- 1School of Agriculture, Ningxia University, Yinchuan, China
- 2Breeding Base for State Key Laboratory of Land Degradation and Ecological Restoration in Northwest China, Ningxia University, Yinchuan, China
- 3Ministry of Education Key Laboratory for Restoration and Reconstruction of Degraded Ecosystem in Northwest China, Ningxia University, Yinchuan, China
- 4CAS Engineering Laboratory for Yellow River Delta Modern Agriculture, Institute of Geographic Sciences and Natural Resources Research, Chinese Academy of Sciences, Beijing, China
Soil extracellular ecoenzymatic activities (EEA) are major players in the biogeochemical cycles and are closely related to the metabolic demand and nutrient supply in microbes. However, their effects on biogeochemistry along the elevation gradient on mountain ecosystems in arid regions remain unclear. To address this, we investigated the variations of soil microbial resource limitation and the relative contributing factors along the elevation gradient of the Helan Mountains, northwest China. The results showed that the relative abundance of total microbial, bacterial, fungal, actinomycetes, and N-acquiring enzymatic activities (N-Acetyl-β-D-glucosaminidase and Leucine- α-aminopeptidase, that are NAG and LAP, respectively) in the soil first increased and then decreased with an increase in elevation. This variation pattern could be due to the changes in soil temperature and moisture along the elevation gradient. Soil enzyme stoichiometry and resource allocation further revealed that the microbial metabolism activity in the Helan Mountains was limited by carbon (C) and phosphorus (P). Furthermore, the two limited elements were significantly higher at the mid and high altitudes (2,139–2,438 m) than at low altitudes (1,380–1,650 m). Additionally, redundancy analysis revealed that the soil water content and bulk density played a crucial role in microbial community structures, while the soil pH had the most influence on soil EEA and ecoenzymatic stoichiometry. Our findings revealed the patterns of soil microbial community structure, extracellular enzyme activities, and microbial metabolism at various elevations, which will help in understanding the microbial resource limitation and nutrient cycling in mountain ecosystems in arid regions.
Introduction
Soil microbes play a significant role in soil elements cycling, affecting soil development and function (Li et al., 2015; Palomo et al., 2016). The differences in resource demand, competition, and adaptation strategies among microbial species influence the microbial community structure and function (Mooshammer et al., 2014a; Chen et al., 2018). For example, bacteria and fungi have different growth strategies, nutrient demands, and substrate utilization capacities (Clemmensen et al., 2013; Riggs and Hobbie, 2016). The microbial communities acquire carbon and nutrients from the soil by secreting ecoenzymes, which decompose the soil macromolecular substances, promoting material circulation and energy flow, especially when resources are limited (Stark et al., 2014). However, research on soil nutrients and microbial properties, especially the microbial metabolic limitations (enzymatic stoichiometry) is limited (Cui et al., 2019b; Chang et al., 2019). Hence, it is urgent to explore the microbial community composition and their metabolic constraints for better understanding of long-term protection conditions and to offer scientific guidance for practical management of mountain ecosystems.
Extracellular ecoenzymatic activities (EEA) are major players in the biogeochemical cycles, which are closely related to the metabolic demand and nutrient supply in microbes (Jones et al., 2009; Dong et al., 2019). Ecoenzymatic stoichiometry (EES) is a reliable indicator of microbial resources limitations and denotes the nutrient demand and supply in soil microorganisms, revealing the microbial nutritional status and resource constraints, (Hill et al., 2014; Zhang et al., 2019a). Both EEA and EES have been extensively applied in various ecosystems and scales to indicate the limitations of microbial resources. (Rosinger et al., 2019; Xiao et al., 2020). For example, previous studies on soil metabolic activity found that Chinese forest soil microbial metabolism is mainly limited by P (Cui et al., 2022). Furthermore, Wu et al. (2021a) revealed that an increase in secondary succession processes increases P limitation but decreases N limitation on soil microbial metabolism. These nutrient limitation shifts are caused by changes in vegetation and environmental factors. Similarly, in mountain ecosystems, vegetation and environmental factors change dramatically with elevation, and it has been shown that extracellular enzyme activity and their ratios vary along elevation gradient (Zuo et al., 2018). However, our current understanding of microbial metabolism pattern along elevation gradient is limited due to few studies and inconsistent results on the variation of EEA and EES across elevation gradient. Therefore, it is necessary to identify the spatial patterns of soil EEA and EES, and elucidate the microbial resource limitations in mountain ecosystems.
Extracellular ecoenzymatic activities and EES are largely affected by temperature, precipitation, soil physicochemical parameters, and soil microbial properties (Kivlin and Treseder, 2014; Zhang et al., 2019b; Xiao et al., 2020; Zheng et al., 2020; Zhu et al., 2020). The spatial pattern of EEA and EES across a wide climatic gradient can be affected by various factors. Studies on latitudinal gradient have shown that EES in temperate grasslands of northern China is dependent on soil nutrient stoichiometry (Peng and Wang 2016), whereas in subtropical forest was controlled by climatic factors (Jian et al., 2021). Moreover, several studies on elevation gradient have found that EES in Tibetan Plateau is largely affected by vegetation type (Cao et al., 2022), while microbial metabolism in the Qinghai-Tibetan Plateau is mainly influenced by pH and available nutrients (He et al., 2020). As determined in previous studies, EEA and EES and their driving factors vary across climate zones, especially in elevation gradient. However, few studies have explored the effects of biotic and abiotic factors on microbial metabolism along elevational gradient in arid climate zone.
The Helan Mountains is the highest mountain to the east of Alxa Plateau, blocking the Siberian cold current while protecting the Yinchuan Plain. The vegetation type, soil physicochemical properties, aggregate stability, and soil organic carbon chemical components vary drastically along the elevation gradients of Helan Mountains (Zeng et al., 2020; Wu et al., 2021b). The Helan Mountains serves as a National Nature Reserve, providing a natural laboratory for studying the biogeochemical cycle in an arid mountainous ecosystem. In this study, we explored the microbial resource limitations, microbial metabolic processes, and reveal their underlying mechanisms along elevation gradients. Specifically, we selected five different vegetation types: steppe desert, Prunus mongolica shrubs, Chinese pine forest, mixed forest (coniferous and broad-leaved), and Qinghai spruce forest to represent the different elevation gradients. This study aimed to 1) reveal the trends of microbial resource limitation and metabolism processes along the elevation gradient; and 2) examine how environmental variables affect microbial resource limitations and metabolism processes in soil along the elevation gradient. We hypothesize that: 1) the microbial resource limitation represented by soil enzyme stoichiometry would vary in the different altitudinal gradient, 2) the C limitations could be higher at high altitudes than at low altitude, which mainly attribute to changes in soil temperature and moisture along the altitudinal gradient. Specifically, we expect that this study will highlight the microbial metabolic patterns, the relationship between the microbial metabolic restriction and potential drivers along the altitude gradient of Helan Mountains.
Materials and methods
Study area description and sampling
This study was conducted in July 2020 at the Helan Mountains located at the border of Ningxia Hui and the Inner Mongolia Autonomous Region, northwest China (38°13′–39°30′N, 105°41′–106°41′E). The mountain’s highest peak is 3,556 m. The region is a typical continental climate with a mean annual temperature, precipitation, and evaporation of −0.8°C, 420 mm, and 2000 mm, respectively, and 80% of precipitation occurs between May–September (Jiang et al., 2019; Zeng et al., 2020). Besides, the vegetation types vary from desert steppe to boreal coniferous forest with increasing elevation (Wu et al., 2021b).
Soil samples were collected from five elevation points: 1,380, 1,650, 2,139, 2,249, and 2,438 m. The dominant plant species at these elevation points were Stipa capillata (1,380 m), Prunus mongolica Maxim (1,650 m), Pinus tabuliformis (2,139 m), Pinus tabuliformis and Populus davidiana (2,249 m), and Picea crassifolia (2,438 m). Six plots were randomly established at each elevation point, measuring 1 m × 1 m in steppe desert, 10 m × 10 m in shrubs, and 20 m × 20 m in forests. Tree, shrubs, and herbs were measured in each quadrat and the species were identified (Zhang and Dong, 2010). The litter layer was removed from each plot, and five soil samples were collected per plot at 0–20 cm of the soil layer using soil auger. Soil samples were combined per plot and thoroughly mixed to create a composite sample and sifted using a 2 mm sieve to remove rocks, litters, and other substances, and then divided into three subsamples. One subsample was stored at 4°C for the analyses of extracellular enzyme activity, another was air-dried to determine the soil physicochemical parameters, and the last one was stored at −80°C for analyzing the microbial communities.
Soil physiochemical analysis
The soil samples were air-dried for physicochemical analyses of the soil organic carbon (SOC), total nitrogen (TN), total phosphorus (TP), and soil pH. The soil pH was measured in a soil: water ratio of 1:2.5 using a pH meter (Zornoza et al., 2007). Soil texture was determined using a laser particle size analyzer (Master-sizer 2,000, Malvern, United Kingdom). The soil bulk density (BD) (g cm−3) was measured using a soil bulk sampler with a stainless steel cutting ring at points adjacent to the soil sampling quadrats (Deng et al., 2019). The SOC and TN concentrations were determined using the Walkley-Black and Kjeldahl methods (Zornoza et al., 2007). The TP was measured by the colorimetric method after wet digestions with H2SO4 and HClO4, as previously described by Wang et al. (2014). Soil available nitrogen (AN) and available phosphorus (AP) were analyzed following the international standard methods as adopted and published by the Institute of Soil Science, Chinese Academy of Sciences (1978).
Phospholipid fatty acids analysis
The phospholipid fatty acid (PLFA) was analyzed to assess the microbial communities in soil samples as described by Frostegård et al. (1991). The lipids were extracted from 5 g of freeze-dried soil sample using a buffer containing chloroform, methanol, and citrate acid (1:2:0.8). Next, the fatty acids in the lipid extracts were converted into free methyl esters by alkaline methanolysis and analyzed by gas chromatography (GC; Hewlett-Packard 5,890). The obtained PLFAs were assigned into eight microbial taxonomic groups according to the published biomarker data (Frostegård and Bååth, 1996; Guo et al., 2016; Spaans et al., 2019). These groups included e nonspecific bacteria (12:0, 14:0, 15:0, 16:0, 17:0, 18:0, 19:0, 20:0, and 24:0), Gram-positive bacteria (a12:0, i13:0, a13:0, i14:0, a14:0, i15:0, a15:0, i16:0, a16:0, i17:0, a17:0, i17: 1ω7c), Gram-negative bacteria (16:1ω7c DMA, 17:1ω8c, 17:0cycloω7c, 19:0cycloω7c, 22: 1ω9c), fungus (18:2ω6c), actinomycetes (10Me16:0,10Me17:0, 10Me18: 1ω7c, 10Me17: 1ω7c), and protozoan (20: 4ω6c, 20: 5ω3c). Since PLFA 18:1ω9c is an indicator of Gram-negative bacteria, and PLFA 16:1ω5c of arbuscular mycorrhizal fungi (AMF) (Orwin et al., 2014; Helfrich et al., 2015), the bacteria and fungi served as independent indicators to characterize the number of soil microorganisms. Finally, the relative abundance of PLFAs in the soil samples was used to calculate the microbial community composition using the formula below (Guo et al., 2016):
where Ri is the relative abundance of PLFAs in one of the eight groups (%),
Extracellular enzyme analysis
The β-glucosidase, cellobiosidase, β-1,4,-N-acetyl-glucosaminidase, leucine aminopeptidase, and alkaline phosphatase (BG, CBH, NAG, LAP, AP, respectively) represent the C-, N- and P- acquisition enzymes were measured using a 96-well microplate fluorometer. Briefly, 1.5 g of fresh soil was added into 150 ml of 50 M sodium acetate buffer to create soil homogenates. Subsequently, 200 μl of the soil homogenates and 50 μl of 200 μM enzyme-substrate were added into the 96-well plates and incubated in the dark at 25°C for 2.5 h. After incubation, the enzyme activities were determined using a microplate fluorometer reader (SynergyH1M, Biotek, United States ) (Saiya-Cork et al., 2002; Moorhead et al., 2016).
Statistical analysis
The plant diversity of the five different vegetation types in the study area was calculated as described by Zhang and Dong. (2010). Soil C, N, and P stoichiometric ratios were calculated on a mass basis of C, N, and P (Pang et al., 2018a). According to this theory, the relative investments of C vs. nutrient acquisition or P vs. N acquisition represent the ratio of soil enzymatic C: N vs. C: P acquisition as a point in Cartesian coordinate system (Moorhead et al., 2016). Thus, the ratios of C: NEEA, C: PEEA, and N: PEEA acquisition enzymes were determined as (BG + CBH): (NAG + LAP), (BG + CBH): AP, and (NAG + LAP): AP, respectively (Zhao et al., 2020). The microbial metabolic limitation was calculated as follows (Chen et al., 2019a):
The vector length (L) denotes the microbial C restriction, while the vector angle (A) denotes the microbial N or P restriction. The higher the vector length, the higher the C restriction. A vector angle >45° indicates P restriction, while <45° indicates N restriction; thus, as the vector angle increases, the P restriction increases while the N restriction decreases (Yu et al., 2022).
Homogeneity of the variance test was performed before executing analysis of variance (ANOVA). The soil physicochemical parameters, microbial community components, and enzyme activities at different altitudes were determined using a one-way analysis of variance (ANOVA), and using the least significant difference (LSD) test. All data analysis was conducted in SPSS 24.0 (SPSS Inc., IL, United States ). Changes in soil C-, N- and P- acquisition enzymes activities and stoichiometry due to elevation was determined by regression analysis, and the relationship between the two parameters was elucidated using the standardized major axis (SMA) estimation method. The distribution and differences in soil microbial community and enzyme activities along the altitudes were determined by a non-metric multidimensional scaling ordination (NMDS). The SMA and NMDS were conducted using the “smatr” and “vegan” packages, respectively, in R software (version 3.6.2). The relationship between soil physicochemical parameters, microbial properties, and enzyme activities was determined by Redundancy analysis (RDA). Pearson correlation analysis was performed to identify the relationships between microorganism communities, enzyme activities and soil physicochemical properties, and statistical analyses were performed in the R (3.6.2 version), using “corrplot” packages. Finally, the Monte Carlo permutation test was used to explore and sort factors that significantly influenced microbial resource limitation. The RDA and Monte Carlo permutation tests were conducted using Canoco software (5.0) for Windows.
Results
Soil physicochemical characteristics and nutrient stoichiometry
The soil physicochemical characteristics at the different elevation gradients are presented in Table 1. The pH and BD decreased with an increase in elevation. Specifically, the pH was significantly higher at 1,380 m and 1,650 m than at 2,139, 2,249, and 2,438 m. The BD was significantly higher at 1,380 m than at 2,438 m, while there were no significant differences in BD at 1,650, 2,139, and 2,249 m (p > 0.05). Contrarily, SWC, SOC, and TN increased with elevation, and were significantly higher at high-elevations (2,249 and 2,438 m) than the low-elevations (1,380 and 1,650 m). However, the content of NO3−-N, NH4+-N and AP first increased with elevation, reaching the highest level at 2,139 m, and then decreased. Similarly, the soil C:N and C:P ratios initially increased and then decreased with an increase in elevation. The highest C:N and C:P ratios were obtained at the mid-elevation of 2,139 m.
Soil microbial community components and structures
There were no significant variances in soil microbial community components and structures between different elevations (Figure 1). The total PLFAs, bacterial, fungal, actinomycetes, F: B, and protozoa content initially increased and then decreased with an increase in elevation. Besides, GN and GP: GN were highest at 2,249 m. The trend of total microbial PLFAs across the different altitudes was: 2,139 m > 2,249 m > 1,650 m > 1,380 m > 2,438 m (Figure 1A). Based on the NMDS plot, there were no significant variances in the microbial community structure across the different elevations (Supplementary Figure S1A; p > 0.05).
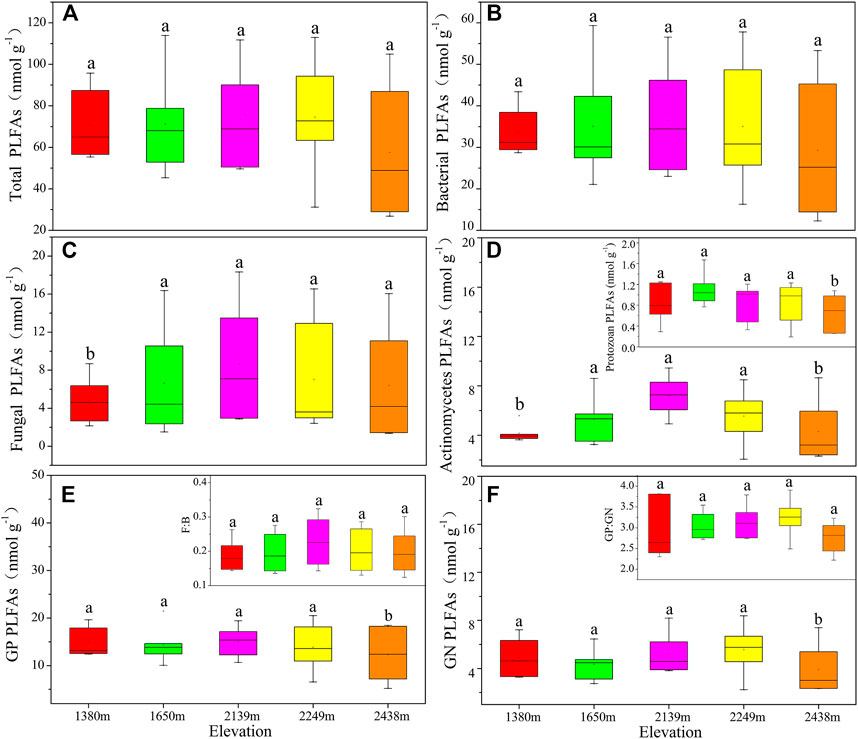
FIGURE 1. The soil community components based on PLFA profiling at different altitude gradients. Different small letters indicate significant difference (p < 0.05) among different altitudes (n = 6). The same below. F:B: the ratio of fungi to bacteria; GP: gram-positive bacterial; GN: gram-negative bacterial; GP: GN: gram-positive bacteria to Gram-negative bacteria ratio. Total PLFAs (A), bacterial PLFAs (B), fungal PLFAs (C), actinomycetes and protozoa PLFAs (D), GP PLFAs and F:B (E), GN PLFAs and GP:GN (F).
The relative abundance of the soil microbial community varied significantly across different altitudes (p < 0.05; Supplementary Figure S2). The highest relative abundance of nonspecific bacteria was observed at 1,650 m, while fungi (18:2ω 6c) were highest at 2,139 m (Supplementary Figure S2A). Actinomycetes and protozoa exhibited no significant variations in abundance at the different altitudes (p > 0.05); however, the abundances of actinomycetes at 1,380 m and protozoa at 1,650 m were higher than in the other altitudes (Supplementary Figure S2B). Similarly, 18:1w9c did not vary significantly across the different altitudes (p > 0.05). The 16:1w5c values were significantly lower at 2,438 m than in the other altitudes (Supplementary Figure S2C). Moreover, the abundance of GP was highest at 2,438 m, but GN had no significant differences across the different altitudes (p > 0.05; Supplementary Figure S2D).
Soil enzyme activities and stoichiometry
The activities of the five selected enzymes were significantly different at the different altitudes (p < 0.05; Supplementary Figure S1B). Specifically, CBH and AKP activities increased drastically with elevation, reaching a maximum value at 2,438 m, while BG, NAG, and LAP had the greatest values at 2,139 m, 2,249 m, and 1,650 m, respectively (Figure 2). Our findings also indicated that C: NEEA, C: PEEA, and N: PEEA were significantly different at the various elevations (p < 0.05; Figure 3). Specifically, C: NEEA increased from 1,380 m to 2,438 m (Figure 3A), while the C: PEEA and N: PEEA values were highest at 2,249 m and 1,650 m, respectively (Figures 3B,C).
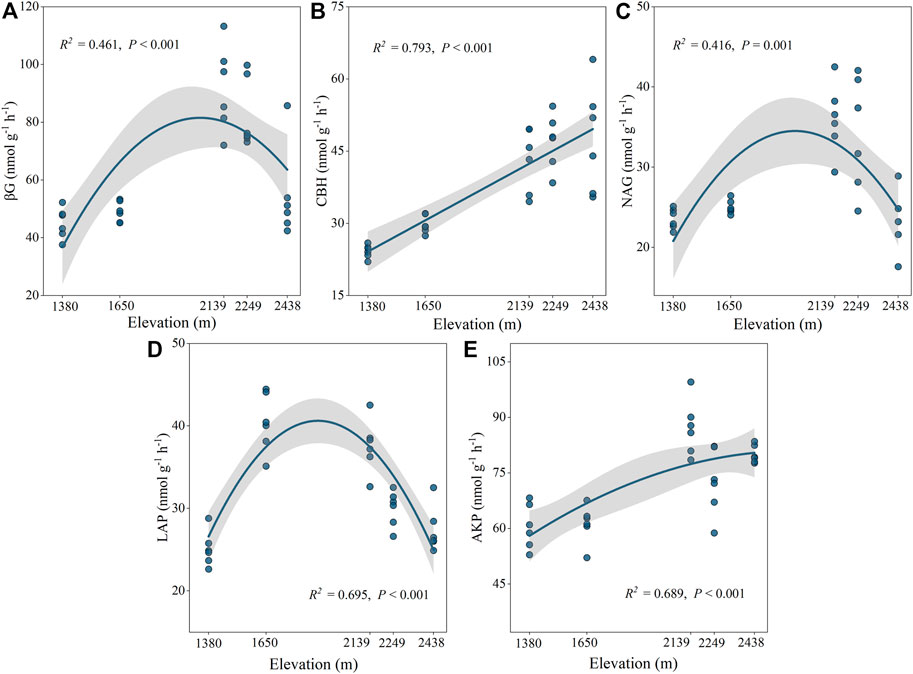
FIGURE 2. Ecoenzymatic activities at different altitudes. BG: β-1,4-glucosidase (A), CBH: Cellobiohydrolase (B), NAG: β-1,4-N-acetylglucosaminidase (C), LAP: leucine aminopeptidase (D), AKP: alkaline phosphatase (E).
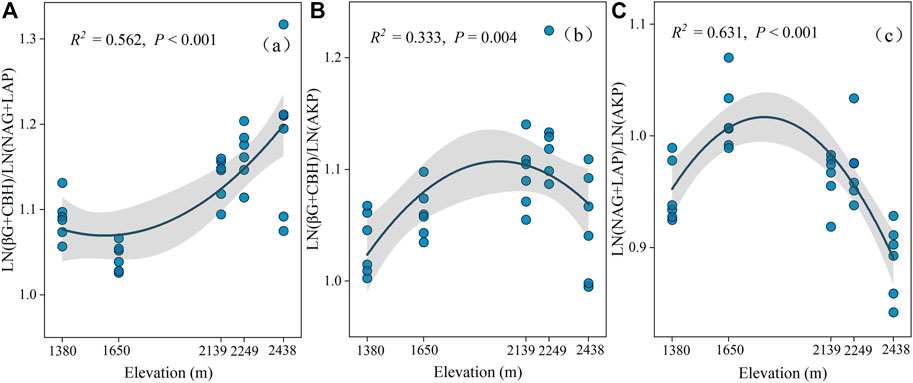
FIGURE 3. The ecoenzymatic stoichiometry of Ln (BG + CBH): Ln (NAG + LAP) (A), Ln (BG + CBH): Ln (AKP) (B), Ln (NAG + LAP): Ln (AKP) (C) at different altitudes.
The five enzyme activities were significantly positively correlated, and the regression slopes of C: NEEA, C: PEEA, and N: PEEA activities were 1.88, 1.67, and 1.07, respectively (Figure 4). The (BG + CBH) enzymes had higher investments than the (NAG + LAP) and AKP enzymes (Figures 4A,B), while microbes were inclined to higher investment in AKP enzyme than in the (NAG + LAP) enzyme (Figure 4C).
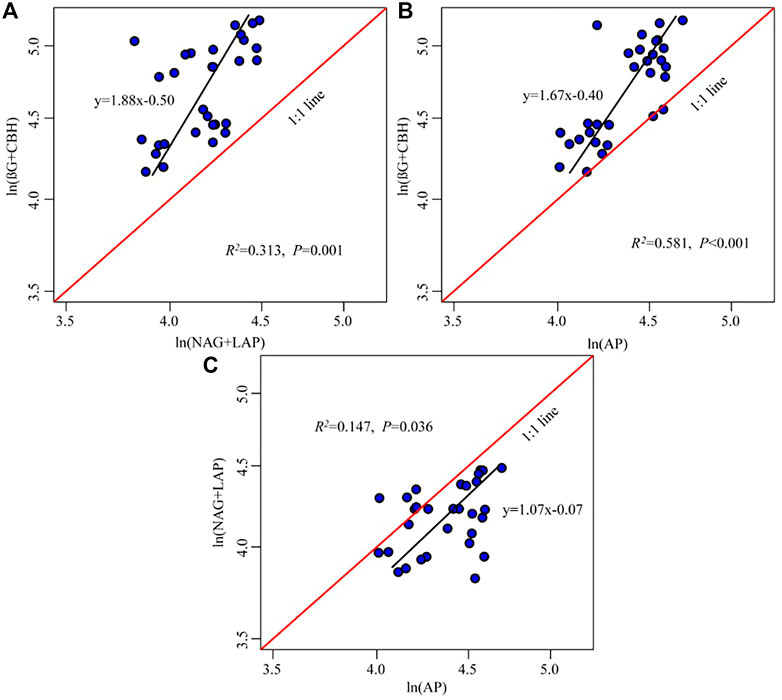
FIGURE 4. Regressions analyses of ln (BG + CBH): ln (NAG + LAP) (A), ln (BG + CBH): ln (AKP) (B), and ln (NAG + LAP): ln (AKP) (C). The black line is the regression line, while the red line is the reference line with slope = 1. The black regression line to the left from the 1:1 line suggests more resources devoted to the enzyme on the y-axis compared with the x-axis [e.g., ln (BG + CBH) compared to ln (NAG + LAP)]. The regression line to the right from the 1:1 line suggests more resources devoted to the enzyme on the x-axis compared with the y-axis.
Microbial C restriction was highest at 2,249 m (Figure 5A). Additionally, the vector angles were >45°, implying that the microbial metabolisms were limited by soil P (Figure 5B). The microbial P restriction was highest and lowest at 2,438 m and 2,249 m, respectively. Moreover, the C and P restrictions were higher at high altitudes (2,139–2,438 m) than at low altitudes (1,380–1,650 m). Overall, the microbial C restriction and N/P restriction showed a negative correlation (p < 0.05; Figure 5C).
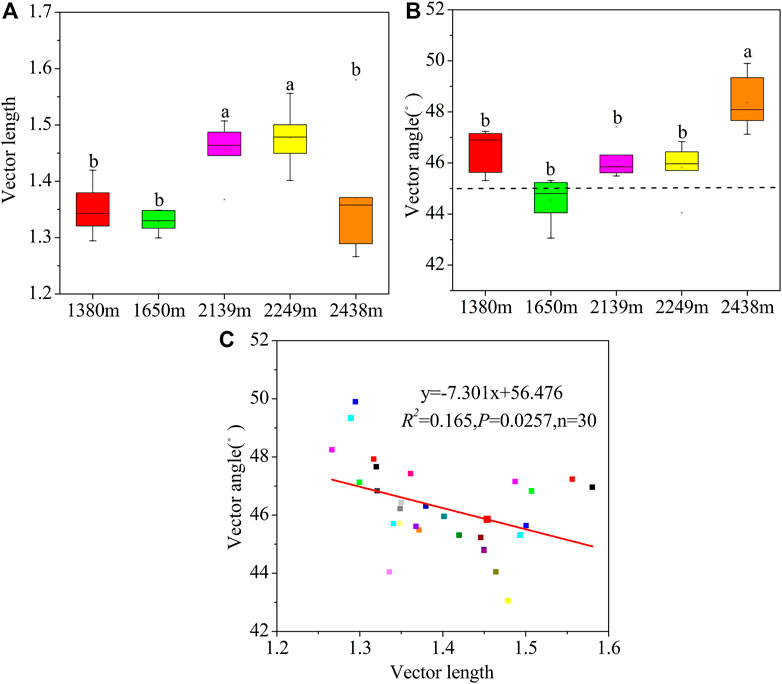
FIGURE 5. Variations in vector length (A), angles (B), and the relationship between vector length and angles (C) at the different altitudes. Vector length is represented by soil microbial C limitation, and microbial C limitation increases as the values increase. Vector angles are represented by soil microbial N/P limitation, angles >45° represent P limitation, and angles <45° represent N limitation.
Soil nutrient, microbes, and enzymes interactions
Soil nutrient, microbes, and enzyme properties were closely related (Supplementary Figure S3). Specifically, the soil BD, pH and AP had a significant negative correlation with SOC, TN, C:P, N:P, and CBH (p < 0.05). Conversely, the SOC and TN positively correlated with the soil C:P, N:P, CBH, and AKP (p < 0.05). Soil C:N, C:P, and N:P also positively correlated with BG, CBH, NAG, and AKP (Supplementary Figure S3). Similarly, the total microbial, bacterial, fungal, actinomycetes, protozoa, GP, and GN were positively correlated. The BG and CBH positively correlated with AKP, C:NEEA, C:PEEA, and vector length, and C:NEEA positively correlated with C:PEEA, vector length, and vector angle (p < 0.05, Supplementary Figure S3).
The first two axes accounted for 81.97% of the soil physicochemical and microbial properties (Figure 6A). Specifically, sand and TP were strongly negatively correlated with most microbial properties (p < 0.05), while clay, silt, SOC, soil nutrients, and stoichiometry correlated positively with most microbial properties (p < 0.05). Based on the Monte Carlo test, SWC and BD were the most influential variables on microbial properties (Table 3).
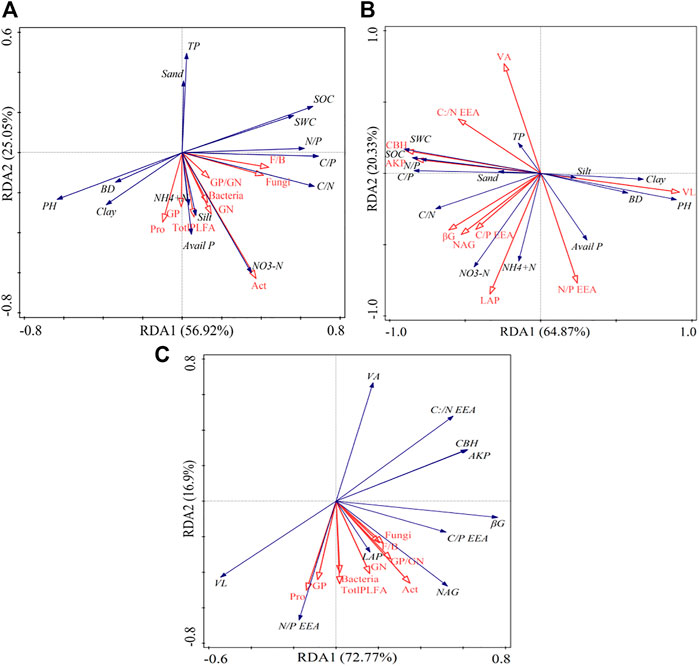
FIGURE 6. Redundancy analysis (RDA) showing the relationships between soil physicochemical properties and microbial components (A), soil physicochemical properties and enzymatic variables (B), soil physicochemical properties, soil microbial components, and enzymatic variables (C). SWC: soil water content; BD: bulk density; SOC: soil organic carbon; TN: total nitrogen; TP: total phosphorus; AP: available phosphorus; NO3−-N: nitrate nitrogen; NH4+-N: ammonium nitrogen; C/N: the ratio of SOC to TN; C/P: the ratio of SOC to TP; N/P: the ratio of TN to TP.
The RDA analysis further revealed that the soil enzyme activities and their stoichiometry were shaped by the variations in soil physicochemical properties, constituting 85.20% of the soil enzymes and their stoichiometry (Figure 6B). Specifically, pH and BD had a strong negative correlation with the soil enzyme activities and their stoichiometry. The SWC, SOC, and soil nutrients stoichiometry positively correlated with soil enzyme activities. Additionally, the Monte Carlo test revealed that the pH was the most influential variable on soil enzyme activity and stoichiometry (Table 2).
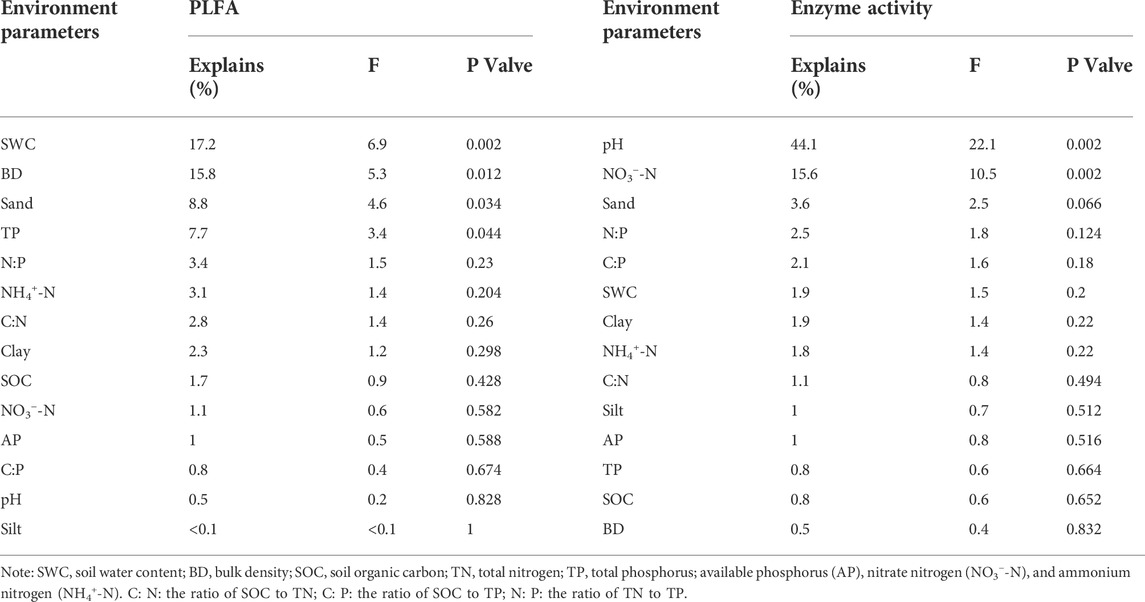
TABLE 2. Results of redundancy analysis of soil microbial and enzymatic variation using environmental variables, determined by forward selection procedure with unrestricted permutation tests.
The RDA analysis also revealed a negative and positive relationship between the soil microbial properties and enzyme activities and their stoichiometry (Figure 6C). The soil enzymatic activity and their stoichiometry were responsible for 89.67% of the soil microbial community components and structures variation, with N: PEEA as the most significant contributing 11.6% of the variation (Table 3 and Figure 6C).
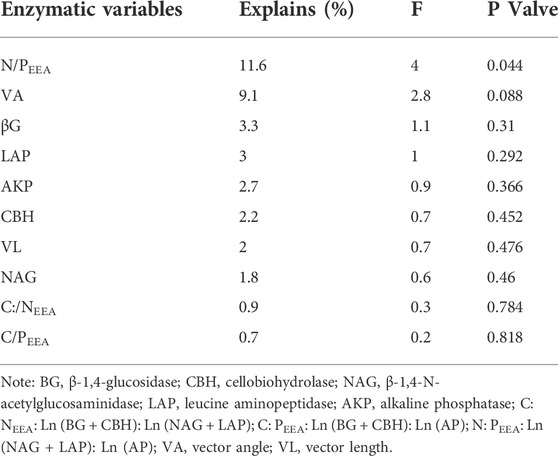
TABLE 3. Results of redundancy analysis of soil microbial variation and enzymatic variables, determined by forward selection procedure with unrestricted permutation tests.
Discussion
Variations in the microbial community and enzyme activities
The difference in vegetation species richness and soil properties in the mountain ecosystems is a result of the changes in altitude, which affects the microbial community (Kaiser et al., 2010; Tang et al., 2020). Forest ecosystems with different levels of tree species diversity differ significantly in litter quantity, quality, and root exudate input, which in turn affect soil bacterial diversity and community structure (Zhang et al., 2019a; Rivest et al., 2019). In the Helan Mountains, the abundance of soil microorganisms from the highest to the lowest across the different altitudes are bacteria, actinomycetes, fungi, and protozoa, with the highest abundance recorded at 2,139 m (Figure 1), attribute to higher plant richness (Supplementary Table S1) and higher nutrient availability (Table 1) at 2,139 m. Moreover, previous studies have shown that diverse plant communities promote high soil microbial diversity (Lange et al., 2015). Zak et al. (2003) also found that plant community species are related to the PLFA content of soil microbial community, with the increase of plant species richness, the PLFA content of bacteria and actinomycetes in soil decreases, while the PLFA content of fungi increases. In the study, the diversity of plant communities was significantly higher at mid and high elevations. For example, the broad-leaved trees were distributed between 2,139–2,438 m in Helan Mountains. Thus, their nutrient-rich litter provides sufficient nutrients to the microorganisms, hence the high soil microbial diversity and abundance. Our results are consistent with those of previous studies, indicating that high plant diversity can increase soil bacterial biomass (Chen et al., 2019b; Zhang et al., 2019c).
Fungi and bacteria are the two most important microbial species in soil ecosystems. Previous studies suggests that F: B ratio reflects the variation in the relative abundance of the two populations, with F: B ˂ one indicating the dominance of bacteria biomass (De et al., 2016). In the present study, the abundance of fungi, bacteria and their ratio were higher at 2,139 m than at the other altitudes, indicating that mid elevation provides a more stable ecosystem and suitable growth environment for fungi (Figure 1). This is parallel with the findings of Liu et al. (2013), who established that the highest fungal abundance in Helan Mountains was between 1900–2,100 m, implying that temperature, water, organic matter, and other soil conditions at these altitudes are more suitable for fungal growth. The F: B ratio denotes the variation in the relative abundance of two populations. The higher the ratio, the more stable the ecosystem. In this study, relative abundance analysis of eight microbial indicators revealed differences in the number of soil microorganisms at different altitudes, and altitudes 2,139–2,249 m exhibited the highest abundance (Supplementary Figure S2). The high biodiversity richness at this altitude is consistent with the central expansion theory of the mountain ecosystems, where water is the limiting factor in the low altitude areas, and heat in the high-altitude areas, hence the large productivity and high species diversity in the central region (Jiang et al., 2007).
When the soil nutrients are limited, microorganisms increase their C, N, and P acquisition to meet their nutrient needs by increasing their resource input, majorly resource enzymes, which increases the activity of decomposing enzymes (Mooshammer et al., 2014b). Soil enzymes are key in SOC decomposition and nutrient recycling. Microbial demand is influenced by the five extracellular enzymes, reflected in the C, N, and P availability. The activities of various enzymes were highest at the middle altitude (2,139 m) and were significantly increased between 1,650 and 2,139 m (Figure 2). This can be attributed to the vegetation type in the Helan Mountains, which changes from the desert-grassland-shrub-forest with an increase in altitude. Liu et al. (2013) revealed that the highest plant species richness, developed roots, better litter quality, and higher diversity of plant root exudates in the Helan Mountains occur at 1700–2,200 m. Besides, precipitation increases while soil temperature decreases with an increase in altitude, ensuring good hydrothermal conditions, which enhance soil enzyme activity at the mid-altitudes.
Variations in ecoenzymatic stoichiometry and nutrient limitation
The soil microbial nutrient acquisition is characterized by enzyme stoichiometry, which reflects the acquisition ability of microbial nutrients, availability of limited resources, and energy and nutrients limitations (Li et al., 2020). Understanding the limitation of microbial resources is key to predicting the microbial metabolic responses following global climate change and long-term vegetation enclosure (Zhang et al., 2019a; Zheng et al., 2020). In this study, the soil C: NEEA and C: PEEA ratios were higher in the mid and higher elevations (2,139–2,248 m) than in lower elevations (1,380–1,650 m) (Figure 3). Moreover, the standard principal axis regression analysis revealed that soil microbes are more highly dependent on (BG + CBH) enzymes than (NAG + LAP) and AP enzymes. Additionally, the vector length was significantly higher at mid and higher altitudes than at lower altitudes, implying a significant C limitation at the mid and higher elevations (Fanin et al., 2016; Zhang et al., 2021). All these results support our first hypothesis. The microbes also had a higher dependency on the P-acquiring enzyme than (NAG + LAP) enzyme (Figure 4). This implies that the microbes preferred to obtain C- and P-cycle enzymes than N-cycle enzymes, indicating that C and P were limited in the study area. The richness and diversity of plants affect the structure and diversity of soil microbial community, plants usually dominate in nutrient competition with soil microorganisms (Regan et al., 2016). Improvement in vegetation diversity increases the absorption of soil nutrients by plant communities, resulting in insufficient energy (C) and nutrients (nitrogen and phosphorus) available to microorganisms (Lemanski et al., 2019; Yan et al., 2022). This trigger microorganisms to secrete more energy and nutrients related enzymes to alleviate the restriction of nutrient metabolism caused by plant competition. Therefore, an increase in plant diversity and vegetation coverage makes it more difficult for the originally insufficient energy and nutrients in Helan Mountain to be utilized by microorganisms, which are gradually limited by C and P with the increase of altitude gradient, microorganisms (Figure 7). Simultaneously, the results reveal that microorganisms play a better role in obtaining carbon and phosphorus resources.
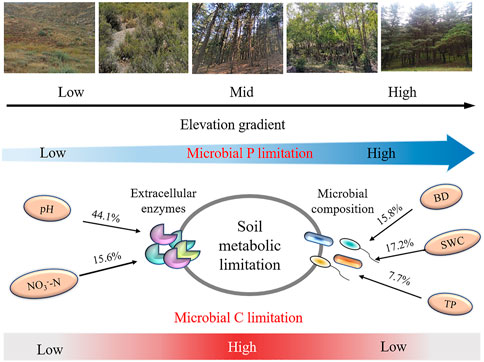
FIGURE 7. The conceptual diagram illustrating how enzyme stoichiometry and microbe nutrient limitation respond to elevation increas in Helan Mountains. BD: bulk density; SWC: soil water content; TP: total phosphorus; NO3−-N: nitrate nitrogen.
The vector angles were significantly higher in mid and high elevations (2,139–2,248 m) than at the lower elevations (1,380–1,650 m), implying a greater P limitation in the mid and higher elevations (Figure 5). These findings partially support our second hypothesis. In this study, microorganisms at high altitudes were more restricted by P, which may be due to: 1) soil P mainly comes from rock weathering (Helfenstein et al., 2018). Low temperature all year round at high altitude reduces rock weathering. Soil P becomes the main limiting element because of its continuous loss and difficulty to be supplemented (Hou et al., 2020); 2) P is an essential nutrient element for plant growth and metabolism. In high altitude areas, plant density and growth status are good (mixed forest and Picea crassifolia), which intensifies the competition of aboveground and underground ecological processes for soil P, thus microorganisms can alleviate P limitation by improving AKP activity. Vegetation enclosure contributes to increased soil N through plant litter input, fine roots in the soil, and less human activity (Zhang et al., 2016). In this study, the vector angle was <45°, implying that the area was subject to N limitation (Figure 5). This can be explained by the lower (BG + CBH): (NAG + LAP) ratio (1.04) and higher (NAG + LAP): AP ratio (1.02) than that of the global and tropical soils (Sinsabaugh et al., 2009; Waring et al., 2013). Besides, based on the EES theory, microorganisms experience a certain degree of nitrogen limitation in temperate forests (Jing et al., 2020). Thus, the limited N availability in areas with dry climates in our study may be due to the sparse Prunus mongolica shrubs with low inputs of litter and fine roots biomass (Delgado-Baquerizo et al., 2015; Wu et al., 2021b).
4.3 The Relative Contributions of Different Factors to Microbial and Enzyme
Soil microbial abundance and soil organic matter are closely related (Deng et al., 2016). Soil TP, TN, and their ratios, which are indices that effectively measure SOM (Wal et al., 2006), positively or negatively correlate with soil microorganisms (Figure 6A). Therefore, indicators of the quantity and quality of SOM may have led to the change in soil microbial abundance after long-term vegetation enclosure (Stevenson et al., 2016; Xiao et al., 2020). The pH positively or negatively impacts the soil PLFA due to the influence of parent materials, vegetation types, and research sites (Hu et al., 2020). The negative effect of pH on soil microbial PLFA may be due to the effect of substrate on the soil microbial community. Plants indirectly influence underground microbial community, which maintains its own needs by decomposing the organic matter released by the plant roots (Massaccesi et al., 2015). The change in plant diversity also alters the type and quantity of the corresponding root exudates, the acidity and alkalinity of the substrate decomposed by microorganisms, as well as the composition and structure of the microbial community (Chen and Chen, 2019). Further research is needed to quantitatively understand the correlation mechanism between plants and soil microorganisms. Soil enzyme activity is regulated by various factors, including soil physicochemical properties, which affect extracellular enzyme activity by altering the substrate concentration and nutrient availability. Additionally, soil moisture content affects enzyme activity by inhibiting the diffusion of compounds and altering the reaction conditions and sites for various enzymatic reactions (Kivlin and Treseder, 2014). Based on the redundancy analysis, SWC positively correlated with the soil enzyme activities and their stoichiometry, implying that high soil moisture content enhances the soil enzyme activity (Figure 6B). In this study, with the increase in altitude, an increase in soil water alleviated the restriction of water on plants, promoting the increase in plant species richness and diversity. Meanwhile, the low temperature at high altitude limited the decomposition of organic carbon, and the increase in carbon content mediated the substrate concentration of enzyme action, thus promoting the increase in soil carbon-related extracellular enzyme activity (Weintraub et al., 2013). Besides, soil pH changes the conformation of enzyme activity sites; thus, investigating the effect of pH on soil extracellular enzyme activity is key to understanding plant and microbial communities (Sinsabaugh et al., 2002; Jing et al., 2016). There was a significant negative correlation between the soil pH and enzyme activities and their stoichiometry in our study. Specifically, alkalinity decreased with an increase in altitude. Usually, as soil alkalinity gradually decreases, its inhibitory impact on soil enzyme activity gradually weakens (Peng and Wang, 2016).
Soil nutrient content indirectly affects the soil enzymes by influencing plants and microbial growth, leading to the interdependence between the soil enzyme activity and nutrients (Pang et al., 2018b). When the nutrient utilization rate is low, microorganisms meet the plant nutrients demand by secreting the corresponding enzymes (Olander and Vitousek, 2000; Wallenius et al., 2011). This is because the soil enzyme activity is jointly regulated by plant nutrient demand and supply, resulting in an indistinct quantitative relationship between the enzyme activity and nutrient elements. However, a significant relationship between enzyme activity and SOC exists due to the higher carbon level serving as a substrate for microbial growth, indirectly promoting a higher enzyme activity (Balota et al., 2003; Cui et al., 2019a). In this study, the SOC, soil nutrients, and their stoichiometry positively correlated with the soil microorganism and enzyme activities, attributed to SOC and soil nutrients supplying energy to the soil microorganisms and enzymes (Sinsabaugh et al., 2009). Therefore, the relationship between the soil nutrients, microbial, and enzyme activities implies that the microbial properties in different vegetation types depend on the nutrient input and hydrothermal variations along the altitude gradient.
Conclusion
This study illustrates the patterns of soil microbial community structure, soil enzyme activity, and microbial resource limitation in the mountain ecosystem in arid regions. We demonstrated that soil pH and BD decrease with an increase in elevation, while SWC, SOC, TN, CBH, and AKP activities increase with an increase in elevation, reaching the maximum at 2,438 m. Total microbial, bacterial, fungal, actinomycetes, F: B, BG, NAG, and LAP first increased and then decreased with the increase in the elevation. Soil microbial metabolism was limited by C and P because of higher investment in C- and P-acquiring enzymes than N-acquiring enzymes. Furthermore, microbial P limitation increased with elevation, while C limitation was highest at mid elevation. Overall, edaphic factors, mainly soil pH, SWC, and BD are the main regulators of the microbial metabolism processes. These findings will provide new knowledge that can be implemented to enhance soil microbial limitations in the mountain ecosystem in arid regions.
Data availability statement
The original contributions presented in the study are included in the article/Supplementary Material, further inquiries can be directed to the corresponding authors.
Author contributions
MW: Conceptualization, Methodology, Software, and Drafting of the manuscript; XL and YH: Resources, Visualization, Software, and Data curation; ZL and YZ: Software, review, and editing; LC and XX: Validation, Formal analysis, Visualization, and Field investigations; DP and XL: Resources, review, editing, and Supervision. All authors contributed to the article and approved the submitted version.
Funding
This project was funded by the Key Research and Development Project of Ningxia Hui Autonomous Region (2021BEG02005, 2020BFG03006, and 2021BEB04001), the Open Project Program of Key laboratory of Desertification Control and Soil and Water Conservation of Ningxia (KF202203), and the National Natural Science Foundation of China (32201631; 32101524).
Acknowledgments
The authors appreciate the editor and the reviewers for their constructive suggestions.
Conflict of interest
The authors declare that the research was conducted in the absence of any commercial or financial relationships that could be construed as a potential conflict of interest.
Publisher’s note
All claims expressed in this article are solely those of the authors and do not necessarily represent those of their affiliated organizations, or those of the publisher, the editors and the reviewers. Any product that may be evaluated in this article, or claim that may be made by its manufacturer, is not guaranteed or endorsed by the publisher.
Supplementary material
The Supplementary Material for this article can be found online at: https://www.frontiersin.org/articles/10.3389/fenvs.2022.1041964/full#supplementary-material
References
Balota, E. L., Colozzi-Filho, A., Andrade, D. S., and Dick, R. P. (2003). Microbial biomass in soils under different tillage and crop rotation systems. Biol. Fertil. Soils 38, 15–20. doi:10.1007/s00374-003-0590-9
Cao, X., Shi, Z., Chen, J., Liu, S., Zhang, M., Chen, M., et al. (2022). Extracellular enzyme characteristics and microbial metabolic limitation in soil of subalpine forest ecosystems on the eastern Qinghai-Tibetan Plateau. Plant Soil. doi:10.1007/s11104-022-05521-2
Chang, E. H., Li, P., Li, Z. B., Xiao, L., Zhao, B. H., Su, Y. Y., et al. (2019). Using water isotopes to analyze water uptake during vegetation succession on abandoned cropland on the Loess Plateau, China. Catena 181, 104095. doi:10.1016/j.catena.2019.104095
Chen, C., Chen, H. Y. H., Chen, X. L., and Huang, Z. Q. (2019a). Meta-analysis shows positive effects of plant diversity on microbial biomass and respiration. Nat. Commun. 10 (1), 1332. doi:10.1038/s41467-019-09258-y
Chen, H., Li, D. J., Mao, Q. G., Xiao, K. C., and Wang, K. L. (2019b). Resource limitation of soil microbes in karst ecosystems. Sci. Total Environ. 650, 241–248. doi:10.1016/j.scitotenv.2018.09.036
Chen, L. Y., Liu, L., Mao, C., Qin, S. Q., Wang, J., Liu, F. T., et al. (2018). Nitrogen availability regulates topsoil carbon dynamics after permafrost thaw by altering microbial metabolic efficiency. Nat. Commun. 9, 3951. doi:10.1038/s41467-018-06232-y
Chen, X. L., and Chen, H. Y. H. (2019). Plant diversity loss reduces soil respiration across terrestrial ecosystems. Glob. Chang. Biol. 25 (4), 1482–1492. doi:10.1111/gcb.14567Clemmensen
Clemmensen, K. E., Bahr, A., Ovaskainen, O., Dahlberg, A., Ekblad, A., Wallander, H., et al. (2013). Roots and associated fungi drive longterm carbon sequestration in boreal forest. Science 339, 1615–1618. doi:10.1126/science.1231923
Cui, Y., Bing, H., Moorhead, D. L., Delgado-Baquerizo, M., Ye, L., Yu, J., et al. (2022). Ecoenzymatic stoichiometry reveals widespread soil phosphorus limitation to microbial metabolism across Chinese forests. Commun. Earth Environ. 3, 184. doi:10.1038/s43247-022-00523-5
Cui, Y. X., Bing, H. J., Fang, L. C., Jiang, M., Shen, G. T., Yu, J. L., et al. (2019a). Extracellular enzyme stoichiometry reveals the carbon and phosphorus limitations of microbial metabolisms in the rhizosphere and bulk soils in alpine ecosystems. Plant Soil 458 (1), 7–20. doi:10.1007/s11104-019-04159-x
Cui, Y. X., Fang, L. C., Deng, L., Guo, X. B., Han, F., Ju, W. L., et al. (2019b). Patterns of soil microbial nutrient limitations and their roles in the variation of soil organic carbon across a precipitation gradient in an arid and semi-arid region. Sci. Total Environ. 658, 1440–1451. doi:10.1016/j.scitotenv.2018.12.289
De, G. V., Bekele, I., Dipchansingh, D., Wuddivira, M. N., De, C. S., Boman, M., et al. (2016). Microbial community structure and function of soil following ecosystem conversion from native forests to teak plantation forests. Front. Microbiol. 7, 1976. doi:10.3389/fmicb.2016.01976
Delgado-Baquerizo, M., García-Palacios, P., Milla, R., Gallardo, A., and Maestre, F. T. (2015). Soil characteristics determine soil carbon and nitrogen availability during leaf litter decomposition regardless of litter quality. Soil Biol. Biochem. 81, 134–142. doi:10.1016/j.soilbio.2014.11.009
Deng, L., Peng, C. H., Huang, C. B., Wang, K. B., Liu, Q. Y., Liu, Y. L., et al. (2019). Drivers of soil microbial metabolic limitation changes along a vegetation restoration gradient on the Loess Plateau, China. Geoderma 353, 188–200. doi:10.1016/j.geoderma.2019.06.037
Deng, Q., Cheng, X. L., Hui, D. F., Zhang, Q., Li, M., and Zhang, Q. F. (2016). Soil microbial community and its interaction with soil carbon and nitrogen dynamics following afforestation in central China. Sci. Total Environ. 541, 230–237. doi:10.1016/j.scitotenv.2015.09.080
Dong, C. C., Wang, W., Liu, H. Y., Xu, X. T., and Zeng, H. (2019). Temperate grassland shifted from nitrogen to phosphorus limitation induced by degradation and nitrogen deposition: Evidence from soil extracellular enzyme stoichiometry. Ecol. Indic. 101, 453–464. doi:10.1016/j.ecolind.2019.01.046
Fanin, N., Moorhead, D., and Bertrand, I. (2016). Eco-enzymatic stoichiometry and enzymatic vectors reveal differential C, N, P dynamics in decaying litter along a land-use gradient. Biogeochemistry 129, 21–36. doi:10.1007/s10533-016-0217-5
Frostegard, A., and Baath, E. (1996). The use of phospholipid fatty acid analysis to estimate bacterial and fungal biomass in soil. Biol. Fertil. Soils 22, 59–65. doi:10.1007/BF00384433
Frostegard, A., Tunlid, A., and Baath, E. (1991). Microbial biomass measured as total lipid phosphate in soils of different organic content. J. Microbiol. Methods 14, 151–163. doi:10.1016/0167-7012(91)90018-L
Guo, X. P., Chen, H. Y. H., Meng, M. J., Biswas, S. R., Ye, L. X., and Zhang, J. C. (2016). Effects of land use change on the composition of soil microbial communities in a managed subtropical forest. For. Ecol. Manag. 373, 93–99. doi:10.1016/j.foreco.2016.03.048
He, Q., Wu, Y., Bing, H., Zhou, J., and Wang, J. (2020). Vegetation type rather than climate modulates the variation in soil enzyme activities and stoichiometry in subalpine forests in the eastern Tibetan Plateau. Geoderma 374, 114424. doi:10.1016/j.geoderma.2020.114424
Hill, B. H., Elonen, C. M., Jicha, T. M., Kolka, R. K., Lehto, L. L. P., Sebestyen, S. D., et al. (2014). Ecoenzymatic stoichiometry and microbial processing of organic matter in Northern bogs and 500 fens reveals a common P-limitation between peatland types. Biogeochemistry 120, 203224.501. doi:10.1007/s10533-014-9991-0
Helfenstein, J. L., Tamburini, F., Sperber, C. V., Massey, M. S., Pistocchi, C., Chadwick, O. A., et al. (2018). Combining spectroscopic and isotopic techniques gives a dynamic view of phosphorus cycling in soilEcoenzymatic stoichiometry and microbial processing of organic matter in northern bogs and fens reveals a common P-limitation between peatland types. Nat. Commun 9120, 3226203–3226224. doi:10.1038/s41467-018-05731-2
Helfrich, M., Ludwig, B., Thoms, C., Gleixner, G., and Flessa, H. (2015). The role of soil fungi and bacteria in plant litter decomposition and macroaggregate formation determined using phospholipid fatty acids. Appl. Soil Ecol. 96, 261–264. doi:10.1016/j.apsoil.2015.08.023
Hou, E. Q., Luo, Y. Q., Kuang, Y. W., Chen, C. R., Lu, X. K., Jiang, L. F., et al. (2020). Global meta-analysis shows pervasive phosphorus limitation of aboveground plant production in natural terrestrial ecosystems. Nat. Commun. 11, 637. doi:10.1038/s41467-020-14492-w
Hu, P. L., Xiao, J., Zhang, W., Xiao, L. M., Yang, R., Xiao, D., et al. (2020). Response of soil microbial communities to natural and managed vegetation restoration in a subtropical karst region. Catena 195, 104849. doi:10.1016/j.catena.2020.104849
Jian, Z., Ni, Y., Zeng, L., Lei, L., Xu, J., Xiao, W., et al. (2021). Latitudinal patterns of soil extracellular enzyme activities and their controlling factors in Pinus massoniana plantations in subtropical China. For. Ecol. Manag. 495, 119358. doi:10.1016/j.foreco.2021.119358
Jiang, Y., Kang, M. Y., Zhu, Y., and Xu, G. C. (2007). Plant biodiversity patterns on helan mountain, China. Acta Oecol. 32, 125–133. doi:10.1016/j.actao.2006.12.003
Jiang, Y. L., Lei, Y. B., Qin, W., Korpelainen, H., and Li, C. Y. (2019). Revealing microbial processes and nutrient limitation in soil through ecoenzymatic stoichiometry and glomalinrelated soil proteins in a retreating glacier forefield. Geoderma 338, 313–324. doi:10.1016/j.geoderma.2018.12.023
Jing, X., Chen, X., Fang, J. Y., Ji, C. J., Shen, H. H., Zheng, C. Y., et al. (2020). Soil microbial carbon and nutrient constraints are driven more by climate and soil physicochemical properties than by nutrient addition in forest ecosystems. Soil Biol. Biochem. 141, 107657. doi:10.1016/j.soilbio.2019.107657
Jing, X., Yang, X. X., Ren, F., Zhou, H. K., Zhu, B., and He, J. S. (2016). Neutral effect of nitrogen addition and negative effect of phosphorus addition on topsoil extracellular enzymatic activities in an alpine grassland ecosystem. Appl. Soil Ecol. 107, 205–213. doi:10.1016/j.apsoil.2016.06.004
Jones, D. L., Kielland, K., Sinclair, F. L., Dahlgren, R. A., Newsham, K. K., Farrar, J. F., et al. (2009). Soil organic nitrogen mineralization across a global latitudinal gradient. Glob. Biogeochem. Cycles 23 (1), 1–5. doi:10.1029/2008GB003250
Kaiser, C., Koranda, M., Kitzler, B., Fuchslueger, L., Schnecker, J., Schweiger, P., et al. (2010). Belowground carbon allocation by trees drives seasonal patterns of extracellular enzyme activities by altering microbial community composition in a beech forest soil. New Phytol. 187 (3), 843–858. doi:10.1111/j.1469-8137.2010.03321.x
Kivlin, S. N., and Treseder, K. K. (2014). Soil extracellular enzyme activities correspond with abiotic factors more than fungal community composition. Biogeochemistry 117, 23–37. doi:10.1007/s10533-013-9852-2
Lange, M., Eisenhauer, N., Sierra, C. A., Bessler, H., Engels, C., Griffiths, R. I., et al. (2015). Plant diversity increases soil microbial activity and soil carbon storage. Nat. Commun. 6, 6707. doi:10.1038/ncomms7707
Lemanski, K., Armbruster, M., and Bonkowski, M. (2019). Linking soil microbial nutrient limitation to fertilizer regime and sugar beet yield. Plant Soil 441 (1-2), 253–259. doi:10.1007/s11104-019-04114-w
Li, J. J., Zhou, X. M., Yan, J. X., Li, H. J., and He, J. Z. (2015). Effects of regenerating vegetation on soil enzyme activity and microbial structure in reclaimed soils on a surface coal mine site. Appl. Soil Ecol. 87, 56–62. doi:10.1016/j.apsoil.2014.11.010
Li, J. W., Shangguan, Z. P., and Deng, L. (2020). Dynamics of soil microbial metabolic activity during grassland succession after farmland abandonment. Geoderma 363, 114167. doi:10.1016/j.geoderma.2019.114167
Liu, B. R., Zhang, X. Z., Hu, T. H., and Li, W. J. (2013). Soil microbial diversity under typical vegetation zones along an elevation gradient in Helan Mountains. Acta eco. Sin. 33 (22), 7211–7220. doi:10.5846/stxb201208061110
Massaccesi, L., Bardgett, R. D., Agnelli, A., Ostle, N., Wilby, A., and Orwin, K. H. (2015). Adjustment of microbial nitrogen use efficiency to carbon:nitrogen imbalances regulates soil nitrogen cycling. Nat. Commun. 177 (3), 747–759. doi:10.1007/s00442-014-3135-z
Moorhead, D. L., Sinsabaugh, R. L., Hill, B. H., and Weintraub, M. N. (2016). Vector analysis of ecoenzyme activities reveal constraints on coupled C, N and P dynamics. Soil Biol. Biochem. 93, 1–7. doi:10.1016/j.soilbio.2015.10.019
Mooshammer, M., Wanek, W., Haemmerle, I., Fuchslueger, L., Hofhansl, F., Knoltsch, A., et al. (2014a). Adjustment of microbial nitrogen use efficiency to carbon: Nitrogen imbalances regulates soil nitrogen cycling. Nat. Commun. 5, 3694. doi:10.1038/ncomms4694
Mooshammer, M., Wanek, W., Zechmeister-Boltenstern, S., and Bichter, A. (2014b). Stoichiometric imbalances between terrestrial decomposer communities and their resources: Mechanisms and implications of microbial adaptations to their resources. Front. Microbiol. 5, 22. doi:10.3389/fmicb.2014.00022
Olander, L. P., and Vitousek, P. M. (2000). Regulation of soil phosphatase and chitinase activity by N and P availability. Biogeochemistry 49, 175–191. doi:10.1023/A:1006316117817
Orwin, K. H., Ostle, N., Wilby, A., and Bardgett, R. D. (2014). Effects of species evenness and dominant species identity on multiple ecosystem functions in model grassland communities. Oecologia 174, 979–992. doi:10.1007/s00442-013-2814-5
Palomo, A., Fowler, S. J., Gülay, A., Rasmussen, S., Sicheritz-Ponten, T., and Smets, B. F. (2016). Metagenomic analysis of rapid gravity sand filter microbial communities suggests novel physiology of Nitrospira spp. ISME J. 10, 2569–2581. doi:10.1038/ismej.2016.63
Pang, D. B., Cao, J. H., Dan, X. Q., Guan, Y. H., Peng, X. W., Cui, M., et al. (2018a). Recovery approach affects soil quality in fragile karst ecosystems of southwest China: Implications for vegetation restoration. Ecol. Eng. 123, 151–160. doi:10.1016/j.ecoleng.2018.09.001
Pang, D. B., Wang, G. Z., Li, G. J., Sun, Y. L., Liu, Y. G., and Zhou, J. X. (2018b). Ecological stoichiometric characteristics of two typical plantations in the karst ecosystem of southwestern China. Forests 9, 56. doi:10.3390/f9020056
Peng, X. Q., and Wang, W. (2016). Stoichiometry of soil extracellular enzyme activity along a climatic transect in temperate grasslands of northern China. Soil Biol. Biochem. 98, 74–84. doi:10.1016/j.soilbio.2016.04.008
Regan, K., Stempfhuber, B., Schloter, M., Rasche, F., Prati, D., Philippot, L., et al. (2016). Spatial and temporal dynamics of nitrogen fixing, nitrifying and denitrifying microbes in an unfertilized grassland soil. Soil Biol. Biochem. 6, 214–226. doi:10.1016/j.soilbio.2016.11.011
Riggs, C. E., and Hobbie, S. E. (2016). Mechanisms driving the soil organic matter decomposition response to nitrogen enrichment in grassland soils. Soil Biol. Biochem. 99, 54–65. doi:10.1016/j.soilbio.2016.04.023
Rivest, M., Whalen, J. K., and Rivest, D. (2019). Tree diversity is not always a strong driver of soil microbial diversity: A 7-yr-old diversity experiment with trees. Ecosphere 10 (4), e02685. doi:10.1002/ecs2.2685
Rosinger, C., Rousk, J., and Sanden, H. (2019). Can enzymatic stoichiometry be used to determine growth-limiting nutrients for microorganisms? A critical assessment in two subtropical soils. Soil Biol. Biochem. 128, 115–126. doi:10.1016/j.soilbio.2018.10.011
Saiya-Cork, K. R., Sinsabaugh, R. L., and Zak, D. R. (2002). The effects of long term nitrogen deposition on extracellular enzyme activity in an Acer saccharum forest soil. Soil Biol. Biochem. 34, 1309–1315. doi:10.1016/S0038-0717(02)00074-3
Sinsabaugh, R. L., Carreiro, M. M., and Repert, D. A. (2002). Allocation of extracellular enzymatic activity in relation to litter composition, N deposition, and mass loss. Biogeochemistry 60, 1–24. doi:10.1023/A:1016541114786
Sinsabaugh, R. L., Hill, B. H., and Follstad Shah, J. J. (2009). Ecoenzymatic stoichiometry of microbial organic nutrient acquisition in soil and sediment. Nature 462, 795–798. doi:10.1038/nature08632
Spaans, F., Caruso, T., Hammer, E. C., and Montgomery, I. (2019). Trees in trimmed hedgerows but not tree health increase diversity of oribatid mite communities in intensively managed agricultural land. Soil Biol. Biochem. 138, 107568. doi:10.1016/j.soilbio.2019.107568
Stark, S., Männistö, M. K., and Eskelinen, A. (2014). Nutrient availability and pH jointly constrain microbial extracellular enzyme activities in nutrient-poor tundra soils. Plant Soil 383, 373–385. doi:10.1007/s11104-014-2181-y
Stevenson, B. A., Sarmah, A. K., Smernik, R., Hunter, D. W. F., and Fraser, S. (2016). Soil carbon characterization and nutrient ratios across land uses on two contrasting soils: Their relationships to microbial biomass and function. Soil Biol. Biochem. 97, 50–62. doi:10.1016/j.soilbio.2016.02.009
Tang, M. Z., Li, L., Wang, X. L., You, J., Li, J. N., and Chen, X. (2020). Elevational is the main factor controlling the soil microbial community structure in alpine tundra of the Changbai Mountain. Sci. Rep. 10 (1), 12442. doi:10.1038/s41598-020-69441-w
Wal, A. V. D., van Veen, J. A., Smant, W., Boschker, H. T. S., Bloem, J., Kardol, P., et al. (2006). Fungal biomass development in a chronosequence of land abandonment. Soil Biol. Biochem. 38, 51–60. doi:10.1016/j.soilbio.2005.04.017
Wallenius, K., Rita, H., Mikkonen, A., Lappi, K., Lindström, K., Hartikainen, H., et al. (2011). Effects of land use on the level, variation and spatial structure of soil enzyme activities and bacterial communities. Soil Biol. Biochem. 43, 1464–1473. doi:10.1016/j.soilbio.2011.03.018
Wang, Q. K., Wang, S. L., He, T. X., Liu, L., and Wu, J. B. (2014). Response of organic carbon mineralization and microbial community to leaf litter and nutrient additions in subtropical forest soils. Soil Biol. Biochem. 71, 13–20. doi:10.1016/j.soilbio.2014.01.004
Waring, B. G., Weintraub, S. R., and Sinsabaugh, R. L. (2013). Ecoenzymatic stoichiometry of microbial nutrient acquisition in tropical soils. Biogeochemistry 117, 101–113. doi:10.1007/s10533-013-9849-x
Weintraub, S. R., Wieder, W. R., Cleveland, C. C., and Townsend, A. R. (2013). Organic matter inputs shift soil enzyme activity and allocation patterns in a wet tropical forest. Biogeochemistry 114, 313–326. doi:10.1007/s10533-012-9812-2
Wu, M. Y., Pang, D. B., Chen, L., Li, X. B., Liu, L. Z., Liu, B., et al. (2021b). Chemical composition of soil organic carbon and aggregate stability along an elevation gradient in Helan Mountains, northwest China. Ecol. Indic. 131, 108228. doi:10.1016/j.ecolind.2021.108228
Wu, Y., Chen, W., Li, Q., Guo, Z., Li, Y., Zhao, Z., et al. (2021a). Ecoenzymatic stoichiometry and nutrient limitation under a natural secondary succession of vegetation on the Loess Plateau, China. Land Degrad. Dev. 32, 399–409. doi:10.1002/ldr.3723
Xiao, L., Liu, G., Li, P., Li, Q., and Xue, S. (2020). Ecoenzymatic stoichiometry and microbial nutrient limitation during secondary succession of natural grassland on the Loess Plateau. China. Soil Tillage Res. 200, 104605. doi:10.1016/j.still.2020.104605
Yan, B. S., Duan, M. C., Wang, R. C., Li, J. J., Wei, F. R., Chen, J. R., et al. (2022). Planted forests intensified soil microbial metabolic nitrogen and phosphorus limitation on the Loess Plateau, China. Catena 211, 105982. doi:10.1016/j.catena.2021.105982
Yu, J. L., Bing, H. J., Chang, R. Y., Cui, Y. X., Shen, G. T., Wang, X. X., et al. (2022). Microbial metabolic limitation response to experimental warming along an altitudinal gradient in alpine grasslands, eastern Tibetan Plateau. Catena 214, 106243. doi:10.1016/j.catena.2022.106243
Zak, D. R., Holmes, W. E., White, D. C., Peacock, A. D., and Tilman, D. (2003). Plant diversity, soil microbial communities, and ecosystem function: Are there any links? Ecology 84 (8), 2042–2050. doi:10.1890/02-0433
Zeng, X. M., Wei, C. F., Liu, X. H., and Zhang, L. N. (2020). Qinghai spruce (Picea crassifolia) and Chinese pine (Pinus tabuliformis) show high vulnerability and similar resilience to earlygrowing-season drought in the Helan Mountains, China. Ecol. Indic. 110, 105871. doi:10.1016/j.ecolind.2019.105871
Zhang, J. T., and Dong, Y. R. (2010). Factors affecting species diversity of plant communities and the restoration process in the loess area of China. Ecol. Eng. 36, 345–350. doi:10.1016/j.ecoleng.2009.04.001
Zhang, J. Y., Ai, Z. M., Liang, C. T., Wang, G. L., Liu, G. B., and Xue, S. (2019a). How microbes cope with short-term N addition in a Pinus tabuliformis forest-ecological stoichiometry. Geoderma 337, 630–640. doi:10.1016/j.geoderma.2018.10.017
Zhang, Q., Wu, J. J., Yang, F., Lei, Y., Zhang, Q. F., and Cheng, X. L. (2016). Alterations in soil microbial community composition and biomass following agricultural land use change. Sci. Rep. 6, 36587. doi:10.1038/srep36587
Zhang, Q., Zhang, D. D., Wu, J. J., Li, J. S., Feng, J., and Cheng, X. L. (2021). Soil nitrogen-hydrolyzing enzyme activity and stoichiometry following a subtropical land use change. Land Degrad. Dev. 32, 4277–4287. doi:10.1002/ldr.4034
Zhang, W., Xu, Y. D., Gao, D. X., Wang, X., Liu, W. C., Deng, J., et al. (2019b). Ecoenzymatic stoichiometry and nutrient dynamics along a revegetation chronosequence in the soils of abandoned land and Robinia pseudoacacia plantation on the Loess Plateau, China. Soil Biol. Biochem. 134, 1–14. doi:10.1016/j.soilbio.2019.03.017
Zhang, X., Huang, Y. T., Liu, S. R., Fu, S. L., Ming, A. G., Li, X. Z., et al. (2019c). Mixture of tree species enhances stability of the soil bacterial community through phylogenetic diversity. Eur. J. Soil Sci. 70 (3), 644–654. doi:10.1111/ejss.12780
Zhao, F. Z., Feng, X. X., Guo, Y. X., Ren, C. J., Wang, J., and Doughty, R. (2020). Elevation gradients affect the differences of arbuscular mycorrhizal fungi diversity between root and rhizosphere soil. Agric. For. Meteorology 284, 107894. doi:10.1016/j.agrformet.2019.107894
Zheng, H. F., Liu, Y., Chen, Y. M., Zhang, J., Li, H. J., Wang, L. F., et al. (2020). Short-term warming shifts microbial nutrient limitation without changing the bacterial community structure in an alpine timberline of the eastern Tibetan Plateau. Geoderma 360, 113985. doi:10.1016/j.geoderma.2019.113985
Zhu, X. M., Liu, M., Kou, Y. P., Liu, D. Y., Liu, Q., Zhang, Z. L., et al. (2020). Differential effects of N addition on the stoichiometry of microbes and extracellular enzymes in the rhizosphere and bulk soils of an alpine shrubland. Plant Soil 449, 285–301. doi:10.1007/s11104-020-04468-6
Zornoza, R., Mataix-Solera, J., Guerrero, C., Arcenegui, V., Mayoral, A. M., Morales, J., et al. (2007). Soil properties under natural forest in the Alicante province of Spain. Geoderma 142, 334–341. doi:10.1016/j.geoderma.2007.09.002
Keywords: soil extracellular enzymes, enzymatic stoichiometry, microbial metabolic limitation, mountain ecosystem, elevation gradient
Citation: Wu M, Liu Z, Chen L, Pang D, Xu X, Zhang Y, Ni X, Hu Y and Li X (2022) Elevation gradient shapes microbial carbon and phosphorous limitations in the Helan Mountains, Northwest China. Front. Environ. Sci. 10:1041964. doi: 10.3389/fenvs.2022.1041964
Received: 12 September 2022; Accepted: 25 October 2022;
Published: 07 November 2022.
Edited by:
Bin Guo, Shandong University of Science and Technology, ChinaReviewed by:
Yang Li, Anhui University of Science and Technology, ChinaDong Wang, Henan University, China
Copyright © 2022 Wu, Liu, Chen, Pang, Xu, Zhang, Ni, Hu and Li. This is an open-access article distributed under the terms of the Creative Commons Attribution License (CC BY). The use, distribution or reproduction in other forums is permitted, provided the original author(s) and the copyright owner(s) are credited and that the original publication in this journal is cited, in accordance with accepted academic practice. No use, distribution or reproduction is permitted which does not comply with these terms.
*Correspondence: Danbo Pang, pang89028@163.com; Xuebin Li, lixuebin@nxu.edu.cn