- 1School of Municipal and Environmental Engineering, Resources and Environment Innovation Institute, Shandong Jianzhu University, Jinan, China
- 2School of Life Sciences, Qilu Normal University, Jinan, China
- 3School of Architecture and Urban Planning, Shandong Jianzhu University, Jinan, China
- 4Shandong Industry Research Environmental Technology Co., Ltd., Jinan, China
Mining activities has generated large amounts of mine tailings each year, and these tailings usually contain high concentrations of heavy metal pollutants, which not only cause serious damage to the local and surrounding soil ecosystems, but also harm human health via the transmission of food chain. Phytoremediation is treated as environmentally friendly, long-term effective and low-cost restoration method. However, tailing soil acidification, low organic matter content, poor water holding capacity and compaction make plant struggle to survive. Biochar, a soil conditioner can promote plant growth by improving the physical, chemical and biological properties of soil, thus strengthening the ability of phytoremediation in the contaminated tailings. This review elaborates how the physicochemical properties of biochar affect phytoremediation; and summarized how the raw materials of biochar affect the physicochemical characteristics. Finally, the future research directions are prospected.
1 Introduction
Mining industries is considered the basis of many industrial sectors because it provides energy and raw materials for many others. However, some studies indicated that the increasing demand and low production rate of mine concentrate result in more than 14 billion tons of mine discard waste and tailings are generated in the process of mineral exploitation every year (Adiansyah et al., 2015). Mine tailings usually contain high contamination of heavy metals (HMs) such as cadmium (Cd), lead (Pb), zinc (Zn), copper (Cu), chromium (Cr), cobalt (Co), mercury (Hg), nickel (Ni), and arsenic (As) (Maboeta et al., 2018). These HMs may infiltrate river and soil around mines through wet deposition and leakage from mine tailings ponds (Kowitwiwat and Sampanpanish, 2020). HMs in soil can not only decreasing the number of soil beneficial microorganisms by hamper their enzymatic and metabolic activities, but also having a fatal effect on invertebrates and reptiles by stimulate the formation of reactive oxygen species (Ahmed et al., 2022). This will lead to the destruction of the soil ecosystem balance and the reduction of soil microbial biodiversity. What’s more, HMs can enter and accumulate in surrounding people through the food chain, respiratory inhalation, skin contact and other routes of exposure (Cheng et al., 2020). Some HMs have strong toxic to human organs even in trace amounts, which increases the risk of serious physical diseases and mental illness (Ayuso-Alvarez et al., 2019; Li et al., 2019; Chen et al., 2020). People living downstream of mine tailings may develop nervous system disturbance, pneumonia, kidney inflammation, sesophageal, liver and other cancers attributed to long-term consumption of vegetables, rice and drinking water contaminated with upstream HMs (Shu et al., 2018; Zhao et al., 2022). Considering the potential ecological environment destruction, health hazards and economic losses caused by untreated tailings, it is essential to rehabilitate tailings to lessen its risks.
At present, the developed tailings remediation technology can be divided into physical treatment, chemical remediation and bioremediation. Compare with bioremediation, physical treatment and chemical remediation are costly and usually affect soil properties, fertility, and biodiversity (Shah and Daverey, 2020). For example, during vitrification the tailings soil is melted at high temperatures (>1,300°C) and forms a glass matrix after rapid cooling, which effectively immobilizes various heavy metals (Hu et al., 2021). But the high energy consumption caused by high temperature will make the treatment very costly, not suitable for large-scale tailings remediation, and the remediated tailings lose ecological function which cannot be used for plant cultivation. Phytoremediation in bioremediation is considered to be an environmentally friendly, long-term effective and low-cost method (Praveen and Pandey, 2020). Phytoremediation includes phytoextraction, phytovolatilization, phytoimmobilization and phytostabilization (Liu et al., 2018). Generally, phytoremediation can mainly be divided into two broad techniques: phytoextraction, which relies on the excessive uptake of heavy metals by plants from the soil and their accumulation in branches, shoots and leaves, and phytostabilization, which stabilizes heavy metals to a harmless state by immobilizing them in the soil through the plant root system (Liu et al., 2018). At present, phytostabilization is widely used to stabilize heavy metals in tailings and generally regarded as an effective method to achieve long-term tailings remediation. This remediation technology contributes to the restoration of ecosystem functions, give tailings a certain landscape value and reduce the risk of exposure to HMs of human (Zalesny et al., 2021). The biomass materials obtained from phytoremediation can be used to produce biomass fuels such as bioethanol, biodiesel and biogas, which have good economic value (Sameena and Puthur, 2021). However, due to extreme pH values, high salinity, low organic matter content, low water retention capacity, and high concentrations of HMs make plant are struggle to survive and growth in tailing soil (Wang et al., 2017). This situation makes phytoremediation usually has a longer restoration cycle compared to physical and chemical remediation, which limits the application of phytoremediation. Therefore, how to improve the physicochemical and biological properties of tailings soil to make it suitable for plant growth is of great significance to improve the efficiency of phytoremediation.
Biochar is a kind of carbon-rich material with high specific surface area, abundant pore structure and various surface functional group which has been proven to have positive effects on soil physical, chemical and biological properties (Marcińczyk and Oleszczuk, 2022). In recent years, many researchers have noticed that the combination of biochar and phytoremediation can play a more active role in tailings remediation. Biochar addition to tailings soil can improves soil structure, increases tailings soil fertility and reduces the bioavailability of heavy metals, providing a better environment for the establishment of stable plants, thus improving the efficiency of phytoremediation. The mechanism of biochar assisted phytoremediation in mine tailings remediation and the effects of different biochar types are summarised in short in this paper. We aim to provide suggestions and references for future research by reviewing past related research.
2 Soil physical properties improvement
Tailings are produced by crushing and grinding during the beneficiation process, and it usually consists of sand, fine grained minerals, water and high concentrations of heavy metals (Sun et al., 2018; Pérez et al., 2021). The composition of the tailings determines its poor soil structure, poor water retention, limited nutrient retention, and susceptibility to water erosion (Macdonald et al., 2017). Therefore, biochar can improve the physicochemical and biological properties to promote plant survival and growth if it is used as a soil amendment before the establishment of plants in tailings soils. Most of the experiments reported are potted experiments under greenhouse conditions, in which tailings soil is mixed with a certain mass fraction of biochar in pots, and resistant plants are sown in the pots to study the effect and mechanism of biochar-enhanced phytoremediation by comparing with control leases. This section discusses the research results of biochar to improve soil structure, enhance soil water holding capacity and reduce soil erosion and its related mechanisms.
2.1 Soil structure
Soil structure, the basis of soil material exchange and energy transfer, is the material carrier regulating soil water, fertilizer, air and heat, which has an important influence on plant growth (Šimanský et al., 2019; Shi et al., 2022). Good soil structure can helps plant roots to breathe and absorb water and nutrients. Adding biochar to soil can improve soil structure by decreasing soil bulk density, increase total soil porosity, and promoting the stability of soil aggregates (Islam et al., 2021).
2.1.1 Soil bulk density
Soil bulk density is an indicator of soil compaction, which not only directly affects soil porosity and pore size, but also affects soil aeration, available water, plant available nutrients and microbial activity (Alghamdi, 2018). High soil compaction can inhibit plant growth by affecting plant photosynthetic rate, transpiration rate, stomatal conductance and enzyme activity, resulting in a significant decrease in plant biomass (leaf number, dry mass of stem, leaves and roots) (Grzesiak et al., 2013; Wang et al., 2019). Xiu et al. (2019) found that 3% biochar addition can reduce soil bulk density by 9.93%. Biochar can reduce soil volume density through a variety of mechanisms. Firstly, the bulk density of biochar is much lower than the average bulk density of soil, so mixing biochar with soil can reduce the overall density of soil through dilution effect (Garcia-Perez et al., 2022). Secondly, the abundant hydroxyl and carboxyl groups on the surface of biochar make it have the ability to combine with soil particles to form soil pores (Ayaz et al., 2022). Finally, the increase in organic carbon concentration caused by biochar addition can promote bioturbation, thereby reducing soil bulk density (Blanco-Canqui, 2021).
2.1.2 Soil pore structure
Soil pore structure plays an important role in soil function, affecting not only nutrient transport, air exchange and water storage, but also plant root extension and microbial activities (Yang et al., 2022). The movement of gas and water in soil is mainly affected by the number of pores, the size distribution of pore structure and other geometric features (Fan et al., 2020). Wang et al. (2020) discovered that 3% biochar addition can compared with the control group, soil micropores (5–30 µm) was significantly increased by 12.71%. The increase in total porosity in soil is usually attributed to the highly porous internal structure of biochar particles (Zhou et al., 2018). Besides, biochar can increases the volume of micropores by filling the pores between soils (Herawati et al., 2021). Finally, the accumulation or rearrangement between biochar and surrounding soil particles can also create new pores (Yang and Lu, 2021).
2.1.3 Soil aggregate
Soil aggregate is the basic unit of soil structure and the material basis of soil fertility, which plays an important role in soil structure stability (Tian et al., 2020). Aggregate stability affects many soil properties and processes, including carbon and nitrogen cycling, water and nutrient storage and transport, root penetration and development, and soil erosion (Heikkinen et al., 2019; Fan et al., 2021). Hu et al. (2021) observed that the stability of soil aggregates increased with the increase of biochar application rate. The mean weight diameter of aggregates increased by 49.77%, 78.34%, and 187.56% with the dosage of 2.5%, 5%, and 7% biochar. The addition of biochar brought with a large input of soluble salt like Na+,K+,Ca2+, and Mg2+(Burrell et al., 2016). Increased electrolytes in the soil contribute to the stability of soil aggregates by promoting flocculation of clay particles and binding of individual soil particles to aggregates (Phocharoen et al., 2018). Biochar is rich in organic carbon, which can promote the formation of soil humus, and the short-range attraction exerted by humus can dominate the interaction of clay particles, thus improving the stability of soil aggregates (Huang et al., 2016). Improved soil conditions are conducive to microbial growth and reproduction, and exocytic exudates (binding agents, polysaccharide, and glycoprotein) produced by fungi and bacteria can bond soil particles into large aggregates (Rabbi et al., 2020; Fan et al., 2021).
2.2 Water holding capacity
Soil water holding capacity (SWHC) is one of the most important factors in the soil properties because they affect the plant available water that supports plant physiological activities such as photosynthesis and transpiration (Atkinson and Aitkenhead, 2018). Basso et al. (2013) found that the addition of biochar increased soil water holding capacity by 23% relative to the control. The study by Ghassemi-Golezani and Farhangi-Abriz (2022) found that the mechanism of biochar in increasing soil water holding capacity may be related to its specific physical and chemical properties such as high specific surface area and porosity. Biochar affects soil water holding capacity through various mechanisms, but the increase of micropores structure and total soil porosity are considered to be the main mechanisms (Bikbulatova et al., 2018). The macropore (>80 µm) contribute to facilitate the rapid flow of water through the soil under the action of gravity but micropores (5–30 µm) can hold water by capillary forces (Li et al., 2021; Zhao and Hu, 2021). At the same time, the relatively high hydrophilicity of some biochar and the abundant oxygen-containing functional groups on the surface can also increase soil water retention (Zhang et al., 2019a). In addition, the addition of biochar will improve the aggregation and stability of soil aggregates, thus affecting the water retention capacity of soil (Batista et al., 2018). Finally, the increase of soil organic matter caused by biochar addition can slow down soil evaporation and water infiltration (Lal, 2020).
2.3 Soil erosion and nutrient loss
Tailings usually have a loose structure and a large accumulation slope, resulting in erosion or even crumbling and landslides when subjected to heavy rainfall during the rainy season (Wang et al., 2017). Soil erosion will cause the already inadequate fertility of the tailings soil to continue to decline, especially a large loss of organic matter in the soil. The loss of rich nutrients in the soil means that there are not enough nutrients for plant growth. Biochar is considered to have the potential to improve soil lose and nutrient retention (Crews and Rumsey, 2017). Hseu et al. (2014) noted that with the increase of biochar application rate, soil loss decreased significantly. Under extreme rainfall events, biochar incorporation could reduce soil loss content by at least 35%. Increase in soil surface roughness and improvement in soil properties, including porosity, aggregate stability, organic matter concentration and hydraulic conductivity, are considered to be the main factors in reducing soil erosion (Blanco-Canqui, 2021). Rubin et al. (2020) noted that 10% woody biochar reduced cumulative ammonium leaching by 65% and nitrate leaching by 92%. The reduction in inorganic nitrogen leaching may mainly be due to retention and adsorption on the surface area and pore space of the biochar (Feng et al., 2020). Peng et al. (2021) discovered that the application of 4% corn stover biochar can reduce the accumulation of leached total phosphorus by 20.2% compared to the control soil. Biochar affects phosphorus leaching losses directly or indirectly by adsorption of phosphorus, increasing phosphorus retention in the soil and promoting assimilation of phosphorus by plants (Yang et al., 2021a).
3 Soil chemical properties improvement
Extreme pH, low levels of nutrients and high concentrations of heavy metals in tailings soils limit plant germination, growth and development. Biochar has stable chemical properties, can exist in soil for a long time, and has the potential to improve soil chemical properties for a long time. Biochar enhances phytoremediation by neutralizing soil pH, providing nutrient support, and reducing heavy metal bioavailability. In this section of this paper, the research results and related mechanisms of biochar in improving soil pH and fertility and reducing heavy metal bioavailability are discussed.
3.1 Soil pH
The pH of tailings is keep low in normal or natural condition due to the large amount of acidic wastewater produced by tailings, especially sulfide tailings (Yin et al., 2021; Saedi et al., 2022). Acidification of tailings soil can lead to the mobility of heavy metals and toxicity to plants increased, and lead to plant deficiency of P, Ca, K, Mg, and Mo (Dai et al., 2017). Many studies have reported a significant increase in soil pH after biochar application (De la Rosa et al., 2022). Aamer et al. (2020) observed that 2% biochar application increased soil pH from 5.48 to 6.11. There are three main mechanisms by which biochar raises soil pH and reduces exchange acidity: first, the carbonate or oxide formed during pyrolysis can reacts with H+; then the high pH of biochar itself can neutralize the acid in the soil; finally, various functional groups such as hydroxyl and carboxyl groups on the surface of biochar can absorb H+ too (Lu et al., 2022).
The addition of biochar not only increased soil pH, but also enhanced soil pH buffering capacity (pHBC). The pHBC is important for maintaining the stability of soil properties and the stability of the whole ecosystem because it affects many soil reaction processes, such as the mineralization of organic matter; the activity of soil microorganisms and the available form of heavy metals (Dvorackova et al., 2022). Shi et al. (2017) discovered that 5% application of corn stover biochar increased the pHBC by 86.34%. The mechanism of biochar enhancing soil pHBC may be through increasing soil cation exchange capacity (CEC) and soil organic matter content (SOM) (Dai et al., 2017).
3.2 Soil fertility
Poor nutrients in tailings are one of the main constraints to phytoremediation. The addition of biochar can directly or indirectly increase soil fertility, thus increasing germination, early growth, root and stem length, and chlorophyll content of plants (Shamim et al., 2018).
3.2.1 Soil organic matter
SOM, such as humus and humus-like substances, can be mineralized to release nutrients for assimilation by plants and microorganisms (Ding et al., 2016). SOM plays an important role in maintaining soil quality and sustaining soil ecosystems, and its content affects many physical and chemical properties of the soil, such as aggregate stability, water retention capacity, and pH (Simansky et al., 2016). However, most tailings are severely deficient in organic matter, with many tailings having less than 1% organic matter (Lin et al., 2021a; Qin et al., 2022). Li et al. (Hua et al., 2014) found that soil improvement by biochar could effectively increase soil organic matter, and the degree of impact increased with the increase of biochar. The addition of 8% biochar could increase organic matter content by 41%–75%. Biochar can reduce the decomposition of soil organic matter and thus promote carbon sequestration in low organic carbon soils (Fatima et al., 2020). Liu et al. (2022b) found that 1% addition of wheat straw biochar reduced CO2 emissions from soil organic matter mineralization by 28%. Biochar is rich in organic compounds, especially unstable organic compounds, which affect soil organic matter by providing a usable energy source for microbial metabolism, growth and reproduction (Liu et al., 2022a). In addition, biochar can stabilize the organic matter content of the soil by inhibiting the mineralization of organic matter. Biochar can forming stable organic-inorganic complexes through adsorbing labile OC, and controlling β-Glucosidase activity related to soil organic matter degradation, thereby promoting the formation and stabilization of soil SOM (Luo et al., 2020; Liu et al., 2022b). Although biochar incorporation can increase the biomass of soil microorganisms, the increased efficiency of microbial utilization of unstable C can protect SOC and promote carbon sequestration (Fatima et al., 2020). Finally, biochar has a huge specific surface area, rich pore structure and functional groups such as hydroxyl and carboxyl groups, which make it have a strong adsorption capacity and can improve the ability of soil to adsorb and retain organic matter (Cen et al., 2021).
3.2.2 Cation exchange capacity
CEC represents the ability of soil to retain positively charged ions and is the main source of soil buffering capacity (Razzaghi et al., 2021). CEC can be used as an index to evaluate the ability of soil to retain and release nutrients, such as ammonium, nitrate, P, Mg, and Ca (Mattila and Rajala, 2022). Kaur and Sharma (2019) noticed that the addition of 10% biochar could significantly increase soil CEC by 26.54%–33.18%. The main mechanism of increasing CEC by adding biochar may be that the surface negative charge and charge density of biochar increase the negative charge density of soil (Hailegnaw et al., 2019). In addition, biochar surface oxidation functional groups such as carboxylic acids, lactones and phenols contribute to increasing the negatively charged biochar density (Domingues et al., 2020). SOM is a rich source of soil negative charge, and the addition of biochar can enhance soil CEC by increasing soil organic matter especially humic acid (Šimanský et al., 2017). The increase in soil pH after the addition of biochar may lead to deprotonation of functional groups of minerals, resulting in more negative charges, contributing to the improvement of CEC (Hailegnaw et al., 2019).
3.2.3 N and P
On the one hand the tailings are deficient in nitrogen and phosphorus, on the other hand the plant available forms of these nutrients are easily lost through leaching, conversion to gaseous forms and in precipitation reactions (Gul and Whalen, 2016). Biochar improves soil nitrogen retention and provides more effective phosphorus, while reducing soil losses of nitrogen and phosphorus, thereby promoting plant growth and nutrient uptake. Xu et al. (2016) found that application of 2%, 4%, and 8% corn stover biochar reduced total leached N accumulation by 18.8%, 19.5%, and 20.2%. Gonzaga et al. (2022) observed that the addition of sludge biochar increased the availability P concentration from 60.20 to 124.36 mg kg−1 in bulk soils and from 192.9 to 245.9 mg kg−1 in the rhizosphere. Biochar improves soil nitrogen fixation and reduces soil nitrogen fixation losses through mechanisms such as biotic (increases in microbial biomass and activity) and abiotic (adsorption of NO3−, NH4+ and organic nitrogen by biochar) nitrogen fixation, enhanced nitrogenation, reduced leaching, and denitrification (Asadyar et al., 2021). Although these processes may reduce plant utilization of N for a short period of time, recent evidence suggests that N adsorbed by biochar can eventually be used by plants (Bai et al., 2015). Soil N may be mineralized to inorganic N when there is not enough N in the soil for plant use or microbial activity (Liu et al., 2017). At the same time, biochar improves the nitrogen use efficiency of plants, thereby promoting plant growth (Sathe et al., 2021). Biochar contains various organic and inorganic forms of P that can be used as a source of these nutrients to provide available P to the soil (Sui et al., 2022). In addition, biochar can activate soil endogenous phosphorus by affecting P-related complexation and metabolic effects, and increase the content of available P in soil (Yang et al., 2021b). Biochar can also improve soil phosphorus retention by adsorbing phosphorus and releasing it gradually to make it available to plants (Matin et al., 2020).
3.3 Heavy metal bioavailability
Exposure to high concentration of heavy metals in tailings will lead to the gradual reduction of plant biomass and photosynthetic activity, which has a serious stress on the survival, growth or reproduction of colonizing plants (Ibrahim et al., 2022). The addition of biochar can reduce the uptake of heavy metals by plants by significantly reducing the mobility and bioavailability of heavy metals, thereby alleviating plant stress and promoting plant growth. Ibrahim et al. (2022) discovered that the addition of 4% Casuarina biochar could decreased the concentrations of Cd, Cu, Pb, and Zn by 37.2%, 40.2%, 89.2%, and 35.5% in the shoot, and by 25.7%, 32.3%, 85.0%, and 25.2% in the root. Biochar can directly adsorb and fix heavy metals in tailings. The abundant functional groups, porous structure, and ionic charge on the surface of biochar contribute to the fixation of cationic metals in the soil by co-precipitation, surface functional groups complexation, ion exchange, physical adsorption and π-π-electron interactions (Jia et al., 2022). The improvement of soil by biochar, including the increase of soil pH, is an important factor affecting the bioavailability of heavy metals in soil. The increase of pH value promotes the transformation of heavy metals from exchangeable state to bound state and other stable forms (Irshad et al., 2020). In addition, tailings are rich in oxides such as silica, whose charge depends on pH (Fashola et al., 2019). The increase of pH lead to the deprotonation of acidic functional groups (hydroxyl and carboxyl groups, etc.) of tailing particles, resulting in more negative charges of tailing particles, thus enhancing the adsorption capacity of tailing for heavy metals (Yan et al., 2021c). Finally, biochar affects the redox potential of heavy metals, for example, biochar converts Cr6+ to Cr3+ which is less toxic and mobile by continuously transferring electrons (Xu et al., 2020; Narayanan and Ma, 2022).
4 Soil biological properties improvement
In recent years, many researchers have found that tailings native microbial communities can enhance plant tolerance and improve plant colonization in tailings soils (Gazitua et al., 2021). Microorganisms in tailings soils can affect the physical and chemical properties of plant roots and influence the uptake of nutritions and heavy metals by plants (Shen et al., 2022). Application of biochar can enhance phytoremediation by influencing microbial abundance and diversity. This section discusses the role of microorganisms in phytoremediation and the findings and related mechanisms of the action of biochar in enhancing microbial activity and abundance.
4.1 Role of microorganisms in phytoremediation
Microorganisms especially root microorganisms play a crucial role in the process of tailings phytoremediation, such as decomposition of organic matter and conversion of plant available nutrients, formation and stabilization of soil aggregates, fixation of heavy metals, and suppression of plant diseases (Bhanse et al., 2022). Microorganisms can mineralize organic matter, converting organic nitrogen and organic phosphorus into orthophosphate anions (HPO42−and H2PO41−) and inorganic nitrogen (NO3−and NH4+), the major forms of nitrogen and phosphorus that are absorbed by plants (Richardson et al., 2009). Soil microorganisms can bind to soil particles through mycelium or play the role of as binder for soil aggregate in soil particles by secreting insoluble extracellular compounds (Ren et al., 2022). Microorganisms reduce tailings heavy metal bioavailability through direct and indirect mechanisms, including intracellular and extracellular chelation, enzymatic transformation, methylation, alkylation and metal dealkylation, and adsorption of polymers from the cell surface (Fashola et al., 2019). Besides, the addition of biochar can lead to antagonism, competition or parasitism between microorganisms and pathogens in soil by improving soil physicochemical conditions and controlling soil microbial community structure (Gao et al., 2019). The reduction in the number of pathogens can reduce plant morbidity and inhibit the damage to plants. Finally, root microorganisms induce the activities of peroxidase enzymes such as catalase (CAT), peroxidase (POD), peroxidase and polyphenol oxidase (PPO) to improve plant tolerance to heavy metals (Benidire et al., 2021).
4.2 Microbial abundance and diversity
Biochar application can significantly promote the growth of soil microorganisms, increase the abundance of microbial communities and affect the diversity of microbial communities (Hua et al., 2021). Li et al. (2022) found that adding 2% tobacco stalk biochar could increase the microbial biomass of carbon and nitrogen in soil by 87.28% and 54.33%. Yang et al. (2022) found that the use of 1% rice straw biochar prepared at 550°C increased the Shannon diversity index and decreased the Simpson index. In biochar modified soil, the Shannon index of bacteria increased by 3.1% and Simpson index decreased by 29.0%, and the Shannon index of fungi increased by 22.6% and Simpson index decreased by 69.2%. The addition of biochar will bring unstable carbon derived from the pyrolysis of incomplete biomass, which is preferentially used by soil microbial communities, thus stimulating the growth, activity and reproduction of microorganisms (Plaza et al., 2016; Luo et al., 2020). Second, the porous structure and large surface area of biochar provide habitat for soil microorganisms, protecting them from desiccation and predation by large soil organisms (Poveda et al., 2021). Final, biochar can play an indirect role in microbial abundance by altering other environmental factors, such as soil pH and heavy metal availability (Yang et al., 2022). An overview of the improvements in the physical, chemical and biological properties of the soil resulting from the addition of biochar is shown in Figure 1.
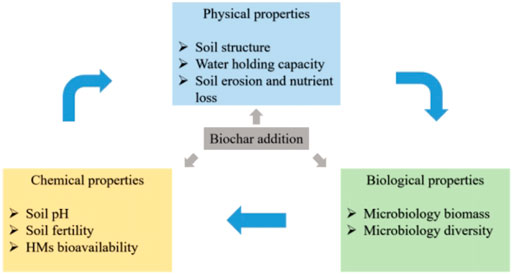
FIGURE 1. The improvement of soil physical, chemical and biological properties caused by biochar addition.
5 Plant biomass and roots
Plant biomass and roots play an important role and can significantly affect phytoremediation efficiency (Niu et al., 2021). This section discusses the role of plant biomass and roots in phytoremediation along with the findings and the associated mechanisms of the role of biochar in enhancing plant biomass and promoting root development.
5.1 Role of plant biomass and roots in phytoremediation
Plant biomass is a key factor affecting phytoremediation potential. The increase of plant biomass can dilute the content of heavy metals in plant tissues to reduce their damage (Cheng et al., 2020). Besides, roots play an important role in plant growth because well-developed roots are better able to absorb and transfer water and nutrients from the soil, contributing to above-ground growth. The enhanced photosynthesis of aboveground leaves can provide sufficient nutrients for root growth, thus forming a virtuous cycle. Phytoremediation efficiency is strongly dependent on the nature of root secretions, because root secretions, including hydrogen ions, organic acid anions, phytochelators, enzymes, and carbon-containing metabolites, which can promote complex formation and reduce heavy metal toxicity through chelation or hydrogen bonding (Yang et al., 2020). These roots secretions also attract beneficial rhizosphere growth-promoting bacteria, which facilitate plant growth under stress (Liu et al., 2021).
5.2 Plant biomass and roots
Biochar addition promotes plant biomass increase and root development. Ren et al. (2021) found that the application of biochar not only increased the total root area by 91.35%, but also increased the net photosynthetic rate by 77.3% and the total biomass by 72.5%. Biochar-induced improvement in root growth and development is associated with improved soil structure and enhanced availability of nutrients (Chang et al., 2021). In addition, it has recently been found that the addition of biochar can also change the gene expression of plants, downregulating the biosynthesis pathway defense genes and up-regulating the growth promoting genes (Li et al., 2016). Finally, biochar can inhibit pathogen growth, survival, virulence and activity by inducing systemic plant defense responses, improving soil nutrient availability, regulating soil microbial community structure, and adsorbing enzymes and organic acids produced by pathogenic microorganisms (Alaylar et al., 2021; Hou et al., 2022). Figure 2 shows the improvement in plant growth due to the addition of biochar.
6 Effects of different biochar characteristics and used dosage
6.1 Characteristics of biochar
Many researchers have confirmed that different biochar feedstock type, preparation process and used dosage can have varying degrees of impact on the improvement of physical, chemical and biological properties. The previous sections has explained in detail how the physicochemical properties of biochar determine its potential to enhance phytoremediation. But the physicochemical properties of biochar are directly affected by the type of biomass raw material and pyrolysis conditions. Therefore, the comparison of biochar prepared from different raw materials and under different pyrolysis conditions is the basis for the application of biochar to enhance the phytoremediation of tailings pollution.
6.1.1 Feedstock type
By summarizing relevant studies, it can be found that the mainly biomass sources of biochar preparation are crop residues, forestry biomass and organic solid waste, mainly including straw, seed husk, pericarp, wood chips, bark, branches, animal waste and sludge. Plant biomass is normally characterized by low ash content, high calorific value, high bulk density and small void fraction, while organic solid waste is generally characterized by high ash content, low calorific value, low bulk density and high void fraction (Jafri et al., 2018). Sludge biochar have been targeted for particular focus because of its phosphorus-rich properties, but there is a risk of contamination with heavy metals remaining in it after preparation (Zhang et al., 2021).
In general, the specific surface area and porosity of organic solid waste biochar are much smaller than that of plant biochar, which are mainly related to the release of volatiles during pyrolysis: excessive ash will block pores, especially micropores, resulting in a decrease in surface area and porosity (Ji et al., 2022). Compared with cellulose and hemicellulose, lignin is more stable in the pyrolysis process, so lignin-rich biomass will produce biochar with macroporous structure, while cellulose-rich biomass mainly produces biochar with microporous structure (Li et al., 2017). The larger volatile substances content of plant-based biochar compared with organic solid waste biochar at relatively low temperature is due to the presence of hemicellulose and cellulose (Li et al., 2018). Organic solid wastes often contain a large number of inorganic minerals, and the precipitation of soluble alkali metal salts and mineral components in the pyrolysis process leads to the increase of organic solid waste biochar ash and pH value (Xu et al., 2022). Biochar derived from organic solid waste showed more CEC and oxygenated surface functional groups than biochar derived from plants, possibly because the alkali metals in the raw material promoted the generation of oxygenated surface functional groups (Murtaza et al., 2022). Compared with biochar from non-woody materials, wood biochar has lower ash content but higher C content, aromaticity and stability because carbon compounds such as lignin, which are rich in the raw material, are not easily degraded during pyrolysis (Xu et al., 2022). The content of N and P in organic solid waste biochar such as manure is usually high, which may be due to the high content of protein and free amino acid in the raw material, and the decomposition of protein and free amino acid will release N and P (Ji et al., 2022).
6.1.2 Pyrolysis conditions
Among many pyrolysis conditions that affect the physicochemical properties of biochar, such as pyrolysis temperature, heating rate, residence time and pyrolysis atmosphere, pyrolysis temperature is one of the most influential factors (Shaaban et al., 2014). In general, biochar prepared at higher temperatures has higher C content, specific surface area, pore volume, ash, aromaticity and thermal stability, while biochar prepared at lower temperatures has more O and H content, yield, hydrophilic, volatile substances, acidic functional groups, available nitrogen and CEC (Zhao et al., 2017).
Higher pyrolysis temperature is conducive to the release of volatile substances from the raw material and the formation and volatilization of intermediate melt, thus forming more micropore volume and larger specific surface area, but also leads to the decrease of biochar production (Shaaban et al., 2014). Because more water and oxygen-containing groups are removed during pyrolysis, biochar prepared at high pyrolysis temperature has high C content, aromaticity, stability and low hydrophilicity (Lin et al., 2021b). The increase in ash content at high pyrolysis temperature is due to the combustion of organic matter and the residue of inorganic components (Tomczyk et al., 2020). The increase in pH with increasing temperature is mainly due to more alkali metal salt in ash produced during pyrolysis, and additional support is provided by the appearance of basic functional groups, the disappearance of acidic functional groups and the release of alkali metal salts from the raw material (Murtaza et al., 2022). Due to the dehydration and deoxygenation of biomass raw materials, the content of oxygen-containing functional groups is low (Tomczyk et al., 2020). The CEC of biochar depends on the nature and distribution of oxygen-containing functional groups on the surface of biochar, so the CEC of biochar decreases with the increase of pyrolysis temperature (Liang et al., 2016). The phosphorus contents of biochar increased with the increase of pyrolysis temperature, mainly because high temperature caused the decomposition of biomass structure, resulting in higher residual phosphate (Yang and Lu, 2021). The continuous decrease of N content in biochar with the increase of pyrolysis temperature is due to the high temperature leads to the escape of N element in the form of non-coagulable forms such as HCN and NH3 or organic N in the coagulable liquid phase (Xu et al., 2021).
6.2 Used dosage
The effects of biochar on tailing soil and plants are closely related to the amount of biochar added. Shi et al. (2022) found that the addition of appropriate amounts of biochar would improve soil structure, including increasing soil porosity, stabilizing soil triple comparison, promoting the formation of soil aggregates and improving the stability of aggregates. But if the application of biochar is too large, it may reduce the effect of soil structure improvement. Liu et al. (2020) noted the application of 5% biochar stimulated plant growth and recorded the maximum biomass. However, if the amount of biochar applied is higher than this level, biochar can inhibit plant growth. Excessive addition of biochar may inhibit plant growth. This may be due to the too high water holding capacity of the soil and less oxygen in the pore space, which leads to the anaerobic state of plant roots (Chang et al., 2021). It is also possible that the adsorption capacity of biochar is too strong in the early stage, which slows the release of available nutrients in the later stage, resulting in a lack of fertilizer (Li et al., 2022). This is also may be related to the formation of toxic compounds during the charring process, such as polycyclic aromatic hydrocarbons (PAHs) (Mikajlo et al., 2022).
The reduced bioavailability of heavy metals through biochar application may be beneficial for the establishment of plant mulch in tailings soils for long-term phytostabilization (Forjan et al., 2018). Table 1 summarizes the research progress of biochar application in phytoremediation including different biomass sources, different pyrolysis conditions and different dosing rates.
7 Conclusion and future prospects
This article reviews how the addition of biochar causes changes in soil physical, chemical and biological properties, and how these changes have salutary effect on enhanced phytoremediation. The addition of biochar will simultaneously affect physical, chemical and biological properties, and these properties will also interact with each other to jointly strengthen the effect of phytoremediation. The application of biochar is beneficial to reduce the mobility and bioavailability of heavy metals in tailings, improve soil structure, enhance soil fertility, promote plant growth, and promote soil microbial activity. Therefore, in order to improve soil properties before large-scale biochar utilization, more extensive studies on biochar-soil-plant systems are needed to promote the effect of phytoremediation of heavy metal-contaminated soil, so as to enhance phytoremediation. Future research should focus on the following issues that need attention: First, at present, many studies are limited to laboratory scale and conducted under greenhouse conditions, so there is a lack of field studies to evaluate the ability of biochar to enhance. Second, microorganisms play an important role on phytoremediation, so more research should be emphasized on the biochar-soil-microbe-plant interaction. Third, whether the pollutants (PAHs and HMs) may carried by biochar, especially modified biochar, will cause secondary pollution to the environment is a problem worthy of attention.
Author contributions
YS and YZ performed the experiments and wrote the paper. HY, JS, JZ contributed to the Data analysis and picture drawing. XZ and BL conceived and designed the paper. XZ and YS supported the funding.
Funding
This research was supported by National key research and development Program (SQ2021YFE012527) Research and development project of the Ministry of housing and urban rural development (K20210424), National science foundation of Shandong Province (No. ZR2020ME236), Natural Science Foundation of Shandong Province (ZR202111300353), Doctoral Research Fund of Shandong Jianzhu University and Doctoral Research Fund of Qilu Normal University.
Acknowledgments
The authors would like to thank all the referees for their constructive comments and suggestions.
Conflict of interest
Author JS was employed by the company Shandong Industry Research Environmental Technology Co., Ltd.
The remaining authors declare that the research was conducted in the absence of any commercial or financial relationships that could be construed as a potential conflict of interest.
Publisher’s note
All claims expressed in this article are solely those of the authors and do not necessarily represent those of their affiliated organizations, or those of the publisher, the editors and the reviewers. Any product that may be evaluated in this article, or claim that may be made by its manufacturer, is not guaranteed or endorsed by the publisher.
References
Aamer, M., Shaaban, M., Hassan, M. U., Huang, G. Q., Liu, Y., Tang, H. Y., et al. (2020). Biochar mitigates the N2O emissions from acidic soil by increasing the nosZ and nirK gene abundance and soil pH. J. Environ. Manag. 255, 109891. doi:10.1016/j.jenvman.2019.109891
Adiansyah, J. S., Rosano, M., Vink, S., and Keir, G. (2015). A framework for a sustainable approach to mine tailings management: Disposal strategies. J. Clean. Prod. 108, 1050–1062. doi:10.1016/j.jclepro.2015.07.139
Ahmed, S., Fatema Tuj, Z., Mahdi, M. M., Nurnabi, M., Alam, M. Z., and Choudhury, T. R. (2022). Health risk assessment for heavy metal accumulation in leafy vegetables grown on tannery effluent contaminated soil. Toxicol. Rep. 9, 346–355. doi:10.1016/j.toxrep.2022.03.009
Alaylar, B., Güllüce, M., Egamberdieva, D., Wirth, S., and Bellingrath-Kimura, S. D. (2021). Biochar mediated control of soil-borne phytopathogens. Environ. Sustain. 2021 (4), 329–334. doi:10.1007/s42398-021-00187-5
Alghamdi, A. G. (2018). Biochar as a potential soil additive for improving soil physical properties—A review. Arab. J. Geosci. 11 (24), 766. doi:10.1007/s12517-018-4056-7
Asadyar, L., Xu, C. Y., Wallace, H. M., Xu, Z., Reverchon, F., and Bai, S. H. (2021). Soil-plant nitrogen isotope composition and nitrogen cycling after biochar applications. Environ. Sci. Pollut. Res. 28 (6), 6684–6690. doi:10.1007/s11356-020-11016-3
Atkinson, C. J., and Aitkenhead, M. (2018). How good is the evidence that soil-applied biochar improves water-holding capacity? Soil Use Manag. 34 (2), 177–186. doi:10.1111/sum.12413
Ayaz, M., Feiziene, D., Feiza, V., Tilvikiene, V., Baltrenaite-Gediene, E., and Khan, A. (2022). The impact of swine manure biochar on the physical properties and microbial activity of loamy soils. Plants (Basel) 11 (13), 1729. doi:10.3390/plants11131729
Ayuso-Alvarez, A., Simon, L., Nunez, O., Rodriguez-Blazquez, C., Martin-Mendez, I., Bel-Lan, A., et al. (2019). Association between heavy metals and metalloids in topsoil and mental health in the adult population of Spain. Environ. Res. 179, 108784. doi:10.1016/j.envres.2019.108784
Bai, S. H., Reverchon, F., Xu, C. Y., Xu, Z. H., Blumfield, T. J., Zhao, H. T., et al. (2015). Wood biochar increases nitrogen retention in field settings mainly through abiotic processes. Soil Biol. Biochem. 90, 232–240. doi:10.1016/j.soilbio.2015.08.007
Basso, A. S., Miguez, F. E., Laird, D. A., Horton, R., and Westgate, M. (2013). Assessing potential of biochar for increasing water-holding capacity of sandy soils. GCB Bioenergy 5 (2), 132–143. doi:10.1111/gcbb.12026
Batista, E., Shultz, J., Matos, T. T. S., Fornari, M. R., Ferreira, T. M., Szpoganicz, B., et al. (2018). Effect of surface and porosity of biochar on water holding capacity aiming indirectly at preservation of the Amazon biome. Sci. Rep. 8, 10677. doi:10.1038/s41598-018-28794-z
Benidire, L., Madline, A., Pereira, S. I. A., Castro, P. M. L., and Boularbah, A. (2021). Synergistic effect of organo-mineral amendments and plant growth-promoting rhizobacteria (PGPR) on the establishment of vegetation cover and amelioration of mine tailings. Chemosphere 262, 127803. doi:10.1016/j.chemosphere.2020.127803
Bhanse, P., Kumar, M., Singh, L., Awasthi, M. K., and Qureshi, A. (2022). Role of plant growth-promoting rhizobacteria in boosting the phytoremediation of stressed soils: Opportunities, challenges, and prospects. Chemosphere 303 (1), 134954. doi:10.1016/j.chemosphere.2022.134954
Bikbulatova, S., Tahmasebi, A., Zhang, Z., Rish, S. K., and Yu, J. (2018). Understanding water retention behavior and mechanism in bio-char. Fuel Process. Technol. 169, 101–111. doi:10.1016/j.fuproc.2017.09.025
Blanco-Canqui, H. (2021). Does biochar application alleviate soil compaction? Review and data synthesis. Geoderma 404, 115317. doi:10.1016/j.geoderma.2021.115317
Burrell, L. D., Zehetner, F., Rampazzo, N., Wimmer, B., and Soja, G. (2016). Long-term effects of biochar on soil physical properties. Geoderma 282, 96–102. doi:10.1016/j.geoderma.2016.07.019
Cen, R., Feng, W., Yang, F., Wu, W., Liao, H., and Qu, Z. (2021). Effect mechanism of biochar application on soil structure and organic matter in semi-arid areas. J. Environ. Manage. 286, 112198. doi:10.1016/j.jenvman.2021.112198
Chang, Y., Rossi, L., Zotarelli, L., Gao, B., Shahid, M. A., and Sarkhosh, A. (2021). Biochar improves soil physical characteristics and strengthens root architecture in Muscadine grape (Vitis rotundifolia L.). Chem. Biol. Technol. Agric. 8 (1), 7. doi:10.1186/s40538-020-00204-5
Chen, H., Tang, L., Wang, Z., Su, M., Tian, D., Zhang, L., et al. (2020). Evaluating the protection of bacteria from extreme Cd (II) stress by P-enriched biochar. Environ. Pollut. 263, 114483. doi:10.1016/j.envpol.2020.114483
Cheng, S., Chen, T., Xu, W. B., Huang, J., Jiang, S. J., and Yan, B. (2020). Application research of biochar for the remediation of soil heavy metals contamination: A review. Molecules 25 (14), 3167. doi:10.3390/molecules25143167
Crews, T., and Rumsey, B. (2017). What agriculture can learn from native ecosystems in building soil organic matter: A review. Sustainability 9 (4), 578. doi:10.3390/su9040578
Dai, Z., Zhang, X., Tang, C., Muhammad, N., Wu, J., Brookes, P. C., et al. (2017). Potential role of biochars in decreasing soil acidification - a critical review. Sci. Total Environ. 581-582, 601–611. doi:10.1016/j.scitotenv.2016.12.169
De la Rosa, J. M., Santa-Olalla, A., Campos, P., Lopez-Nunez, R., Gonzalez-Perez, J. A., Almendros, G., et al. (2022). Impact of biochar amendment on soil properties and organic matter composition in trace element-contaminated soil. Int. J. Environ. Res. Public Health 19 (4), 2140. doi:10.3390/ijerph19042140
Ding, Y., Liu, Y., Liu, S., Li, Z., Tan, X., Huang, X., et al. (2016). Biochar to improve soil fertility. A review. Agron. Sustain. Dev. 36 (2), 36. doi:10.1007/s13593-016-0372-z
Domingues, R. R., Sánchez-Monedero, M. A., Spokas, K. A., Melo, L. C. A., Trugilho, P. F., Valenciano, M. N., et al. (2020). Enhancing cation exchange capacity of weathered soils using biochar: Feedstock, pyrolysis conditions and addition rate. Agronomy 10 (6), 824. doi:10.3390/agronomy10060824
Dvorackova, H., Dvoracek, J., Gonzalez, P. H., and Vlcek, V. (2022). Effect of different soil amendments on soil buffering capacity. Plos One 17 (2), e0263456. doi:10.1371/journal.pone.0263456
Fan, R., Zhang, B., Li, J., Zhang, Z., and Liang, A. (2020). Straw-derived biochar mitigates CO2 emission through changes in soil pore structure in a wheat-rice rotation system. Chemosphere 243, 125329. doi:10.1016/j.chemosphere.2019.125329
Fan, X., Pan, H., Ping, Y., Jin, G., and Song, F. (2021). The underlying mechanism of soil aggregate stability by fungi and related multiple factor: A review. Eurasian Soil Sc. 55 (2), 242–250. doi:10.1134/s1064229322020065
Fashola, M. O., Ngole-Jeme, V. M., and Babalola, O. O. (2019). Heavy metal immobilization potential of indigenous bacteria isolated from gold mine tailings. Int. J. Environ. Res. 14 (1), 71–86. doi:10.1007/s41742-019-00240-6
Fatima, S., Riaz, M., Al-Wabel, M. I., Arif, M. S., Yasmeen, T., Hussain, Q., et al. (2020). Higher biochar rate strongly reduced decomposition of soil organic matter to enhance C and N sequestration in nutrient-poor alkaline calcareous soil. J. Soils Sediments 21 (1), 148–162. doi:10.1007/s11368-020-02753-6
Feng, Y., Yang, X., Singh, B. P., Mandal, S., Guo, J., Che, L., et al. (2020). Effects of contrasting biochars on the leaching of inorganic nitrogen from soil. J. Soils Sediments 20 (8), 3017–3026. doi:10.1007/s11368-019-02369-5
Forjan, R., Rodriguez-Vila, A., Pedrol, N., and Covelo, E. F. (2018). Application of compost and biochar with Brassica juncea L. To reduce phytoavailable concentrations in a settling pond mine soil. Waste Biomass Valorization 9 (5), 821–834. doi:10.1007/s12649-017-9843-y
Gao, Y., Lu, Y., Lin, W., Tian, J., and Cai, K. (2019). Biochar suppresses bacterial wilt of tomato by improving soil chemical properties and shifting soil microbial community. Microorganisms 7 (12), 676. doi:10.3390/microorganisms7120676
Garcia-Perez, T., Pelaez-Samaniego, M. R., Delgado-Noboa, J., and Chica, E. J. (2022). Combined effect of biochar and fertilizers on andean highland soils before and after cropping. Sustainability 14 (14), 8912. doi:10.3390/su14148912
Gazitua, M. C., Morgante, V., Poupin, M. J., Ledger, T., Rodriguez-Valdecantos, G., Herrera, C., et al. (2021). The microbial community from the early-plant colonizer (Baccharis linearis) is required for plant establishment on copper mine tailings. Sci. Rep. 11 (1), 10448. doi:10.1038/s41598-021-89769-1
Ghassemi-Golezani, K., and Farhangi-Abriz, S. (2022). Improving plant available water holding capacity of soil by solid and chemically modified biochars. Rhizosphere 21, 100469. doi:10.1016/j.rhisph.2021.100469
Gonzaga, M. I. S., Santos, J. C. D., de Almeida, A. Q., da Ros, K., and Santos, W. M. (2022). Nitrogen and phosphorus availability in the rhizosphere of maize plants cultivated in biochar amended soil. Archives Agron. Soil Sci. 68 (8), 1062–1074. doi:10.1080/03650340.2020.1869215
Grzesiak, S., Grzesiak, M. T., Hura, T., Marcińska, I., and Rzepka, A. (2013). Changes in root system structure, leaf water potential and gas exchange of maize and triticale seedlings affected by soil compaction. Environ. Exp. Bot. 88, 2–10. doi:10.1016/j.envexpbot.2012.01.010
Gu, P. X., Zhang, Y. M., Xie, H. H., Wei, J., Zhang, X. Y., Huang, X., et al. (2020). Effect of cornstalk biochar on phytoremediation of Cd-contaminated soil by Beta vulgaris var. cicla L. Ecotoxicol. Environ. Saf. 205, 111144. doi:10.1016/j.ecoenv.2020.111144
Gul, S., and Whalen, J. K. (2016). Biochemical cycling of nitrogen and phosphorus in biochar-amended soils. Soil Biol. Biochem. 103, 1–15. doi:10.1016/j.soilbio.2016.08.001
Hailegnaw, N. S., Mercl, F., Pračke, K., Száková, J., and Tlustoš, P. (2019). Mutual relationships of biochar and soil pH, CEC, and exchangeable base cations in a model laboratory experiment. J. Soils Sediments 19 (5), 2405–2416. doi:10.1007/s11368-019-02264-z
Heikkinen, J., Keskinen, R., Soinne, H., Hyväluoma, J., Nikama, J., Wikberg, H., et al. (2019). Possibilities to improve soil aggregate stability using biochars derived from various biomasses through slow pyrolysis, hydrothermal carbonization, or torrefaction. Geoderma 344, 40–49. doi:10.1016/j.geoderma.2019.02.028
Herawati, A., MujiyoSyamsiyah, J., Baldan, S. K., and Arifin, I. (2021). Application of soil amendments as a strategy for water holding capacity in sandy soils. IOP Conf. Ser. Earth Environ. Sci. 724 (1), 012014. doi:10.1088/1755-1315/724/1/012014
Hou, J. B., Pugazhendhi, A., Phuong, T. N., Thanh, N. C., Brindhadevi, K., Velu, G., et al. (2022). Plant resistance to disease: Using biochar to inhibit harmful microbes and absorb nutrients. Environ. Res. 214, 113883. doi:10.1016/j.envres.2022.113883
Hseu, Z. Y., Jien, S. H., Chien, W. H., and Liou, R. C. (2014). Impacts of biochar on physical properties and erosion potential of a mudstone slopeland soil. Sci. World J. 2014, 1–10. doi:10.1155/2014/602197
Hu, F., Xu, C., Ma, R., Tu, K., Yang, J., Zhao, S., et al. (2021). Biochar application driven change in soil internal forces improves aggregate stability: Based on a two-year field study. Geoderma 403, 115276. doi:10.1016/j.geoderma.2021.115276
Hua, B., Li, Z. W., Gao, W. K., Feng, H. L., Chen, N., Li, J. Y., et al. (2021). Soil amendment in plastic greenhouse using modified biochar: Soil bacterial diversity responses and microbial biomass carbon and nitrogen. Biotechnol. Lett. 43 (3), 655–666. doi:10.1007/s10529-020-03046-1
Hua, L., Lu, Z., Ma, H., and Jin, S. (2014). Effect of biochar on carbon dioxide release, organic carbon accumulation, and aggregation of soil. Environ. Prog. Sustain. Energy 33 (3), 941–946. doi:10.1002/ep.11867
Huang, X. R., Li, H., Li, S., Xiong, H. L., and Jiang, X. J. (2016). Role of cationic polarization in humus-increased soil aggregate stability. Eur. J. Soil Sci. 67 (3), 341–350. doi:10.1111/ejss.12342
Ibrahim, E. A., El-Sherbini, M. A. A., and Selim, E.-M. M. (2022). Effects of biochar on soil properties, heavy metal availability and uptake, and growth of summer squash grown in metal-contaminated soil. Sci. Hortic. 301, 111097. doi:10.1016/j.scienta.2022.111097
Irshad, M. K., Chen, C., Noman, A., Ibrahim, M., Adeel, M., and Shang, J. Y. (2020). Goethite-modified biochar restricts the mobility and transfer of cadmium in soil-rice system. Chemosphere 242, 125152. doi:10.1016/j.chemosphere.2019.125152
Islam, M. A., Limon, M. S. H., Romić, M., and Islam, M. A. (2021). Hydrochar-based soil amendments for agriculture: A review of recent progress. - 14(2).
Jafri, N., Wong, W. Y., Doshi, V., Yoon, L. W., and Cheah, K. H. (2018). A review on production and characterization of biochars for application in direct carbon fuel cells. Process Saf. Environ. Prot. 118, 152–166. doi:10.1016/j.psep.2018.06.036
Jain, S., Khare, P., Mishra, D., Shanker, K., Singh, P., Singh, R. P., et al. (2020). Biochar aided aromatic grass cymbopogon martini (roxb.) wats. Vegetation: A sustainable method for stabilization of highly acidic mine waste. J. Hazard. Mater. 390, 121799. doi:10.1016/j.jhazmat.2019.121799
Ji, M., Wang, X., Usman, M., Liu, F., Dan, Y., Zhou, L., et al. (2022). Effects of different feedstocks-based biochar on soil remediation: A review. Environ. Pollut. 294, 118655. doi:10.1016/j.envpol.2021.118655
Jia, Y., Li, J., Zeng, X., Zhang, N., Wen, J., Liu, J., et al. (2022). The performance and mechanism of cadmium availability mitigation by biochars differ among soils with different pH: Hints for the reasonable choice of passivators. J. Environ. Manage. 312, 114903. doi:10.1016/j.jenvman.2022.114903
Kaur, V., and Sharma, P. (2019). Effect of prosopis juliflora biochar on physico-chemical properties of naphthalene and phenanthrene contaminated soil. Polycycl. Aromat. Compd. 41 (7), 1406–1417. doi:10.1080/10406638.2019.1678185
Kowitwiwat, A., and Sampanpanish, P. (2020). Phytostabilization of arsenic and manganese in mine tailings using Pennisetum purpureum cv. Mott supplemented with cow manure and acacia wood-derived biochar. Heliyon 6 (7), e04552. doi:10.1016/j.heliyon.2020.e04552
Lal, R. (2020). Soil organic matter and water retention. Agron. J. 112 (5), 3265–3277. doi:10.1002/agj2.20282
Li, C., Ahmed, W., Li, D., Yu, L., Xu, L., Xu, T., et al. (2022). Biochar suppresses bacterial wilt disease of flue-cured tobacco by improving soil health and functional diversity of rhizosphere microorganisms. Appl. Soil Ecol. 171, 104314. doi:10.1016/j.apsoil.2021.104314
Li, H., Dong, X., da Silva, E. B., de Oliveira, L. M., Chen, Y., and Ma, L. Q. (2017). Mechanisms of metal sorption by biochars: Biochar characteristics and modifications. Chemosphere 178, 466–478. doi:10.1016/j.chemosphere.2017.03.072
Li, L., Zhang, Y.-J., Novak, A., Yang, Y., and Wang, J. (2021). Role of biochar in improving sandy soil water retention and resilience to drought. Water 13 (4), 407. doi:10.3390/w13040407
Li, S., Barreto, V., Li, R., Chen, G., and Hsieh, Y. P. (2018). Nitrogen retention of biochar derived from different feedstocks at variable pyrolysis temperatures. J. Anal. Appl. Pyrolysis 133, 136–146. doi:10.1016/j.jaap.2018.04.010
Li, X. X., Zhang, X., Wang, X. L., and Cui, Z. J. (2019). Phytoremediation of multi-metal contaminated mine tailings with Solanum nigrum L. and biochar/attapulgite amendments. Ecotoxicol. Environ. Saf. 180, 517–525. doi:10.1016/j.ecoenv.2019.05.033
Li, Z. Y., Qi, X. B., Fan, X. Y., Du, Z. J., Hu, C., Zhao, Z. J., et al. (2016). Amending the seedling bed of eggplant with biochar can further immobilize Cd in contaminated soils. Sci. Total Environ. 572, 626–633. doi:10.1016/j.scitotenv.2016.05.020
Liang, C., Gascó, G., Fu, S., Méndez, A., and Paz-Ferreiro, J. (2016). Biochar from pruning residues as a soil amendment: Effects of pyrolysis temperature and particle size. Soil Tillage Res. 164, 3–10. doi:10.1016/j.still.2015.10.002
Lin, H., Jiang, X., Li, B., Dong, Y., and Qian, L. (2021a). Soilless revegetation: An efficient means of improving physicochemical properties and reshaping microbial communities of high-salty gold mine tailings. Ecotoxicol. Environ. Saf. 207, 111246. doi:10.1016/j.ecoenv.2020.111246
Lin, J., Zhang, Q., Xia, H., and Cheng, S. (2021b). Effect of pyrolysis temperature on pyrolysis of pine saw dust and application of bio-char. Int. J. Environ. Sci. Technol. (Tehran). 19 (3), 1977–1984. doi:10.1007/s13762-021-03159-8
Liu, H. Q., Lu, X. B., Li, Z. H., Tian, C. Y., and Song, J. (2021). The role of root-associated microbes in growth stimulation of plants under saline conditions. Land Degrad. Dev. 32 (13), 3471–3486. doi:10.1002/ldr.3955
Liu, H., Wang, X., Song, X., Leng, P., Li, J., Rodrigues, J. L. M., et al. (2022a). Generalists and specialists decomposing labile and aromatic biochar compounds and sequestering carbon in soil. Geoderma 428, 116176. doi:10.1016/j.geoderma.2022.116176
Liu, J., Huang, W., Mo, A. L., Ni, J., Xie, H. Y., Hu, J. S., et al. (2020). Effect of lychee biochar on the remediation of heavy metal-contaminated soil using sunflower: A field experiment. Environ. Res. 188, 109886. doi:10.1016/j.envres.2020.109886
Liu, L. W., Li, W., Song, W. P., and Guo, M. X. (2018). Remediation techniques for heavy metal-contaminated soils: Principles and applicability. Sci. Total Environ. 633, 206–219. doi:10.1016/j.scitotenv.2018.03.161
Liu, M., Zhu, J., Yang, X., Fu, Q., Hu, H., and Huang, Q. (2022b). Biochar produced from the straw of common crops simultaneously stabilizes soil organic matter and heavy metals. Sci. Total Environ. 828, 154494. doi:10.1016/j.scitotenv.2022.154494
Liu, Z. Q., He, T. Y., Cao, T., Yang, T. X., Meng, J., and Chen, W. F. (2017). Effects of biochar application on nitrogen leaching, ammonia volatilization and nitrogen use efficiency in two distinct soils. J. Soil Sci. Plant Nutr. 17 (2), 0–528. doi:10.4067/s0718-95162017005000037
Lu, H. L., Li, K. W., Nkoh, J. N., Shi, Y. X., He, X., Hong, Z. N., et al. (2022). Effects of the increases in soil pH and pH buffering capacity induced by crop residue biochars on available Cd contents in acidic paddy soils. Chemosphere 301, 134674. doi:10.1016/j.chemosphere.2022.134674
Luo, C., Yang, J., Chen, W., and Han, F. (2020). Effect of biochar on soil properties on the Loess Plateau: Results from field experiments. Geoderma 369, 114323. doi:10.1016/j.geoderma.2020.114323
Maboeta, M. S., Oladipo, O. G., and Botha, S. M. (2018). Ecotoxicity of mine tailings: Unrehabilitated versus rehabilitated. Bull. Environ. Contam. Toxicol. 100 (5), 702–707. doi:10.1007/s00128-018-2322-8
Macdonald, S. J., Jordan, G. J., Bailey, T. G., and Davidson, N. (2017). Early seedling establishment on aged Tasmanian tin mine tailings constrained by nutrient deficiency and soil structure, not toxicity. Soil Res. 55 (7), 692–703. doi:10.1071/SR16190
Marcińczyk, M., and Oleszczuk, P. (2022). Biochar and engineered biochar as slow- and controlled-release fertilizers. J. Clean. Prod. 339, 130685. doi:10.1016/j.jclepro.2022.130685
Matin, N. H., Jalali, M., Antoniadis, V., Shaheen, S. M., Wang, J. X., Zhang, T., et al. (2020). Almond and walnut shell-derived biochars affect sorption-desorption, fractionation, and release of phosphorus in two different soils. Chemosphere 241, 124888. doi:10.1016/j.chemosphere.2019.124888
Mattila, T. J., and Rajala, J. (2022). Estimating cation exchange capacity from agronomic soil tests: Comparing Mehlich-3 and ammonium acetate sum of cations. Soil Sci. Soc. Am. J. 86 (1), 47–50. doi:10.1002/saj2.20340
Mikajlo, I., Pourrut, B., Louvel, B., Hynst, J., and Zahora, J. (2022). Plant-soil nitrogen, carbon and phosphorus content after the addition of biochar, bacterial inoculums and nitrogen fertilizer. J. Plant Nutr. 1, 15. doi:10.1080/01904167.2022.2043369
Murtaza, G., Ahmed, Z., and Usman, M. (2022). Feedstock type, pyrolysis temperature and acid modification effects on physiochemical attributes of biochar and soil quality. Arab. J. Geosci. 15 (3), 305. doi:10.1007/s12517-022-09539-9
Narayanan, M., and Ma, Y. (2022). Influences of biochar on bioremediation/phytoremediation potential of metal-contaminated soils. Front. Microbiol. 13, 929730. doi:10.3389/fmicb.2022.929730
Niu, H., Leng, Y. F., Li, X. C., Yu, Q., Wu, H., Gong, J. C., et al. (2021). Behaviors of cadmium in rhizosphere soils and its interaction with microbiome communities in phytoremediation. Chemosphere 269, 128765. doi:10.1016/j.chemosphere.2020.128765
Peng, Y. T., Sun, Y. Q., Fan, B. Q., Zhang, S., Bolan, N. S., Chen, Q., et al. (2021). Fe/Al (hydr)oxides engineered biochar for reducing phosphorus leaching from a fertile calcareous soil. J. Clean. Prod. 279, 123877. doi:10.1016/j.jclepro.2020.123877
Pérez, R., Tapia, Y., Antilén, M., Casanova, M., Vidal, C., Silambarasan, S., et al. (2021). Rhizosphere management for phytoremediation of copper mine tailings. J. Soil Sci. Plant Nutr. 21 (4), 3091–3109. doi:10.1007/s42729-021-00591-0
Phocharoen, Y., Aramrak, S., Chittamart, N., and Wisawapipat, W. (2018). Potassium influence on soil aggregate stability. Commun. Soil Sci. Plant Analysis 49 (17), 2162–2174. doi:10.1080/00103624.2018.1499752
Plaza, C., Giannetta, B., Fernández, J. M., López-de-Sá, E. G., Polo, A., Gascó, G., et al. (2016). Response of different soil organic matter pools to biochar and organic fertilizers. Agric. Ecosyst. Environ. 225, 150–159. doi:10.1016/j.agee.2016.04.014
Poveda, J., Martinez-Gomez, A., Fenoll, C., and Escobar, C. (2021). The use of biochar for plant pathogen control. Phytopathology 111 (9), 1490–1499. doi:10.1094/phyto-06-20-0248-rvw
Praveen, A., and Pandey, V. C. (2020). Pteridophytes in phytoremediation. Environ. Geochem. Health 42 (8), 2399–2411. doi:10.1007/s10653-019-00425-0
Qin, J., Zhao, H., Dai, M., Zhao, P., Chen, X., Liu, H., et al. (2022). Speciation distribution and influencing factors of heavy metals in rhizosphere soil of miscanthus floridulus in the tailing reservoir area of dabaoshan iron polymetallic mine in northern guangdong. Processes 10 (6), 1217. doi:10.3390/pr10061217
Rabbi, S. M. F., Minasny, B., McBratney, A. B., and Young, I. M. (2020). Microbial processing of organic matter drives stability and pore geometry of soil aggregates. Geoderma 360, 114033. doi:10.1016/j.geoderma.2019.114033
Razzaghi, F., Arthur, E., and Moosavi, A. A. (2021). Evaluating models to estimate cation exchange capacity of calcareous soils. Geoderma 400, 115221. doi:10.1016/j.geoderma.2021.115221
Ren, C., Liu, K. S., Dou, P. P., Li, J. H., and Wang, K. (2022). The changes in soil microorganisms and soil chemical properties affect the heterogeneity and stability of soil aggregates before and after grassland conversion. Agriculture 12 (2), 307. doi:10.3390/agriculture12020307
Ren, T., Wang, H., Yuan, Y., Feng, H., Wang, B., Kuang, G., et al. (2021). Biochar increases tobacco yield by promoting root growth based on a three-year field application. Sci. Rep. 11 (1), 21991. doi:10.1038/s41598-021-01426-9
Richardson, A. E., Barea, J.-M., McNeill, A. M., and Prigent-Combaret, C. (2009). Acquisition of phosphorus and nitrogen in the rhizosphere and plant growth promotion by microorganisms. Plant Soil 321 (1-2), 305–339. doi:10.1007/s11104-009-9895-2
Rubin, R. L., Anderson, T. R., and Ballantine, K. A. (2020). Biochar simultaneously reduces nutrient leaching and greenhouse gas emissions in restored wetland soils. Wetlands 40 (6), 1981–1991. doi:10.1007/s13157-020-01380-8
Saedi, A., Jamshidi-Zanjani, A., Darban, A. K., Mohseni, M., and Nejati, H. (2022). Utilization of lead–zinc mine tailings as cement substitutes in concrete construction: Effect of sulfide content. J. Build. Eng. 57, 104865. doi:10.1016/j.jobe.2022.104865
Sameena, P. P., and Puthur, J. T. (2021). Heavy metal phytoremediation by bioenergy plants and associated tolerance mechanisms. Soil Sediment Contam. Int. J. 30 (3), 253–274. doi:10.1080/15320383.2020.1849017
Sathe, P. S., Adivarekar, R. V., and Pandit, A. B. (2021). Valorization of peanut shell biochar for soil amendment. J. Plant Nutr. 45 (4), 503–521. doi:10.1080/01904167.2021.1963771
Shaaban, A., Se, S.-M., Dimin, M. F., Juoi, J. M., Mohd Husin, M. H., and Mitan, N. M. M. (2014). Influence of heating temperature and holding time on biochars derived from rubber wood sawdust via slow pyrolysis. J. Anal. Appl. Pyrolysis 107, 31–39. doi:10.1016/j.jaap.2014.01.021
Shah, V., and Daverey, A. (2020). Phytoremediation: A multidisciplinary approach to clean up heavy metal contaminated soil. Environ. Technol. Innovation 18, 100774. doi:10.1016/j.eti.2020.100774
Shamim, M., Saha, N., and Hye, F. B. (2018). Effect of biochar on seed germination, early growth of Oryza sativa L. and soil nutrients. Trop. Plant Res. 5 (3), 336–342. doi:10.22271/tpr.2018.v5.i3.042
Shen, W., Feng, Z., Song, H., Jin, D., Fu, Y., and Cheng, F. (2022). Effects of solid waste-based soil conditioner and arbuscular mycorrhizal fungi on crop productivity and heavy metal distribution in foxtail millet (Setaria italica). J. Environ. Manage. 313, 114974. doi:10.1016/j.jenvman.2022.114974
Shi, G., Wu, Y., Li, T., Fu, Q., and Wei, Y. (2022). Mid- and long-term effects of biochar on soil improvement and soil erosion control of sloping farmland in a black soil region, China. J. Environ. Manage. 320, 115902. doi:10.1016/j.jenvman.2022.115902
Shi, R. Y., Hong, Z. N., Li, J. Y., Jiang, J., Abdulaha-Al Baquy, M., Xu, R. K., et al. (2017). Mechanisms for increasing the pH buffering capacity of an acidic ultisol by crop residue-derived biochars. J. Agric. Food Chem. 65 (37), 8111–8119. doi:10.1021/acs.jafc.7b02266
Shu, X.-H., Zhang, Q., Lu, G.-N., Yi, X.-Y., and Dang, Z. (2018). Pollution characteristics and assessment of sulfide tailings from the Dabaoshan Mine, China. Int. Biodeterior. Biodegrad. 128, 122–128. doi:10.1016/j.ibiod.2017.01.012
Šimanský, V., Horák, J., Igaz, D., Balashov, E., and Jonczak, J. (2017). Biochar and biochar with N fertilizer as a potential tool for improving soil sorption of nutrients. J. Soils Sediments 18 (4), 1432–1440. doi:10.1007/s11368-017-1886-y
Simansky, V., Horak, J., Igaz, D., Jonczak, J., Markiewicz, M., Felber, R., et al. (2016). How dose of biochar and biochar with nitrogen can improve the parameters of soil organic matter and soil structure? Biologia 71 (9), 989–995. doi:10.1515/biolog-2016-0122
Šimanský, V., Juriga, M., Jonczak, J., Uzarowicz, Ł., and Stępień, W. (2019). How relationships between soil organic matter parameters and soil structure characteristics are affected by the long-term fertilization of a sandy soil. Geoderma 342, 75–84. doi:10.1016/j.geoderma.2019.02.020
Sui, L., Tang, C., Cheng, K., and Yang, F. (2022). Biochar addition regulates soil phosphorus fractions and improves release of available phosphorus under freezing-thawing cycles. Sci. Total Environ. 848, 157748. doi:10.1016/j.scitotenv.2022.157748
Sun, W., Ji, B., Khoso, S. A., Tang, H., Liu, R., Wang, L., et al. (2018). An extensive review on restoration technologies for mining tailings. Environ. Sci. Pollut. Res. 25 (34), 33911–33925. doi:10.1007/s11356-018-3423-y
Tian, X. Q., Li, Z., Wang, L. C., Wang, Y. F., Li, B., Duan, M. C., et al. (2020). Effects of biochar combined with nitrogen fertilizer reduction on rapeseed yield and soil aggregate stability in upland of purple soils. Int. J. Environ. Res. Public Health 17 (1), 279. doi:10.3390/ijerph17010279
Tomczyk, A., Sokołowska, Z., and Boguta, P. (2020). Biochar physicochemical properties: Pyrolysis temperature and feedstock kind effects. Rev. Environ. Sci. Biotechnol. 19 (1), 191–215. doi:10.1007/s11157-020-09523-3
Wang, K., Zhang, X., Sun, C., Yang, K., Zheng, J., and Zhou, J. (2020). Biochar application alters soil structure but not soil hydraulic conductivity of an expansive clayey soil under field conditions. J. Soils Sediments 21 (1), 73–82. doi:10.1007/s11368-020-02786-x
Wang, L., Ji, B., Hu, Y., Liu, R., and Sun, W. (2017). A review on in situ phytoremediation of mine tailings. Chemosphere 184, 594–600. doi:10.1016/j.chemosphere.2017.06.025
Wang, M., He, D., Shen, F., Huang, J., Zhang, R., Liu, W., et al. (2019). Effects of soil compaction on plant growth, nutrient absorption, and root respiration in soybean seedlings. Environ. Sci. Pollut. Res. 26 (22), 22835–22845. doi:10.1007/s11356-019-05606-z
Xiu, L. Q., Zhang, W. M., Sun, Y. Y., Wu, D., Meng, J., and Chen, W. F. (2019). Effects of biochar and straw returning on the key cultivation limitations of Albic soil and soybean growth over 2 years. Catena 173, 481–493. doi:10.1016/j.catena.2018.10.041
Xu, N., Tan, G. C., Wang, H. Y., and Gai, X. P. (2016). Effect of biochar additions to soil on nitrogen leaching, microbial biomass and bacterial community structure. Eur. J. Soil Biol. 74, 1–8. doi:10.1016/j.ejsobi.2016.02.004
Xu, S., Chen, J., Peng, H., Leng, S., Li, H., Qu, W., et al. (2021). Effect of biomass type and pyrolysis temperature on nitrogen in biochar, and the comparison with hydrochar. Fuel 291, 120128. doi:10.1016/j.fuel.2021.120128
Xu, X., Wu, Y., Wu, X., Sun, Y., Huang, Z., Li, H., et al. (2022). Effect of physicochemical properties of biochar from different feedstock on remediation of heavy metal contaminated soil in mining area. Surfaces Interfaces 32, 102058. doi:10.1016/j.surfin.2022.102058
Xu, Z. B., Xu, X. Y., Zhang, Y., Yu, Y. L., and Cao, X. D. (2020). Pyrolysis-temperature depended electron donating and mediating mechanisms of biochar for Cr(VI) reduction. J. Hazard. Mater. 388, 121794. doi:10.1016/j.jhazmat.2019.121794
Yang, C., Liu, J., Ying, H., and Lu, S. (2022). Soil pore structure changes induced by biochar affect microbial diversity and community structure in an Ultisol. Soil Tillage Res. 224, 105505. doi:10.1016/j.still.2022.105505
Yang, C., and Lu, S. (2021). Pyrolysis temperature affects phosphorus availability of rice straw and canola stalk biochars and biochar-amended soils. J. Soils Sediments 21 (8), 2817–2830. doi:10.1007/s11368-021-02993-0
Yang, L., Wu, Y. C., Wang, Y. C., An, W. Q., Jin, J., Sun, K., et al. (2021b). Effects of biochar addition on the abundance, speciation, availability, and leaching loss of soil phosphorus. Sci. Total Environ. 758, 143657. doi:10.1016/j.scitotenv.2020.143657
Yang, L., Wu, Y., Wang, Y., An, W., Jin, J., Sun, K., et al. (2021a). Effects of biochar addition on the abundance, speciation, availability, and leaching loss of soil phosphorus. Sci. Total Environ. 758, 143657. doi:10.1016/j.scitotenv.2020.143657
Yang, T., Xu, Y., Huang, Q., Sun, Y., Liang, X., Wang, L., et al. (2021c). An efficient biochar synthesized by iron-zinc modified corn straw for simultaneously immobilization Cd in acidic and alkaline soils. Environ. Pollut. 291, 118129. doi:10.1016/j.envpol.2021.118129
Yang, Y., Liu, Y., Li, Z., Wang, Z., Li, C., and Wei, H. (2020). Significance of soil microbe in microbial-assisted phytoremediation: An effective way to enhance phytoremediation of contaminated soil. Int. J. Environ. Sci. Technol. (Tehran). 17 (4), 2477–2484. doi:10.1007/s13762-020-02668-2
Yin, F., Li, Y. J., Zhao, Q., Li, C., Li, J., and Tian, S. L. (2021). Experimental and model predictive investigation into the relationship of pH alternation with addition of lime in acid tin tailing treatments. Bull. Environ. Contam. Toxicol. 107 (6), 1202–1207. doi:10.1007/s00128-021-03299-0
Zalesny, R. S., Casler, M. D., Hallett, R. A., Lin, C.-H., and Pilipović, A. (2021). “Bioremediation and soils,” in Soils and landscape restoration, 237–273.
Zhang, L., Jing, Y., Chen, G., Wang, X., and Zhang, R. (2019a). Improvement of physical and hydraulic properties of desert soil with amendment of different biochars. J. Soils Sediments 19 (7), 2984–2996. doi:10.1007/s11368-019-02293-8
Zhang, M. Y., Wang, J., Bai, S. H., Zhang, Y. L., Teng, Y., and Xu, Z. H. (2019b). Assisted phytoremediation of a co-contaminated soil with biochar amendment: Contaminant removals and bacterial community properties. Geoderma 348, 115–123. doi:10.1016/j.geoderma.2019.04.031
Zhang, X., Yu, J., Huang, Z., Li, H., Liu, X., Huang, J., et al. (2021). Enhanced Cd phytostabilization and rhizosphere bacterial diversity of Robinia pseudoacacia L. by endophyte Enterobacter sp. YG-14 combined with sludge biochar. Sci. Total Environ. 787, 147660. doi:10.1016/j.scitotenv.2021.147660
Zhao, B., Peng, T., Hou, R., Huang, Y., Zong, W., Jin, Y., et al. (2022). Manganese stabilization in mine tailings by MgO-loaded rice husk biochar: Performance and mechanisms. Chemosphere 308, 136292. doi:10.1016/j.chemosphere.2022.136292
Zhao, S.-X., Ta, N., and Wang, X.-D. (2017). Effect of temperature on the structural and physicochemical properties of biochar with apple tree branches as feedstock material. Energies 10 (9), 1293. doi:10.3390/en10091293
Zhao, X., and Hu, M. (2021). Capillary forces between particles: Role of biochar in improving water retention capacity of soil. Arab. J. Geosci. 14 (17), 1769. doi:10.1007/s12517-021-08211-y
Keywords: biochar, phytoremediation, heavy metal, mine tailings, soil
Citation: Shi Y, Zang Y, Yang H, Zhang X, Shi J, Zhang J and Liu B (2022) Biochar enhanced phytostabilization of heavy metal contaminated mine tailings: A review. Front. Environ. Sci. 10:1044921. doi: 10.3389/fenvs.2022.1044921
Received: 15 September 2022; Accepted: 20 October 2022;
Published: 02 November 2022.
Edited by:
Liu Zhirong, East China University of Technology, ChinaReviewed by:
Valeria Ancona, Department of Earth System Sciences and Technologies for the Environment (CNR), ItalyTao Yu, East China University of Technology, China
Copyright © 2022 Shi, Zang, Yang, Zhang, Shi, Zhang and Liu. This is an open-access article distributed under the terms of the Creative Commons Attribution License (CC BY). The use, distribution or reproduction in other forums is permitted, provided the original author(s) and the copyright owner(s) are credited and that the original publication in this journal is cited, in accordance with accepted academic practice. No use, distribution or reproduction is permitted which does not comply with these terms.
*Correspondence: Xu Zhang, 15668303582@163.com; Bing Liu, b-liu@sdjzu.edu.cn
†These authors have contributed equally to this work