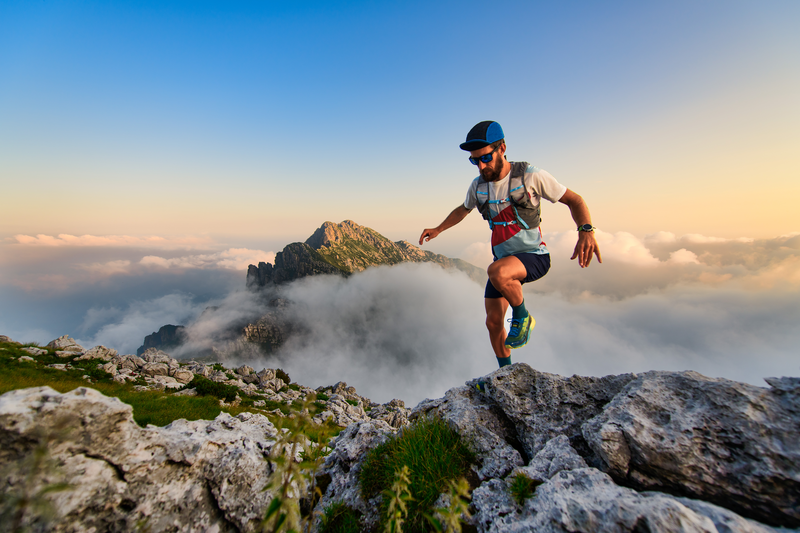
94% of researchers rate our articles as excellent or good
Learn more about the work of our research integrity team to safeguard the quality of each article we publish.
Find out more
ORIGINAL RESEARCH article
Front. Environ. Sci. , 04 January 2023
Sec. Toxicology, Pollution and the Environment
Volume 10 - 2022 | https://doi.org/10.3389/fenvs.2022.1048345
This article is part of the Research Topic Hazardous Pollutants in the Environment: Analysis, Assessment and Remediation View all 10 articles
The Nanwan village, a green ecological village in Taizhou city, is used as a recycling area (recycling for heavy metal) for electronic circuit boards and as crushing and stacking sites of waste circuit boards for nearly 20 years from 1980s to 2000s. At present, although the e-waste recycling activities in Taizhou city have been effectively controlled, and many areas polluted by the e-dismantling activities have been gradually remediated except Nanwan village. Nanwan village seems to have been forgotten for its special geographical location, which has attracted our attention because of its ecological and food safety issues. Accordingly, the content of polychlorinated biphenyls (PCBs) and polybrominated diphenyl ethers (PBDEs) in the surface soil around the ruins and four edible agricultural crops were investigated. The main conclusions are as follows: among the four dismantling ruins and surrounding soil samples investigated, the content of Σ20PCBs in vegetable field topsoil of 2(20) is 1,321.3 ± 132.1 μg kg−1; the content of Σ8PBDEs in the paddy soil of 3(1S) is 7,216.9 ± 232.0 μg kg−1; biological toxicity events are likely to occur frequently in 2(20) and 3(1S). PCBs and PBDEs have both horizontal diffusion in distance and vertical diffusion in depth. The lifetime carcinogenic and non-carcinogenic risks of PCBs and PBDEs are at a low risk level, except for the non-carcinogenic risk of PCBs for children in 3(1S). The lifetime carcinogenic and non-carcinogenic risks of PCBs and PBDEs in the edible parts of garlic, ginger, mung beans, and oranges were all at acceptable or negligible levels.
The disassembly activities of electronic products in Taizhou City, Zhejiang Province, began in the 1970s. The initial family workshop until today’s metal recycling industry base spanned for 40 years (Fu et al., 2012). In Taizhou, people once used e-waste to recycle useful metals for profit, and then threw the garbage to the land and rivers under their feet. The electronic products in Taizhou are mainly disassembled in Fengjiang and Jinqing town in Luqiao District, and in Zeguo and Wenqiao town in Wenling City. Nanwan village is a small village located south of Qingyu village in Wenqiao Town near the seaside of Yueqing Bay. It is surrounded by mountains on three sides and faces the sea on the other side. Its special geographical location leads to the inconvenient transportation, and it is used as a recycling area for electronic circuit boards and as crushing and stacking sites of waste circuit boards. The disassembly of electronic circuit boards in Nanwan village began in the early of 1980s. At first, people directly stacked the waste electronic circuit boards after recycling useful metals in open air. As the time passed, the stacking of waste circuit boards occupied a large area of the farmland, and then the waste electronic circuit boards were crushed and stacked in open air. The plastic powder of waste electronic circuit boards once produced in Nanwan village can be stacked into several “white hills” each year, where the plastic electronic circuit boards turn white after crushing. With the strict control of local government in the early 2000s, e-waste dismantling activities in Nanwan village were banned gradually, only the stacked electronic circuit boards and powders left, and told people what happened ever.
Generally, the dismantling of waste electronic circuit boards causes serious combined pollution problems of polychlorinated biphenyls (PCBs), polybrominated diphenyl ethers (PBDEs), heavy metal ions, and so on (Fu et al., 2012; Das et al., 2020; Liu et al., 2021). The direct stacking of electronic circuit boards and the powder without any treatment of the stacking sites have increased PCBs and PBDEs pollution in the groundwater and soil. According to a survey by Zhejiang Environmental Science Design and Research Institute, the soils in Fengjiang Town, Luqiao District, Taizhou City, Wenqiao and Zeguo town in Wenling City have been jointly polluted by PCBs and PBDEs to varying degrees, thus affecting the land quality and causing significant environmental safety problems.
Although the industrial use of PCBs and PBDEs has been banned (Li et al., 2020), considering that PBDEs and PCBs are quite stable in the environment and difficult to degrade. At the same time, these compounds are insoluble in water and soluble in fat, easy to be absorbed by animals, and gradually enriched in the food chain, thus endangering human health. In 2006, to chase for “green ecological village”, local people in Nanwan village removed the “white hills” to deep pits behind the village or at the foot of the mountain, directly buried the waste circuit boards and their plastic powder, and then covered them with sea mud. The extensive landfill without leakage prevention and anti-seepage treatment will cause unpredictable pollution to the groundwater and soil after long-term atmospheric settlement, surface overflow, underground runoff, and vertical infiltration. In 2011, the plastic powder in Nanwan village had new utilization value as an adhesive to make sintered bricks. The “white hill” was dug up and sold to brick kiln factories far away. After 2014, the sale of waste electronic circuit boards plastic powder was basically completed, and the ruins were left empty. Some areas were covered with a layer of sea mud, while some areas were directly exposed. A few years later, some ruins remained barren with little plant growth. This phenomenon occurred, mainly because the waste electronic circuit boards and their plastic powder had been stacked for a long time (even more than 20 years), and pollutants such as PBDEs, PCBs, and heavy metal ions have already penetrated the ruins and the surrounding soils or groundwater, which seriously threatened the food safety of the ruins, the surrounding farmland, and vineyards, and as well as threatened the health of the surrounding residents. Today, local agricultural and sideline products are labeled “green” or “organic” and sold all over the country, and their ecology and food safety are worrying.
At present, although the e-waste recycling activities in Taizhou city have been effectively controlled, and the areas polluted by the e-dismantling activities in Fengjiang town of Luqiao District and in Zeguo and Wenqiao town of Wenling City, have been gradually remediated. So far, many studies have focused on PCBs and PBDEs in soils, sediments, house dusts, plants in the well-known e-waste dismantling sites such as Guiyu and Longtang in Guangdong Province, Wenqiao and Fengjiang in Taizhou city, Zhejiang Province (Yang Z Z, 2009; Yu, et al., 2018; Li et al., 2019; Liu et al., 2019; Shi et al., 2019; Mao et al., 2021; Zhou et al., 2021). Few studies focus on the soil pollution and food safety issues caused by the dismantling and accumulation of waste circuit boards and their plastic powder in Nanwan Village, let alone the remediation of contaminated soil in Nanwan Village. Accordingly, four stacking ruins of electronic circuit boards and their plastic powder in Nanwan village, Wenqiao Town, Wenling City, Taizhou City were selected as the research areas, and the compound pollution of PCBs and PBDEs in the ruins, surrounding soils, and around the four crops were investigated to assess its ecological and food safety, also provide a theoretical basis for the subsequent remediation of contaminated soil in Nanwan Village.
After the investigation, four dismantling ruins, namely, No. 1, No. 2, No. 3, and No. 4 were selected as the dismantling or stacking ruins, which were stacked with the electronic circuit board or its plastic powder for more than 10 years Figures 1, 2 show the geographical locations and current situation of the sampling points.
The No. 1 sampling point is located on the hillside in the east of Nanwan village, where the plastic powder of electronic circuit boards has the longest stacking history and the largest scale. The plastic circuit board powder was cleaned up and sold in 2011–2012. At present, it is filled with a thick layer of sea mud, exposed, and without plant growth. The sampling point is located in 5 m away from No. 1 ruins [1(5)] at the foot of the hillside (now abandoned). Vineyards, orange orchards, and paddy fields lie at the foot of No. 1 ruins.
The No. 2 sampling point is on the hillside to the north of No. 1 ruins. Initially, waste electronic circuit boards were stacked after copper recycling (more than 20 years), and then a large number of circuit boards plastic powder was covered on the waste circuit board. The electronic circuit board powder was cleaned and sold in 2011–2014, and the waste electronic circuit board was removed in 2019. Currently, it is covered with a layer of soil. At No. 2 ruins, many electronic circuit boards and their plastic powder are directly exposed because of long-term rain erosion. During sampling, a 30–40 cm-thick circuit board powder is present on the surface, and the bottom soil (>40 cm) under the circuit board powder [2(0)] was collected after removing the surface plastic powder. Then, the vegetable field topsoil at 20 and 30 m around [2(20) and 2(30)]were collected. The topsoil at 2(20) contains a large amount of circuit board powder, circuit boards, and topsoil washed from No. 2 ruins, and the bottom layer contains circuit boards and their powder that cannot be sampled. Pumpkin and other vegetables were planted in this area. The vegetable field at 2(30) is a slightly higher than the vegetable field at 2(20).
The No. 3 ruins are located at the foot of the mountain at the east of Nanwan village adjacent to No. 2 ruins. The electronic circuit board powder stacked in there has been cleaned and sold from 2011 to 2014, and the surface is covered with a layer of sea mud. At present, a few citrus trees are planted in the sample plot, but the survival rate is very low, and weeds are rarely grown. The east and north areas of No. 3 ruins are hillsides. The south of No. 3 ruins is a paddy field, which is approximately 40 cm lower than the No. 3 ruins. A vegetable field is located at the west of No. 3 ruins, and this field is as high as the No. 3 ruins. The 1 m vegetable field soil to the west and 1 m paddy soil to the south of No. 3 ruins [3(1W) and 3(1S)] were sampled.
The No. 4 ruins are located at the foot of the mountain in the northeast of Nanwan village, where electronic circuit board powder has been stacked till today. In 2006, to chase for the “green ecological village”, the “white hill” near the roads were removed to No. 4 ruins. The villagers dug a deep pit at the foot of the mountain, filled in the plastic powder, and covered it with a thick layer of sea mud. The No. 4 ruins is a sloping land with a slope of approximately 25°. Ginger was planted in No. 4 ruins during sampling, and the soil samples at 1 and 20 m away (low terrain direction) from No. 4 ruins [4(1) and 4(20)], as well as the ginger were collected.
No. 1, No. 2, and No. 3 ruins are relatively close and located on the hillside or foot of the same mountain. To investigate the attenuation of PCBs and PBDEs with distance, the ruins of the accumulation area as the center at 100 and 200 m (100 and 200) paddy soil away from No. 1, No. 2, and No. 3 ruins in the low terrain direction near the coast around the electronic circuit board powder stacking ruins were sampled.
Three different soil samples were collected in each location, wrapped with Aluminum foil and took to the lab, natural air drying, then grinding and screening, equal mixing of different samples at the same location, put in the dryer before use (no more than 30 days). Oranges from the orchard 100 m away from No. 1, mung beans 2 m away, and garlic 5 m away from the vegetable field to the west of No. 3, and ginger planted on the coastal mud of No. 4 ruins were collected to investigate the contents of PCBs and PBDEs in the edible parts of the 4 crops. No. 4 ruins is located at the foot of the mountain behind Nanwan village, and this area is less than 50 m away from the villagers’ gathering area. In addition, the paddy fields 100 and 200 m away from No. 1, No. 2, and No. 3 ruins, the orchard 100 m away from No. 1, and the 3(1S) paddy field are at the same altitude. The vegetable fields soils at 2 and 5 m to the west of No. 3 ruins (3(2) and 3(5)) and 2(20) are at the same height. The 2(30) vegetable field is slightly higher than 2(20), and the altitudes of No. 1 and No.2 ruins are the highest with values that are at least 10 m higher than the paddy field. Table 1 shows the detail information of the soil samples.
Chemical reagents such as dichloromethane (ACS), n-hexane, neutral silica gel, neutral alumina, and anhydrous sodium sulfate (superior pure) were used directly without special treatments. n-Hexane (HPLC), eight-component PBDE mixed standard (batch No.214061106), and 20-component PCBs mixed standard (batch No.212071226) were obtained from J&K Scientific Ltd. (Shanghai). Neutral silica gel was placed on aluminum foil, baked at 550°C in muffle furnace for at least 12 h, and then cooled to 180°C for 1 h for activation. After cooling in dryer, the sample was transferred into a flask. Ultrapure water with mass ratio of 5% was added dropwise, and the mixture was shaken violently to prevent the silica gel from caking. The activated silica gel was inactivated, and then placed in the dryer for standby. Alumina was packed in the flask (no more than half of the volume). The bottle was loosely covered with aluminum foil, baked at 130°C for at least 12 h, plugged and sealed after cooling, and placed in the dryer for standby. Anhydrous Na2SO4 was calcined in a muffle furnace at 660°C for at least 6 h before use.
The sample extraction, cleanup, and chemical analysis of the PCBs and PBDEs were conducted using established methods (Li et al., 2018). In total, 10 ± 0.0005 g soil samples were spiked with 10 ng chemical surrogates (PCB209 and 2, 4, 5, 6-tetrachloro-m-xylene) and extracted via accelerated solvent extraction with mixed solvent (hexane/dichloromethane (DCM), 1:1, v/v). The cleaned extracts were passed through a silica gel/Florisil packed column for cleanup. The column was packed with 5 cm deactivated Florisil, 15 cm deactivated silica gel, 5 cm neutral alumina, and 5 cm anhydrous sodium sulfate from bottom to top. Elution was subsequently conducted using 20 ml of hexane, followed by 20 ml of DCM. The elute was concentrated to 0.5 ml by rotary evaporation, and then transferred to a nitrogen blowing pipe to blow nitrogen to 1 ml. The sample was passed through a 0.22-μm organic filter membrane, bottled, and placed in refrigerator at −4°C before GC-MS analysis.
Twenty PCB congeners (PCB 8, PCB 18, PCB 28, PCB 44, PCB 52, PCB 66, PCB 77, PCB 101, PCB 105, PCB 118, PCB 126, PCB 128, PCB 138, PCB 153, PCB 170, PCB 180, PCB 187, PCB 195, PCB 206, and PCB 209) and eight PBDE congeners (BDE 28, BDE 47, BED 99, BDE 100, DBE 153, BDE 154, BDE 181, and BDE 209) were determined using the Agilent (6890) gas chromatography/mass spectrometry (5975inert MSD, GC/MS) instrument. DP-5MS column was used, and the device was operated in electron impact (EI) ionization mode. The temperatures of the injector and detector were set to 260°C and 280°C, respectively. In total, 1 μl of the extracts was injected in the pulsed splitless mode. The carrier gas was nitrogen (99.99% purity), and the ion source and quadrupole temperatures were set at 230°C and 150°C. For PCBs, 30 m × 250 μm×0.25 μm DP-5MS column was used, the carrier flow rate was 1 ml min−1, and the oven program was started at 100°C. This temperature was then increased at a rate of 20°C min−1 to 230°C, then at 5°C min−1 to 240°C, kept at 3 min, increased at a rate of 20°C min−1 to 300°C, and finally kept for 5 min. For PBDEs, 15 m × 250 μm × 0.25 μm DP-5MS column was used. The carrier flow rate was 1.8 ml min−1, and the oven program was started at 120°C, which was then increased at a rate of 20°C min−1 to 325°C, and then kept for 6 min.
At present, no clear method has been established for the ecological risk assessment of PCBs in farmland soil at home or abroad. The ecological risk analysis of PCBs in farmland soil in Nanwan village was carried out by using the Environmental Quality Standard for sediments proposed by Long et al. (Long et al., 1995; Yao, 2019), and this guide is used as the national standard of the United States by the USEPA, as well as in related research (Yao, 2019). The standard proposed that organic matter in sediments can be divided into two boundaries, namely, low toxic effects (ERL) and toxic effects in value (ERM), and the thresholds of ERL and ERM were 22.7 and 180 μg kg−1, respectively. When the target pollutant concentration is less than ERL, it represents a minimal-effects range, in which effects are rarely observed. When the targe contaminant concentration is greater than ERM, the probability of biological effects is greater than 50% (Long et al., 1995; Wang et al., 2018).
For PCBs and PBDEs in soils and crops, USEPA exposure calculation model was used to calculate the human health risk assessment. The exposure to non-carcinogenic and carcinogenic risks of human population to PCBs and PBDEs in soils was estimated with the reference to the Regional Screening Levels (RSL) (Wu et al., 2019; Chen et al., 2021; Sun et al., 2021). Three routes that cover soil ingestion, soil dermal contact, and soil inhalation were considered to count the average daily dose (ADD, mg kg−1 day−1) of soil. ADD of PCBs and PBDEs congeners through soil were calculated as follows:
where ADDing, ADDder and ADDinh were cancer risk via ingestion, dermal contact, and inhalation of soil; Cs represents concentration of pollutant in soil.
Only intake was considered to count the ADD of the crops (ADDp). ADDp of PCBs and PBDEs congeners through food intake were calculated as follows:
Cp represents concentration of pollutant in the edible parts of the crops. The meaning and value of associated parameters used for the risk assessment are shown in Table 2, which are obtained from the USEPA and the corresponding literature, and listed in Table 2 (USEPA, 2008, USEPA, 2015, USEPA, 2018; Yu et al., 2011; Wu et al., 2018; Wu et al., 2019; Xu et al., 2014; Xu et al., 2019; Yu et al., 2020; Chen et al., 2021; Sun et al., 2021; Chinese Nutrition Society, 2022.).
PCBs and PBDEs are non-carcinogenic to adults and children (USEPA, 2018). PCBs and BDE 209 have carcinogenic risks to human health. The carcinogenic and non-carcinogenic risks associated with exposure to PCBs and PBDEs were evaluated based on the Risk Assessment Guidance for Superfund methodology from the USEPA (USEPA, 2008). As described in the USEPA screening level equations for preliminary remediation goals, the non-carcinogenic child, non-carcinogenic adult, and carcinogenic risk (age adjusted) were counted for soil, while the non-carcinogenic and carcinogenic risks were counted for crops (USEPA, 2018). The risks were calculated based on the estimated daily intake concentrations. For the non-carcinogenic risk (TQ), ADD is divided by the reference dose (RfD) (mg kg−1 day−1) (USEPA, 1992), while a TQ < 1 suggests acceptable hazard, while a TQ > 1 indicates potential risk meriting further investigation/remediation (Lim et al., 2014; Yu et al., 2020). For the lifetime carcinogenic risk (TR), ADD is multiplied by the slope factor (SF) (per mg kg−1 d−1) (Wu et al., 2019; Chen et al., 2021; Alfaro et al., 2022). According to USEPA, the individual lifetime carcinogenic risk value is 10–6, and the individual lifetime acceptable risk value is limited to 10–4 (USEPA, 2015; Yu et al., 2020).
The distribution of ∑20PCBs in the dismantling ruins and the surrounding soil are shown in Figure 3. The content of ∑20PCBs in 2(20) topsoil was 1,321.3 ± 132.1 μg kg−1, which was the highest value, followed by 471.8 ± 32.8 μg kg−1 at the paddy soil of 3(1S). This value reached 72.2 ± 24.2 μg kg−1 at the paddy soil of 1(5) and is lower than that at 3(1S). The concentration of ∑20PCBs at the farmland soil in 4(1) was 6.4 ± 1.1 μg kg−1, which was the lowest value.
FIGURE 3. Distribution of Σ20PCBs and Σ8PBDEs in the dismantling ruins and surrounding soils. 1(5): 5 m away from No. 1 ruins; 2(0): No. 2 ruins; 2(20): 20 m away from No.2 ruins; 2(30):30 m away from No.2 ruins; 3(1S):1 m away to the south of No.3 ruins; 3(1W): 1 m away to the west of No.3 ruins; 4(1): 1 m away from No.4 ruins; 4(20): 20 m away from No.4 ruins; 100: 100 m away from No.1, No.2 and No.3 ruins; 200: 200 m away from No.1, No.2 and No.3 ruins.
Different content of ∑20PCBs in different dismantling ruins and surrounding soils due to the different stacking time of electronic circuit board plastic powder. The pollutant content around the ruins with long stacking time is high, and the pollutant content around the ruins with short stacking time is relatively low. The geographical site of the No. 3 ruins is the most convenient. Thus, the accumulation time of plastic powder in No. 3 dismantling ruins is the longest. Therefore, the concentration of ∑20PCBs at the paddy soil in 3(1S) is the highest. The No.4 ruins had the shortest time for the stacking of plastic powder, resulting in the lowest ∑20PCB concentration.
Based on the ∑20PCBs in the subsoil of 2(0), vegetable field topsoil of 2(20) and 2(30), the ∑20PCBs in the topsoil of 2(20) was the highest with the value of 1,321.3 ± 132.1 μg kg−1, followed by 23.1 ± 1.3 μg kg−1 in the subsoil in 2(0). The ∑20PCBs in 2(30) was the lowest with the value of 7.1 ± 0.9 μg kg−1. Considering that the No. 2 dismantling ruins are on the hillside, the plastic powder and the pollutants have been washed by rain for a long time and gathered at the foot of the mountain under the action of gravity. Consequently, the ∑20PCBs of the topsoil in 2(20) is remarkably higher than that in the subsoil in 2(0). The content of ∑20PCBs of farmland topsoil in 2(30) is lower than that of the subsoil (>40 cm) in 2(0) and the topsoil in 2(20). The main reason is that the altitude of 2(30) is slightly higher than that of 2(20), it is further away from the No. 2 ruins, and the waste plastic powder and its pollutants were washed from the No. 2 ruins and gathered at 2(20) but not at 2(30).
The content of ∑20PCBs of paddy soil in 3(1S) and 3(1W) remarkably differed, and the content of ∑20PCBs in 3(1S) reached 471.8 ± 32.8 μg kg−1. Meanwhile, the content in 3(1W) was only 7.7 ± 1.5 μg kg−1, mainly because 3(1W) has the same altitude as the No. 3 ruins, while the paddy field in 3(1S) is approximately 40 cm lower than the No. 3 ruins, and the pollutants preferentially gather and diffuse to a low-lying direction.
The content of ∑20PCBs in paddy soil of 100 and 200 m away from No. 1, No. 2, and No. 3 dismantling “ruins” decreased with the increase in radial distance, and it reached 14.2 ± 0.8 μg kg−1 at a distance of 100 m away and 9.2 ± 1.4 μg kg−1 at a distance of 200 m. The content of ∑20PCBs at the 100 m paddy soil is lower than that in 1(5) and 3(1S), and the content of ∑20PCBs at the 200 m paddy soil is lower than that at the 100 m paddy soil. With the increase in the distance from the ruins of plastic powder stacking, the content of ∑20PCBs in soil gradually decreased as a result of the horizontal diffusion of pollutants. Therefore, the main sources of PCBs in the soil of Nanwan village are the dismantling of electronic circuit boards and the accumulation of waste plastic circuit board powders.
The content of ∑20PCBs in paddy soil at 100 m away from No. 1, No. 2, and No. 3 dismantling ruins is slightly higher than that of 2(30), mainly because the 100 and 200 m paddy fields have the same altitude as that of 3(1S) and are lower than the vegetable field of 2(20). However, the vegetable field of 2(30) is slightly higher than that of 2(20). Pollutant diffusion preferentially spreads from No. 2 ruins to the vegetable field of 2(20), and then to the paddy soil of 100 m. The diffusion of PCBs includes the horizontal diffusion of pollutants and the vertical diffusion caused by groundwater and surface runoff. The diffusion of PCBs from 2(20) to 2(30) mainly involves horizontal diffusion caused by soil and atmosphere.
In addition, the content of ∑20PCBs in the vegetable field soil of 4(1) is 6.4 ± 1.1 μg kg−1, which is lower than 11.3 ± 3.5 μg kg−1 at 4(20). Considering that the No. 4 ruins is inclined, the content of ∑20PCBs in farmland soil of 4(20) is significantly lower than that in 4(1). Considering the downward accumulation of pollutants caused by rainwater scouring, the content of ∑20PCBs in the vegetable field soil of 4(1) is lower than that of 4(20).
China’s Soil Environmental Quality—Risk control Standard for Contamination of Agricultural Land (Trial, GB15618-2018) does not provide corresponding guidance values for PCBs in farmland soil. Different countries and regions abroad provide different regulatory guidance values of ΣPCBs for farmland soil. The Canadian soil quality guide stipulates that ΣPCBs in farmland soil is limited to 0.5 mg kg−1, and the preventive values of ΣPCBs by German soil protection regulations for sensitive use are 0.1 mg kg−1 (humus content <8%) and 0.05 mg kg−1 (humus content >8%). The soil quality standards of the Netherlands and Czech Republic stipulate that ΣPCBs in farmland soil is limited to 0.02 mg kg−1, while that of the United States stipulates that the screening value of ΣPCBs in soil based on groundwater protection is 0.026 mg kg−1. The content of Σ20PCBs in the topsoil of 2(20) exceeded the screening value or the abovementioned limiting values for farmland soil. The content of Σ20PCBs in the paddy soil of 3(1S) is only lower than the limit of ΣPCBs in the farmland soil specified by the Canadian soil quality guide, indicating the presence of ecological risks in the No. 2 and No. 3 ruins and the surrounding farmland soils, and this area is not suitable for agricultural use any more.
Other studies on PCBs in farmland soil carried out in the adjacent areas of this study pointed out that the average concentration of ΣPCBs was 0.509 mg kg−1, the maximum concentration was 7.914 mg kg−1, and the minimum concentration was 0.027 mg kg−1 in Wenqiao Town, Wenling City in 2007. According to the detailed investigation of soil pollution in 2013, the Wenqiao town has 42 sites, the average concentration of ΣPCBs was 0.206 mg kg−1, the maximum and minimum concentrations were 2.994 and 0.023 mg kg−1, and the over standard rate of ΣPCBs was 40.48%. In the present study, the average content of Σ20PCBs in all soil samples was 0.142 mg kg−1, the maximum concentration was 1.321 mg kg−1, and the minimum concentration was 0.006 mg kg−1. Evidently, the pollution degree of PCBs in the study area has improved with the passage of time, because the dismantling activities of electronic products had stopped as early as around 2006, the input of large number of exogenous PCBs has stopped, and the original PCBs in the soil gradually migrated and transformed, resulting in a decreasing trend of PCBs content in the study area.
In comparison with the ΣPCBs in the soils of other regions, which are also the dismantling places of e-waste in this study, the average content of Σ39PCBs in the soil of an electronic waste recycling plant in Guanzhong area of China was 18.95 μg kg−1, which reached 20.31 μg kg−1 in the soil of Jiangcungou landfill, and then reached 33.89 μg kg−1 in the soil of waste compression station and waste recovery point (Liu et al., 2021). The content of ΣPCBs in an electronic waste recycling town soil of northern Vietnam is in the range of 2.0–7,200 μg kg−1 (Fujimori et al., 2018). The highest content of Σ26PCBs in the topsoil of e-waste recycling sites and nearby open-air waste sites near New Delhi (North), Kolkata (East), Mumbai (West), and Chennai (South) was 437 μg kg−1 in Chennai, followed by 102 μg kg−1 in Mumbai (Chakraborty et al., 2018). The average content of Σ19PCBs in the soil of Fengjiang Town (another town for e-waste disassembly and recycling), Luqiao District, Taizhou city, is 431 (11.7–2.61 × 103) μg kg−1, while that in Luqiao coastal metal resources regeneration industrial base was 15.5 (0.350–50.8) μg kg−1 (Liu et al., 2019). The contents of ΣPCBs in the soil of Guanzhong electronic waste resource recycling plant, Jiangcungou landfill, Luqiao waste compression station, and Luqiao Binhai waste recycling metal resource regeneration industrial base were considerably lower than that in the study area, and while those in the soil of Fengjiang Town, Luqiao District, northern Vietnam, and southern India (Chennai) were much higher than that in the study area. This finding was obtained, because the types and concentrations of pollutants differ because of the different types of electronic products disassembled in different electronic product disassembly sites and different service times.
The content of ΣPCBs was 3.25 (2.92–3.38) μg kg−1 in the organic farmland soil in Hong Kong, China and 5.96 (2.67–16.5) μg kg−1 in the ordinary farmland soil (Yu et al., 2011). The average concentration of Σ7PCBs in the farmland topsoil in the Yellow River irrigation area is 11.18 μg kg−1 (Yao, 2019). The concentration of Σ209PCBs in soils across China was in the range of 64.3–4,358 pg g−1 (Mao et al., 2021). The concentrations of Σ26PCBs is 0.256–2.140 μg kg−1 on dry weight basis in surface soils (Wang et al., 2010). The content of Σ7PCBs in farmland soil in the southwest of Buenos Aires Province, Argentina is 0.04–1.67 μg kg−1 (Tombesi et al., 2017). The levels of PCBs ranged from 10.46 ng g−1 for PCB 105 to 89.46 ng g−1 for PCB180, with an average PCB value of 25.47 ± 1.26 ng g−1, in the banks of the Umgeni River soil, KwaZulu-Natal, South Africa (Gakuba et al., 2019). The content of ∑PCBs was <0.003–0.807 ng g−1, and congener Nos. 114, 138, and 187 were the most abundant in Victoria Land, East Antarctica (Corsolini et al., 2019). The content of Σ18PCBs in 25 soil sampling sites within a radius of 6 km around an e-waste treatment site in Hangzhou was 0.051–1.835 μg kg−1(Zhou et al., 2020). In comparison with the content of ΣPCBs in farmland soil, the content of ∑20PCBs in the study area is significantly higher, except for the riverbank soil in South Africa, which is obviously affected by the disassembly of electronic circuit boards and the accumulation of plastic powder of waste circuit boards.
By comparing the detection results of Σ20PCBs in the soils of different electronic circuit board plastic powder dismantling ruins with the Environmental Quality Standard for sediments proposed by Long et al., the content of Σ20PCBs in the topsoil of 2(20) and the paddy soil of 3(1S) exceeds the ERM value, indicating that the probability of biological toxicity events would frequently occur, and PCBs could be toxic to the organisms in the study area. The contents of Σ20PCBs in the subsoil (>40 cm) of 2(0) and the topsoil of 1(5) are between the ERL and ERM value, indicating that the probability of biological toxicity events may occur, and PCBs may have toxic effects on organisms in the study area. However, the content of Σ20PCBs in other soil samples is lower than ERL value, indicating that the probability of biological toxicity effect would be rarely observed.
Based on the distribution characteristics of PCBs, among the 20 PCBs investigated, the contents of PCB 101, PCB 138, and PCB 153 in the topsoil of 2(20) are higher than the ERM value, and the contents of PCB 44, PCB 66, PCB 105, PCB 118, PCB 128, PCB 170, PCB 180, and PCB 187 in the topsoil of 2(20) and those of PCB 101, PCB 118, PCB 126, PCB 138, PCB 153, and PCB 209 in the paddy soil of 3(1S) are between the ERL and ERM value. Whether from the total amount of PCBs or the content of individual PCBs, certain ecological risks are present in the ruins of waste electronic circuit board plastic powder accumulation in Nanwan village and the surrounding farmland soil, and some areas are not suitable for use as farmland. The composition of PCBs in the topsoil of 2(20) and the paddy soil of 3(1S) is similar, indicating that the content of PCBs containing four chlorine atoms (4Cl), five chlorine atoms (5CL), six chlorine atoms (6Cl), seven chlorine atoms (7Cl), and 10 chlorine protons (10Cl) are higher, while the content of PCBs containing two chlorine atoms (2Cl), three chlorine atoms (3Cl), eight chlorine atoms (8Cl), and nine chlorine atoms (9Cl) are low.
The distribution of Σ8PBDEs content in the waste electronic circuit board plastic powder stacking ruins and surrounding soil is shown in Figure 3. The content of Σ8PBDEs in the dismantling ruins and surrounding soils is similar to that of ∑20PCBs. The contents of Σ8PBDEs in the vegetable field soil of 2(20) and paddy soil of 3(1S) are significantly higher than that in other points. The highest value of Σ8PBDEs in the dismantling ruins and surrounding soils was recorded in the paddy soil of 3(1S) with a value of 7,216.9 ± 232.0 μg kg−1, followed by 6,094.3 ± 389.2 μg kg−1 in the vegetable field soil of 2(20), and these values slightly differ from the content of ∑20PCBs. The content of Σ8PBDEs was 1,010.8 ± 36.2 μg kg−1 in the paddy soil of 1(5) and 40.3 ± 1.4 μg kg−1 in the vegetable field of 4(1).
Considering the combined action of horizontal and vertical diffusion of pollutants, the content of Σ8PBDEs in the topsoil (0-10 cm) of 2(20) is higher than that in the subsoil (>40 cm) of 2(0) (677.8 ± 35.7 μg kg−1), and the lowest value of 276.1 ± 22.2 μg kg−1 was obtained in the vegetable field soil of 2(30). The content of Σ8PBDEs in the paddy soil of 3(1S) and vegetable field soil of 3(1W) remarkably differ with the value of 7,216.9 ± 232.0 μg kg−1 in the paddy soil of 3(1S), which is far higher than 177.6 ± 2.3 μg kg−1 in the vegetable field soil of 3(1W). In addition, the content of Σ8PBDEs in the vegetable field soil of 4(1) is 40.3 ± 1.4 μg kg−1, which is also lower than 135.3 ± 6.4 μg kg−1 in 4(20), mainly because the ruins of No. 4 is sloped with a certain inclination, and the combined action of horizontal and vertical diffusion increased the content of Σ8PBDEs in the vegetable field soil of 4(20).
In the paddy soils 100 and 200 m away from the ruins of No. 1, No. 2, and No. 3, the content of Σ8PBDEs decreased with the increase in radial distance with values of 667.3 ± 26.2 μg kg−1 at 100 m and 191.5 ± 12.5 μg kg−1 at 200 m. The content of Σ8PBDEs in paddy soil of 100 m is lower than those in the paddy soil of 1(5), vegetable field soil of 2(20), and paddy soil of 3(1S), but it is higher than that in the vegetable field soil 2(30) (276.1 ± 22.2 μg kg−1) and that in the vegetable field soil of 3(1W) (177.6 ± 2.3 μg kg−1). This phenomenon is also the result of the joint action of horizontal diffusion and vertical diffusion.
Eight PBDEs were all detected in the dismantling ruins and all surrounding soil samples, and the content of BDE 209 was notably high. Among all the PBDEs investigated, the percentage content of BDE 209 exceeded 20% in most soil samples, except that in the vegetable field soil of 2(20) and the paddy soil of 3(1S). The percentage content of BDE 209 was 53.2% in the paddy soil 100 m away from No. 1, No. 2, and No. 3 ruins, 55.9% in paddy soil of 3(1W), and 65.7% in the paddy soil 200 m away from No. 1, No. 2, and No. 3 ruins. The highest percentage content of BDE 209 was 88.3% in the vegetable field soil of 4(20).
Zhongzhi Yang inspected the contents of ∑39PBDEs in soil samples of Nanwan village in 2006 and found that the content of BDE 209 accounted for 72.4% of the detected Σ39PBDEs. Moreover, PBDEs in soil samples in Nanwan village had a degradation trend (Yang, 2009). This result was consistent with the findings of Zhongzhi Yang’s research, except for the high percentage content of BDE 209 in the vegetable field soil of 4(20), in which the percentage content of BDE 209 in other samples is significantly lower than Zhongzhi Yang’s research results. This finding was obtained, because the dismantling activities of electronic circuit boards in Nanwan village had stopped around 2006, and the accumulated plastic circuit board powder was gradually cleaned up after that. Without the input of exogenous PBDEs, the highly brominated PBDEs existing in the environment were gradually degraded to low brominated PBDEs, thus decreasing the percentage content of BDE 209. The percentage content of BDE 209 in the vegetable field soil of 4(20) is high, mainly because the stacking time of PBDEs is short, and the accumulated plastic powder of electronic circuit board has not been cleaned up because of inconvenient transportation. In addition, the percentage contents of BDE 47, BDE 99, and BDE 209 in the study area were high, which is consistent with the research results of Zhongzhi Yang. Therefore, the PBDEs in the study area were obtained from the dismantling activities of electronic products and the accumulation of dismantling waste.
Although the human intake of PBDEs through food would lead to liver enlargement, affect the development of brain, reproductive organs, neurobehavior, and interfere with the level of thyroxine, a soil environmental quality standard for farmland soil on PBDEs has not been formulated at home or abroad. Moreover, neither farmland soil nor construction land in China has not been provided with guidance or control value to PBDEs. The general soil screening value of USEPA sets the soil screening value based on groundwater protection as follows: 97 μg kg−1 for BDE 47, 160 μg kg−1 for BDE 99, and 53 mg kg−1 for BDE 209. In comparison with the results for the soil screening value based on groundwater protection provided by the USEPA, the values for BDE 47 in the paddy soil of 1(5), the subsoil of 2(0), the vegetable field soil of 2(20), and the paddy soil of 3(1S) were all exceeded, and the exceeding rate was 40%. The BDE 47 content in the vegetable field soil of 2(20) and paddy soil of 3(1S) are 15 and 20 times higher than the screening value based on groundwater protection. The BDE 99 content in the paddy soil of 1(5), the subsoil of 2(0), the vegetable field soil of 2(20), the paddy soil of 3(1S), and the 100 m paddy soil all exceeded the screening value based on groundwater protection, and the exceeding rate was 50%. The BDE 99 contents of the vegetable field soil of 2(20) and the paddy soil of 3(1S) were 11 and 12 times higher than the screening value based on groundwater protection. The content of PBDEs in some soil samples at or around the stacking ruins of waste electronic circuit board plastic powder in Nanwan village are higher than the screening value based on groundwater protection in the United States, indicating that the soil in the village may have a certain risk to groundwater caused by the pollution of PBDEs.
The concentrations of BDE 209 were in the range of 0.496–2.040 μg kg−1 on dry weight basis in the surface soils of the Tongzhou district, Beijing City, and it accounts for more than 96% of the total PBDEs (Wang et al., 2010). The average concentration of ∑PBDEs in the farmland soil of Longtang-Shijiao town, Guangdong Province, was 19.0 ± 20.1 μg kg−1, which was much lower than that in the roadside soil and in topsoil near the e-waste dismantling sites (Jiao et al., 2016). The concentrations of Σ8PBDEs were in the range of 29–240 ng kg−1 in Jinsha, which is one of the regional background sites in Central China (Zhan et al., 2019). The average ∑8PBDEs in the farmland soil of Baotou city, China, ranged from 1.25 μg kg−1–13.3 μg kg−1, with an average of 3.62 μg kg−1, and a median of 2.91 μg kg−1 (Chen et al., 2020). In comparison with this study, the content of ∑PBDEs in farmland soil in the study area was remarkably higher than that in other areas of China, indicating that the farmland soil in Nanwan village was obviously affected by the disassembly activities of electronic circuit board and the stacking of waste plastic powder.
The content of Σ25PBDEs in 66 farmland soil samples collected from an e-waste dismantling area in Taizhou city was 0.13–11.80 μg kg−1, in which the mass concentration of BDE 209 accounted for 50.9%–98.3% (Zhang et al., 2018). The content of PBDEs was 182.5 ± 38.72 μg kg−1 in farmland soil near an e-waste dismantling area in Wenling city, China (Zhang et al., 2015). The concentrations of Σ12PBDEs in the farmland soil of e-waste dismantling site in Fengjiang Town and Binhai Town were 21.8–1,310 and 6.19–220 μg kg−1, respectively (Wei et al., 2020). The content of Σ13PBDEs around an e-waste dismantling site in Guiyu, Guangdong Province was 62–76 μg kg−1 in rice field soil and 46-58 μg kg−1 in vegetable field soil (Li et al., 2018). The total concentration of ΣPBDEs in the surrounding area of an e-waste dismantling park was 11.6–3.60 × 104 μg kg−1 in Guiyu (Ge et al., 2020). PBDEs were the most abundant at 0.343–69.306 μg kg−1 in soils from an e-waste disposal site in Hangzhou (Zhou et al., 2020). The Σ13PBDE concentrations in soils around an open-burning sites in northern Vietnam were 7.6 (1.6 ± 62.0) μg kg−1 in 2012, 12.0 (0.1 ± 48.0) μg kg−1 in 2013, and 16.0 (2.1 ± 39.0) μg kg−1 in 2014. The concentrations were 1,200 (67-9,200) μg kg−1 in 2012, 480 (37-3,900) μg kg−1 in 2013, and 490 (39-2,900) μg kg−1 in 2014 in soils around the electronic waste-processing workshops (Matsukami et al., 2017). Generally, the measured concentrations of PBDEs in the soils analyzed in the present study were higher than or close to those in other areas at home or abroad, which are also the areas of electronic product disassembly.
The vertical distribution of Σ20PCBs and Σ8PBDEs in farmland soil around the ruins is shown in Figure 4. When the depth away from the surface soil increased from 0-10, 10-20, 20-30, and 30-40 cm to >40 cm, the content of Σ20PCBs in farmland soils of 1(5) changed from 48.075 ± 5.133, 96.376 ± 43.293, 25.879 ± 2.199, and 7.49 ± 0.737 μg kg−1 to 8.625 ± 0.363 μg kg−1, respectively. The disassembly activities of electronic circuit boards in Nanwan village stopped around 2006, and the stacking electronic circuit board powder had been removed from 2011 to 2014 (except No. 4 ruins). Although the paddy field was deserted at the time of sampling, nearly 10 years had passed, and it is impossible to be barren at that place all the time. With the progress of farming activities, the 0-20 cm topsoil turned up and down coupled with the migration and diffusion of pollutants. As a result, the content of Σ20PCBs in paddy soil with the depth of 10–20 cm is higher than that at 0-10 cm. As shown in Figure 4, with the continuous increase in depth from the surface, the content of Σ20PCBs remarkably decreased because of the vertical diffusion of pollutants. Therefore, the PCBs in the soil were obtained from the disassembly activities and the stacking of electronic circuit boards, and the PCBs in the soil gradually dispersed from the surface to the deep soil until a certain number of PCBs remained detected at the soil depth of >40 cm. The content of Σ20PCBs increased slightly as the depth exceeded 40 cm because of the dual action of surface subsidence and groundwater migration.
The percentage contents of 5Cl, 6Cl, and 7Cl are relatively high, and their total contents accounted for 73%, 77%, 76%, 57%, and 36% of the total amount of Σ20PCBs at the depth of 0–10, 10–20, 20–30, 30–40, and >40 cm soil layer, respectively. Similarly, except for the topsoil, with the increase in soil vertical depth, the percentage contents of PCBs containing 5Cl, 6Cl, and 7Cl decreased gradually, and the percentage contents of PCBs containing 2Cl, 3Cl, and 4Cl increased gradually, indicating that PCBs in the soil tended to degrade gradually from high chlorination to low chlorination.
When the depth away from the surface soil increased from 0–10, 10–20, 20–30, and 30–40 cm to >40 cm, the contents of Σ8PBDEs in paddy soil at 1(5) changed from 1,501.239 ± 52.965, 520.315 ± 19.425, 279.794 ± 14.974, and 117.457 ± 8.695 μg kg−1 to 119.336 ± 10.673 μg kg−1, respectively. The content of Σ8PBDEs in paddy soil of 1(5) decreased vertically with the increase in depth, indicating that the content of Σ8PBDEs in soil gradually diffuses vertically from the surface to the deep layer, and a few PBDEs were detected in the soil at a depth higher than 40 cm. The content of Σ8PBDEs increased slightly at a depth >40 cm as a result of the dual action of surface subsidence and groundwater migration. Zhongzhi Yang (Yang, 2009) sampled in the same study area around 2006 and found that the concentration of PBDEs decreased rapidly with the increase in soil depth, which is consistent with the results of the present study. By contrast, when Zhongzhi Yang obtained the samples, the disassembly activities of electronic circuit board were still in progress, and the vertical change of PBDEs observed was only within the range of 0–20 cm from the surface, while the soil depth investigated in the present study was deeper, and the soil depth range >40 cm from the surface was investigated.
Among the eight PBDEs investigated, BDE 47, BDE 99, and BDE 209 have relatively high percentage contents in the paddy soil of 1(5) because of the large commercial consumption of BDE 47, BDE 99, and BDE 209. The highest percentage content of PBDEs was observed in BDE 209; among all soil samples at the depth of 0 to >40 cm, the lowest percentage content of BDE 209 was 23% at the depth of 0–10cm, while the highest percentage of 53% was observed at the depth of 20–30 cm, and it arrange 42%–47% in other depth’s soil. The contents of BDE 99 at the soil depths of 0–10, 10–20, 20–30, 30–40, and >40 cm were 27%, 22%, 18%, 7%, and 9% respectively, showing an obvious downward trend. The percentage contents of BDE 47 at the soil depths of 0–10, 10–20, 20–30, 30–40, and >40 cm were 20%, 15%, 7%, 11%, and 14%, respectively. Among all the eight PBDEs investigated, the lowest percentage contents were obtained for BDE 28 and BDE 154, the values at all soil depths did not exceed 8%.
To further investigate the effect of soil organic pollutants on the crops around the ruins, we investigated the contents of Σ20PCBs and Σ8PBDEs in the directly edible parts of four crops, such as oranges planted in the 100 m orchard away from No. 1 ruins, mung beans and garlic planted in the 2 m and 5 m vegetable field to the west of No. 3 ruins, and ginger in the No. 4 stacking ruins. The results are shown in Figure 5. The contents of Σ20PCBs in the edible parts of garlic, ginger, mung bean, and orange were 8.269 ± 2.735, 30.448 ± 2.287, 6.429 ± 2.065, and 7.458 ± 1.250 μg kg−1, respectively. The percentage contents of PCBs containing 5Cl, 6CL, and 7Cl in the edible part of garlic all exceeded 10%, and the total amount of these three kinds of chlorinated PCBs to Σ20PCBs reached 64%. The percentage contents of PCBs containing 3Cl, 4Cl, 5Cl, and 6Cl in edible parts of ginger all exceeded 10%, and the total percentage content of these four kinds of chlorinated PCBs to Σ20PCBs reached 73%. The percentage contents of PCBs containing 3Cl, 4Cl, 5Cl, 6Cl, and 7Cl in the edible parts of mung bean all exceeded 10%, and the total percentage content of these kinds of chlorinated PCBs to Σ20PCBs reached 92%. The percentage content of PCBs containing 5Cl in the edible part of oranges was particularly high, reaching 68%, and the percentage contents of other chlorinated PCBs were all less than 10%. In addition, among the four crops investigated, the content of Σ20PCBs in the edible part of ginger reached 30.448 ± 2.287 μg kg−1, which exceeded the first-class limitation value of construction land set in the National Soil Environmental Quality Standard GB 36620–2018 (Trial, GB 36600-2018). This high content of PCBs in construction land might pose risks to human health, and possible food safety problems should be looked into.
The contents of Σ8PBDEs in the edible parts of garlic, ginger, mung bean, and orange were 1,923.132 ± 39.545, 197.197 ± 7.050, 35.621 ± 1.461, and 178.77 ± 3.577 μg kg−1, respectively. Among the four crops investigated, the content of Σ8PBDEs in garlic reached 1,923.123 ± 39.545 μg kg−1. Although no relevant standard data are available, this content is significantly higher than the content of PBDEs in farmland soil at home or abroad, which may cause food safety problems that need to be solved. The percentage content of BDE 209 to Σ8PBDEs in the edible parts of garlic and orange accounted for 99% and 87% respectively, indicating that garlic and orange easily absorb BDE 209. In comparison with other PBDEs, the percentage content of BDE 99 in the edible part of ginger and mung bean was the highest, reaching 58% and 37%, respectively.
Among the four crops investigated, whether Σ20PCBs or Σ8PBDEs, the content in the edible parts of ginger and garlic are higher than that in the edible parts of oranges and mung beans. The edible parts of ginger and garlic grow in the soil, while the edible parts of mung beans and oranges hang on the branches of plants, indicating that the PCBs and PBDEs in crops were mainly derived from contaminated soil. The contents of 20 PCBs and 8 PBDEs in different crops differed mainly because of the different types of dismantling and stacking waste electronic circuit boards in different dismantling ruins, as well as the different types of pollutants. In addition, the absorption and accumulation of different kinds of PCBs and PBDEs by different crops differed.
Table 3 shows the lifetime carcinogenic risk level and non-carcinogenic level of PCBs and PBDEs in the dismantling ruins and surrounding soils. Among all the soil samples, only Σ20PCBs in the topsoil of 2(20) and the paddy soil of 3(1S) had a TR value that is higher than the individual lifetime carcinogenic risk value (10–6), and both values were lower than the individual lifetime carcinogenic acceptable risk level (10–4), indicating that the lifetime carcinogenic risk level of PCBs and PBDEs in the dismantling ruins and surrounding farmland soil are at acceptable or negligible levels. Among all the soil samples, only the TQ value of PCBs for children in the topsoil of 2(20) is higher than 1, suggesting that the non-carcinogenic risk of PCBs is high among children. All the TQ values of PBDEs for children and adults are lower than 1, indicating that the PBDE level is safe for non-carcinogenic risk.
The crop samples collected in the present study are directly edible parts. Therefore, the food chain is the main exposure route for health risk assessment. According to the average intake of each crop in lifetime, the carcinogenic risk level and non-carcinogenic level of the corresponding crops in lifetime can be calculated. The results are shown in Table 4. As shown in Table 4, the TQ values of PBDEs for the four crops are far less than 1, indicating that the non-carcinogenic risks of PBDEs and PCBs levels are at a safe level. The TR values of BDE 209 in the edible parts of garlic, ginger, mung bean, and orange are all lower than 10–6, indicating the carcinogenic levels caused by BDE 209 in the four crops are at negligible levels. However, the TR values of PCBs in the edible parts of garlic, ginger, mung bean, and orange are all between 10–6 and 10–4, indicating that the carcinogenic levels caused by PCBs in the crops are at acceptable levels.
Among the four dismantling ruins and surrounding soil samples investigated, the most polluted soil was the vegetable field topsoil of 2(20) and the paddy soil of 3(1S), the content of Σ20PCBs in the vegetable field topsoil of 2(20) was 1,321.3 ± 132.1 μg kg−1, and the content of Σ8PBDEs in the paddy soil of 3(1S) reached 7,216.9 ± 232.0 μg kg−1. With the increase in soil depth, the content of Σ20PCBs and Σ8PBDEs remarkably decreased because of the vertical diffusion of pollutants. Therefore, the pollutants in the soil came from the disassembly activities and accumulation of electronic circuit boards, and the pollutants in the soil gradually dispersed from the surface soil to the deep soil, until a certain number of pollutants were still detected at the soil depth above 40 cm. The content of Σ20PCBs and Σ8PBDEs increased slightly when the depth exceeded 40 cm because of the dual action of surface subsidence and groundwater migration. In comparison with the content of ∑20PCBs and ∑8PBDEs in other farmland in this study, the content of ∑20PCBs and ∑8PBDEs of the study area was remarkably higher than that at home or abroad as a result of the dismantling activities of electronic circuit boards and the accumulation of plastic powder of waste circuit boards.
Ecological risk assessment showed that the content of Σ20PCBs in the vegetable field topsoil of 2(20) and the paddy soil of 3(1S) exceed the ERM value, indicating that biological toxicity events are likely to occur frequently, and PCBs could be toxic to the organisms in the study area. The contents of Σ20PCBs in the subsoil of 2(0) and topsoil of 1(5) are between ERL and ERM values, indicating that biological toxicity events may occur. Among all the soil samples, the lifetime carcinogenic and non-carcinogenic risks of PBDEs were at a safe level, and only the TQ value of PCBs for children in 2(20) topsoil is higher than 1, suggesting that the non-carcinogenic risk of PCBs is high among children. PCBs and PBDEs in the edible parts of garlic, ginger, mung bean, and orange are at a safe level for non-carcinogenic risk. The carcinogenic risks caused by BDE 209 and ∑20PCBs of the crops are at negligible levels or acceptable levels.
Although this study can help in the decision-making process for monitoring soil pollution and food safety in Nanwan village, it also has some limitations. The risk assessment did not consider the synergistic or antagonistic effects between PCBs and PBDEs in soils or crops, or both. Moreover, the risk assessment did not consider the cumulative exposure to other environmental pollutants with a toxicity mechanism, such as Cu2+, Cd2+, and Pd2+. The co-existence of such pollutants will affect the risk assessment of daily exposure. Therefore, systematic studies with larger scale sampling are necessary to expand the database.
Although the human intake of PBDEs through food would lead to some diseases, no soil environmental quality standard has been established for farmland soil on PBDEs has been formulated at home or abroad. Accordingly, appropriate and protective soil standards need to be urgently established to minimize human exposure from food producing animals housed outdoors. Moreover, pollution sources of POPs (e.g., PCBs and PBDEs) in soils need to be eliminated, and contaminated sites and reservoirs need to be controlled, secured, and remediated to reduce exposure and guarantee food safety.
The original contributions presented in the study are included in the article/Supplementary Material, further inquiries can be directed to the corresponding author.
All authors contributed equally for the conception and writing of the manuscript.
This work was supported by Zhejiang Province National Science Foundation of China (No.LY20E080013) and Taizhou science and technology planning project by Taizhou Science and Technology Bureau (1902gy17).
The authors declare that the research was conducted in the absence of any commercial or financial relationships that could be construed as a potential conflict of interest.
All claims expressed in this article are solely those of the authors and do not necessarily represent those of their affiliated organizations, or those of the publisher, the editors and the reviewers. Any product that may be evaluated in this article, or claim that may be made by its manufacturer, is not guaranteed or endorsed by the publisher.
Alfaro, M. R., Ugarte, O. M., Lima, L. H. V., Silva, J. R., da Silva, F. B. V., da Silva Lins, S. A., et al. (2022). Risk assessment of heavy metals in soils and edible parts of vegetables grown on sites contaminated by an abandoned steel plant in Havana. Environ. Geochem. Health 44 (1), 43–56. doi:10.1007/s10653-021-01092-w
Chakraborty, P., Selvaraj, S., Nakamura, M., Prithiviraj, B., Cincinelli, A., and Bang, J. J. (2018). PCBs and PCDD/Fs in soil from informal e-waste recycling sites and open dumpsites in India: Levels, congener profiles and health risk assessment. Sci. Total Environ. 621, 930–938. doi:10.1016/j.scitotenv.2017.11.083
Chen, Y. P., Zhao, Y., Zhao, M. M., Wu, J. H., and Wang, K. B. (2021). Potential health risk assessment of HFRs, PCBs, and OCPs in the Yellow River basin. Environ. Pollut. 275, 116648. doi:10.1016/j.envpol.2021.116648
Chen, Y., Zhang, A., Li, H., Peng, Y., Jun, J., Liu, M., et al. (2020). Concentrations and distributions of polybrominated diphenyl ethers (PBDEs) in surface soils and tree bark in Inner Mongolia, northern China, and the risks posed to humans. Chemosphere 247, 125950. doi:10.1016/j.chemosphere.2020.125950
Chinese Nutrition Society, (2022). Dietary guidelines for Chinese residents. Available at: https://www.jandonline.org/article/S0002-8223(00)00257-1/fulltext#relatedArticles.
Corsolini, S., Baroni, D., Martellini, T., Pala, N., and Cincinelli, A. (2019). PBDEs and PCBs in terrestrial ecosystems of the Victoria Land, Antarctica. Chemosphere 231, 233–239. doi:10.1016/j.chemosphere.2019.05.126
Das, P., Gabriel, J. C. P., Tay, C. Y., and Lee, J. M. (2020). Value-added products from thermochemical treatments of contaminated e-waste plastics. Chemosphere 269, 129409. doi:10.1016/j.chemosphere.2020.129409
Fu, J., Wang, T., Wang, P., Qu, G., Wang, Y., Zhang, Q., et al. (2012). Temporal trends (2005-2009) of PCDD/Fs, PCBs, PBDEs in rice hulls from an e-waste dismantling area after stricter environmental regulations. Chemosphere 88 (3), 330–335. doi:10.1016/j.chemosphere.2012.03.006
Fujimori, T., Takigami, H., Agusa, T., Takaoka, M., Suzuki, G., Tue, N. M., et al. (2018). Soil pollution by chlorobenzenes and polychlorinated biphenyls from an electronic waste recycling area in Northern Vietnam. Int. J. Environ. Pollut. 63 (4), 283–297. doi:10.1504/ijep.2018.10019177
Gakuba, E., Moodley, B., Ndungu, P., and Birungi, G. (2019). Evaluation of persistent organochlorine pesticides and polychlorinated biphenyls in Umgeni River bank soil, KwaZulu-Natal, South Africa. Water sa. 45, 592–607. doi:10.17159/wsa/2019.v45.i4.7540
Gb36600, (2018). National standards of the people's republic of China. Soil Environ. Qual. - Risk control Stand. Contam. Dev. land (Trial).
Ge, X., Ma, S. T., Zhang, X. L., Yang, Y., Li, G. Y., and Yu, Y. X. (2020). Halogenated and organophosphorous flame retardants in surface soils from an e-waste dismantling park and its surrounding area: Distributions, sources, and human health risks. Environ. Int. 139, 105741. doi:10.1016/j.envint.2020.105741
Jiao, X., Chen, S., Deng, Y. J., and Wang, Y. X. (2016). PBDEs dynamic in farmland soils from an E-waste dismantling area in China (in Chinese). Acta Sci. Circumstantiae 36 (12), 4472–4481.
Li, H., Guardia, M. J. L., Liu, H., Hale, R. C., Mainor, T. M., Harvey, E., et al. (2019). Brominated and organophosphate flame retardants along a sediment transect encompassing the Guiyu, China e-waste recycling zone. Sci. Total Environ. 646, 58–67. doi:10.1016/j.scitotenv.2018.07.276
Li N, N., Chen, X. W., Deng, W. J., Giesy, J. P., and Zheng, H. L. (2018). PBDEs and dechlorane plus in the environment of Guiyu, southeast China: A historical location for E-waste recycling (2004, 2014). Chemosphere 199, 603–611. doi:10.1016/j.chemosphere.2018.02.041
Li Q, Q., Lu, Y., Wang, P., Wang, T., Zhang, Y., Suriyanarayanan, S., et al. (2018). Distribution, source, and risk of organochlorine pesticides (OCPs) and polychlorinated biphenyls (PCBs) in urban and rural soils around the Yellow and Bohai Seas, China. Environ. Pollut. 239, 233–241. doi:10.1016/j.envpol.2018.03.055
Li, Y., Chang, Q. M., Luo, Z., Zhang, J., Liu, Y. C., Duan, H. B., et al. (2020). Transfer of POP-BFRs within e-waste plastics in recycling streams in China. Sci. Total Environ. 717, 135003. doi:10.1016/j.scitotenv.2019.135003
Lim, Y. W., Kim, H. H., Lee, C. S., Shin, D. C., Chang, Y. S., and Yang, J. Y. (2014). Exposure assessment and health risk of poly-brominated diphenyl ether (PBDE) flame retardants in the indoor environment of elementary school students in Korea. Sci. Total Environ. 470-471, 1376–1389. doi:10.1016/j.scitotenv.2013.09.013
Liu, C., Wei, B. K., Bao, J. S., Wang, Y., Jin, J., Tang, Y. E., et al. (2019). Polychlorinated biphenyls in the soil–crop–atmosphere system in e-waste dismantling areas in Taizhou: Concentrations, congener profiles, uptake, and translocation. Environ. Pollut. 257, 113622. doi:10.1016/j.envpol.2019.113622
Liu, W., Yuan, Q., and Wang, S. (2021). Distribution characteristics of PCBs in typical soil and sludge in Guanzhong area. J. Northwest Univ. ( Nat. Sci. Ed. 51 (1), 33–42.
Long, E. R., MacDonald, D. D., Smith, S. L., and Calder, F. D. (1995). Incidence of adverse biological effects within ranges of chemical concentrations in marine and estuarine sediments. Environ. Manag. 19 (1), 81–97. doi:10.1007/bf02472006
Mao, S., Liu, S., Zhou, Y., An, Q., Zhou, X., Mao, Z., et al. (2021). The occurrence and sources of polychlorinated biphenyls (PCBs) in agricultural soils across China with an emphasis on unintentionally produced PCBs. Environ. Pollut. 271, 116171. doi:10.1016/j.envpol.2020.116171
Matsukami, H., Suzuki, G., Someya, M., Uchida, N., Tue, N. M., Tuyen, L. H., et al. (2017). Concentrations of polybrominated diphenyl ethers and alternative flame retardants in surface soils and river sediments from an electronic waste-processing area in northern Vietnam, 2012–2014. Chemosphere 167, 291–299. doi:10.1016/j.chemosphere.2016.09.147
Shi, J., Xiang, L., Luan, H., Wei, Y., Ren, H., and Chen, P. (2019). The health concern of polychlorinated biphenyls (PCBs) in a notorious e-waste recycling site. Ecotoxicol. Environ. Saf. 186, 109817. doi:10.1016/j.ecoenv.2019.109817
Sun, J., Hang, T. J., Cao, L., Fan, X. L., Feng, Y. L., Li, T., et al. (2021). Assessment of polybrominated diphenyl ethers and emerging brominated flame retardants in Pheretima (a Traditional Chinese Medicine): Occurrence, residue profiles, and potential health risks. Environ. Pollut. 276, 116680. doi:10.1016/j.envpol.2021.116680
Tombesi, N., Pozo, K., Alvarez, M., Pribylova, P., Kukucka, P., Audy, O., et al. (2017). Tracking polychlorinated biphenyls (PCBs) and polybrominated diphenyl ethers (PBDEs) in sediments and soils from the southwest of Buenos Aires Province, Argentina (South eastern part of the GRULAC region). Sci. Total Environ. 575, 1470–1476. doi:10.1016/j.scitotenv.2016.10.013
USEPA (2008). Regional screening levels for chemical contaminants at superfund sites. Arlington, VA, USA: Chem. Specif. Param.
USEPA (2015). Regional screening levels for chemical contaminants at Superfund sites. Available at: https://www.epa.gov/risk/regional-screening-levels-rsls.
USEPA (2018). Regional screening levels (RSLs)-User's guide. Natl. Cent. Environ. Assess. c/o-Risk Website. Available at: https://www.epa.gov/risk/regional-screening-levels-rsls-usersguide.
Wang, D., Wang, Y., Singh, V. P., Zhu, J., Jiang, L., Zeng, D., et al. (2018). Ecological and health risk assessment of PAHs, OCPs, and PCBs in Taihu Lake basin. Ecol. Indic. 92, 171–180. doi:10.1016/j.ecolind.2017.06.038
Wang, T., Wang, Y. W., Fu, J. J., Wang, P., Li, Y. M., Zhang, Q. H., et al. (2010). Characteristic accumulation and soil penetration of polychlorinated biphenyls and polybrominated diphenyl ethers in wastewater irrigated farmlands. Chemosphere 81 (8), 1045–1051. doi:10.1016/j.chemosphere.2010.07.045
Wei, B. K., Liu, C., Wang, Y., and Jin, J. (2020). Polybrominated diphenyl ether in E-waste dismantling sites in Taizhou city, zhejiang province: Concentration, distribution, and migration trend. Environ. Sci. 41 (10), 4740–4748. doi:10.13227/j.hjkx.202003188
Wu, J., Hu, J. C., Wang, S. J., Jin, J. X., Wang, R., Wang, Y., et al. (2018). Levels, sources, and potential human health risks of pcns, pcdd/fs, and pcbs in an industrial area of shandong province, China. Chemosphere 199, 382–389. doi:10.1016/j.chemosphere.2018.02.039
Wu, Q., Leung, J. Y. S., Du, Y., Kong, D., Shi, Y., Wang, Y., et al. (2019). Trace metals in e-waste lead to serious health risk through consumption of rice growing near an abandoned e-waste recycling site: Comparisons with PBDEs and AHFRs. Environ. Pollut. 247, 46–54. doi:10.1016/j.envpol.2018.12.051
Xu, C., Niu, L., Zou, D., Zhu, S., and Liu, W. (2019). Congener-specific composition of polychlorinated biphenyls (PCBs) in soil-air partitioning and the associated health risks. Sci. Total Environ. 684, 486–495. doi:10.1016/j.scitotenv.2019.05.334
Xu, P., Lou, X., Ding, G., Shen, H., Wu, L., Chen, Z., et al. (2014). Association of pcb, pbde and pcdd/f body burdens with hormone levels for children in an e-waste dismantling area of zhejiang province, China. Sci. Total Environ. 499, 55–61. doi:10.1016/j.scitotenv.2014.08.057
Yang, Z. Z. (2009). Study on the environmental behavior of polybrominated diphenyl ethers in contaminated areas, China. BeiJing, China: Research Center for Eco-environmental Science, Chinese Academy of Science.
Yao, Y. T. (2019). Vertical distribution characteristics and influence factors—taking the area of jie fang zha as an example. Nei Meng Gu, China: Inner Mongolia Agricultural University.
Yu, B. M., Brenda, L. N., Wang, H. S., Leung, A. O. W., Chow, K. L., and Wong, M. H. (2011). Cancer risk assessment of polybrominated diphenyl ethers (PBDEs) and polychlorinated biphenyls (PCBs) in former agricultural soils of Hong Kong. J. Hazard. Mater. 195, 92–99. doi:10.1016/j.jhazmat.2011.08.010
Yu, H. Y., Liu, Y. F., Shu, X. Q., Ma, L. M., and Pan, Y. W. (2020). Assessment of the spatial distribution of organochlorine pesticides (OCPs) and polychlorinated biphenyls (PCBs) in urban soil of China. Chemosphere 243, 125392. doi:10.1016/j.chemosphere.2019.125392
Yu, Y., Lin, B., Liang, W., Li, L., Hong, Y., Chen, X., et al. (2018). Associations between PBDEs exposure from house dust and human semen quality at an e-waste areas in South China-A pilot study. Chemosphere 198, 266–273. doi:10.1016/j.chemosphere.2018.01.150
Zhan, L. X., Lin, T., Cheng, H. R., Wang, Z. W., Cheng, Z. N., Zhou, D., et al. (2019). Atmospheric deposition and air–soil exchange of polybrominated diphenyl ethers (PBDEs) in a background site in Central China. Environ. Sci. Pollut. Res. 26 (31), 31934–31944. doi:10.1007/s11356-019-06312-6
Zhang, L., Wang, J. X., Xu, F., Zhang, G., Wang, Y. J., Zhang, W., et al. (2015). Distribution and source apportionment of polybrominated diphenyl ethers(PBDEs)in soils and dusts in E waste recycling and aurrounding areas. J. Agro-Environment Sci. 34 (009), 1730–1736.
Zhang, S. J., Zhou, X., Li, M. L., and Zhu, G. H. (2018). Pollution characteristic and environmental transport behavior of PBDEs in agriculture soil around an electric waste dismantling venue. Environ. Pollut. Control 40 (7), 819–823.
Keywords: electronic circuit board, soil pollution, PCB, PBDEs, health risk
Citation: Zhang Z, Chen LH, Tao ML, Zhou DD, Zhang Y, Yao J, Kong QN and Guo BB (2023) Occurrence and distribution characteristics of PCBs and PBDEs in farmland soils adjacent to electronic circuit board dismantling ruins. Front. Environ. Sci. 10:1048345. doi: 10.3389/fenvs.2022.1048345
Received: 19 September 2022; Accepted: 08 December 2022;
Published: 04 January 2023.
Edited by:
Jun Wu, Harbin Engineering University, ChinaReviewed by:
Wenjie Ren, Institute of Soil Science (CAS), ChinaCopyright © 2023 Zhang, Chen, Tao, Zhou, Zhang, Yao, Kong and Guo. This is an open-access article distributed under the terms of the Creative Commons Attribution License (CC BY). The use, distribution or reproduction in other forums is permitted, provided the original author(s) and the copyright owner(s) are credited and that the original publication in this journal is cited, in accordance with accepted academic practice. No use, distribution or reproduction is permitted which does not comply with these terms.
*Correspondence: Zhen Zhang, emhhbmd6aGVuLTkxMUAxNjMuY29t
Disclaimer: All claims expressed in this article are solely those of the authors and do not necessarily represent those of their affiliated organizations, or those of the publisher, the editors and the reviewers. Any product that may be evaluated in this article or claim that may be made by its manufacturer is not guaranteed or endorsed by the publisher.
Research integrity at Frontiers
Learn more about the work of our research integrity team to safeguard the quality of each article we publish.