, and reading. Children’s daily average exposure (E) was calculated by integrating the time-activity data along the day with the concentrations of air pollutants measured in each microenvironment, using the following equation (Eq. 1) (Ott, 1982; Jai Devi et al., 2013):
Where Cj is the average pollutant concentration measured in the microenvironment (j) and Tj is the time spent in the microenvironment (j). The total number of microenvironments is (m), so that:
Furthermore, the contribution of each microenvironment (in %) to the daily exposure of children to the studied air pollutants was calculated by the following equation (Eq. 2) (Cunha-Lopes et al., 2019; Lizana et al., 2020), to show the importance of each microenvironment to the daily exposure of children:
2.3 Monitoring of air pollutants
During the measurement campaign, direct-reading instruments were used to simultaneously monitor indoor (kitchens, bedrooms, and living rooms) and outdoor concentrations of PM2.5, PM10, TVOCs, CO, and CO2 in all surveyed residences. Air monitoring was conducted at about 1 m above the floor which is corresponding to the children’s breathing zone. The locations of the air monitors in the residential microenvironments were chosen carefully to obtain representative measurements as well as to minimize any annoyance to the occupants. The monitoring period covered seven consecutive days for each residence, aiming at collecting representative data from typical daily occupancy routines in all residential microenvironments. The applied air monitors were checked for any potential mechanical problems through daily visits to each selected site. Furthermore, paired air monitors were co-located in the laboratory for parallel testing in order to obtain consistent results before every monitoring campaign.
Continuous 24 h monitoring of PM2.5 and PM10 concentrations with a time resolution of 1 min was carried out both indoors and outdoors at each selected household using a photometric light-scattering instrument known as the DustTrak aerosol monitor (model 8533; TSI Inc. MN, United States) at a flow rate of 3 L/min (resolution: 0.001 mg/m3, concentration range: 0.001–150 mg/m3, particle size range: 0.1–15 μm, and accuracy: ± 5%). The DustTrak monitors were factory calibrated once/year before conducting the field campaign to ensure reliable measurements. Also, zero filter checks were conducted to the dust monitors before using them. During each measurement campaign, PM2.5 and PM10 concentrations measured by the DustTrak monitors were corrected against the simultaneous and co-located gravimetric air samplers (Wallace et al., 2011; Javed and Guo, 2021). Therefore, Personal Environmental Monitors (PEMs) (model 200, MSP Co., Minneapolis, MN) were applied to simultaneously collect PM2.5 and PM10 samples on polytetrafluoroethylene (PTFE) filters for subsequent gravimetric analysis according to a procedure described elsewhere (Abdel-Salam, 2021). This process confirmed a good agreement between PEMs and DustTrak monitors. The PM10 and PM2.5 results of the DustTrak monitors were corrected by using the reference gravimetric method to provide accurate concentration values.
Simultaneous continuous measurements of TVOCs, CO, and CO2 were taken place outdoors and indoors (24 h/day, 1 min time resolution) for 7 days at each surveyed home. CO and CO2 concentrations were monitored by using the Q-Trak monitors (model 8551; TSI Inc. Shoreview, MN, United States). The Q-Trak monitor contains an electro-chemical sensor for measuring CO levels (resolution: 1 ppm, concentration range: 0–500 ppm, and accuracy: ± 3%). Furthermore, the monitor uses the non-dispersive infrared (NDIR) mechanism for monitoring CO2 concentrations (resolution: 1 ppm, concentration range: 0–5,000 ppm, and accuracy: ± 3%). For TVOCs monitoring, the DirectSense II monitor (GrayWolf Sensing Solution, Shelton, United States) was applied. The GrayWolf monitor is equipped with a Photo Ionization Detector (PID) (resolution: 0.001 ppm, concentration range 0–20 ppm, and accuracy: ± 1%). The gaseous monitors applied in this study were calibrated once/year by the respective manufacturer before the measurement campaign. Moreover, zero calibration was also carried out to all gaseous monitors before each monitoring period.
2.4 Air exchange rates
Air exchange rate (AER) is the rate at which outdoor air replaces indoor air within a confined space (i.e., air changes per hour) and is expressed in h−1. AER within a naturally ventilated residence may differ from room to room according to occupants’ behavior such as window opening (Bekö et al., 2016). In this study, AER was measured in the bedrooms and living rooms of all residences by the tracer gas decay method (ASTM, 2011), using sulfur hexafluoride (SF6) as the tracer gas. During this process, a small volume of the tracer gas was injected into the room, allowed to mix thoroughly to ensure a uniform concentration, and then the SF6 concentrations were measured during the decay process at known times. The SF6 measurements were carried out with the Innova 1412i Photoacoustic Multi-gas Monitor (Luma-Sense Technologies Inc. Santa Clara, CA, United States). The decay rate of the tracer gas was then used to estimate the average AER for living rooms and bedrooms (ISO, 2000).
2.5 Statistical analysis
Statistical analysis was performed by IBM SPSS Statistics for Windows, version 25 (IBM Corp. Armonk, NY, United States) and Microsoft Office Excel 2019 (Microsoft Inc.), at a significance level of 5% (p < 0.05 is statistically significant). The data were checked for normality by the Shapiro-Wilk test. Pearson correlation test was used to evaluate the strength of associations between pollutants’ concentrations in different microenvironments as well as between indoor pollutants’ levels and various determinant factors. For variables with two independent groups, the t-test was applied to evaluate the statistical differences in indoor concentrations of air pollutants.
3 Results and discussion
3.1 Indoor air pollutants’ concentrations
Descriptive statistics for daily outdoor and indoor concentrations of PM2.5, PM10, TVOCs, CO, and CO2 are presented in Table 2. The studied residential microenvironments (livingrooms, bedrooms, and kitchens) showed significant variations in the indoor concentrations of the studied air pollutants. The results confirmed that daily average levels of PM10, PM2.5, TVOCs, and CO in bedrooms were significantly lower than those observed in living rooms (p < 0.01). In contrast, daily average levels of CO2 in bedrooms were significantly higher (p < 0.002) than those observed in living rooms. This could be due to the smaller size of bedrooms (Table 1) and lower AERs measured in bedrooms compared to those recorded in living rooms. In the surveyed residences, the average AER in bedrooms (1.4 ± 0.4 h−1; range: 0.5–1.9 h−1; median value: 1.5 h−1) was significantly lower (p < 0.0001) than that measured in living rooms (2.6 ± 0.5 h−1; range: 1.1–3.5 h−1; median value: 2.6 h−1) over the monitoring period. This could be due to the relatively longer ventilation times in living rooms than bedrooms as occupants tend to close doors and windows when they are sleeping. This was confirmed by the occupants of the selected homes. For domestic kitchens, average concentrations of the studied air pollutants were significantly higher than those found in bedrooms (p < 0.01) and living rooms (p < 0.05), which implies considerable contribution from cooking activity. The small volume of almost 70% of the monitored kitchens and the lack of exhaust systems in most kitchens (Table 1) could result in inadequate ventilation and thus higher levels of combustion-generated air pollutants in these kitchens (Sun and Wallace, 2021; Zhang et al., 2021).
As there are no national IAQ standards currently exist for indoor air pollutants, an evaluation of IAQ was conducted by comparing the measured indoor pollutants’ concentrations with international guidelines and limit values of well-known organizations. The daily average concentrations of PM2.5 and PM10 measured in the residential microenvironments of the surveyed residences exceeded the World Health Organization (WHO) daily guideline values for PM2.5 and PM10 of 15 μg/m3 and 45 μg/m3, respectively (World Health Organization, 2021b). This is probably due to significant contribution from external sources and indoor occupants’ activities. On the other hand, the daily average concentrations of CO and CO2 recorded in the residential microenvironments were compared to the WHO daily guideline of 3.5 ppm (World Health Organization, 2021b) and the American Society of Heating, Refrigerating and Air-Conditioning Engineers (ASHRAE) Standard 62.1, respectively. The ASHRAE Standard 62.1 recommends maintaining indoor CO2 levels no greater than 700 ppm above its outdoor concentrations (ASHRAE, 2013). No exceedances were found for average indoor CO and CO2 levels in all monitored homes. Furthermore, the daily average TVOCs concentrations in the monitored living rooms and bedrooms were lower than the Leadership in Energy and Environmental Design (LEED) daily limit value of 500 μg/m3 (LEED, 2016). For domestic kitchens, only 6 residences (almost 17% of the studied residences) exceeded the daily LEED limit value.
Results of other published studies on IAQ that were carried out elsewhere in homes relied mainly on natural ventilation during the summer season are shown in Table 3. The results of this research (Table 2) were compared to those reported in other countries for evaluating levels of indoor air pollution in various urban residential areas, even though indoor air pollutants’ concentrations could differ considerably according to many factors (e.g., building characteristics, geographical location, intensity of emission sources, and the measurement conditions). For PM, average indoor levels of PM2.5 and PM10 observed in the living rooms of this research were greater than those found in previous studies (Wigzell et al., 2000; Byun et al., 2010; Héroux et al., 2010; Lai et al., 2010; Pekey et al., 2010; Cattaneo et al., 2011; MacNeill et al., 2014; Tunno et al., 2015; Tong et al., 2018), except only in Agra, India (Massey et al., 2012). For air measurements performed in the bedrooms, average indoor PM10 levels measured in this study were greater than those observed in Korea (Byun et al., 2010) and in United States (Simons et al., 2007), whereas average indoor levels of PM2.5 measured in the current study were lower than those recorded in Baltimore, United States (Simons et al., 2007) and Lanzhou, China (Li et al., 2016). Furthermore, average indoor levels of PM10 found in the domestic kitchens in this research were less than those reported in Delhi, India (Kulshreshtha et al., 2008), while average PM2.5 concentrations recorded in the kitchens in this research were higher than those reported in Oxford, UK (Wigzell et al., 2000), Lanzhou, China (Li et al., 2016), and Delhi, India (Kulshreshtha et al., 2008).
For the other studied pollutants, average levels of CO and TVOCs observed in the living rooms of this research were lower than those reported in Lodi, Italy (Cattaneo et al., 2011) and Sapporo, Japan (Saijo et al., 2004), respectively. On contrary, average concentrations of CO2 observed in the living rooms of this research were higher than those found in Lodi, Italy (Cattaneo et al., 2011). For measurements performed in the bedrooms, average indoor CO and TVOCs levels observed in the current research were less than those recorded in United States (Pickett and Bell, 2011) but were greater than those obtained from UK (Raw et al., 2004). Moreover, average levels of CO2 and TVOCs recorded in the bedrooms of this study were higher than those observed in United States (Pickett and Bell, 2011) and Korea (Kwon et al., 2015), respectively. Also, average levels of CO and CO2 found in the kitchens in this research were less than those reported in Delhi, India (Kulshreshtha et al., 2008), whereas average CO concentrations observed in the kitchens of the current research were greater than those reported in England, United Kingdom (Raw et al., 2004).
3.2 Relationship between IAQ and outdoor pollution level
One critical aspect influencing IAQ is the exchange between indoor air and the outdoor environment. The indoor/outdoor (I/O) ratios were calculated for the studied pollutants to evaluate the effect of ambient air pollution as well as the strength of indoor emission sources on residential indoor air quality (Chen and Zhao, 2011; Rivas et al., 2019). Statistical results of the I/O ratios of PM2.5, PM10, TVOCs, CO, and CO2 for the residential microenvironments of all selected homes are presented as box plots in Figures 2A,B, respectively. The top and bottom edges of the vertical lines represent the maximum and minimum values, respectively. The upper, middle, and lower lines of each box represent the 25th, 50th (median), and 75th percentiles, respectively. The dot point inside each box represents the average value of the data. The average I/O ratios for PM10 were 0.72 ± 0.09 (range: 0.56–0.91; median value: 0.72) in living rooms, 0.49 ± 0.08 (range: 0.38–0.70; median value: 0.49) in bedrooms, and 0.95 ± 0.36 (range: 0.41–1.86; median value: 0.86) in kitchens. The average ambient levels of PM10 were higher than indoor concentrations by 100% in living rooms and bedrooms, and almost 58% in kitchens. This could be attributed to the various outdoor emission sources of PM10 and its low infiltration from outdoors (Mannan and Al-Ghamdi, 2021). For PM2.5, the average I/O ratios were 0.82 ± 0.16 (range: 0.57–1.12; median value: 0.79) in living rooms, 0.50 ± 0.13 (range: 0.26–0.78; median value: 0.47) in bedrooms, and 1.04 ± 0.37 (range: 0.44–1.77; median value: 0.98) in kitchens. The average ambient levels of PM2.5 were higher than indoor values by about 78% in living rooms, 100% in bedrooms, and 53% in kitchens. For the studied microenvironments, the average I/O ratios of fine PM fraction were higher than those of coarse PM10. This is probably due to the lower deposition velocities of PM2.5 together with its higher infiltration from outdoor sources when compared to PM10 (Thatcher and Layton, 1995; Chen and Zhao, 2011; Bo et al., 2017).
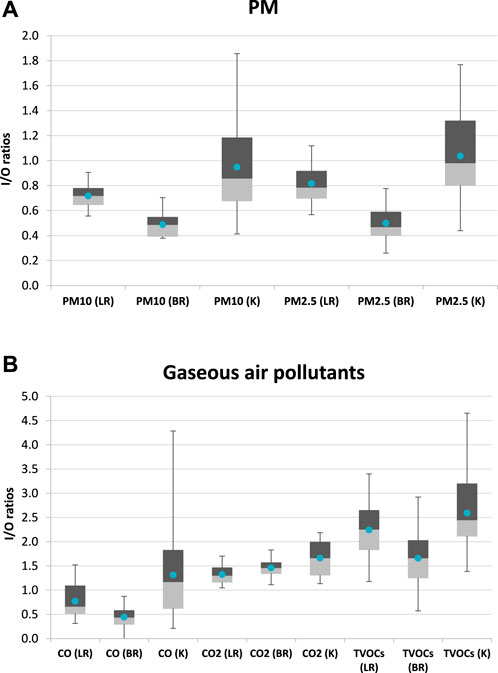
FIGURE 2. I/O ratios of target air pollutants: (A) PM2.5 and PM10, (B) TVOCs, CO, and CO2 for kitchens (K), bedrooms (BR), and living rooms (LR) of the studied households.
In the studied households, the average I/O ratios of CO were 0.77 ± 0.34 (range: 0.31–1.52; median value: 0.67) in living rooms, 0.44 ± 0.24 (range: 0.00–0.87; median value: 0.44) in bedrooms, and 1.31 ± 0.89 (range: 0.21–4.29; median value: 1.18) in kitchens. The daily average ambient levels of CO were higher than indoor concentrations by about 72% in living rooms, 100% in bedrooms, and 47% in kitchens. For CO2, the average I/O ratios were 1.32 ± 0.18 (range: 1.05–1.70; median value: 1.31) in living rooms, 1.46 ± 0.18 (range: 1.11–1.83; median value: 1.46) in bedrooms, and 1.66 ± 0.35 (range: 1.13–2.19; median value: 1.67) in kitchens. The results showed that average I/O ratios for CO2 were more than 1 in all indoor microenvironments. This indicates considerable contribution from indoor sources, such as human respiration and combustion processes, and this agrees with previous studies (Abdel-Salam, 2015; Gall et al., 2016). Additionally, the average I/O ratios of TVOCs were 2.24 ± 0.60 (range: 1.18–3.40; median value: 2.26) in living rooms, 1.66 ± 0.61 (range: 0.57–2.92; median value: 1.67) in bedrooms, and 2.59 ± 0.76 (range: 1.39–4.65; median value: 2.45) in kitchens. The daily average ambient levels of TVOCs were higher than indoor concentrations in bedrooms by only 19%, while average outdoor TVOCs levels did not exceed their corresponding values in both living rooms and kitchens. As CO2, this also suggests considerable contribution from indoor emission sources and this agrees with previous studies (Kwon et al., 2015; Mečiarová et al., 2017). Such higher indoor than outdoor levels of CO2 and TVOCs in the studied microenvironments imply inadequate ventilation (Stamatelopoulou et al., 2019; Sahu and Gurjar, 2020). For all investigated residences, average I/O ratios of the monitored pollutants were found relatively greater in the domestic kitchens than in the living rooms and bedrooms. This indicates considerable contribution from domestic cooking as well as poor ventilation in the kitchen environments (Sun and Wallace, 2021).
Associations between indoor and outdoor levels of the studied pollutants were determined to assess how outdoor pollutants’ levels influence the indoor values. Strong associations were observed between ambient and indoor (living room) concentrations of PM2.5 (r = 0.84, p < 0.01), PM10 (r = 0.74, p < 0.01), and CO (r = 0.79, p < 0.01). This is consistent with other IAQ studies (Yin et al., 2019; Ścibor et al., 2019). In comparison, no statistically significant associations were found between outdoor and indoor (living room) levels of TVOCs and CO2. Additionally, significant associations were recorded between ambient and bedroom concentrations of PM2.5 (r = 0.73, p < 0.01), PM10 (r = 0.56, p < 0.01), and CO (r = 0.66, p < 0.01); whereas no statistically significant associations were observed between outdoor and bedroom concentrations of TVOCs and CO2. The results indicate that outdoor sources are considered a main contributor to the indoor living room and bedroom levels of PM10, PM2.5, and CO, and that the occupants open windows even when the outdoor air is polluted (i.e., during rush hours). However, stronger outdoor/indoor associations were found in living rooms than in bedrooms for the two PM size fractions and CO concentrations. This could be attributed to the significantly higher air exchange rates in living rooms when compared to bedrooms (p < 0.0001), and thus more infiltration of polluted air and traffic emissions from outdoors into living rooms (Cattaneo et al., 2011; Langer et al., 2016). Therefore, effective control strategies to reduce outdoor air pollution can result in improved IAQ. In kitchens of the selected residences, no significant associations were observed between indoor and outdoor concentrations of target pollutants. This implies that indoor concentrations of studied air pollutants recorded in the kitchens were affected mainly by indoor emissions from cooking rather than outdoor sources.
3.3 Assessment of children’s exposure to air pollutants
People spend significant fractions (almost 65%) of their daily time at home, and even more for children and elderly (Brasche and Bischof, 2005; Morawska et al., 2017). Due to the COVID-19 pandemic, the time people spent at home increased significantly for all age groups (Toyosada et al., 2021; Bhat et al., 2022). Currently, there is no clear path toward ending the pandemic as successive waves of COVID-19 infections are generated by new coronavirus variants (World Health Organization, 2022b). Generally, the governments encourage people to stay at home to reduce human contact particularly when there is a notable increase in the number of new coronavirus infections. This, in turn, increases the importance of assessing exposure to indoor air pollutants in residential buildings particularly for vulnerable people. In the present study, the daily average children exposure was estimated in the surveyed residences through performing microenvironmental monitoring of all studied pollutants in each residence together with using time-activity diary for each participant to quantify the time spent in each microenvironment. Figure 3 presents the average time (in hours) spent by each child in each microenvironment and the results are shown for a typical period of 24 h. The time-activity pattern of children showed that they spent in average 98% of their time at home, where they spent approximately 49% in living rooms, 47% in bedrooms, and 2% in kitchens. Undoubtedly, this indicates the significance of residential IAQ for children’s exposure and health during the outbreak of COVID-19.
For all surveyed residences, the daily average children exposure (E) to PM2.5, PM10, TVOCs, CO, and CO2 was evaluated using Eq. 1, and the results are shown in Table 4. The results indicated that the daily average children exposure to PM2.5 and PM10 exceeded the WHO air quality guideline levels. This implies the importance of adopting proper control actions to reduce exposure of children to particulate matter. On the other hand, the daily average exposure of children to TVOCs, CO, and CO2 was below the LEED, WHO, and ASHRAE standards, respectively. Moreover, the average contribution of indoor microenvironments in the studied residences to the daily children exposure to all studied pollutants was calculated using Eq. 2 and the results (expressed in%) are shown as box plots in Figure 4. Living rooms showed the largest contribution to the daily exposure of children to PM10 (56%), PM2.5 (59%), TVOCs (57%), and CO (61%). This is due to the long time spent by children in living rooms, in addition to more contribution from both outdoor sources and indoor human activities with more occupancy in living rooms than in bedrooms (Table 1). In contrast, bedrooms showed the largest impact on the daily average children exposure to CO2 with an average contribution of 49%. This could be attributed to the smaller size of this type of room combined with lower AERs when compared to living rooms and this is consistent with other studies (Almeida-Silva et al., 2014; Stamatelopoulou et al., 2019; Kozielska et al., 2020). Therefore, it is necessary to increase natural ventilation in bedrooms especially when the density of traffic is low.
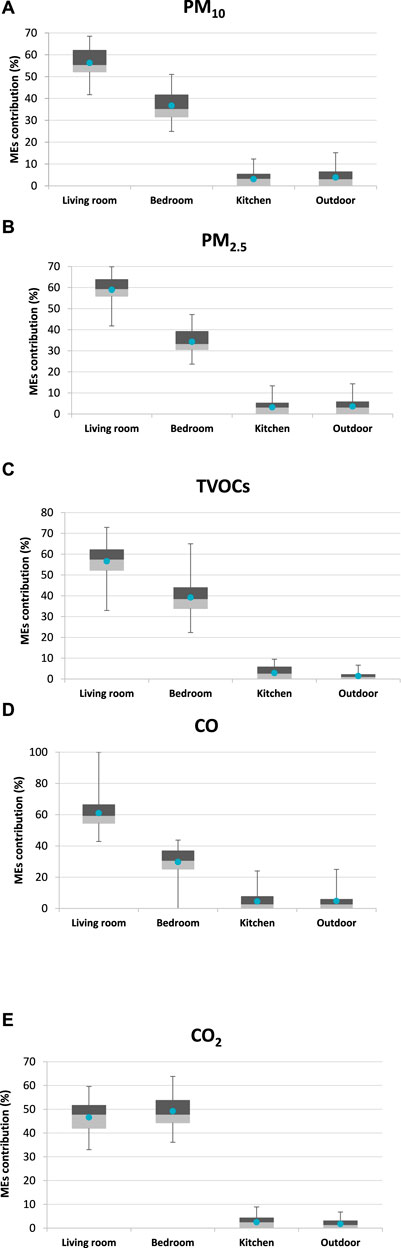
FIGURE 4. Average contribution (%) of the studied microenvironments (MEs) to daily average children exposure to the studied pollutants: (A) PM10, (B) PM2.5, (C) TVOCs, (D) CO, and (E) CO2.
3.4 Relationship between IAQ and other influencing factors
In the current study, results indicated that the most contributing microenvironments to the daily average children exposure to the studied pollutants were living rooms and bedrooms as children spent 96% of their time in these two microenvironments. Table 5 shows the correlation between indoor pollutants’ levels measured in bedrooms and living rooms and many potential influencing factors. Furthermore, associations between pollutants’ concentrations in bedrooms and living rooms of the surveyed dwellings were evaluated. Statistically significant associations were observed between bedroom and living room concentrations of PM2.5 (r = 0.73, p < 0.01), PM10 (r = 0.65, p < 0.01), TVOCs (r = 0.60, p < 0.01), CO (r = 0.82, p < 0.01), and CO2 (r = 0.67, p < 0.01), suggesting that the two microenvironments were affected by similar pollution sources, and this is consistent with other studies (Jones et al., 2007; Kozielska et al., 2020; Vardoulakis et al., 2020).
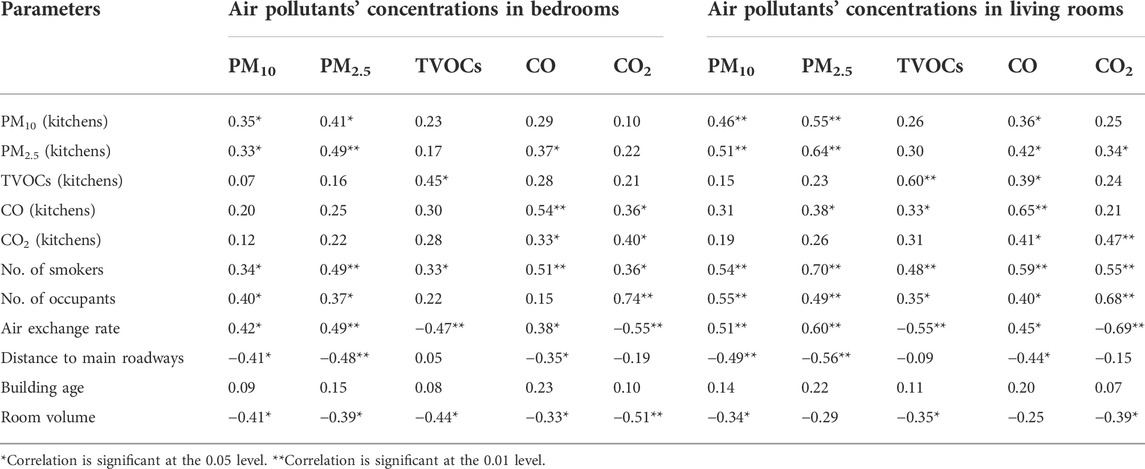
TABLE 5. Correlations between indoor pollutants’ levels in bedrooms and living rooms and possible determinant factors.
3.4.1 Factors related to indoor activities and human occupancy
Indoor human activities such as cleaning, smoking, and cooking are routinely undertaken in households and can greatly influence residential IAQ (World Health Organization, 2010; Rivas et al., 2019). Cooking is an important domestic activity that generates several combustion products during stove operation and may significantly increase indoor pollutants’ levels in the residential microenvironments (Buonanno et al., 2009; Madureira et al., 2015; Mullen et al., 2016). Indeed, statistically significant associations were found between indoor levels of studied pollutants measured in the domestic kitchens of the surveyed dwellings and their corresponding levels recorded in bedrooms and living rooms (Table 5). This indicates considerable contribution from the cooking activity to the studied pollutants in bedrooms and living rooms. The absence of mechanical ventilation systems in 21 kitchens of the studied residences (Table 1) and the reliance only on natural ventilation in these kitchens can result in insufficient ventilation in the kitchen environment and thus accumulation of combustion products and cooking fumes during domestic cooking operations that can lead to the transfer of air pollutants to the other residential microenvironments (Pinto and Viegas, 2013; Sun and Wallace, 2021; Zhang et al., 2021). As expected, kitchens provided with mechanical ventilation systems showed considerable reduction in indoor (bedroom and living room) levels of PM10 (average = 89.9 vs. 110.8 μg/m3 for living rooms; p < 0.004 and 60.3 vs. 76.2 μg/m3 for bedrooms; p < 0.008), PM2.5 (average = 61.1 vs. 79.8 μg/m3 for living rooms; p < 0.007, and 36.9 vs. 49.7 μg/m3 for bedrooms; p < 0.006), TVOCs (average = 278.9 vs. 371.1 μg/m3 for living rooms; p < 0.001, and 213.2 vs. 269.9 μg/m3 for bedrooms; p < 0.05), CO (average = 0.6 vs. 1.2 ppm for living rooms; p < 0.001, and 0.4 vs. 0.7 ppm for bedrooms; p < 0.002), and CO2 (average = 563.7 vs. 658.9 ppm for living rooms; p < 0.001, and 632.5 vs. 708.8 ppm for bedrooms; p < 0.001) when compared to kitchens relied only on natural ventilation.
Another major indoor activity that can negatively affect residential IAQ is indoor smoking (Héroux et al., 2010; Langer et al., 2016; Ni et al., 2020). In the present study, smoking was recorded in living rooms of only eight residences (Table 1). However, smoking residences showed significantly higher indoor (bedroom and living room) levels of PM10 (average = 118.0 vs. 93.1 μg/m3 for living rooms; p < 0.002, and 81.7 vs. 62.6 μg/m3 for bedrooms; p < 0.003), PM2.5 (average = 95.2 vs. 61.4 μg/m3 for living rooms; p < 0.000, and 59.2 vs. 37.4 μg/m3 for bedrooms; p < 0.000), TVOCs (average = 396.9 vs. 294.6 μg/m3 for living rooms; p < 0.001, and 328.5 vs. 206.4 μg/m3 for bedrooms; p < 0.001), CO (average = 1.4 vs. 0.7 ppm for living rooms; p < 0.001, and 0.8 vs. 0.4 ppm for bedrooms; p < 0.002), and CO2 (average = 706.4 vs. 573.9 ppm for living rooms; p < 0.000, and 740.8 vs. 642.4 ppm for bedrooms; p < 0.003) compared to those in nonsmoking ones, and this agrees with other studies (Romagnoli et al., 2016; Stamatelopoulou et al., 2019; Faria et al., 2020; Ni et al., 2020). Moreover, statistically stronger correlations were recorded between number of smokers and pollutants’ levels in living rooms than in bedrooms (Table 5) as smoking mainly occurred in living rooms.
The study also considered cleaning as an indoor activity that is commonly undertaken in the dwellings. Home cleaning was performed mainly by vacuuming and sweeping in the studied residences, but it varied in its frequency from a daily activity observed in 16 homes to an irregular activity in 20 homes (Table 1). For PM, daily cleaned residences showed significantly higher indoor (bedroom and living room) levels of PM10 (average = 112.2 vs. 87.7 μg/m3 for living rooms; p < 0.001, and 73.6 vs. 61.5 μg/m3 for bedrooms; p < 0.02) and PM2.5 (average = 80.2 vs. 59.8 μg/m3 for living rooms; p < 0.002, and 47.7 vs. 37.8 μg/m3 for bedrooms; p < 0.05) than their corresponding values in irregularly cleaned ones. Other studies have also reported similar results as more frequent cleaning may increase resuspension of deposited dust from various indoor surfaces (Corsi et al., 2008; Knibbs et al., 2012; Vicente et al., 2020). For gaseous pollutants, only indoor TVOCs concentrations were significantly influenced by regular cleaning as a result of the frequent use of cleaning agents, which is in line with previous studies (Bari et al., 2015; Stamatelopoulou et al., 2019). Significantly higher TVOCs levels were only observed in living rooms of daily cleaned residences than those found in residences of irregular cleaning (average = 360.1 vs. 283.1 μg/m3; p < 0.002).
Human occupancy is an important indoor factor that can influence IAQ as higher number of occupants is typically related to increased indoor activities and more pollutants’ emissions (Mjörnell et al., 2019; Abdel-Salam, 2021). In the current study, the number of occupants ranged from 1 to 3 in the children’s bedrooms, and from 3 to 6 in the living rooms, as shown in Table 1. For PM, stronger correlations were realized between number of occupants and indoor concentrations of both PM size fractions in living rooms than in bedrooms, as living rooms had higher occupancy than bedrooms. This is consistent with previous studies that have linked higher occupancy and crowding with increased resuspension of deposited particles from internal surfaces during walking and other physical activities of the residents (Thatcher and Layton, 1995; Qian et al., 2014; Lewis et al., 2018; Faria et al., 2020). For gaseous air pollutants, strong association was only found between number of occupants and levels of CO2 in both living rooms and bedrooms. Previous studies have also reported similar findings as occupants exhale CO2 during respiration (Persily and de Jonge, 2017; Abdel-Salam, 2022).
3.4.2 Factors related to household characteristics
IAQ is influenced by building characteristics, such as ventilation, building age, distance from major roadways, presence of attached garages, etc. (Morawska et al., 2011; Singleton et al., 2017). Ventilation is considered a key factor of IAQ. In this study, statistically significant associations were observed between AER and indoor PM10, PM2.5, and CO concentrations in living rooms and, to a lesser extent, in bedrooms (Table 5). This could be attributed to the relatively higher AERs in living rooms compared to those measured in bedrooms (as discussed in Section 3.2), where outdoor air is a major source of PM and CO from traffic emissions. In contrast, statistically significant, but negative, associations were recorded between AER and indoor levels of TVOCs and CO2 in bedrooms and living rooms. This indicates that ventilation is an important control tool for indoor TVOCs and CO2. Previous studies have also revealed that increased ventilation can reduce indoor TVOCs and CO2 concentrations (Park and Ikeda, 2006; Kauneliene et al., 2016; Mečiarová et al., 2017). The present study also investigated the effect of nearby main roadways on indoor pollutants’ concentrations. Table 5 shows stronger negative associations between distance to major roadways and PM2.5, PM10, and CO levels in living rooms than those observed in bedrooms. Again, this could be attributed to higher ventilation rates and thus more infiltration of traffic emissions to living rooms than bedrooms. Many previous studies have also confirmed similar observations that proximity of naturally ventilated buildings to main traffic roads can negatively affect IAQ, especially for air pollutants of outdoor origin (Tong et al., 2016; Hu et al., 2017; Vardoulakis et al., 2020).
Home age and room volume are other building-related characteristics that can also affect IAQ (Mannan and Al-Ghamdi, 2021). Old buildings may contain leaks and cracks in their structure which can result in more infiltration of polluted air from outdoors to indoor environments (Lai et al., 2012; Li et al., 2017). In the current study, average age of the surveyed residences was only 8 years (range: 3–20 years) (Table 1). Table 5 shows no statistically significant associations between home age and indoor air pollutants’ concentrations recorded in bedrooms and living rooms. The effect of home age was not significant in the current study as most of the investigated buildings were built recently and no cracks were observed in their structure. On the other hand, significant negative associations were recorded between volume of bedrooms and pollutants’ concentrations measured in bedrooms. In comparison, significant negative, but weaker, associations were only seen between volume of living rooms and indoor concentrations of PM10, TVOCs, and CO2 measured in living rooms. Many studies have also showed that room volume is inversely associated with indoor pollutants’ levels where more dilution occurs in rooms of larger volume (Nasir and Colbeck, 2013; Urso et al., 2015; Kozielska et al., 2020).
Floor level (FL) and recent renovation performed to the studied residences were also considered in this study. Almost 39% of the studied homes (14 homes) were present at ground and first FL, whereas the remaining homes (22 homes) were at higher FL (from the 2nd to the 7th FL) (Table 1). There were no statistically significant differences in the indoor (bedroom and living room) pollutants’ concentrations between residences at lower FL (≤1st FL) and those at higher FL (>1st FL). Similarly, latest renovation performed to the studied residences had no significant effect on indoor levels of target air pollutants in living rooms and bedrooms. For all surveyed residences, there were no recent indoor renovations performed in the last year prior to monitoring, as shown in Table 1, and this is probably due to the outbreak of coronavirus and its restrictions.
4 Conclusion
In the current study, children were found to spend in average 98% of their time at home (49% in living rooms, 47% in bedrooms, and 2% in kitchens). Obviously, this shows the importance of residential IAQ for children’s exposure and health during the spread of coronavirus. The results also showed that children in the studied residences are particularly exposed to high PM2.5 and PM10 levels exceeding the WHO air quality guidelines which can be dangerous for their health. This indicates the importance of taking effective control actions to reduce PM concentrations and children’s exposure in the residential microenvironments. Children’s exposure to gaseous air pollutants (TVOCs, CO, and CO2) was also evaluated and no exceedances were found when compared to the international standards. Furthermore, results indicated that the microenvironments that contribute most to the daily average children exposure to indoor pollutants were bedrooms and living rooms. Living rooms showed the largest impact on the daily average children exposure to PM2.5, PM10, TVOCs, and CO with average contributions of 59%, 56%, 57%, and 61%, respectively. This could be due to the large number of occupants in the living rooms and thus more indoor activities conducted in this microenvironment, as well as the high ingress of the outdoor air pollutants through the doors and windows which were frequently opened when the living rooms were occupied. On the other hand, bedrooms showed the highest impact on the children’s daily exposure to CO2 with an average value of 49%. This could be due to inadequate ventilation and small volume of this type of room. Therefore, it is important to improve ventilation in bedrooms particularly during periods of low traffic densities. In addition, stronger associations were found between indoor and outdoor concentrations of both PM size fractions and CO in living rooms than in bedrooms. This is possibly due to the significantly higher AERs in living rooms than bedrooms, and thus more migration of vehicular emissions and air pollutants originating outdoors into the living rooms. Preventive measures should therefore be focused on any outdoor sources of PM10, PM2.5, and CO surrounding the monitored homes. In comparison, no significant associations were recorded between outdoor and indoor levels of TVOCs and CO2 in these two microenvironments. This implies the importance of indoor sources for these two gaseous air pollutants.
This study also evaluated the significance of various determinant factors which may influence levels of studied pollutants in the residential microenvironments of the largest contribution to the daily average children exposure. Indoor activities of the inhabitants (e.g., cooking of food, indoor smoking, and cleaning activities) significantly increased indoor pollutants’ levels in living rooms and bedrooms. Therefore, children’s exposure can be reduced by increasing ventilation in domestic kitchens through applying appropriate exhaust fans or range hoods, preventing indoor smoking, and keeping the children away from the residence during extensive cleaning activities. Additionally, other factors such as human occupancy, ventilation rate, proximity to major roadways, and room volume were also found to significantly influence indoor concentrations of air pollutants in the surveyed households.
The study demonstrated a strong dependence of residential IAQ on the external environment and thus any intervention practices for reducing indoor emissions, though helpful, would be insufficient without effective control strategies to reduce ambient air pollution. However, it is recommended to use air purifiers to clean indoor air and improve IAQ, or to apply a properly designed heating, ventilation, and air conditioning (HVAC) systems instead of the widespread use of natural ventilation. This research can provide useful information and recommendations to improve residential IAQ and protect the children’s health. Also, the study can contribute to the development of IAQ standards in Egypt.
Data availability statement
The raw data supporting the conclusion of this article will be made available by the authors, without undue reservation.
Ethics statement
Ethical review and approval was not required for the study on human participants in accordance with the local legislation and institutional requirements. Written informed consent from the participants was not required to participate in this study in accordance with the national legislation and the institutional requirements.
Author contributions
The author confirms being the sole contributor of this work and has approved it for publication.
Conflict of interest
The author declares that the research was conducted in the absence of any commercial or financial relationships that could be construed as a potential conflict of interest.
Publisher’s note
All claims expressed in this article are solely those of the authors and do not necessarily represent those of their affiliated organizations, or those of the publisher, the editors and the reviewers. Any product that may be evaluated in this article, or claim that may be made by its manufacturer, is not guaranteed or endorsed by the publisher.
References
Abdel-Salam, M. M. (2019). Investigation of indoor air quality at urban schools in Qatar. Indoor Built Environ. 28, 278–288. doi:10.1177/1420326x17700948
Abdel-Salam, M. M. (2015). Investigation of PM2.5 and carbon dioxide levels in urban homes. J. Air Waste Manag. Assoc. 65, 930–936. doi:10.1080/10962247.2015.1040138
Abdel-Salam, M. M. (2021). Outdoor and indoor factors influencing particulate matter and carbon dioxide levels in naturally ventilated urban homes. J. Air Waste Manag. Assoc. 71, 60–69. doi:10.1080/10962247.2020.1834009
Abdel-Salam, M. M. (2022). Relationship between residential indoor air quality and socioeconomic factors in two urban areas in Alexandria, Egypt. Build. Environ. 207, 108425. doi:10.1016/j.buildenv.2021.108425
Almeida-Silva, M., Wolterbeek, H. T., and Almeida, S. M. (2014). Elderly exposure to indoor air pollutants. Atmos. Environ. X. 85, 54–63. doi:10.1016/j.atmosenv.2013.11.061
Amoatey, P., Omidvarborna, H., Baawain, M., Al-Mamun, A., Bari, A., and Kindzierski, W. (2020). Association between human health and indoor air pollution in the gulf cooperation council (GCC) countries: A review. Rev. Environ. Health 35, 157–171. doi:10.1515/reveh-2019-0065
ASHRAE (2013). Ventilation for acceptable indoor air quality. Atlanta, GA, USA: American Society of Heating, Refrigerating and Air-Conditioning Engineers.
ASTM (2011). Standard test method for determining air change in a single zone by means of a tracer gas dilution. West Conshohocken, PA: American Society for Testing Materials.
Bari, M. A., Kindzierski, W. B., Wheeler, A. J., Héroux, M. È., and Wallace, L. A. (2015). Source apportionment of indoor and outdoor volatile organic compounds at homes in Edmonton, Canada. Build. Environ. 90, 114–124. doi:10.1016/j.buildenv.2015.03.023
Bateson, T., and Schwartz, J. (2008). Children’s response to air pollutants. J. Toxicol. Environ. Health A 71, 238–243. doi:10.1080/15287390701598234
Bekö, G., Gustavsen, S., Frederiksen, M., Bergsøe, N., Kolarik, B., Gunnarsen, L., et al. (2016). Diurnal and seasonal variation in air exchange rates and interzonal airflows measured by active and passive tracer gas in homes. Build. Environ. 104, 178–187. doi:10.1016/j.buildenv.2016.05.016
Bhat, M. A., Eraslan, F. N., Awad, A., Malkoç, S., Üzmez, Ö. Ö., Döğeroğlu, T., et al. (2022). Investigation of indoor and outdoor air quality in a University campus during COVID-19 lock down period. Build. Environ. 219, 109176. doi:10.1016/j.buildenv.2022.109176
Bo, M., Salizzoni, P., Clerico, M., and Buccolieri, R. (2017). Assessment of indoor-outdoor particulate matter air pollution: A review. Atmosphere 8, 136. doi:10.3390/atmos8080136
Brasche, S., and Bischof, W. (2005). Daily time spent indoors in German homes–baseline data for the assessment of indoor exposure of German occupants. Int. J. Hyg. Environ. Health 208, 247–253. doi:10.1016/j.ijheh.2005.03.003
Buonanno, G., Morawska, L., and Stabile, L. (2009). Particle emission factors during cooking activities. Atmos. Environ. X. 43, 3235–3242. doi:10.1016/j.atmosenv.2009.03.044
Byun, H., Bae, H., Kim, D., Shin, H., and Yoon, C. (2010). Effects of socioeconomic factors and human activities on children’s PM10 exposure in inner-city households in Korea. Int. Arch. Occup. Environ. Health 83, 867–878. doi:10.1007/s00420-010-0523-5
Canha, C., Mandin, C., Ramalho, O., Wyart, G., Ribéron, J., Dassonville, C., et al. (2016). Assessment of ventilation and indoor air pollutants in nursery and elementary schools in France. Indoor Air 26, 350–365. doi:10.1111/ina.12222
CAPMAS (2020). Egypt statistical yearbook, Cairo, Egypt: Central Agency for Public Mobilization and Statistics.
Cattaneo, A., Peruzzo, C., Garramone, G., Urso, P., Ruggeri, R., Carrer, P., et al. (2011). Airborne particulate matter and gaseous air pollutants in residential structures in Lodi province, Italy. Indoor Air 21, 489–500. doi:10.1111/j.1600-0668.2011.00731.x
CDC (2020). Implementation of mitigation strategies for community COVID-19 transmission. Atlanta, GA: US. Department of Health and Human Services, CDC. Available At: https://www.cdc.gov/coronavirus/2019-ncov/community/community-mitigation.html (Accessed on July 3, 2022).
Chen, C., and Zhao, B. (2011). Review of relationship between indoor and outdoor particles: I/O ratio, infiltration factor and penetration factor. Atmos. Environ. X. 45, 275–288. doi:10.1016/j.atmosenv.2010.09.048
Clark, N. A., Demers, P. A., Karr, C. J., Koehoorn, M., Lencar, C., Tamburic, L., et al. (2010). Effect of early life exposure to air pollution on development of childhood asthma. Environ. Health Perspect. 118, 284–290. doi:10.1289/ehp.0900916
Corsi, R. L., Siegel, J. A., and Chiang, C. (2008). Particle resuspension during the use of vacuum cleaners on residential carpet. J. Occup. Environ. Hyg. 5, 232–238. doi:10.1080/15459620801901165
Cunha-Lopes, I., Martins, V., Faria, T., Correia, C., and Almeida, S. M. (2019). Children’s exposure to sized-fractioned particulate matter and black carbon in an urban environment. Build. Environ. 155, 187–194. doi:10.1016/j.buildenv.2019.03.045
Faria, T., Martins, V., Canha, N., Diapouli, E., Manousakas, M., Fetfatzis, P., et al. (2022). Assessment of children's exposure to carbonaceous matter and to PM major and trace elements. Sci. Total Environ. 807, 151021. doi:10.1016/j.scitotenv.2021.151021
Faria, T., Martins, V., Correia, C., Canha, N., Diapouli, E., Manousakas, M., et al. (2020). Children’s exposure and dose assessment to particulate matter in Lisbon. Build. Environ. 171, 106666. doi:10.1016/j.buildenv.2020.106666
Gall, E., Cheung, T., Luhung, I., Schiavon, S., and Nazaroff, W. (2016). Real-time monitoring of personal exposures to carbon dioxide. Build. Environ. 104, 59–67. doi:10.1016/j.buildenv.2016.04.021
Gonzalo, F. d. A., Griffin, M., Laskosky, J., Yost, P., and González-Lezcano, R. A. (2022). Assessment of indoor air quality in residential buildings of New England through actual data. Sustainability 14, 739. doi:10.3390/su14020739
Héroux, M. E., Clark, N., Van Ryswyk, K., Mallick, R., Gilbert, N. L., Harrison, I., et al. (2010). Predictors of indoor air concentrations in smoking and non-smoking residences. Int. J. Environ. Res. Public Health 7, 3080–3099. doi:10.3390/ijerph7083080
Hu, J., Li, N., Lv, Y., Liu, J., Xie, J., and Zhang, H. (2017). Investigation on indoor air pollution and childhood allergies in households in six Chinese cities by subjective survey and field measurements. Int. J. Environ. Res. Public Health 14, 979. doi:10.3390/ijerph14090979
ISO (2000). Thermal performance of buildings. Determination of air change in buildings. Tracer gas dilution method; ISO 12569. Geneva, Switzerland: International Organization for Standardization.
Jai Devi, J., Gupta, T., Jat, R., and Tripathi, S. N. (2013). Measurement of personal and integrated exposure to particulate matter and co-pollutant gases. Environ. Sci. Pollut. Res. 20, 1632–1648. doi:10.1007/s11356-012-1179-3
Javed, W., and Guo, B. (2021). Performance evaluation of real-time DustTrak monitors for outdoor particulate mass measurements in a desert environment. Aerosol Air Qual. Res. 21, 200631. doi:10.4209/aaqr.200631
Jones, J., Stick, S., Dingle, P., and Franklin, P. (2007). Spatial variability of particulates in homes: Implications for infant exposure. Sci. Total Environ. 376, 317–323. doi:10.1016/j.scitotenv.2007.01.060
Kauneliene, V., Prasauskas, T., Krugly, E., Stasiulaitiene, I., Ciuzas, D., Seduikyte, L., et al. (2016). Indoor air quality in low energy residential buildings in Lithuania. Build. Environ. 108, 63–72. doi:10.1016/j.buildenv.2016.08.018
Kawahara, J., Tanaka, S., Tanaka, C., Aoki, Y., and Yonemoto, J. (2012). Daily inhalation rate and time-activity/location pattern in Japanese preschool children. Risk Anal. 32, 1595–1604. doi:10.1111/j.1539-6924.2011.01776.x
Knibbs, L. D., Congrong, H., Duchaine, C., and Morawska, L. (2012). Vacuum cleaner emissions as a source of indoor exposure to airborne particles and bacteria. Environ. Sci. Technol. 46, 534–542. doi:10.1021/es202946w
Kozielska, B., Mainka, A., Zak, M., Kaleta, D., and Mucha, W. (2020). Indoor air quality in residential buildings in Upper Silesia, Poland. Build. Environ. 177, 106914. doi:10.1016/j.buildenv.2020.106914
Kulshreshtha, P., Khare, M., and Seetharaman, P. (2008). Indoor air quality assessment in and around urban slums of Delhi city, India. Indoor Air 18, 488–498. doi:10.1111/j.1600-0668.2008.00550.x
Kwon, J. H., Kim, E., Chang, M. H., Park, E. A., Hong, Y. C., Ha, M., et al. (2015). Indoor total volatile organic compounds exposure at 6 months followed by atopic dermatitis at 3 years in children. Pediatr. Allergy Immunol. 26, 352–358. doi:10.1111/pai.12393
Lai, A. C., Fung, J. L., Li, M., and Leung, K. Y. (2012). Penetration of fine particles through rough cracks. Atmos. Environ. X. 60, 436–443. doi:10.1016/j.atmosenv.2012.06.053
Lai, S. C., Ho, K. F., Zhang, Y. Y., Lee, S. C., Huang, Y., and Zou, S. C. (2010). Characteristics of residential indoor carbonaceous aerosols: A case study in guangzhou, pearl river delta region. Aerosol Air Qual. Res. 10, 472–478. doi:10.4209/aaqr.2010.05.0037
Langer, S., Ramalho, O., Derbez, M., Riberon, J., Kirchner, S., and Mandin, C. (2016). Indoor environmental quality in French dwellings and building characteristics. Atmos. Environ. X. 128, 82–91. doi:10.1016/j.atmosenv.2015.12.060
LEED (2016). Building design and construction guide. Washington, DC: The United States Green Building Council USGBC.
Lewis, R. D., Ong, K. H., Emo, B., Kennedy, J., Kesavan, J., and Elliot, M. (2018). Resuspension of house dust and allergens during walking and vacuum cleaning. J. Occup. Environ. Hyg. 15, 235–245. doi:10.1080/15459624.2017.1415438
Li, A., Ren, T., Yang, C., Lv, W., and Zhang, F. (2017). Study on particle penetration through straight, L, Z and wedge-shaped cracks in buildings. Build. Environ. 114, 333–343. doi:10.1016/j.buildenv.2016.12.024
Li, T., Cao, S., Fan, D., Zhang, Y., Wang, B., Zhao, X., et al. (2016). Household concentrations and personal exposure of PM2.5 among urban residents using different cooking fuels. Sci. Total Environ. 548–549, 6–12. doi:10.1016/j.scitotenv.2016.01.038
Lizana, J., Almeida, S. M., Serrano-Jiménez, A., Becerra, J. A., Gil-Báez, M., Barrios-Padura, A., et al. (2020). Contribution of indoor microenvironments to the daily inhaled dose of air pollutants in children. The importance of bedrooms. Build. Environ. 183, 107188. doi:10.1016/j.buildenv.2020.107188
MacNeill, M., Kearney, J., Wallace, L., Gibson, M., Héroux, M. E., Kuchta, J., et al. (2014). Quantifying the contribution of ambient and indoor-generated fine particles to indoor air in residential environments. Indoor Air 24, 362–375. doi:10.1111/ina.12084
Madureira, J., Paciencia, I., Ramos, E., Barros, H., Pereira, C., Teixeira, J. P., et al. (2015). Children's health and indoor air quality in primary schools and homes in Portugal-study design. J. Toxicol. Environ. Health A 78, 915–930. doi:10.1080/15287394.2015.1048926
Mannan, M., and Al-Ghamdi, S. G. (2021). Indoor air quality in buildings: A comprehensive review on the factors influencing air pollution in residential and commercial structure. Int. J. Environ. Res. Public Health 18, 3276. doi:10.3390/ijerph18063276
Massey, D., Kulshrestha, A., Masih, J., and Taneja, A. (2012). Seasonal trends of PM10, PM5.0, PM2.5 and PM1.0 in indoor and outdoor environments of residential homes located in North-Central India. Build. Environ. 47, 223–231. doi:10.1016/j.buildenv.2011.07.018
Mečiarová, L., Vilčeková, S., Burdová, E., and Kiselák, J. (2017). Factors effecting the total volatile organic compound (TVOC) concentrations in Slovak households. Int. J. Environ. Res. Public Health 14, 1443. doi:10.3390/ijerph14121443
Mendell, M., Eliseeva, E., Davies, M., Spears, M., Lobscheid, A., Fisk, W., et al. (2013). Association of classroom ventilation with reduced illness absence: A prospective study in California elementary schools. Indoor Air 23, 515–528. doi:10.1111/ina.12042
Mendell, M. J. (2007). Indoor residential chemical emissions as risk factors for respiratory and allergic effects in children: A review. Indoor Air 17, 259–277. doi:10.1111/j.1600-0668.2007.00478.x
Mentese, S., Mirici, N., Elbir, T., Palaz, E., Mumcuoğlu, D., Cotuker, O., et al. (2020). A long-term multi-parametric monitoring study: Indoor air quality (IAQ) and the sources of the pollutants, prevalence of sick building syndrome (SBS) symptoms, and respiratory health indicators. Atmos. Pollut. Res. 11, 2270–2281. doi:10.1016/j.apr.2020.07.016
Mjörnell, K., Johansson, D., and Bagge, H. (2019). The effect of high occupancy density on IAQ, moisture conditions and energy use in apartments. Energies 12, 4454. doi:10.3390/en12234454
Morawska, L., Ayoko, G. A., Bae, G. N., Buonanno, G., Chao, C. Y. H., Clifford, S., et al. (2017). Airborne particles in indoor environment of homes, schools, offices and aged care facilities: The main routes of exposure. Environ. Int. 108, 75–83. doi:10.1016/j.envint.2017.07.025
Morawska, L., Mengersen, K., Wang, H., Tayphasavanh, F., Darasavong, K., and Holmes, N. S. (2011). Pollutant concentrations within households in Lao PDR and association with housing characteristics and occupants’ activities. Environ. Sci. Technol. 45, 882–889. doi:10.1021/es102294v
Mullen, N. A., Li, J., Russell, M. L., Spears, M., Less, B. D., and Singer, B. C. (2016). Results of the California healthy homes indoor air quality study of 2011–2013: Impact of natural gas appliances on air pollutant concentrations. Indoor Air 26, 231–245. doi:10.1111/ina.12190
Nandasena, S., Wickremasinghe, A., and Sathiakumar, N. (2013). Indoor air pollution and respiratory health of children in the developing world. World J. Clin. Pediatr. 2, 6–15. doi:10.5409/wjcp.v2.i2.6
Nasir, Z. A., and Colbeck, I. (2013). Particulate pollution in different housing types in a UK suburban location. Sci. Total Environ. 445, 165–176. doi:10.1016/j.scitotenv.2012.12.042
Ni, Y. M., Shi, G. C., and Qu, J. M. (2020). Indoor PM2.5, tobacco smoking and chronic lung diseases: A narrative review. Environ. Res. 181, 108910–10. doi:10.1016/j.envres.2019.108910
Odeh, I., and Hussein, T. (2016). Activity pattern of urban adult students in an eastern Mediterranean society. Int. J. Environ. Res. Public Health 13, 960–965. doi:10.3390/ijerph13100960
Ott, W. R. (1982). Concepts of human exposure to air pollution. Environ. Int. 7, 179–196. doi:10.1016/0160-4120(82)90104-0
Park, J. S., and Ikeda, K. (2006). Variations of formaldehyde and VOC levels during 3 years in new and older homes. Indoor air 16, 129–135. doi:10.1111/j.1600-0668.2005.00408.x
Pekey, B., Bozkurt, Z., Pekey, H., Dogan, G., Zararsız, A., Efe, N., et al. (2010). Indoor/outdoor concentrations and elemental composition of PM10/PM2.5 in urban/industrial areas of Kocaeli City, Turkey. Indoor Air 20, 112–125. doi:10.1111/j.1600-0668.2009.00628.x
Persily, A., and de Jonge, L. (2017). Carbon dioxide generation rates for building occupants. Indoor Air 27, 868–879. doi:10.1111/ina.12383
Pickett, A., and Bell, M. (2011). Assessment of indoor air pollution in homes with infants. Int. J. Environ. Res. Public Health 8, 4502–4520. doi:10.3390/ijerph8124502
Pinto, M., and Viegas, J. (2013). The influence of ventilation systems on domestic gas appliances: An experimental study. Build. Environ. 69, 1–13. doi:10.1016/j.buildenv.2013.07.008
Qian, J., Peccia, J., and Ferro, A. (2014). Walking-induced particle resuspension in indoor environments. Atmos. Environ. X. 89, 464–481. doi:10.1016/j.atmosenv.2014.02.035
Raw, G. J., Coward, S. K., Brown, V. M., and Crump, D. R. (2004). Exposure to air pollutants in English homes. J. Expo. Sci. Environ. Epidemiol. 14 (1), S85–S94. doi:10.1038/sj.jea.7500363
Rivas, I., Fussell, J., Kelly, F., and Querol, X. (2019). “Indoor sources of air pollutants,” in Indoor air pollution. Editors R. E. Hester, and R. M. Harrison (London, UK: Royal Society of Chemistry), 1–34.
Romagnoli, P., Balducci, C., Perilli, M., Vichi, F., Imperiali, A., and Cecinato, A. (2016). Indoor air quality at life and work environments in Rome, Italy. Environ. Sci. Pollut. Res. 23, 3503–3516. doi:10.1007/s11356-015-5558-4
Sahu, V., and Gurjar, B. R. (2020). Spatial and seasonal variation of air quality in different microenvironments of a technical University in India. Build. Environ. 185, 107310. doi:10.1016/j.buildenv.2020.107310
Saijo, Y., Kishi, R., Sata, F., Katakura, Y., Urashima, Y., Hatakeyama, A., et al. (2004). Symptoms in relation to chemicals and dampness in newly built dwellings. Int. Arch. Occup. Environ. Health 77, 461–470. doi:10.1007/s00420-004-0535-0
Schwartz, J. (2004). Air pollution and children’s health. Pediatrics 113, 1037–1043. doi:10.1542/peds.113.s3.1037
Schweizer, C., Edwards, R., Bayer-Oglesby, L., Gauderman, W., Ilacqua, V., Jantunen, M., et al. (2007). Indoor time–microenvironment–activity patterns in seven regions of Europe. J. Expo. Sci. Environ. Epidemiol. 17, 170–181. doi:10.1038/sj.jes.7500490
Ścibor, M., Balcerzak, B., Galbarczyk, A., Targosz, N., and Jasienska, G. (2019). Are we safe inside? Indoor air quality in relation to outdoor concentration of PM10 and PM2.5 and to characteristics of homes. Sustain. Cities Soc. 48, 101537. doi:10.1016/j.scs.2019.101537
Selgrade, M., Plopper, C., Gilmour, M., Conolly, R., and Foos, B. (2008). Assessing the health effects and risks associated with children's inhalation exposures—asthma and allergy. J. Toxicol. Environ. Health A 71, 196–207. doi:10.1080/15287390701597897
Shah, S., Jeong, K. S., Park, H., Hong, Y-C., Kim, Y., Kim, B., et al. (2020). Environmental pollutants affecting children’s growth and development: Collective results from the MOCEH study, a multi-centric prospective birth cohort in Korea. Environ. Int. 137, 105547. doi:10.1016/j.envint.2020.105547
Simons, E., Curtin-Brosnan, J., Buckley, T., Breysse, P., and Eggleston, P. A. (2007). Indoor environmental differences between inner city and suburban homes of children with asthma. J. Urban Health 84, 577–590. doi:10.1007/s11524-007-9205-3
Singleton, R., Salkoski, A. J., Bulkow, L., Fish, C., Dobson, J., Albertson, L., et al. (2017). Housing characteristics and indoor air quality in households of Alaska Native children with chronic lung conditions. Indoor Air 27, 478–486. doi:10.1111/ina.12315
Stamatelopoulou, A., Asimakopoulos, D. N., and Maggos, T. (2019). Effects of PM, TVOCs and comfort parameters on indoor air quality of residences with young children. Build. Environ. 150, 233–244. doi:10.1016/j.buildenv.2018.12.065
Sun, L., and Wallace, L. A. (2021). Residential cooking and use of kitchen ventilation: The impact on exposure. J. Air Waste Manag. Assoc. 71, 830–843. doi:10.1080/10962247.2020.1823525
Thatcher, T. L., and Layton, D. W. (1995). Deposition, re-suspension, and penetration of particles within a residence. Atmos. Environ. X. 29, 1487–1497. doi:10.1016/1352-2310(95)00016-r
Tong, X., Wang, B., Dai, W., Cao, J., Ho, S., Kwok, T., et al. (2018). Indoor air pollutant exposure and determinant factors controlling household air quality for elderly people in Hong Kong. Air Qual. Atmos. Health 11, 695–704. doi:10.1007/s11869-018-0576-2
Tong, Z., Chen, Y., Malkawi, A., Adamkiewicz, G., and Spengler, J. (2016). Quantifying the impact of traffic-related air pollution on the indoor air quality of a naturally ventilated building. Environ. Int. 89-90, 138–146. doi:10.1016/j.envint.2016.01.016
Toyosada, K., Nakagawa, C., Mitsunaga, T., and Kose, H. (2021). Effect of the COVID‐19 pandemic on residential water use behavior in Japan. Water 13, 3129. doi:10.3390/w13213129
Tunno, B. J., Shields, K. N., Cambal, L., Tripathy, S., Holguin, F., Lioy, P., et al. (2015). Indoor air sampling for fine particulate matter and black carbon in industrial communities in Pittsburgh. Sci. Total Environ. 536, 108–115. doi:10.1016/j.scitotenv.2015.06.117
Urso, P., Cattaneo, A., Garramone, G., Peruzzo, C., Cavallo, D., and Carrer, P. (2015). Identification of particulate matter determinants in residential homes. Build. Environ. 86, 61–69. doi:10.1016/j.buildenv.2014.12.019
Van Tran, V., Park, D., and Lee, Y-C. (2020). Indoor air pollution, related human diseases, and recent trends in the control and improvement of indoor air quality. Int. J. Environ. Res. Public Health 17, 2927. doi:10.3390/ijerph17082927
Vardoulakis, S., Giagloglou, E., Steinle, S., Davis, A., Sleeuwenhoek, A., Galea, K., et al. (2020). Indoor exposure to selected air pollutants in the home environment: A systematic review. Int. J. Environ. Res. Public Health 17, 8972. doi:10.3390/ijerph17238972
Vicente, E. D., Vicente, A. M., Evtyugina, M., Calvo, A. I., Oduber, F., Alegre, C. B., et al. (2020). Impact of vacuum cleaning on indoor air quality. Build. Environ. 180, 107059. doi:10.1016/j.buildenv.2020.107059
Wallace, L. A., Wheeler, A. J., Kearney, J., Van Ryswyk, K., You, H., Kulka, R. H., et al. (2011). Validation of continuous particle monitors for personal, indoor, and outdoor exposures. J. Expo. Sci. Environ. Epidemiol. 21, 49–64. doi:10.1038/jes.2010.15
Wigzell, E., Kendall, M., and Nieuwenhuijsen, M. J. (2000). The spatial and temporal variation of particulate matter within the home. J. Expo. Sci. Environ. Epidemiol. 10, 307–314. doi:10.1038/sj.jea.7500091
World Health Organization (2018). Air pollution and child health: Prescribing clean air: Summary. Geneva: World Health Organization. Available At: https://apps.who.int/iris/handle/10665/275545 (Accessed on June 30, 2022).
World Health Organization (2021a). Coronavirus disease (COVID-19). Available At: https://www.who.int/health-topics/coronaviruse#tab=tab_1 (Accessed on July 2, 2022).
World Health Organization (2005). “Effects of air pollution on children’s health and development–A review of the evidence,” in WHO regional Office for europe (Denmark: WHO Press).
World Health Organization (2010). “Guidelines for indoor air quality: Selected pollutants,” in WHO regional Office for europe (Copenhagen, Denmark: WHO Press).
World Health Organization (2022a). Household air pollution and health. Available At: https://www.who.int/en/news-room/fact-sheets/detail/household-air-pollution-and-health (Accessed on June 28, 2022).
World Health Organization (2022b). Strategic preparedness, readiness and response plan to end the global COVID-19 emergency in 2022. Geneva: World Health Organization.
World Health Organization (2021b). WHO global air quality guidelines. Particulate matter (PM2.5and PM10), ozone, nitrogen dioxide, sulfur Dioxide and carbon monoxide. Geneva: World Health Organization.
Yin, H., Liu, C., Zhang, L., Li, A., and Ma, Z. (2019). Measurement and evaluation of indoor air quality in naturally ventilated residential buildings. Indoor Built Environ. 28, 1307–1323. doi:10.1177/1420326x19833118
Keywords: children, indoor air pollution, homes, exposure, COVID-19, microenvironments
Citation: Abdel-Salam MMM (2022) Assessment of children’s exposure to air pollutants in urban residences during the COVID-19 pandemic. Front. Environ. Sci. 10:1050623. doi: 10.3389/fenvs.2022.1050623
Received: 22 September 2022; Accepted: 18 October 2022;
Published: 28 October 2022.
Edited by:
Barbara Kozielska, Silesian University of Technology, PolandReviewed by:
Worradorn Phairuang, Kanazawa University, JapanPatricio Perez, University of Santiago, Chile
Copyright © 2022 Abdel-Salam. This is an open-access article distributed under the terms of the Creative Commons Attribution License (CC BY). The use, distribution or reproduction in other forums is permitted, provided the original author(s) and the copyright owner(s) are credited and that the original publication in this journal is cited, in accordance with accepted academic practice. No use, distribution or reproduction is permitted which does not comply with these terms.
*Correspondence: Mahmoud M. M. Abdel-Salam, mahmoud_asalam@alexu.edu.eg