- Department of Building, Civil and Environmental Engineering, Concordia University, Montreal, QC, Canada
Marine oil spills are serious ecological disasters that have massive adverse impacts on the environment. The impacts are even worse once the spilled oil is stranded on a shoreline. A series of shoreline cleanup methods are deployed to remove spilled oil, but their performance can be affected by the stranded oil. This review therefore comprehensively investigates the characteristics of spilled oil on the shoreline and explores their effects on the effectiveness of shoreline response operations. First, the five basic groups of spilled oil (i.e., non-persistent light oils, persistent light oils, medium oils, heavy oils, and sinking oils) are discussed and each oil fraction is introduced. Three distribution scenarios of adhered oil on shorelines are also analyzed. The effects of oil characteristics, such as oil type, viscosity, evaporation, and composition, on the performance of chemical treatments, physical methods, and biodegradation are then discussed and analyzed. Finally, the article provides recommendations for future research on aspects of shoreline oiling prevention, quick responses, response tool sets, and other considerations, which may have significant implications for future decision-making and the implementation of shoreline cleanup to effectively remove stranded oil.
1 Introduction
Marine oil spills are serious ecological disasters that still occur now and then (CCG, 2018; Chen et al., 2019; Hosseinipooya et al., 2022). According to the latest data and statistics of ITOPF (2022), one large (more than 700 tons) and five medium (between 7 and 700 tons) spill incidents occurred solely from tank vessels in 2021, which presents an upward trend compared to 2020. Spilled oil has adverse impacts on marine environments, and the situation is even worse when oil reaches the shoreline (Bi et al., 2021b). The fate and behaviors of spilled oil on shoreline can change with evaporation, photooxidation, biodegradation, partition, as well as aggregation, and beach morphodynamics (Wang et al., 2020; Huettel, 2022). The stranded oil on shoreline can cause the mortality of vegetation such as marshes, endanger habitats of marine life and coastal communities, accelerate shoreline erosion, and disrupt the tourist industry (McClenachan et al., 2013; Lin et al., 2016). To implement the shoreline cleanup, a series of response operations, which include chemical, physical, and biological methods, have been developed for spill responders to remove the stranded oil as much as possible while minimizing any disturbances to the coastal biota and communities (Wu et al., 2015; ECCC, 2016).
Based on previous studies, practices, and experiences in both laboratories and the field, it is known that the performance of shoreline response operations can be significantly affected by a wide range of factors, such as the oil characteristics, shoreline substrate types, oil types and volumes, and the thickness of the stranded oil (Owens, 2011; Michel and Fingas, 2016; Lyu and Fan, 2021; Zhou et al., 2021). The characteristics of stranded oil are one of the primary factors considered during shoreline cleanup as they not only influence the choice of response method, but also affect the effectiveness of oil removal (Prendergast and Gschwend, 2014). Broadly speaking, oil type and composition, viscosity, American Petroleum Institute (API) gravity/specific density, volatility, water solubility, and adhesion are considered oil characteristics and are related to the fate and behavior of the oil on shorelines, such as evaporation, penetration, retention, and burial (Mehanna et al., 2013; Mayes and Myers, 2014).
The characteristics of oil on shorelines have been studied extensively as spill responders are required to know the behaviors of stranded oil and determine how to deal with the oil. For example, highly weathered oil or oil with a high content of asphaltene or resin fractions on a shoreline will have high viscosity and adhesiveness, which will result in greater oil retention on the surface and less oil penetration into the subsurface (Boufadel et al., 2019). Regular water washing may not be efficient enough to remove such stranded oil, so the use of surface washing agents (SWAs) may be adopted to improve the oil removal performance (Zhang and Liu, 1997; Robertson and Maddox, 2003). For oil with high volatility, water washing and vacuuming can achieve the desired oil removal result although flammability hazards and inhalation toxicity may exist during the operation process (Feng et al., 2021; Berke et al., 2022). Meanwhile, oil stranded on a shoreline goes through continual weathering processes until it is cleaned up, so its physical and chemical properties will change and affect the performance of response options (Díez et al., 2007; Wang et al., 2020).
The aim of this review is therefore to comprehensively investigate the characteristics of spilled oil on shorelines and to explore their impacts on the effectiveness of shoreline response operations. In this review, the spilled oil will be classified according to typical oil properties and each oil fraction will be introduced. Three distribution scenarios of adhered oil on the surface of shoreline substrates will then be analyzed. The effects of oil characteristics on the performance of chemical and physical response methods as well as biodegradation will be discussed in detail. In addition, recommendations relating to oil characteristics on shorelines and shoreline cleanup will be provided.
2 Characteristics of spilled oil on shorelines
2.1 Types of spilled oil on shorelines
Oils are very varied and complex multicomponent mixtures that consist of thousands of chemicals (Chen et al., 2018). According to the oil classification by Office of Response and Restoration in National Oceanic and Atmospheric Administration, the spilled oil can be categorized into five basic groups, namely, non-persistent light oils, persistent light oils, medium oils, heavy oils, and sinking oils (NOAA, 2020). Once they have spilled into the marine environment, different groups of spilled oil may have various fates and transport trajectories due to their varied properties, such as API gravity, volatility, viscosity, flash/pour points, and toxicity (Riazi, 2005; Wang et al., 2020). These groups of oils therefore have varying degrees of impacts on shoreline environments and require different shoreline cleanup responses.
Detailed information regarding these five oil groups (i.e., aspects of their properties, oils, incident examples, and feasible cleanup options) are summarized in Table 1. Non-persistent oils have high volatility and toxicity and usually evaporate rapidly by themselves, so they rarely require an active response (Basaran, 2018). Natural recovery is typically applied to this type of spilled oil, but the cleanup process may be dangerous to spill responders because of the risk of flammability and toxicity (ECCC, 2016). It would be effective to remove persistent light oils such as diesel (2 mPa s at 15°C) and light crudes (5–50 mPa s at 15°C) mostly due to their low viscosity (Fingas, 2016a), but shoreline cleanup responses to medium oil spills should be conducted as soon as possible to ensure the efficient removal of the oils, otherwise the oil contamination of the shoreline environment will be serious and long term. Vacuum, sorbents, mechanical removal, or the use of surface washing agents may be preferred options to deal with medium oils (ECCC, 2016). Heavy oils, such as heavy crude oils, No. 6 fuel oil, and Bunker C, have consistently shown little or even no evaporation losses and have very high viscosity (Speight, 2015; Fritt-Rasmussen et al., 2018). This group of oils can form a sticky film on shoreline substrates or fur-bearing mammals and have long-term detrimental effects on coastal biota. It is quite difficult to effectively remove this type of oil from shorelines using many routine shoreline cleanup methods, but the application of high pressure/temperature washing, manual/mechanical removal, and surface washing agents may be feasible (Fingas, 2013; Zengel et al., 2015). Sinking oils have higher density than seawater, and slurry oils are a typical example. The spill of slurry oils is extremely rare, but once it has happened, the cleanup will be very difficult with low oil recovery efficiency (Jacketti et al., 2020). The use of shallow-water vacuum or sediment relocation may be selected to manage this type of spill (Piraino et al., 2017). However, it should be noted that the final choice of response method in real shoreline cleanup scenarios still depends on the field conditions and relevant regulations.
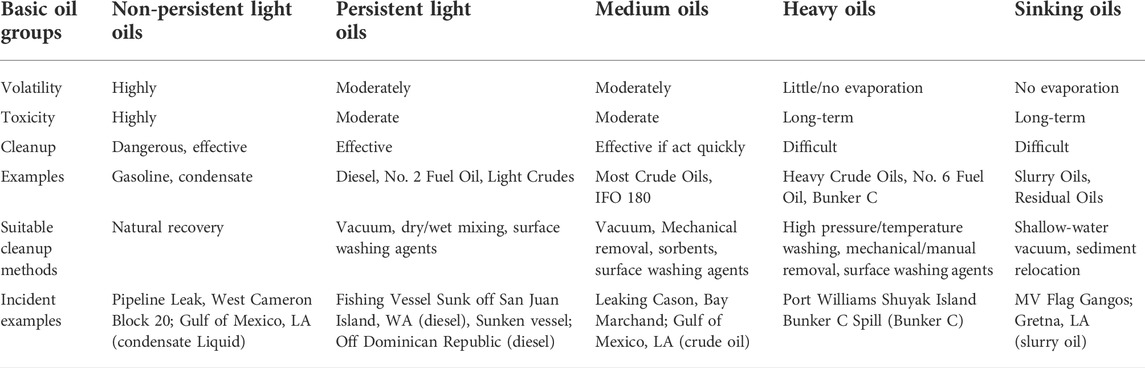
TABLE 1. The properties, oil examples, suitable shoreline cleanup response methods, and incidents examples of different oil groups.
2.2 Components of spilled oil on shorelines
Based on the polarizability and polarity of the spilled oil, they are classified into four fractions: saturates, aromatics, resins, and asphaltenes (Kjemperud, 2013; Wang et al., 2020). Generally, most crude oils, especially light oils, consist of a large proportion of saturates and aromatics (up to over 80 wt%). However, heavy oils have higher resin andasphaltene contents than lighter oils.
2.2.1 Saturates
Typically, saturates are the most abundant fraction of fresh oils. They are hydrocarbons with the maximum number of hydrogens (no double bonds) (Harayama et al., 1999; Fingas, 2014). Alkanes (referred to as paraffins) and cycloalkanes (referred to as naphthenes) are important compounds in the saturate group of oils (Fingas, 2016b). The former has a branched or unbranched chain-like structure with the molecular formula CnH2n+2, while the latter has one or more carbon rings, and its molecular formula is CnH2n (Tissot and Welte, 1984). Alkanes, which have fewer carbon atoms in spilled oil on shoreline substrates, can usually evaporate by themselves during the weathering process and will not remain on a shoreline for a long time. Saturates, particularly those compounds with a smaller molecular weight, including alkanes with 5–30 carbon atoms, are also biodegradable through microorganisms. The saturate fraction of spilled oil will therefore decrease significantly because of evaporation and biodegradation. However, the waxes of saturates resist biodegradation and may form films on the surface of shoreline substrates, which will have effects on the interfacial properties of the spilled oil (Hubbe et al., 2013).
2.2.2 Aromatics
The aromatic fraction of spilled oil has at least one benzene ring, and it is usually less biodegradable than saturates but more toxic to the environment (Anthony, 2006). BTEX (benzene, toluene, ethylbenzene, and xylenes) compounds have monoaromatic hydrocarbons with only one benzene ring, which volatize easily under natural conditions. The monoaromatic component of spilled oil can have toxic effects on microorganisms because it can partition into cell membranes, while the low concentration of monoaromatics can be biodegraded (Lee et al., 2015). The monoaromatics of spilled oil are most mobile in the water phase, so they may easily desorb from shoreline substrates (Salanitro, 2001; Farhadian et al., 2008). Polyaromatic hydrocarbons (PAHs) have at least two benzene rings and thus have higher toxicity, lower volatility, and intermediate biodegradability (Sleight et al., 2020). The content of PAHs with two to four rings in spilled oil can be reduced through the processes of evaporation, dissolution, and photodegradation. Notwithstanding, aromatics with over four rings are very persistent in shoreline environments and can hardly be utilized as substrates for microorganisms; however, they may be degraded through cometabolic transformations (Bartha, 1986).
2.2.3 Resins and asphaltenes
Generally, resins are the smallest polar compounds in spilled oil, but they are not hydrocarbons as their structures contain not only carbon and hydrogen, but also nitrogen, sulfur, and/or oxygen (Harayama et al., 1999). Asphaltenes are chemically similar to resins, but they have the most diversity and greatest molecular weight compared to the other fractions in spilled oil (Barrera et al., 2013; Lewan et al., 2014). Knowledge of resins and asphaltenes is still limited as they are difficult for analysis because of their complex components and structures. Both resins and asphaltenes are persistent in coastal environments and almost not biodegradable due to their extremely low bioavailability (Gallego et al., 2006). Notably, the amount of resins and asphaltenes in spilled oil can greatly affect the oil’s viscosity, with a higher content of the two fractions generating higher viscosity (Luo and Gu, 2007). Weathering processes can increase the weight percentages of resins and asphaltenes in spilled oil and the viscosity of the oil, making the residual oil difficult to remove. Moreover, the content of resins and asphaltenes in spilled oil is able to influence the wetting properties of the surface of the shoreline substrates and sediments, which further increases the difficulties in shoreline cleanup (Lee et al., 2015).
2.3 Adhesion of spilled oil on shoreline matrix
Here, oil adhesion refers to the ability of spilled oil to stick to the surface of shoreline substrates (Lyu et al., 2022). The spilled oil is very adhesive, particularly when it is subject to weathering processes for a long time (Zhang et al., 2019). Once the spilled oil reaches the shore, it contacts with and adheres to the shoreline matrix or other mineral materials. A large amount of oil could therefore strand on a coastal area and adversely impact the marine environment.
As shown in Figure 1, the adhered oil on the shoreline matrix has three major distribution scenarios: adherent surface oil, oil buried in the subsurface, and submerged oil mats (Wang et al., 2020). The pollutant behaviors can be affected by various factors (Yoo et al., 2021). The spilled oil type and composition are primary factors influencing oil adhesion as they are closely related to the physical properties of the stranded oil, including its viscosity, density, and stickiness. Heavier oils are more adhesive to the shoreline matrix than light oils, and weathered oils show more adhesiveness than fresh oils. Moreover, the stranded oil on shoreline substrates usually consists of high ratios of aromatics and asphaltene fractions with a large molecular weight (Konur, 2021). Shoreline characteristics, such as beach slope and substrate types, can also significantly affect spilled oil adhesion (Etkin et al., 2008; Taylor et al., 2021). Shorelines have a wide range of substrate types, which are categorized by the grain size as mud/silt/clay (<0.06 mm), sand (0.06–2 mm), granules (2–4 mm), pebbles (4–64 mm), cobble (64–256 mm), and boulders (>256 mm) (ECCC, 2016). The smaller the shoreline substrate, the greater its surface area, so the more likely spilled oil will be to stick to the shoreline matrix. Other coastal parameters, like the waves, tides, wind, and water flow, also affect oil adhesion. For example, waves and tides may wash away oil that has low adhesiveness and is stuck to the surface of shoreline substrates. Meanwhile, wind can accelerate the aging of adhered oil by evaporating its lighter components and increasing the viscosity and stickiness of the oil, which will result in increased oil adhesion on the shoreline matrix (National Academies of Sciences Medicine, 2016).
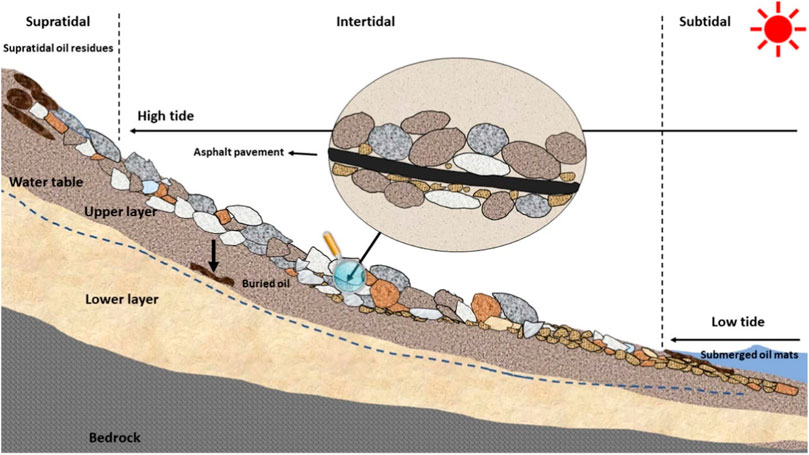
FIGURE 1. The adhered oil on shoreline matrix with three major distribution scenarios (Wang et al., 2020).
3 Effects of oil characteristics on the performance of chemical shoreline response operations
Environmental and pollution control processes involve a series of mechanisms (Huang and Fan, 2021; Rodrigues et al., 2021; Wei et al., 2021). In shoreline cleanup, chemical treatment may provide a good way to remove stranded oil from beach substrates as it has the advantages of a higher oil removal and recovery rate, less involvement of equipment and personnel, and lower cleanup costs compared to other response operations (Fieldhouse, 2008; Chen et al., 2019; Bi et al., 2022a). Specifically, this kind of shoreline response method requires the addition of chemical agents to the stranded oil on the shoreline to change the oil’s physical/chemical properties by, for example, increasing its viscosity, solubilization, and reducing adhesion, thus making it easier for the oil to be removed and/or recovered. SWAs, dispersants, and solidifiers are typical chemical treatment agents for oiled shorelines, but each has different mechanisms (Fingas et al., 1990; Fingas, 2013). SWAs are used to lift adhered oil from substrates but not disperse the oil, while dispersants break the oil up into small droplets to enter the waterbody (Michel et al., 2001). Solidifiers are used to change the physical state of stranded oil from a liquid to a solid form to constrain the re-mobility or spread of the oil (Walker et al., 1999). Oil characteristics have significant effects on the oil removal performance of these agents.
3.1 Chemical treatment for light oils
API gravity is a frequently used indicator in the petroleum industry globally to measure the relative density of petroleum products (Barbosa et al., 2013; Demirbas and Al-Ghamdi, 2015). The API gravity of light crude oils is higher than 31.1°. Light oils have relatively more volatile components and can be treated effectively with chemical methods. For example, Bi et al. (2020) used Hibernia oil with an API gravity of 35.0° in their research to explore oil removal from contaminated sand using a commercial SWA (COREXIT EC9580A) under different environmental conditions. The results showed a higher oil removal performance (up to 79%) with the use of the SWA even though the light oil had already weathered for seven days. When the authors explored the environmental parameter of temperature, they found that a temperature increase could change the stranded oil properties on the sand, resulting in a reduction in the viscosity and adhesion of oil, which contributed to increased oil removal efficiency. Similarly, the rhamnolipid, surfactin, SDS, and Triton X-100 were used as SWAs and their performance evaluated in the recovery of Iranian crude oil with an API gravity of 34.0° from sand (Amani, 2015). Among them, the rhamnolipid attained the highest oil removal performance (80%). However, a high API gravity does not necessarily mean that SWA treatment will be highly effective for oil removal as it is affected by various factors. High wax and asphaltene content can make light oils become difficult to remove and recover because of wax deposition and asphaltenes precipitation (Li et al., 2021). In real oil spill scenarios, it usually takes some time from the occurrence of an oil spill to the time when the spilled oil is cleaned up, so the stranded oil will undergo a series of weathering processes, such as evaporation, emulsification, oxidation, and biodegradation, leaving high percentages of large and recalcitrant components (Ossai et al., 2020; Bi et al., 2022b). It is more difficult to deal with such oiled shorelines by using SWAs even if the oil was originally light crude oil.
3.2 Chemical treatment for petroleum products
Engine oil is one type of synthetic petroleum product and is derived from heavy fractions of crude oils (Yang et al., 2016). Many studies have recently used engine oil as a representative petroleum product to evaluate the oil removal performance of chemical treatments. Chen et al. (2021) explored the removal of Shell Rotella® T4 conventional diesel engine oil from beach sand through the use of cellulose nanocrystal-based nanofluid, and around 75% of the oil was successfully removed under optimal conditions. An increase in temperature led to a decrease in the viscosity of the stranded oil on substrates, which enhanced the performance of the nanofluid. This type of engine oil was very stable with an API gravity of 29.3° and viscosity of 257.08 cP. It showed no weight loss during the weathering process, so its density and viscosity remained the same before and after ageing. These properties of the engine oil enabled the results to be compared between laboratories and studies. Yue et al. (2021) used the same engine oil as the pollution source for shoreline contamination and assessed the performance of a dual responsive nanoclay/sodium alginate washing fluid on oil removal. The results showed that 72.2% of the engine oil could be removed, with mainly temperature and oil concentrations influencing the effectiveness of the washing fluid. Later, other types of washing fluids with pH-responsive phosphoprotein, magnetite nanoparticles, and surfactant-modified nanoclay were further developed to investigate the engine oil removal from shoreline substrates under various environmental conditions (Bi et al., 2021a; Yue et al., 2022a; Yue et al., 2022b). These washing fluids under different conditions can affect oil characteristics (e.g., viscosity, adhesion, mobility, solubilization) to varying degrees and thus have diverse effects on the oil removal performance of the chemical treatment. In the study of Tumeo and Cote (1998), diesel heating oil #1 was selected as the contaminant for porous shoreline substrates. A series of SWAs (PES-51, Corexit 9580, and Grancontrol “O”) were used to conduct column tests on the oiled beach substrates, and the results demonstrated a significant decrease in oil removal effectiveness when the temperature of the solution changed from 20 to 10 °C, partly due to the increased oil viscosity.
3.3 Chemical treatment for heavy oils
The API gravity range of heavy oils is between 10 and 22.3°, and typical examples of these oils include Bunker C fuel oil with 11° API, Cold Lake Blend with 23° API, and Western Canadian Select with 19–22° API (Lee et al., 2015; Suhag et al., 2017). Generally, heavy oils have high viscosity and specific density, high contents of asphaltene and resin fractions, and low H/C ratio (Santos et al., 2014). According to previous research, the use of SWAs is the preferrable method for treating heavy oils stranded on shoreline substrates because the performance of SWAs on heavy oil removal in laboratory, meso-scale, and even field tests has been investigated extensively. In a laboratory setting, Clayton et al. (1995) contaminated different natural substrates, including gravel, rip-rap, and eelgrass, with Bunker C and used Corexit 9580 to remove the stranded oil with an efficacy of 52.9, 21.1, and 23.6%, respectively. More recently, Aquaclean was selected to remove adhered IFO 180 from the surfaces of substrates at 22 °C, and the results showed that the oil removal efficiencies were 38.66% for gravel and 19.09% for sand (Luedeker, 2009). However, the performance of the SWA reduced dramatically to 0.67% for the sand substrates when the temperature was decreased to 4°C because the low temperature significantly affected the characteristics of the adhered oil and the oil removal efficiency of the SWA. In a meso-scale test by Taylor et al. (2014), weathered Cold Lake winter Blend was effectively removed from a granite tile using Corexit 9580. The researchers also found that the time after the weathered oil was applied to the tile was more important than the time the weathered oil remained on the water. In terms of field tests, Bunker C has often been used as the stranded oil on shoreline substrates to evaluate the effectiveness of SWAs (Michel and Benggio, 1995; Pezeshki et al., 1995; Fingas, 2000), but the results of these early field tests were not quantified, and they were only declared to be successful. In general, the use of SWAs is suitable for the removal of heavier oils from shoreline substrates, but its effectiveness can be affected by various factors, such as the oil type and characteristics, temperature, and substrate type.
4 Effects of oil characteristics on the performance of physical shoreline response operations
Physical cleanup and recovery are the most commonly used methods to deal with oiled shorelines. Common physical response operations include manual removal, vacuums, mechanical removal, and sorbents (Feng et al., 2021). Oil properties can significantly affect the performance of physical cleanup. The selection of physical methods therefore requires consideration of this factor. Large numbers of personnel are required for manual cleanup, so this method is typically used only for areas where large machinery cannot reach or be operated (Chen et al., 2019). In addition, with regard to oil characteristics, the manual cleanup is more suitable for small amounts of viscous oil. Sorbents can be used to clean up tiny amounts of spilled oil on a variety of substrates; however, they are ineffective at cleaning up solid oil and less successful at cleaning up heavy viscous oils than they are at cleaning up medium crudes (ECCC, 2016). When used to cure tiny quantities of oil, they need to be replaced often. Vacuuming systems can be used to collect various types of oils and even oiled debris. Vacuum technology collects everything from oiled vegetation to oiled gravel and rocks, but it is not safe for volatile oils. However, using vacuums and pumps for heavy oil is often unsuccessful in the previous attempt, especially at low temperatures or after weathering (Ansell et al., 2001).
The effect of oil properties on the performance of physical cleanup has been observed in previous oil spill responses. Oil slicks with high viscosity can be easily removed and collected through physical recovery due to their low mobility, as was the case in the “Kurdistan” oil spill response. Cleanup teams comprising 500 people using rakes, shovels, and forks removed the Bunker C oil as it reached the shore (Duerden and Swiss, 1981). The waste volumes generated from an oil spill cleanup are a crucial factor in determining the oil spill response operation; the oil viscosity can significantly affect the waste volumes generated through physical response operations. Physical removal, including sorbents, high-pressure heated water, and manual removal, has been applied to remove viscous oil in “Arrow” Bunker C oil spills. A valuable finding in this case is that detailed work documents have provided data on the waste generated by physical removal methods on a beach-by-beach basis (Secretariat and Island, 2009). Compared with the data collected from other oil spill responses, oil with a higher viscosity can result in more waste being generated from physical removal, including washing recovery and manual and mechanical removal (Secretariat and Island, 2009). Statistics based on past oil spill response costs also show that cleanup costs for heavy oil can be significantly higher than those of light oil due to their widespread contamination (Moller et al., 1987).
In general, previous experience with oil spill responses suggests that the key factor affecting the effectiveness and cost of physical cleanup can be viscosity. Heavy oil is more easily stranded on shoreline surfaces and difficult to remove through natural recovery, so physical cleanup and removal can be one option to deal with the problem. However, physical response methods are limited for shorelines affected by heavy oils due to their high viscosity. Some physical methods, such as sorbents and vacuum systems, cannot achieve the desired performance for heavy oils. Viscous heavy oils have shown lower penetration and higher retention than light oils in various beach settings, as shown in Table 2, but their high viscosity and persistence make the physical cleanup of heavy oils expensive, and large amounts of waste are generated (Etkin et al., 2007).
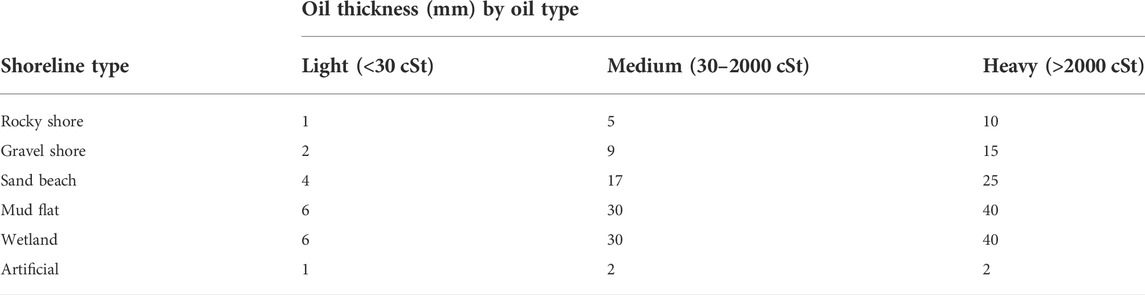
TABLE 2. Maximum surface oil thicknesses for various beach types as function of oil viscosity (Etkin et al., 2007).
5 Effects of oil characteristics on the performance of biological shoreline response operations
To date, mechanical and labor-intensive approaches have been used for shoreline cleanup because of their simplicity (Broman et al., 1983). However, some drawbacks have been observed when applying these techniques. For instance, the application of high pressure may undermine bacterial communities. Moreover, these techniques are only capable of partially decontaminating shorelines. Environmentally benign remediation is thus urgently needed.
Bioremediation can accelerate the natural oil biodegradation process through supplementation with sufficient nutrients and oxygen (Hoff, 1993). More attention has been paid to bioremediation since the 1980’s when the Exxon Valdez oil spill occurred (Hoff, 1993; Bragg et al., 1994). Three techniques are involved in oil spill bioremediation in shoreline, namely, bioaugmentation agents, bioenhancement agents, and phytoremediation (ECCC, 2016). Bioaugmentation is a process in which high-activity bacteria are added to enhance the performance of microorganism populations (Abdulsalam et al., 2011). The physiology and metabolic ability of these microorganisms are of the essence when choosing optimal microorganisms (Boopathy, 2000). The bioenhancement process can stimulate the reproduction of oil-degrading microorganisms and boost the oil biodegradation rate by adjusting some environmental parameters, such as biosurfactants and fertilizers (Prince et al., 2003). Phytoremediation is to accelerate oil degradation with the use of fungi and plants (Yavari et al., 2015).
Bioremediation has proved to be a successful strategy in oil-contaminated shoreline protection. These techniques enable oil biodegradation without having an adverse impact on the environment. Significantly, bioremediation is widely accepted because of its esthetic and cost-effective properties. However, bioremediation requires long-term treatment, even up to years, to achieve great removal efficiency (Margesin et al., 2007).
5.1 Biological treatment for light crude oils
Due to the high percentage of low molecular weight hydrocarbon components, light crude oil has low viscosity and low specific gravity, which enables it to flow freely at a natural ambient temperature (Figure 2A). This increases the risk of shoreline contamination by light crude oil. A field study of light crude oil biodegradation was undertaken in Delaware by Venosa et al. (1996), who aimed to accurately determine whether inorganic minerals and/or microbial inoculation enhanced the bioremediation of light crude oil on a sandy beach. The findings revealed the natural biodegradation of light petroleum if sufficient nutrients are provided. However, the application of microorganisms and nutrients relies on their background concentration at the contaminated shoreline. In addition, the researchers found that light oil biodegradation was more effective in the upper intertidal zone than in the lower zones. It is worth noting that nutrients cannot guarantee effective petroleum biodegradation as the shoreline morphology also matters. Sakaya et al. (2019) evaluated the bioremediation ability of light Arabian crude oil under different coastal conditions over a 42-day period. The results demonstrated that the overall removal of alkanes and PAHs was significantly enhanced under biosimulation at 18 and 28°C, while almost no improvement was observed in sediments with plenty of nutrients. This is because the adsorption/desorption cycle in the sediments makes nutrients less bioavailable to active marine microbial communities (Liang et al., 2013). Moreover, fine droplets of oil dispersed in seawater can increase the oil surface area for the attachment of microorganisms, thus strengthening the biodegradation rate (Daffonchio et al., 2012).
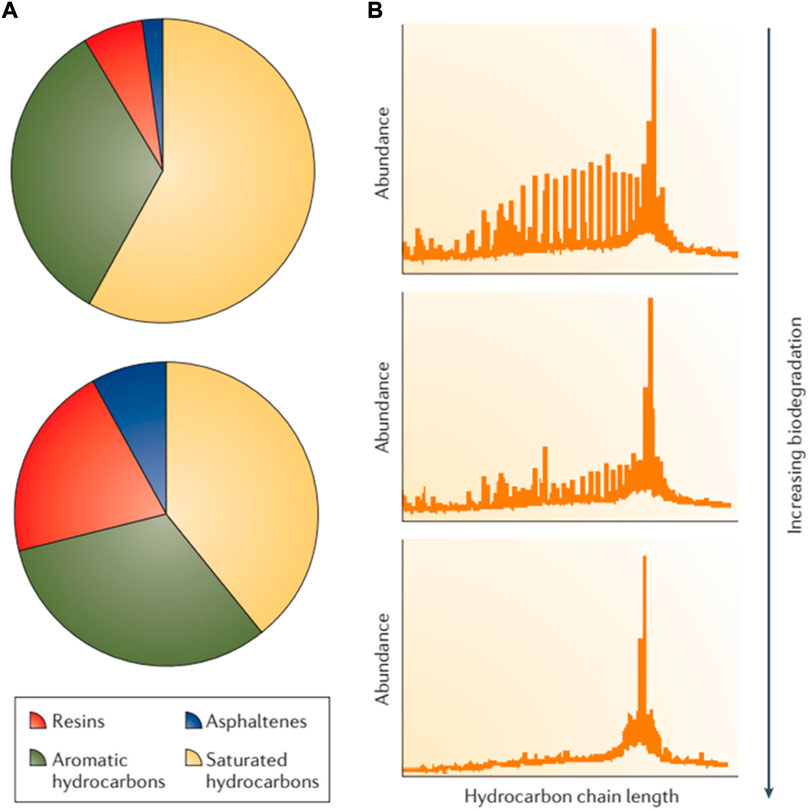
FIGURE 2. Effect of biodegradation on oil composition. (A) Component of light crude oil and slightly biodegraded heavy crude oil, and (B) the components of oil that is increasingly biodegraded (Head et al., 2006).
Many studies have focused on the bioremediation of shorelines post-petroleum contamination. However, less attention has been paid to the response of microorganisms from oiled shorelines to new oil-affected beaches. Reis et al. (2014) therefore assessed the bioremediation ability of microorganisms from a sandy beach already contaminated by oil spills. Their findings proved that Autochthonous, Pseudomonas, Actinomycetales, and Betaproteobacteria are capable of degrading new oil contaminants by increasing their abundance and can remove up to 85% of total petroleum hydrocarbons and 70% of polycyclic aromatic hydrocarbons. Rosa and Triguis (2007) turned their attention to tropical shorelines. A cheap fertilizer (NPK) was found to accelerate the biodegradation process. The subsurface samples were more efficiently degraded than the surface samples, possibly owing to the high humidity of the former. The analysis results suggested that biodegradation is not strong enough to degrade aromatic compounds. Significantly, the fertilizer had a non-toxic effect on the shoreline, which guarantees the applicability of the technique.
5.2 Biological treatment for heavy crude oils
Heavy oil has high viscosity and is high in polar chemicals, resins, and asphaltenes (Figure 2A). Becker et al. (2016) used the fertilizer Osmocote to stimulate oil biodegradation in superficial beach sediments and subsurface sediments. The biostimulation was still sufficient after 92 days of the experiment, but the biodegradation behavior of the hydrocarbon was different. The alkanes were degraded to trace content after 28 days, whereas the phytane and pristane were degraded between 28 and 42 days. In this study, the Osmocote proved an efficient biostimulator for native microorganisms.
Considerable crude oil was released and the seashore severely contaminated as a result of the Qingdao oil pipeline explosion in 2013. To deal with this, Gao et al. (2019) collected sediment from the shoreline and studied the biodegradation differences between the fresh and weathered oil-contaminated samples. The isolation of different strains was dependent on the succession of the sampled bacterial community. Five types of microorganisms displayed high degradation ability for alkanes. Moreover, the sequencing analysis revealed that the strain E4 was dominant in the oil degradation.
Biodegradation as a natural process may proceed slowly depending on the type of oil (i.e., light crude oils degrade faster than heavier oils). Hoff et al. (1995) demonstrated that heavy fuel oils, such as Bunker C (No. 6 fuel oil), are considerably less degradable than light crude oils or diesel fuels when exposed to similar initial concentrations of nutrients and hydrocarbon-degrading bacteria. This may be because of the numerous variations in the aromatic and alkane content of the various oils. It may further explain why significant biodegradation was not confirmed in the case of the 1990 Apex Barges spill in Galveston Bay, Texas. More effort is therefore needed to classify which petroleum products are degradable in the sense of response and restoration.
In general, alkanes and simple aromatics have the fastest biodegradation rates, followed by branched alkanes, alkylated aromatics, and cyclic alkanes. Finally, hopane and stigmastaneare most resistant to biodegradation, which allows them to be used as conserved biomarkers to quantify biodegradation. Typically, 85% of hydrocarbons are removed under optimal conditions within a month or two (Haritash and Kaushik, 2009). Rather less is known about the biodegradation of resins and asphaltenes, whether initially in the oil or generated by photooxidation, although some of them have proved to be biodegradable (Aeppli et al., 2022).
Head et al. (2006) collected data from studies and showed that saturated hydrocarbons are degraded quickly, whereas other hydrocarbons like aromatic ones take longer (Figure 2B), which indicates that the mechanisms involved in saturated hydrocarbon and PAH degradation are different. Saturated hydrocarbon can be biodegraded through direct metabolism (Cutright and Lee, 1994). Regarding aromatic hydrocarbons, differences were also observed between lower and higher weight PAHs. The lower weight PAHs (two or three rings) had a higher degradation rate than the higher weight compounds (four or more rings) (Cerniglia, 1993). Nevertheless, this degradation scale varied for the original concentrations of PAHs.
6 Conclusions and recommendations for future research
The characteristics of spilled oil on shorelines can significantly affect the performance of shoreline response operations. This review systematically investigated the spilled oil from the perspectives of oil types, composition, and adhesion. Five basic groups of the spilled oil, including non-persistent light oils, persistent light oils, medium oils, heavy oils, and sinking oils, were firstly discussed, and four oil components (saturates, aromatics, resins, and asphaltenes) were analysed. Then, three distribution scenarios of adhered oil on shorelines, including adherent surface oil, oil buried in the subsurface, and submerged oil mats, were reviewed. More importantly, the effects of oil characteristics, such as oil types, viscosity, evaporation, as well as composition, on the performance of chemical treatments, physical methods, and biodegradation were discussed in detail. This review has significant implications for future decision-making and the implementation of shoreline cleanup in real cases.
Further research is still required to develop comprehensive shoreline response plans and effective cleanup operations. The corresponding recommendations are as follows. First, it is vitally important to develop technologies for spill identification and detection as well as monitoring and modeling so that timely response countermeasures can be taken. The ideal situation would be that the spilled oil will not be transported to the shore, that could even be considered the performance of shoreline cleanup is “100%“. Second, response operations should be conducted as soon as possible once spilled oil has been stranded on a shoreline because the stranded oil will undergo weathering processes, such as evaporation, photooxidation, and sedimentation, and further increase its adhesion to substrate surfaces, thus reducing response effectiveness. Therefore, it is also suggested to focus on the adhesiveness of spilled oil on shoreline for future research which little work has been done but may greatly affect what kinds of response operations will be used. Third, chemical treatment agents are expected to be with less toxic effects on coastal environmental but have high oil removal efficiency. It would be desirable to reduce the treatment cost and involvement of personnel and equipment for physical response techniques. How to shorten the biological treatment time but ensure its effectiveness is very important for biodegradation in shoreline cleanup. Meanwhile, phytoremediation deserves more attention because it has great potential for restoration of contaminated shorelines. Fourth, a decision framework that takes into consideration the stranded oil type, amount, thickness, weathering degree, viscosity, composition, toxicity, and other oil characteristics needs to be constructed in order to fully understand oil behaviors on shorelines. A corresponding response tool set could then be developed for the relevant organizations and spill responders’ reference. Last, full investigations into the effects of other site conditions (e.g., shoreline types, intertidal zones, tides/waves/currents) and operational factors (e.g., equipment, personnel, waste generation, costs) on the overall performance of shoreline cleanup are also essential to the final response plans and decisions.
Author contributions
This article is the combined effort of all the authors. XC: Conceptualization, data collection, and writing the original manuscript. HB, RY, and ZC: Writing the original manuscript and made corrections. CA: Writing, review, editing, and administration.
Funding
This research was funded by the Multi-partner Research Initiative of Fisheries and Oceans Canada (MPRI 1.09 and 2.01), Fonds de recherche du Quebec-Nature et technologies (2022-NC-299705), the Natural Sciences and Engineering Research Council of Canada (RGPIN-2022-04688), and the China Scholarship Council (202208880011).
Conflict of interest
The authors declare that the research was conducted in the absence of any commercial or financial relationships that could be construed as a potential conflict of interest.
Publisher’s note
All claims expressed in this article are solely those of the authors and do not necessarily represent those of their affiliated organizations, or those of the publisher, the editors and the reviewers. Any product that may be evaluated in this article, or claim that may be made by its manufacturer, is not guaranteed or endorsed by the publisher.
References
Abdulsalam, S., Bugaje, I., Adefila, S., and Ibrahim, S. (2011). Comparison of biostimulation and bioaugmentation for remediation of soil contaminated with spent motor oil. Int. J. Environ. Sci. Technol. 8, 187–194. doi:10.1007/bf03326208
Aeppli, C., Mitchell, D. A., Keyes, P., Beirne, E. C., McFarlin, K. M., Roman-Hubers, A. T., et al. (2022). Oil irradiation experiments document changes in oil properties, molecular composition, and dispersant effectiveness associated with oil photo-oxidation. Environ. Sci. Technol. 56, 7789–7799. doi:10.1021/acs.est.1c06149
Amani, H. (2015). Evaluation of biosurfactants and surfactants for crude oil contaminated sand washing. Pet. Sci. Technol. 33, 510–519. doi:10.1080/10916466.2014.999941
Ansell, D., Dicks, B., Guenette, C., Moller, T., Santner, R., and White, I. (2001). “A review of the problems posed by spills of heavy fuel oils,” in Proceedings of International oil spill conference (Tampa, Florida: American Petroleum Institute), 591–596.
Anthony, I. O. (2006). Biodegradation alternative in the cleanup of petroleum hydrocarbon pollutants. Biotechnol. Mol. Biol. Rev. 1, 38–50. doi:10.5897/BMBR.9000002
Barbosa, L. L., Kock, F. v. V., Silva, R. C., Freitas, J. C., Lacerda, V., and Castro, E. q. V. (2013). Application of low-field NMR for the determination of physical properties of petroleum fractions. Energy fuels. 27, 673–679. doi:10.1021/ef301588r
Barrera, D., Ortiz, D., and Yarranton, H. (2013). Molecular weight and density distributions of asphaltenes from crude oils. Energy fuels. 27, 2474–2487. doi:10.1021/ef400142v
Bartha, R. (1986). Biotechnology of petroleum pollutant biodegradation. Microb. Ecol. 12, 155–172. doi:10.1007/bf02153231
Basaran, I. K. (2018). Can the civil liability convention for oil pollution withstand the pressure of a major oil spill in the arctic ocean? Arct. Yearb. 2018, 293.
Becker, B. R., de Souza, E. S., Martins, R. L., and da Luz Bueno, J. (2016). Bioremediation of oil‐contaminated beach and restinga sediments using a slow‐release fertilizer. Clean. Soil Air Water 44, 1154–1162. doi:10.1002/clen.201500023
Berke, S., Dorgan, K., Kiskaddon, E., Bell, S., Gadeken, K., Clemo, W. C., et al. (2022). Shallow infaunal responses to the deepwater horizon event: Implications for studying future oil spills. Front. Environ. Sci. 1179, 950458. doi:10.3389/fenvs.2022.950458
Bi, H., An, C., Chen, X., Owens, E. H., and Lee, K. (2020). Investigation into the oil removal from sand using a surface washing agent under different environmental conditions. J. Environ. Manage. 275, 111232. doi:10.1016/j.jenvman.2020.111232
Bi, H., An, C., Mulligan, C. N., Chen, Z., Lee, K., Wen, J., et al. (2022a). Application of phase-selective organogelators (PSOGs) for marine oil spill remediation. J. Mar. Sci. Eng. 10, 1111. doi:10.3390/jmse10081111
Bi, H., An, C., Mulligan, C. N., Zhang, K., Lee, K., and Yue, R. (2021a). Treatment of oiled beach sand using a green and responsive washing fluid with nonionic surfactant-modified nanoclay. J. Clean. Prod. 333, 130122. doi:10.1016/j.jclepro.2021.130122
Bi, H., An, C., Owens, E., Lee, K., Chen, Z., Mulligan, C. N., et al. (2021b). A framework for the evaluation and selection of shoreline surface washing agents in oil spill response. J. Environ. Manage. 287, 112346. doi:10.1016/j.jenvman.2021.112346
Bi, H., Mulligan, C. N., An, C., Owens, E., Taylor, E., McCourt, J., et al. (2022b12922). Development of a calcium alginate-cellulose nanocrystal-based coating to reduce the impact of oil spills on shorelines. J. Hazard. Mat. 436, 129228. doi:10.1016/j.jhazmat.2022.129228
Boopathy, R. (2000). Factors limiting bioremediation technologies. Bioresour. Technol. 74, 63–67. doi:10.1016/s0960-8524(99)00144-3
Boufadel, M., Geng, X., An, C., Owens, E., Chen, Z., Lee, K., et al. (2019). A review on the factors affecting the deposition, retention, and biodegradation of oil stranded on beaches and guidelines for designing laboratory experiments. Curr. Pollut. Rep. 5, 407–423. doi:10.1007/s40726-019-00129-0
Bragg, J. R., Prince, R. C., Harner, E. J., and Atlas, R. M. (1994). Effectiveness of bioremediation for the Exxon Valdez oil spill. Nature 368, 413–418. doi:10.1038/368413a0
Broman, D., Ganning, B., and Lindblad, C. (1983). Effects of high pressure, hot water shore cleaning after oil spills on shore ecosystems in the Northern Baltic proper. Mar. Environ. Res. 10, 173–187. doi:10.1016/0141-1136(83)90009-0
Cerniglia, C. E. (1993). Biodegradation of polycyclic aromatic hydrocarbons. Curr. Opin. Biotechnol. 4, 331–338. doi:10.1016/0958-1669(93)90104-5
Chen, B., Ye, X., Zhang, B., Jing, L., and Lee, K. (2019a). Marine oil spills—preparedness and countermeasures, world seas: An environmental evaluation. Elsevier, 407–426.
Chen, G., Lin, J., Hu, W., Cheng, C., Gu, X., Du, W., et al. (2018). Characteristics of a crude oil composition and its in situ waxing inhibition behavior. Fuel 218, 213–217. doi:10.1016/j.fuel.2017.12.116
Chen, Z. K., An, C., Boufadel, M., Owens, E. H., Chen, Z., Lee, K., et al. (2019b). Use of surface-washing agents for the treatment of oiled shorelines: Research advancements, technical applications and future challenges. Chem. Eng. J. 391, 123565. doi:10.1016/j.cej.2019.123565
Chen, Z. K., An, C., Yin, J., Owens, E. H., Lee, K., Zhang, K., et al. (2021). Exploring the use of cellulose nanocrystal as surface-washing agent for oiled shoreline cleanup. J. Hazard. Mat. 402, 123464. doi:10.1016/j.jhazmat.2020.123464
Clayton, J. R., Stransky, B. C., Schwartz, M. J., Lees, D. C., Michel, J., Snyder, B. J., et al. (1995). Development of protocols for testing cleaning effectiveness and toxicity of shoreline cleaning agents in the field. Washington, DC: Marine Spill Response Corporation.
Cutright, T., and Lee, S. (1994). Microorganisms and metabolic pathways for remediation of PAH contaminated soil. Fresenius Environ. Bull. 3, 413–421.
Daffonchio, D., Mapelli, F., Cherif, A., Malkawi, H. I., Yakimov, M. M., Abdel-Fattah, Y. R., et al. (2012). ULIXES, unravelling and exploiting Mediterranean Sea microbial diversity and ecology for xenobiotics’ and pollutants’ clean up. Rev. Environ. Sci. Biotechnol. 11, 207–211. doi:10.1007/s11157-012-9283-x
Demirbas, A., and Al-Ghamdi, K. (2015). Relationships between specific gravities and higher heating values of petroleum components. Pet. Sci. Technol. 33, 732–740. doi:10.1080/10916466.2015.1007384
Díez, S., Jover, E., Bayona, J. M., and Albaigés, J. (2007). Prestige oil spill. III. Fate of a heavy oil in the marine environment. Environ. Sci. Technol. 41, 3075–3082. doi:10.1021/es0629559
Duerden, F. C., and Swiss, J. J. (1981). “Kurdistan-An unusual spill successfully handled,” in Proceedings of 1981 International Oil Spill Conference (Atlanta, Georgia: American Petroleum Institute), 215–219.
ECCC (2016). A field guide to oil spill response on marine shorelines. Ottawa, ON: Environment and Climate Change Canada.
Etkin, D., French-McCay, D., and Michel, J. (2007). Review of the state-of-the-art on modeling interactions between spilled oil and shorelines for the development of algorithms for oil spill risk analysis modeling. NY: US Department of the Interior Minerals Management Services & Environmental Research Consulting, Cortlandt Manor.
Etkin, D. S., Michel, J., McCay, D. F., Boufadel, M., and Li, H. (2008). “Integrating state-of-the-art shoreline interaction knowledge into spill modeling,” in Proceedings of 2008 International Oil Spill Conference (Savannah, Georgia: American Petroleum Institute), 915–922.
Farhadian, M., Duchez, D., Vachelard, C., and Larroche, C. (2008). Monoaromatics removal from polluted water through bioreactors—a review. Water Res. 42, 1325–1341. doi:10.1016/j.watres.2007.10.021
Feng, Q., An, C., Cao, Y., Chen, Z., Owens, E., Taylor, E., et al. (2021). An analysis of selected oil spill case studies on the shorelines of Canada. J. Environ. Inf. Lett. 5, 39–47. doi:10.3808/jei.202100052
Fieldhouse, B. (2008). “Dispersion characteristics of oil treated with surface washing agents for shoreline cleanup,” in Proceedings of the thirty-first AMOP technical seminar on environmental contamination and response (Calgary, AB: Environment Canada), 373–394.
Fingas, M. (2014). Introduction to oil chemistry and properties. Handb. oil spill Sci. Technol. 51, 77. doi:10.1002/9781118989982.ch3
Fingas, M. (2016b). “Oil spills and response,” in Springer handbook of ocean engineering. Editors M. Dhanak, and N. Xiros (Springer, Cham: Springer International Publishing), 1067–1094.
Fingas, M., Stoodley, R., and Laroche, N. (1990). Effectiveness testing of spill-treating agents. Oil Chem. Pollut. 7, 337–348. doi:10.1016/s0269-8579(05)80048-6
Fingas, M. (2013). Surface-washing agents: An update. Prince William Sound Regional Citizens' Advisory Council.
Fingas, M. (2000). “Use of surfactants for environmental applications,” in Surfactants: Fundamentals and applications to the petroleum industry. Editor L. L. Schramm (Cambridge: Cambridge University Press), 461–539.
Fritt-Rasmussen, J., Wegeberg, S., Gustavson, K., Sørheim, K. R., Daling, P. S., Jørgensen, K., et al. (2018). Heavy fuel oil (HFO): A review of fate and behaviour of HFO spills in cold seawater, including biodegradation. Environmental effects and oil spill response.
Gallego, J., González-Rojas, E., Peláez, A., Sánchez, J., García-Martínez, M. J., Ortiz, J., et al. (2006). Natural attenuation and bioremediation of Prestige fuel oil along the Atlantic coast of Galicia (Spain). Org. Geochem. 37, 1869–1884. doi:10.1016/j.orggeochem.2006.07.022
Gao, W., Gao, X., Mi, T., Han, B., Zhang, Y., Li, X., et al. (2019). Degradation potential and diversity of oil-degrading bacteria isolated from the sediments of the Jiaozhou Bay, China. Acta Oceanol. Sin. 38, 54–64. doi:10.1007/s13131-019-1353-2
Harayama, S., Kishira, H., Kasai, Y., and Shutsubo, K. (1999). Petroleum biodegradation in marine environments. J. Mol. Microbiol. Biotechnol. 1, 63–70.
Haritash, A., and Kaushik, C. (2009). Biodegradation aspects of polycyclic aromatic hydrocarbons (PAHs): A review. J. Hazard. Mat. 169, 1–15. doi:10.1016/j.jhazmat.2009.03.137
Head, I. M., Jones, D. M., and Röling, W. F. (2006). Marine microorganisms make a meal of oil. Nat. Rev. Microbiol. 4, 173–182. doi:10.1038/nrmicro1348
Hoff, R., Blenkinsopp, S., Sergy, G., Wang, Z., Henry, C., Roberts, P., et al. (1995). Evaluating biodegradation potential of various oils. Canada: Web.
Hoff, R. Z. (1993). Bioremediation: An overview of its development and use for oil spill cleanup. Mar. Pollut. Bull. 26, 476–481. doi:10.1016/0025-326x(93)90463-t
Hosseinipooya, S. A., Hu, G., Lee, K., Li, J., Ng, K. T. W., and Vu, H. L. (2022). A system dynamics modeling approach for estimation of oily waste generation from marine oil spill response: A case study of an oil spill in central coast of British Columbia. Front. Environ. Sci. 10, 902229. doi:10.3389/fenvs.2022.902229
Huang, K., and Fan, Y. (2021). Parameter uncertainty and sensitivity evaluation of copula-based multivariate hydroclimatic risk assessment. J. Environ. Inf. 38, 131–144.
Hubbe, M. A., Rojas, O. J., Fingas, M., and Gupta, B. S. (2013). Cellulosic substrates for removal of pollutants from aqueous systems: A review. 3. Spilled oil and emulsified organic liquids. BioResources 8, 3038–3097. doi:10.15376/biores.8.2.3038-3097
Huettel, M. (2022). Oil pollution of beaches. Curr. Opin. Chem. Eng. 36, 100803. doi:10.1016/j.coche.2022.100803
Jacketti, M., Beegle-Krause, C., and Englehardt, J. D. (2020). A review on the sinking mechanisms for oil and successful response technologies. Mar. Pollut. Bull. 160, 111626. doi:10.1016/j.marpolbul.2020.111626
Kjemperud, J. (2013). Interaction of different crude oils with model shoreline surfaces: Adsorption and wettability studies Master's thesis. Norwegian University of Science and Technology.
Konur, O. (2021). Biodiesel and petrodiesel fuels: Science, technology, health, and the environment, biodiesel fuels. CRC Press, 3–36.
Lee, K., Boufadel, M., Chen, B., Foght, J., Hodson, P., Swanson, S., et al. (2015). Expert panel report on the behaviour and environmental impacts of crude oil released into aqueous environments. Ottawa, ON: Royal Society of Canada.
Lewan, M., Warden, A., Dias, R., Lowry, Z., Hannah, T., Lillis, P., et al. (2014). Asphaltene content and composition as a measure of Deepwater Horizon oil spill losses within the first 80 days. Org. Geochem. 75, 54–60. doi:10.1016/j.orggeochem.2014.06.004
Li, X., Lu, S., Niu, M., Cheng, R., Gong, Y., and Xu, J. (2021). Asphaltene inhibition and flow improvement of crude oil with a high content of asphaltene and wax by polymers bearing ultra-long side chain. Energies 14, 8243. doi:10.3390/en14248243
Liang, D., Wang, X., Bockelmann-Evans, B. N., and Falconer, R. A. (2013). Study on nutrient distribution and interaction with sediments in a macro-tidal estuary. Adv. Water Resour. 52, 207–220. doi:10.1016/j.advwatres.2012.11.015
Lin, Q., Mendelssohn, I. A., Graham, S. A., Hou, A., Fleeger, J. W., and Deis, D. R. (2016). Response of salt marshes to oiling from the Deepwater Horizon spill: Implications for plant growth, soil surface-erosion, and shoreline stability. Sci. Total Environ. 557, 369–377. doi:10.1016/j.scitotenv.2016.03.049
Luedeker, C. C. (2009). Development of a surface washing agent protocol: Effect of temperature and salinity on the recovery of heavy weight oil from substrate Doctoral dissertation. University of Cincinnati.
Luo, P., and Gu, Y. (2007). Effects of asphaltene content on the heavy oil viscosity at different temperatures. Fuel 86, 1069–1078. doi:10.1016/j.fuel.2006.10.017
Lyu, X., and Fan, Y. (2021). Characterizing impact factors on the performance of data assimilation for hydroclimatic predictions through multilevel factorial analysis. J. Environ. Inf. 38, 68–82.
Lyu, Y., Huang, Q., Zhang, F., Liu, L., Zhang, H., Zhang, Y., et al. (2022). Study on adhesion of heavy oil/brine/substrate system under shear flow condition. J. Pet. Sci. Eng. 208, 109225. doi:10.1016/j.petrol.2021.109225
Margesin, R., Hämmerle, M., and Tscherko, D. (2007). Microbial activity and community composition during bioremediation of diesel-oil-contaminated soil: Effects of hydrocarbon concentration, fertilizers, and incubation time. Microb. Ecol. 53, 259–269. doi:10.1007/s00248-006-9136-7
Mayes, R., and Myers, J. (2014). Petroleum, quantitative Reasoning in the Context of Energy and environment. Rotterdam: SensePublishers, 237–317.
McClenachan, G., Turner, R. E., and Tweel, A. W. (2013). Effects of oil on the rate and trajectory of Louisiana marsh shoreline erosion. Environ. Res. Lett. 8, 044030. doi:10.1088/1748-9326/8/4/044030
Mehanna, A. K., Hegazy, E., Omar, M., Hassan, A., Elkilani, H., and Turki, E. (2013). Modelling of oil spill impacts on shoreline in Egypt, developments in maritime transportation and exploitation of sea resources–IMAM 2013 conference. Spain: A Coruña, 14–17.
Michel, J., and Benggio, B. L. (1995). Testing and use of shoreline cleaning agents during the Morris J. Berman oil spill, International Oil Spill Conference. Long Beach, CA: American Petroleum Institute.
Michel, J., and Fingas, M. (2016). Oil spills: Causes, consequences, prevention, and countermeasures, fossil fuels: Current Status and future directions. Singapore: World Scientific, 159–201.
Michel, J., Walker, A. H., Scholz, D., and Boyd, J. (2001). “Surface-washing agents: Product evaluations, case histories, and guidelines for use in marine and freshwater habitats,” in International oil spill conference (Tampa, FL: American Petroleum Institute).
Moller, T., Parker, H., and Nichols, J. (1987). Comparative costs of oil spill cleanup techniques, International Oil Spill Conference. American Petroleum Institute, 123–127.
National Academies of Sciences Medicine (2016). Spills of diluted bitumen from pipelines: A comparative study of environmental fate, effects, and response. Washington: National Academies Press.
NOAA (2020). Oil types. Retrieved from: https://response.restoration.noaa.gov/oil-and-chemical-spills/oil-spills/oil-types.html.
Ossai, I. C., Ahmed, A., Hassan, A., and Hamid, F. S. (2020). Remediation of soil and water contaminated with petroleum hydrocarbon: A review. Environ. Technol. Innov. 17, 100526. doi:10.1016/j.eti.2019.100526
Owens, E. H. (2011). “Shoreline countermeasures,” in Oil spill science and technology. Editor M. F. Fingas (Boston: Gulf Professional Publishing), 907–921.
Pezeshki, S., DeLaune, R., Nyman, J., Lessard, R., and Canevari, G. (1995). Removing oil and saving oiled marsh grass using a shoreline cleaner, International Oil Spill Conference. Long Beach, CA: American Petroleum Institute.
Piraino, E., Owens, E., Rios, J., and Graham, A. (2017). “Oil behaviour and the response to a sunken oil spill of slurry in Quintero Bay, Chile,” in Proceedings of 2017 International Oil Spill Conference (Long Beach, California: IOSC), 124–133.
Prendergast, D. P., and Gschwend, P. M. (2014). Assessing the performance and cost of oil spill remediation technologies. J. Clean. Prod. 78, 233–242. doi:10.1016/j.jclepro.2014.04.054
Prince, R. C., Bare, R. E., Garrett, R. M., Grossman, M. J., Haith, C. E., Keim, L. G., et al. (2003). Bioremediation of stranded oil on an Arctic shoreline. Spill Sci. Technol. Bull. 8, 303–312. doi:10.1016/s1353-2561(03)00036-7
Reis, I., Almeida, C. M. R., Magalhães, C. M., Cochofel, J., Guedes, P., Basto, M., et al. (2014). Bioremediation potential of microorganisms from a sandy beach affected by a major oil spill. Environ. Sci. Pollut. Res. 21, 3634–3645. doi:10.1007/s11356-013-2365-7
Riazi, M. (2005). Characterization and properties of petroleum fractions. West Conshohocken, PA: ASTM international.
Robertson, D. R., and Maddox, J. H. (2003). “Shoreline surface washing agent test and evaluation protocol for freshwater use in the Great Lakes region,” in International oil spill conference (Vancouver: American Petroleum Institute), 319–325.
Rodrigues, M., Martins, R., Rogeiro, J., Fortunato, A., Oliveira, A., Cravo, A., et al. (2021). A web-based observatory for biogeochemical assessment in coastal regions. J. Environ. Inf. 38, 1–15. doi:10.3808/jei.202100450
Rosa, A. P., and Triguis, J. A. (2007). Bioremediation process on Brazil shoreline. Env. Sci. Poll. Res. Int. 14, 470–476. doi:10.1065/espr2007.02.377
Sakaya, K., Salam, D. A., and Campo, P. (2019). Assessment of crude oil bioremediation potential of seawater and sediments from the shore of Lebanon in laboratory microcosms. Sci. Total Environ. 660, 227–235. doi:10.1016/j.scitotenv.2019.01.025
Salanitro, J. P. (2001). Bioremediation of petroleum hydrocarbons in soil. Soil. Adv. Agron. 72, 53–105. doi:10.1016/S0065-2113(01)72011-1
Santos, R., Loh, W., Bannwart, A., and Trevisan, O. (2014). An overview of heavy oil properties and its recovery and transportation methods. Braz. J. Chem. Eng. 31, 571–590. doi:10.1590/0104-6632.20140313s00001853
Secretariat, J., and Island, B. (2009). Guidelines and strategies for oil spill waste management in arctic regions. Report.
Sleight, T. W., Khanna, V., Gilbertson, L. M., and Ng, C. A. (2020). Network analysis for prioritizing biodegradation metabolites of polycyclic aromatic hydrocarbons. Environ. Sci. Technol. 54, 10735–10744. doi:10.1021/acs.est.0c02217
Suhag, A., Ranjith, R., Balaji, K., Peksaglam, Z., Malik, V., Zhang, M., et al. (2017). Optimization of steamflooding heavy oil reservoirs. Bakersfield, California: OnePetro: SPE Western Regional Meeting.
Taylor, E., Challenger, G., Rios, J., Morris, J., McCarthy, M., and Brown, C. (2014). “Dilbit crude oil weathering on brackish water: Meso-scale tests of behavior and spill countermeasures,” in Proceedings of the thirty-seventh AMOP technical seminar on environmental contamination and response (Canmore, AB: Environment Canada).
Taylor, E., Owens, E., Lee, K., An, C., and Chen, Z. (2021). A review of numerical models for oil penetration, retention, and attenuation on shorelines. J. Environ. Inf. Lett. 5, 27–38. doi:10.3808/jeil.202100051
Tissot, B. P., and Welte, D. H. (1984). Composition of crude oils, petroleum Formation and occurrence. Berlin, Heidelberg: Springer, 375–414.
Tumeo, M. A., and Cote, A. (1998). Effect of flush water temperature and type on commercial shoreline cleaning agent efficiency. J. Soil Contam. 7, 213–226. doi:10.1080/10588339891334230
Venosa, A. D., Suidan, M. T., Wrenn, B. A., Strohmeier, K. L., Haines, J. R., Eberhart, B. L., et al. (1996). Bioremediation of an experimental oil spill on the shoreline of Delaware Bay. Environ. Sci. Technol. 30, 1764–1775. doi:10.1021/es950754r
Walker, A. H., Kucklick, J. H., and Michel, J. (1999). Effectiveness & environmental considerations for countermeasures. Pure Appl. Chem. 71, 67–81. doi:10.1351/pac199971010067
Wang, Z., An, C., Lee, K., Owens, E. H., Chen, Z., Boufadel, M., et al. (2020). Factors influencing the fate of oil spilled on shorelines: A review. Environ. Chem. Lett. 19, 1611–1628. doi:10.1007/s10311-020-01097-4
Wei, H., Hassan, M., Che, Y., Peng, Q., Wang, Q., Su, Y., et al. (2021). Spatio-temporal characteristics and source apportionment of water pollutants in upper reaches of Maotiao River, southwest of China, from 2003 to 2015. J. Environ. Inf. 37, 93–106. doi:10.3808/jei.201900415
Wu, T., Zheng, H., Zhang, S., and Ma, S. (2015). Research of oil spill response technology, international conference on education, management and computing technology (ICEMCT-15). Atlantis Press.
Yang, C., Yang, Z., Zhang, G., Hollebone, B., Landriault, M., Wang, Z., et al. (2016). Characterization and differentiation of chemical fingerprints of virgin and used lubricating oils for identification of contamination or adulteration sources. Fuel 163, 271–281. doi:10.1016/j.fuel.2015.09.070
Yavari, S., Malakahmad, A., and Sapari, N. B. (2015). A review on phytoremediation of crude oil spills. Water Air Soil Pollut. 226, 279–318. doi:10.1007/s11270-015-2550-z
Yoo, Y., Park, H., Choi, Y., Jung, J., Song, H., Kim, J., et al. (2021). Method for determining optimum operational conditions of microbubble scrubber using image processing. J. Environ. Inf. 38, 83–92. doi:10.3808/jei.202100457
Yue, R., An, C., Ye, Z., Bi, H., Chen, Z., Liu, X., et al. (2021). Cleanup of oiled shorelines using a dual responsive nanoclay/sodium alginate surface washing agent. Environ. Res. 205, 112531. doi:10.1016/j.envres.2021.112531
Yue, R., An, C., Ye, Z., Chen, X., Lee, K., Zhang, K., et al. (2022a). Exploring the characteristics, performance, and mechanisms of a magnetic-mediated washing fluid for the cleanup of oiled beach sand. J. Hazard. Mat. 438, 129447. doi:10.1016/j.jhazmat.2022.129447
Yue, R., An, C., Ye, Z., Gao, S., Chen, X., Zhang, B., et al. (2022b). A pH-responsive phosphoprotein surface washing fluid for cleaning oiled shoreline: Performance evaluation, biotoxicity analysis, and molecular dynamic simulation. Chem. Eng. J. 437, 135336. doi:10.1016/j.cej.2022.135336
Zengel, S., Bernik, B. M., Rutherford, N., Nixon, Z., and Michel, J. (2015). Heavily oiled salt marsh following the Deepwater Horizon oil spill, ecological comparisons of shoreline cleanup treatments and recovery. PLoS One 10, e0132324. doi:10.1371/journal.pone.0132324
Zhang, B., Matchinski, E. J., Chen, B., Ye, X., Jing, L., and Lee, K. (2019). “Marine oil spills—oil pollution, sources and effects,” in World seas: An environmental evaluation. Editor C. Sheppard (Academic Press), 391–406.
Zhang, Y., and Liu, H. (1997). “Screening for new surface washing agents and mechanisms of action,” in Proceedings of the twentieth AMOP technical seminar on environmental contamination and response (Ottawa, ON: Environment Canada), 1249–1281.
Keywords: oil spill, oil characteristics, shoreline cleanup, shoreline response operations, biodegradation
Citation: Chen X, Bi H, Yue R, Chen Z and An C (2022) Effects of oil characteristics on the performance of shoreline response operations: A review. Front. Environ. Sci. 10:1033909. doi: 10.3389/fenvs.2022.1033909
Received: 01 September 2022; Accepted: 04 October 2022;
Published: 20 October 2022.
Edited by:
Jianbing Li, University of Northern British Columbia, CanadaReviewed by:
Xiaying Xin, City University of Hong Kong, Hong Kong SAR, ChinaJie Yang, Minjiang University, China
Zoe Li, McMaster University, Canada
Copyright © 2022 Chen, Bi, Yue, Chen and An. This is an open-access article distributed under the terms of the Creative Commons Attribution License (CC BY). The use, distribution or reproduction in other forums is permitted, provided the original author(s) and the copyright owner(s) are credited and that the original publication in this journal is cited, in accordance with accepted academic practice. No use, distribution or reproduction is permitted which does not comply with these terms.
*Correspondence: Chunjiang An, chunjiang.an@concordia.ca