- 1Redox Regulation Laboratory, Department of Zoology, College of Basic Science and Humanities, Odisha University of Agriculture and Technology, Bhubaneswar, India
- 2Department of Zoology, School of Life Sciences, Ravenshaw University, Cuttack, India
- 3Department of Biotechnology, College of Basic Science and Humanities, Odisha University of Agriculture and Technology, Bhubaneswar, India
- 4Department of Veterinary Clinical Sciences, College of Veterinary Medicine, Iowa State University, Ames, IA, United States
The semi-sessile, ectothermic, amphibious mollusc Pila globosa receives less attention for studying its environmental aspects. Its ecological role, ecotoxic and physiological responses to environmental factors including pollution and during dormant periods such as hibernation and estivation to understand key information about many cellular metabolic events need to be revealed. We reviewed the ecotoxic and physiological responses of P. globosa to various abiotic and biotic stressors such as heavy metals, organophosphorous, carbamate and butachlor in relation its adaptation to energy expenditure, fat metabolism in the dormant period, desiccation, etc. The responses analysed in terms of oxidative stress, respiratory and antioxidant enzymes, carbohydrate metabolism and activities of neurotransmitter enzymes such as acetylcholinesterase to better understand the connection between environmental factors and metabolic events in P. globosa. Articles published in the English language from 1952 to July of 2022 in PubMed, Google search engine and AGRICOLA that describes any of the above-mentioned search terms in P. globosa were included in the review article. Articles not containing any of the above terms were excluded. The 41 results were found in PubMed and 10 results from AGRICOLA and about 80 filtered articles from Google, totaling about 80 articles published until July 2022 were included in this review. The varied enzymatic activities observed in this organism under the exposure to various chemicals imply their possible use as biomarkers of eco-toxicological studies in freshwater and grassland ecosystems, for example, alleviated body carbohydrate and protein contents are up to 32% and 37% during aestivation and hibernation, respectively. Similarly, activities of enzymes such as adenosine monophosphate deaminase, adenosine deaminase, succinic dehydrogenase, cytochrome-c-oxidase, and glutamate dehydrogenase are downregulated by 75.5%, 62.6%, 54%, 59%, and 62%, respectively, during above inactive periods. Acetylcholinesterase enzyme is found to be the most susceptible enzyme to be downregulated by 225%, 130%, 64%, 198%, 96.08% in this snail under exposure to environmental pollutants such as phorate (30 mg/L), formothion (37.5 mg/L), trichlorfon (2.5 mg/L), aldicarb (120 mg/L) and butachlor (100 µ moles), respectively. In conclusion, P. globosa can serve as a reliable ectothermic model for comparative ecotoxicological studies in grassland and freshwater environmental monitoring.
1 Introduction
Detailed information and elucidation of the ecotoxic responses of a species are always helpful for its conservation on the one hand and environmental protection on the other hand (Paital and Chainy, 2012). Recently much attention has been paid to find out physiological responses in terms of altered biochemical or enzymatic activities under the fluctuation of environmental factors in ectothermic invertebrates (Pöykiö and Perämäki, 2003; Migaszewski et al., 2011; Stankovic and Jovic, 2012, 2014; Sousa et al., 2014). The abiotic factors of environments such as temperature, water availability, food scarcity, and environmental pollutants like herbicides, pesticides, metals, etc. Impose severe impacts on various organisms (Kasiotis and Emmanouil 2015; Karimi et al., 2017; Kahlon et al., 2018; Sigurnjak et al., 2021). And the effects of the above factors are more sensitive and severe in ectotherms than in endotherms because temperature influences the physiology of the former more than the latter (Paital et al., 2016). Since the physiological responses of an organism share the link with the alteration of ecological responses, selecting a suitable organism will be ideal for studying the effects of abiotic environmental factors using biochemical markers such as enzymatic activities. In this regard, the freshwater apple snail Pila globosa opens a new window to study ecotoxic responses (Panda et al., 2021a; 2021b). However, only 41 articles on this organism are available in PubMed when searched with the term “Pila globosa” out of 223 articles on the search term “apple snail” as of 20.10.2022 (PubMed 2022a; 2022b). It hints that more works need to be done on this species in various aspects starting from the life cycle to the life history trade-off (Panda et al., 2021a; 2021b). The apple snail P. globosa is commonly called as Indian apple snail. It is an ectothermic mollusc adapted in water as well as on land and prefers areas with a large amount of aquatic vegetation (Ali et al., 2021). This snail can also remain for months in dormant stages in the winter and summer seasons. At such time, the organism may be highly sensitive even to mild exposure to low-dose pollutants and other environmental extremities. Therefore, apple snails can be an ideal model as it is a key organism in freshwater as well as grassland ecosystems, and on-demand, it is very easy to quantify and observe metabolic alterations in this organism in a controlled laboratory setting (Panda et al., 2021a; PubMed 2022a; 2022b).
This species is also more sensitive to genotoxic agents (Ali et al., 2021). So, it can be used as a biomarker for pollutants and can be used to monitor freshwater environments by observing their altered physiological activities (Pöykiö and Perämäki, 2003; Migaszewski et al., 2011; Stankovic and Jovic, 2012; Sousa et al., 2014; Stankovic et al., 2014; Kasiotis and Emmanouil 2015; Karimi et al., 2017; Kahlon et al., 2018; Sigurnjak et al., 2021). The freshwater snail P. globosa is very susceptible to organophosphates such as phorate (250%), formothion (130%) and trichlorfon (64%) and carbamates such as aldicarb (198%), herbicides such as butachlor (54.79%) and metals including mercury (44.3%) and nickel (101.55%) (Singh and Agarwal 1981; Sivaramakrishna et al., 1991; Rajyalakshmi et al., 1996; David et al., 2003). On the other hand, its cellular physiology is also influenced when the species is infected with various (human) parasites (vectors), especially for Schistoma (Nakao and Sasaki 2021; Wiroonpan et al., 2021). A as result, the consumption of the infected snails either raw or undercooked, creates a high chance for the transmission of a variety of diseases to livestock and humans from this species.
The snail P. globosa is mainly confined to the oriental and Ethiopia regions (Figure 1). In the oriental region, it is found in India, Sri Lanka, Burma, Thailand, Indonesia, Malaya, Vietnam, and the Philippines, while its habitats in Ethiopian region or Afrotropical Region include Africa, Arabia, and Madagascar. The genus Pila was thought to be originated from the African continent and then dispersed into Asia, probably due to continental drift (Sil et al., 2020). Pila genus includes about 30 species of snail, out of which P. globosa is one of the important species among them having its importance as food value, as fish diet in aquaculture and its semi-sessile, ectothermic nature enables it as a suitable model to study environmental physiology. The common species found in pan India is P. globosa, while P. virens, P. nevililana and P. theobaldi are found in South India.
The shell of P. globosa is 46.5 mm in length and is univalve like in other gastropods, and the top of the shell is called the apex. Apex is formed first, and the growth of the shell starts from it. A thick globe-like, brown, or black-colored single-pieced shell (univalvate) protects the soft body of the apple snail (Panda et al., 2021a, Panda et al., 2021b). The electron paramagnetic resonance spectrum reveals the existence of manganese ions in its shell in different oxidized states having Fe-S center containing protein molecules. Besides, the presence of bands of–OH vibration suggests the congregation of amino acids and its role in its shell generation (Prasuna et al., 2004).
Its aperture is 60.5 mm long and is closed effectively through the operculum that connects dorsally to hinder parts of the foot muscle when the animal is completely withdrawn to the shell (Figure 2). The foot contains many glandular cells which release calcareous contents for which the operculum is calcareous in nature (Prasuna et al., 2004). The exterior of the operculum contains a centrally located nucleus surrounded by 3–7 rings of lines that indicate the growth of the shell. The inner layer exhibits a distinct area called the boss to which the muscles of the operculum are attached (Prasuna et al., 2004; Panda et al., 2021a; 2021b). This special apple-shaped structure with only one opening at the operculum makes this species unique in nature to avoid environmental stressors. Thus, the structure of the body of P. globosa helps the animal from different biotic as well as abiotic stressors.
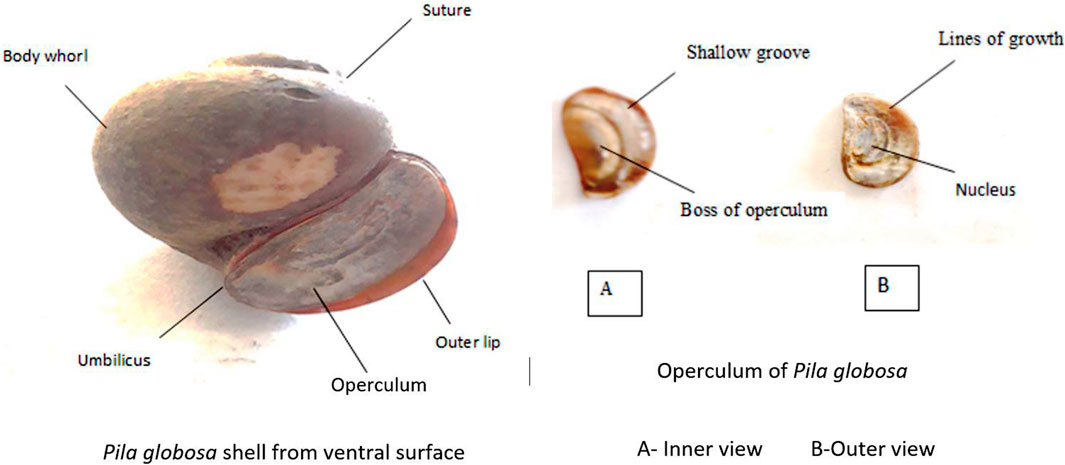
FIGURE 2. Morphology of P. globosa. The internal body parts are protected by the globular body of P. globosa, which is closed by the aperture. There are 3–7 circular lines of growth present on the outer surface of the operculum, and the innermost central circular line is called as the nucleus. Similarly, the central part of the inner surface of the operculum is called as boss.
The anatomy of the shell reveals three distinct layers. The thin outer layer is the periostracum. It is composed of uncalcified, homogenous organic material containing glycoproteins and macromolecules that help nucleate mineral components and act like resin to prevent shell fracture (Naresh et al., 2013). The bulkiest, calcified mid-layer is ostracum, and the brim of the shell aperture forms the right angle to the plate of this layer. The inner layer is hypostarcum or nacre, referred to as the mother of the shell consisting of aragonites and amorphous CaCO3. The brim of the shell aperture runs parallel to it.
The gastropod P. globosa undergoes dormant periods such as hibernation as well as aestivation like other lower animals. Nearly all physiological processes are halted during these periods in order to eliminate hyper- or hypothermia and desiccation. Pila withdraws its soft parts into the shell and is covered by the operculum through secreting dried mucus called the epiphragm to prevent the water deprivation state during that period. Epiphragm is rich in proteins that contribute to17-23 dry weight % and low in carbohydrates that contribute to 0.4-2 dry weight % (Meenakshi 1964). When P. globosa is allowed to aestivate without the operculum exposing its soft tissues, its survivability ranges below ten days, indicating that the operculum prevents water and electrolytes from its soft tissues during aestivation (Meenakshi 1964). Therefore, the operculum plays a vital role in adaptation against abiotic and biotic stress in apple snails by preventing desiccation.
The freshwater snail P. globosa undergoes nearly 80 days of either hibernation or aestivation under the harsh winter or summer environments, respectively (Panda et al., 2021a, Panda et al., 2021b). And the physiological responses of this snail also vary accordingly (Panda et al., 2021a, b). During the dormant period, most physiological activities cease, and many are modulated in terms of changes in enzymatic activities. For instance, the transition of excretory product from ammonia to uric acid is observed during dormant periods due to 62.6 and 75.3% down-regulation of adenosine deaminase and adenosine monophosphate deaminase, respectively (Moorthy et al., 1982). Besides the above, activities of respiratory and mitochondrial enzymes such as succinic dehydrogenase, cytochrome C oxidase, and glutamic dehydrogenase are alleviated by 54, 59, and 62% in response to the action of sterols under the dormant period (Raghupathiramireddy 1967). There are also alterations in carbohydrate and fat metabolism in this snail. For example, 70.6% reduced and 81% elevated carbohydrate, and fat metabolism is recorded in this snail in response to tannery effluent exposure, respectively (Bhattacharya et al., 2016). Thus, the above alteration of physiological activities is essential, crucial, and beneficial for the organism. The ecological environment of its habitat can be monitored by determining such fluctuation of metabolic events in this species (PubMed 2022a; 2022b).
Despite broad availability in India and many other Asian countries, only 41 hits are available in PubMed against P. globosa, which shows the need for more investigation on this species ((Panda et al., 2021a; PubMed 2022a; 2022b, Figure 3). The first work on it was done Lal and Saxena (1952) on uricotelism. Most of the studies were investigated during 1950–1990. Only five articles are present from 2009 to date in PubMed, illustrating that P. globosa is a poorly investigated species. The current direction of research on this organism points to its potential utility as an ecotoxic model for investigating its behavioural, metabolic, and physiologic responses to pollution and other anthropogenic activities.
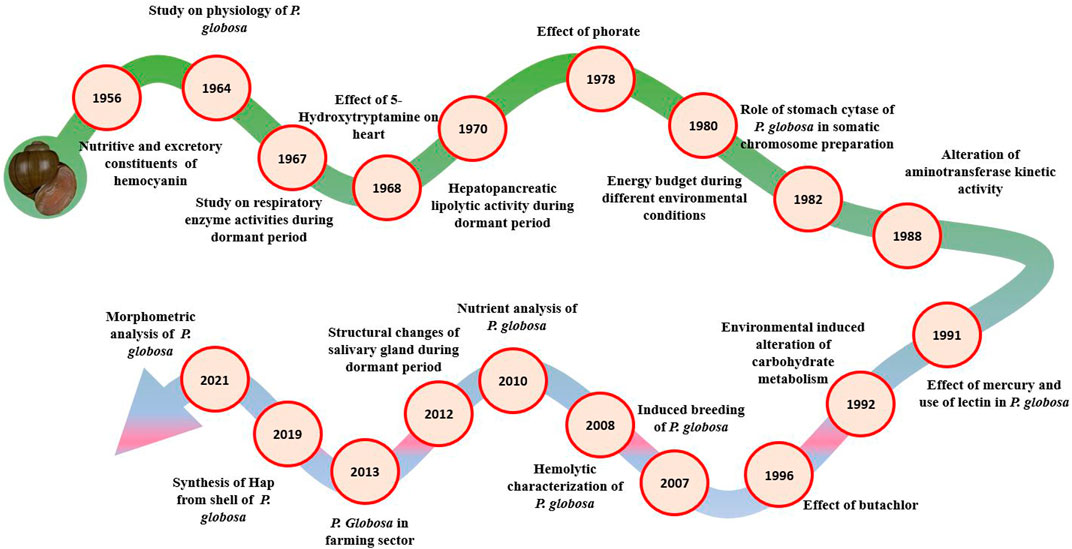
FIGURE 3. Chronology of works done on different aspects of P. globosa. Most of the works have been done on the alteration of excretory patterns and the effect of insecticides on P. globosa. P. globosa is thought to be originated from the African continents. Continental drift results in the dispersion of this species to oriental and Ethiopian regions. In the oriental region, it is found in India, Sri Lanka, Burma, Thailand, Indonesia, Malaya, Vietnam, and Philippines while habitats of the Ethiopian region include Africa, Arabia, and Madagascar.
The molluscs, including P. globosa, are a good source of trace elements and minor elements that are required for the proper growth of humans as well, as they can be utilized as feed for many livestock and fishes (Baby et al., 2010). Because of its low cost and high protein content compared to commercial shrimp feed, P. globosa can be used as feed in prawn farming (Nahid et al., 2013). However, overexploitation of this species for prawn feed can also affect its population. Therefore, Das et al. (2020) proposed an artificial breeding of P. globosa which can eliminate the above problem.
P. globosa can be consumed as a food source, especially for patients and people suffering from malnutrition, as it has a high source of protein and low lipid content and is cheaper than other animal protein sources (Nargis et al., 2011). With the induction of the optic tentacle and pituitary gland, the reproductive capability, especially the egg clutch number, can be increased in P. globosa, which is suggested to improve the commercial farming of this species (Jahan et al., 2007). The alimentary canal is directly proportional to the shell length, and Huq et al. (2002) proposed that the 50–55 mm length of P. globosa has high economic values as snails within this range consume the maximum amount of food. The stomach juices obtained from P. globosa can be used to prepare somatic chromosomes of fern Adiantum capillus-vernis (Linn) and Ampelopetris prolifera (Copel) for cytological studies (Niranjan and Roy 1982). Kumud and Minakshi (1994) found that the digestive tract of P. globosa is slightly acidic, which may have a role in squashing during the preparation of somatic chromosomes. Altogether it indicates that P. globosa has high economic values. Overall, P. globosa can be used as live stocks, commercial farming as well as acts as a good source of trace and minor elements which explains about the economic importance of this species.
Owing to its economic importance, the responses of the organism at biochemical and molecular levels to various abiotic and biotic insults must be studied to better understand the possibility of the use of this snail as bio-indicator species as well as for its judicial exploitation. To study these facets, a comprehensive understanding of its basic life cycle and life history trade-offs is required. A detailed and extensive evaluation of this organism is lacking despite its high demand in aquaculture as fish food, fish baits, and as having human consumption values. The present study was undertaken to review its various life cycle-associated issues in relation to metabolism, physiology, adaptation, etc under various environmental factors including pollutants. This species has a lot of role in ecosystems; it has agricultural values as it is used as fish bait in aquaculture in many countries, and most importantly, it has consumption values. In many countries, including India, China, and Bangladesh, the foot muscle and hepatopancreas have edible value (Panda et al., 2021a; 2021b). However, no scientific literature is available to elegantly review its various aspects of life in relation to biotic and abiotic factors. Especially, information on the responses of the snail at biochemical and molecular levels to environmental stressors, including pollutants, is not available. Such data are needed to manage this species both for its aquaculture and agriculture, as it is hugely found in cultivated grass and water-submerged lands. Here we review the effects of biotic and abiotic factors on its metabolic responses to understand the impact of the environment on its physiology as well as on the survival of this organism.
2 Materials and methods
To make a comprehensive review of this species in relation to environmental effects, we used search terms such as “apple snail,” Pila globosa,” “snail,” etc., in PubMed, AGRICOLA databases, and Google scholar and only peer-reviewed published literature were selected using traditional methods. The electronic databases were used to conduct a comprehensive search of the literature on this topic related to Pila sp., with a focus on the literature written in English.
2.1 Data sources and search strategy
PubMed/MEDLINE is a large database in biomedicine, and the Google search engine eventually stores the entire published peer-reviewed and other articles, while AGRICOLA contains about 6 million records associated with agriculture and allied sciences. It is to be noted that Pila sp is exclusively available in cultivated lands and grasslands, so it is included in the AGRICOLA database. The aforementioned factors played a role in our decision to use the above databases. Of the entire papers available in all the above databases on molluscs, only peer-reviewed literature published in English on “Pila globosa” was screened in relation to various search terms. Search terms such as “ecotoxic”, “physiological responses”, “abiotic and biotic stressors”, “heavy metals”, “organophosphorus”, “carbamate”, “butachlor”, “adaptation” “energy expenditure”, “fat metabolism”, “dormant period”, “desiccation”, “oxidative stress”, “respiratory” and “antioxidant enzymes”, “carbohydrate metabolism” “biological macromolecules”, “neurotransmitter enzymes”, “acetylcholinesterase” along with “Pila globosa” or “P. globosa” were searched ion the two databases electronically.
2.2 Study selection
The entire published peer-reviewed articles in the English language in books, journals, periodicals, and various authentic reputed webpages from 1952 to July of 2022 on “Pila globosa” in relation to the search terms in PubMed/MEDLINE, AGRICOLA, and Google search engine with above search terms were screened in the review article. Articles not containing any of the above terms were not included in the review. Articles matching within the subject area of the Pila sp. Were included in the review, whereas any axillary article in which the above term(s) is merely used was excluded from the review. Since <50 and 10 articles were published on the snail P. globosa in PubMed/MEDLINE and AGRICOLA, respectively, all the articles were included in the study while the articles were filtered in the Google search engine. All the data from the published papers were independently extracted unbiasedly, irrespective of the geographical region. Published literature from all geographical area was selected for screening.
2.3 Data extraction
In order to streamline the entire review process securely and to ensure the reliability and consistency of this mollusc species, we have covered various aspects such as the economic importance, species origin, morpho-anatomical adaptive features, cellular, organ-specific, metabolic, behavioral adaptations against the biotic and abiotic stressors of the species Pila sp. Also, we have elegantly reviewed the effects of the specific chemicals and pollutants on its studied biochemical and molecular pathways. The role of this organism in its ecosystem as a disease carrier and ecologic relation with other co-habitants has also been reviewed for its better exploitation. It will extend the existing understanding of the link between enzymatic and pathophysiological activities or responses in lower animals and P. globosa, in particular. The objective of this article was to systematically review information to elucidate for the first time the ecotoxic responses, such as redox regulatory activities, neurotransmitter enzyme levels, and responses to heavy metal exposure of this snail. As a result, it can be helpful for its conservation in general and environmental ecotoxic and environmental chemistry studies in particular.
2.4 Synthesis and analyses
Once the authors were convinced of the peer-reviewed articles on this species, altogether, 41 and 10 articles were obtained from the PubMed and AGRICOLA databases, respectively on the species, P. globosa were analyzed in the study, and the total articles published on P. globosa in Google search engine along with any additional mentioned search terms were filtered to 80 number and some of them were repetitions among all databases. The subject area of each article was extracted and categorized to describe the economic importance and other life cycle aspects of this species in relation to various environmental factors, which could be natural or anthropogenic in nature. However, the number of peer-reviewed articles on P. globosa indicates scanty studies on this species, starting from physiological responses to life history trade-off, and it was emphatically analyzed in the present review.
3 Circulatory system and its modulation by environmental factors
3.1 Structure of circulatory cells
The blood (hemocyanin) and the heart constitute the circulatory system in P. globosa, and this system, especially the heartbeat, is modulated by a small change in the surrounding environment (Panda et al., 2021a; 2021b). The hemocyte contributes to 5 physiological events such as wound and shell repair, nutrition, transport, excretion, and immunology in univalve organisms (Panda et al., 2021a; 2021b). Mahilini and Rajendran (2008) identified granulocyte I in P. globosa as the smaller and younger hemocytes with a 5.28 µm diameter. They characterized larger hemocytes into granulocytes II and III with diameters of 16.5 and 22.46 µm, respectively. They differentiated two granulocytes based on the staining property having a centrally and eccentrically located nucleus with heavy cytoplasm. However, more studies are still needed to understand the role of different hemocytes in relation to the immune system and detoxification in P. globosa.
3.2 Control of heart rate by 5-hydroxytryptamine
Nowadays, much attention has been paid to selecting preferable controlling agents for a heartbeat in the natural population. Lal and Agarwal (1968a) standardized a medium containing saline solution for sustaining the P. globosa heart by adjusting the composition of different ions such as NaCl, KCl, CaCl2, MgCl2.6H2O, MgCl2.7H2O, Na2HPO4.12H2O at 3.17, 0.373, 0.85, 0.254, 0.028, and 0.063 g, respectively in one liter of distilled water and finally, introduced glucose into it as the source of energy. The chemical agent 5-hydroxytryptamine (5-HT), a neuro-hormone, is found in tissues of apple snail I and is responsible for controlling the heartbeat of P. globosa as it increases the heartbeat, which is prevented by Lysergic Acid Diethylamide (LSD) as stated previously. Later on, it was found that LSD rather increases the heartbeat as it mimics 5-hydroxytryptamine, although the bromine derivative of LSD (10−7 to 10−8 g/ml) sometimes elevates the threshold of 5-HT. The chemical 5-hydroxytryptamine (5-HT) is a derived product from one of the essential amino acids, L-tryptophan; it is required for brain development and is influenced by the environment in many organisms (Booij et al., 2015). Therefore, the management of this molecule for the regulation of the circulatory system is crucial under varying environmental conditions; however, additional research on this aspect of P. globosa is anticipated. Alkaloid reserpine also has the same effect of increasing amplitude but decreasing heartbeat frequency (Lal and Agarwal 1968b). Therefore, LSD and alkaloids can be used as possible heart rhythm controllers in the future by providing the threshold amount of doses in P. globosa in particular.
Although varieties of hemocytes in apple snails have been discovered, the role of these cells in the immune system is yet to be revealed. Similarly, LSD and alkaloids, along with 5-HT, can control the heart rhythm in gastropods in general and in P. globosa in particular. So, the immunological responses indicate that other biochemical mechanisms must be affected in this snail under various ecotoxic conditions, including heavy metal exposure.
4 Ecotoxic responses of snail Pila sp. toward chemicals
Various chemicals used as insecticides, pesticides, and herbicides can affect the non-target species (Figure 4; Table 1). Since P. globosa inhabits pond and grassland ecosystems, there is every chance of bioaccumulating toxic molecules in them. Furthermore, because humans consume it as part of their food in many different places, it is likely to negatively impact human health via biomagnification.
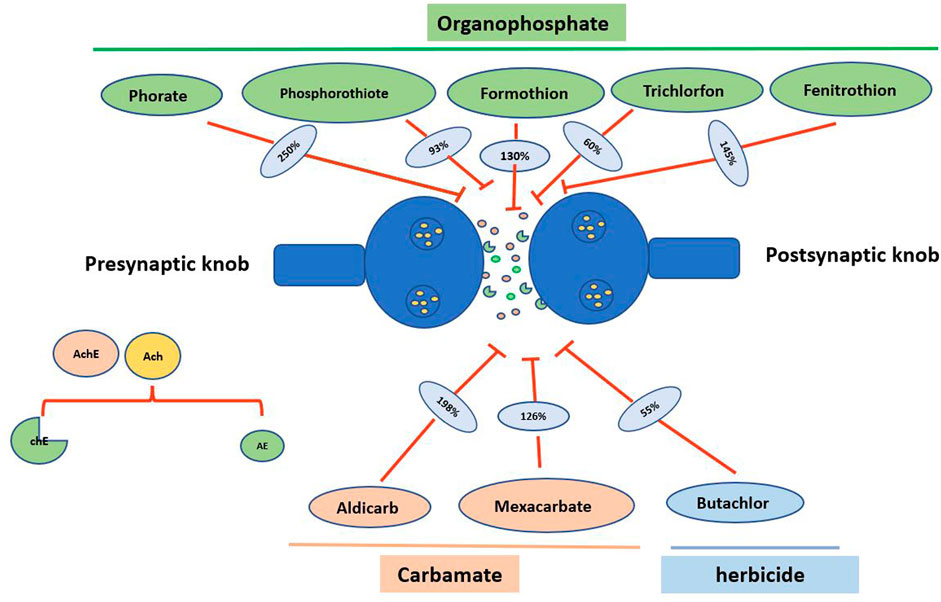
FIGURE 4. Action of pesticides on acetylcholinesterase activity during nerve impulse. Acetylcholine (Ach) a neurotransmitter enzyme that is released from the presynaptic knob where it splits into acetyl coenzyme A (AE) and choline (chE) with the help of the enzyme acetylcholinesterase. Organophosphates (Phorate, phosphorothiote, formothion, trichlorfon and fenitrothion), carbamates (aldicarb and mexacarbate), and herbicide (butachlor) inhibit the action of acetylcholinesterase enzyme thus, block the nerve impulse in the organism. % Inhibition by pesticides is designated in respective blue color oval structure.
4.1 Toxic effects of organophosphorus on P. globosa
Organophosphate is the organophosphorus compound and is mostly used as insecticide in general. An organophosphate compound phorate inhibits the hydrolytic activity of acetylcholinesterase by 175%–325% by forming phosphorylated enzyme complexes, and this effect was largely found to be time-dependent but not dose-dependent. Similarly, another organophosphate, phosphorothioates, also inhibits acetylcholinesterase activity by forming an oxygen analog (Singh and Agarwal 1978). The effects of pesticides such as formothion and trichlorfon on the acetylcholine activity of P. globosa are shown to be time-dependent, similar to phorate. For instance, formothion takes 55 min longer than trichlorfon to achieve maximal effectiveness. Therefore, different organophosphates impact differently in a time or dose-dependent manner on P. globosa, which illustrates the mechanism of the effects of organophosphates.
Some organophosphates exert toxicity selectively under different conditions. For instance, a high concentration of two pesticides, formothion, and trichlorfon, blocks the activity of acetylcholinesterase on excitatory receptors only by 130% and 64%, respectively. It indicates the selective action of organophosphates on two different types of acetylcholine receptors (Singh and Agarwal, 1979). Similarly, carboxy esterase in the hepatopancreas of P. globosa is sensitive to organophosphates such as methyl parathion and fenitrothion (Aparna et al., 2010). The above information suggests the selective active action of organophosphates and is recommended in P. globosa as possible biomarker of organophosphate toxicity.
4.2 Toxic effects of carbamates on P. globosa
Carbamate, another class of insecticides, is nearly similar to the structure as well as the mechanism of organophosphates. Metcalf (1971) proposed that carbamate compounds having N-methyl and N, N -dimethyl groups show anti-acetylcholinesterase activity. However, aldicarb 107%–289% inhibits acetylcholinesterase activity though it does not contain either of the above chemical groups (Singh and Agarwal 1979). It is found that P. globosa is more susceptible to the toxicity of carbamate, such as mexacarbate, in comparison to that carbaryl and aldicarb (Singh and Agarwal 1981). The above studies found that P. globosa is susceptible to carbamates as this compound targets the major neuromuscular enzyme acetylcholinesterase, thus elevating the level and duration of acetylcholine enzyme action.
4.3 Toxic effects of herbicides on P. globosa
Herbicides are generally used to control plants, but these compounds can target non-specific organisms non-selectively. Butachlor, an herbicide, affects non-target species like P. globosa, in which it decreases 54.79% and 96.08% in acetylcholinesterase activity and butyryl-cholinesterase enzymes, respectively (Rajyalakshmi et al., 1996). However, the activity gradually increased up to 82%, possibly due to either operating any degradation mechanism or increasing the activity of both enzymes as a compensatory mechanism. Many other apple snails are found to be non-specifically susceptible to many herbicides (Panda et al., 2021a; 2021b). The above information indicates that P. globosa is susceptible to herbicides such as butachlor.
4.4 Effects of calcium salts
Calcium in the form of calcium chloride may present several significant health hazards depending on the specific circumstances. Exposure to calcium chloride causes activation of the glycolytic pathway and ATP hydrolysis as well as increases in the glucose level in P. globosa. Elevating the activity of the above enzymes helps in a 13.99% increment of succinate dehydrogenase activity through oxidative metabolism in P. globosa (Rao and Kumar 1982). Therefore, calcium chloride also alters physiological activities by targeting respiratory enzymes.
4.5 Toxic effects of metals on P. globosa
The amount of research that has been done on how heavy metals affect the metabolic and physiological responses of the snail P. globosa is quite limited. Therefore, special attention is needed to document the metals available in the environment, particularly in soil and water. Since this snail scrawls on the land, absorption of heavy metals into its body and their effects are expected. In the interest of its conservation, it has been tried to analyze the environmental physiology of P. globosa in the current article.
It is inferred that the decreased carbohydrate and protein contents are crucial in the dormant period in P. globosa. Acetylcholinesterase enzyme is the most susceptible enzyme to respond against pollutants. The decreased activities of respiratory enzymes are found to be vital in the dormant period. Heavy metal reduces the activity of enzymes involved in amino acid metabolism. The above can be reliably studied under pollution or environmental stress using P. globosa as a model organism.
4.5.1 Effects of nickel
Nickel induces toxicity in animals and is known as a respiratory irritant. Nickel exposure to P. globosa causes a 50% reduction of total protein content and a 15% elevation of ammonia level in its body. It also increases the activity of enzymes like aspartate aminotransferase, alanine aminotransferase, and glutamate dehydrogenase by 63.6%, 69.6%, and 69.9%, respectively (David et al., 2003). However, these activities are prominent in the hepatopancreas as compared to other tissues like foot muscle and mantle (David et al., 2003). More studies are required to confirm the enzyme activity profile of this snail to nickel exposure in a tissue-specific way. However, from the available literature, it is prominent that nickel deregulates the protein content in the snail and modulates certain respiratory enzymes, which is more noticeable in the hepato-pancreatic of the snail P. globosa compared to its other tissue. It could be due to the high metabolic status of the hepatopancreas as compared to other tissues (Panda et al., 2021a; Panda et al., 2021b).
4.5.2 Toxic effects of mercury on P. globosa
Being considered as a very toxic element, Mercury induces toxicity and may result in the death of various organisms. Mercury causes high damage and results in a 44.3% decrease in the rate of oxygen-consuming capacity in P. globosa. The trace elements, such as sodium, potassium, and calcium ions, play a key role in maintaining the osmoregulation process under mercury toxicity stress (Sivaramakrishna et al., 1991). Mercury also induces glycogenesis in P. globosa and reduces 54.1% glycogen phosphorylase activity along with progressively 88.1%, 85%, and 81.3% decrease in Na+-K+, Mg2+, and Ca2+ ATPase activities, respectively. However, after progressive exposure, the level of glycogen phosphorylase is 159% increased to compensate for such toxicity (Sivaramakrishna and Radhakrishnaiah 2000). Therefore, P. globosa can be used as a biomarker for mercury contamination as this element alters various metabolic markers such as oxygen consumption, glycogenesis, and activities of respiratory enzymes.
5 Responses of P. globosa to biotic stressors
5.1 P. globosa as intermediate host for trematode
The gastropod P. globosa can be considered as a threat to higher animals due to its parasitic activities, and it also acts as a host for many parasites. Freshwater snails, including P. globosa, are used as intermediate hosts for many parasites, especially by trematodes (Nakao and Sasaki, 2021; Wiroonpan et al., 2021). In turn, they can become a big threat to other higher organisms, including humans. A common trematode, Diplodiscus species, occasionally uses P. globosa as its intermediate host (Anjaneyulu 1967; Afaq-Husain and Shameel 2021; Hu et al., 2021), so there is a possibility for transmission to other higher trophic levels, including human beings. This is observed in some parts of India where the foot muscle of Pila is consumed as food. Metacercariae of trematode Pseudodiplodiscoides pilai reside in the esophagus of P. globosa while they mature in the stomach of this snail (Murty 1973). A trematode, P. pilai resembles Diplodiscus, Catadiscus, and Pseudodiplodiscus in having a single testis but varies in the structure of oral sucker and ventral sucker uses P. globosa as host (Murty 1970). Therefore, the involvement of this apple snail as an intermediate host suggests that it should be boiled enough before using this snail as a diet (Figure 5).
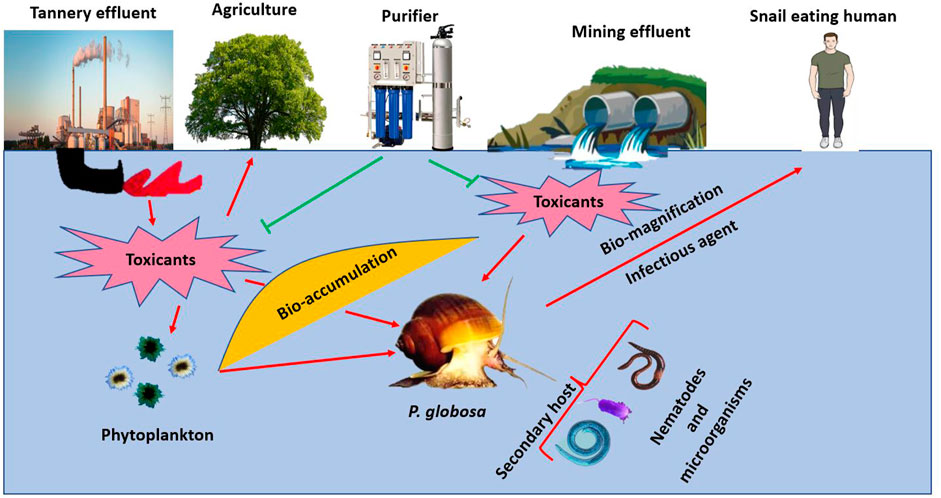
FIGURE 5. Effect of environment on P. globosa and ultimately on humans. Toxicants released from mining and industries are absorbed by phytoplanktons that are consumed by the snails. Because this animal is used as a diet by humans, especially in rural areas, the chances of biomagnification of toxicants are very high. P. globosa also acts as a secondary host for parasites like nematodes and microorganisms, which is likely to be transmitted to snail-eating animals.
5.2 P. globosa as an intermediate host for microorganisms
The snail P. globosa also acts as an intermediate host for many microorganisms. For example, it acts as a host for probiotic microorganisms, Pichia kudriavzevii, which can deplete the cholesterol level by performing various adaptive mechanisms. One mechanism includes 86.30% higher action of bile salt hydrolase and 20.29% inhibition of enzymatic activity by the generation of 3-hydroxy-3-methylglutaryl and absorbing cholesterol (Kathade et al., 2020). Bacteria like Aeromonas, Pseudomonas, Escherichia, and Listeria cause disease in the shell of P. globosa characterized by the formation of the blister in the periostracum of it, which later disconnects the underlying calcified layer (Ajesh and Sreejith 2014). The above information suggests the possible transmission of these microorganisms from P. globosa to higher organisms.
6 Metabolic and physiologic adaptations during dormant phases
When an organism is presented as a bio-indicator species, its life cycle trade-off must be thoroughly comprehended. Especially if the organism has an active reproductive phase (such as the estrus cycle in higher vertebrate females) during their use in experiments or dormant phases, then it must be noted. Because under these phases in the life cycle, many biochemical pathways get shut down and the dormant phases, such as hibernation and estivation, is very prominent in the snail P. globosa. The surrounding environment influences the metabolic activities of ectothermic animals. Very high temperature and low water availability during the summer and very low temperature and moderate water availability during the winter tend to influence the snail to undergo the dormant period, such as hibernation and aestivation, respectively. During the dormant periods, they shift from aquatic habitats to terrestrial habitats. Thus, in response to a change in habitat, the metabolic responses are also fixed as an adaptation by this species to survive in terrestrial habitats, as listed in Tables 2 and 3.
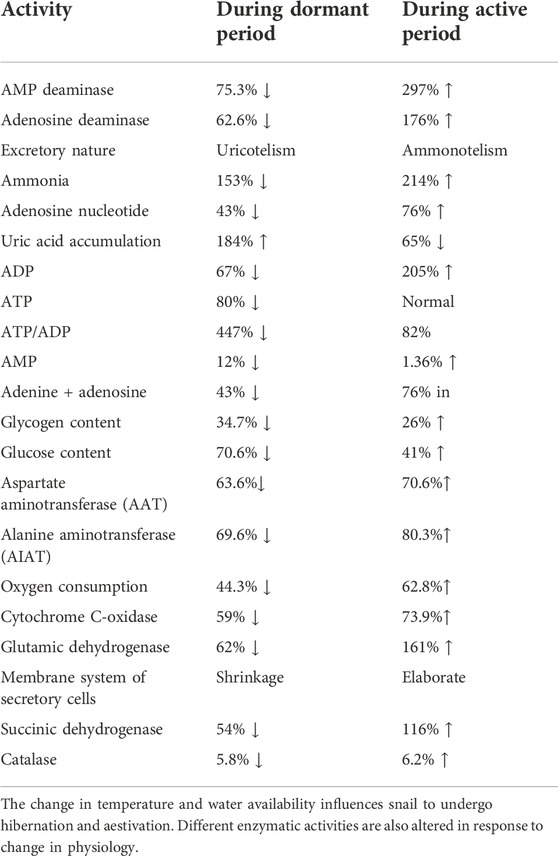
TABLE 2. Environmental induced alteration of different physiological activities of Pila globosa during dormant and active periods.
6.1 Alteration of redox regulatory activities
The antioxidant activities are influenced in response to various abiotic factors such as temperature, salinity, and pH in lower invertebrates (Paital and Chainy 2010; Paital and Chainy 2013a; Paital and Chainy 2013b; Pati et al., 2022). Exposure of untreated effluents released from tannery to P. globosa causes a 1.5 to 3.09 folds increase in antioxidant enzymes as well as 32 and 37% alleviation in carbohydrate and protein levels, respectively, in its different tissues (Bhattacharya et al., 2016). Along with that, comet assay reveals the damage of DNA that leads to genotoxicity (Bhattacharya et al., 2016). It indicates the elevation of antioxidant enzymes during unfavorable conditions, especially to generate oxidative stress, as elaborated in Table 3; Swarnakar et al., 1991. The catalytic potential (low Km and high Vmax) of aspartate and alanine aminotransferases are elevated by exposure to organophosphate malathion in vivo but do not change their activity in vitro. It indicates the role of the intracellular environment on the activity of these enzymes in P. globosa (Sahib and Rao 1988). Therefore, P. globosa can be used as a biomarker to assess the environment, especially the freshwater ecosystem, by determining the changes in antioxidant activities (Bal et al., 2021a, b). Few recent articles published also support this assumption, especially using the change in redox regulatory molecules as tissue and spatiotemporal specific (Panda et al., 2022a; 2022b).
6.2 Transition from ammonotelism to uricotelism
The excretory pattern is crucial for every organism, and in P. globosa, it plays a major role during hibernation and aestivation. During aestivation, 62.6 and 75.3% lower expression of adenosine deaminase and AMP deaminase are observed, respectively, in its hepatopancreas. It happened due to a 43% decrease in the level of adenosine nucleotide and a lower energy state in the snail. It is because ATP is the activator for AMP deaminase during aestivation, and snail decreases 153% lower production of ammonia due to the depleted AMP deaminase activity (Figure 6). On the other hand, the activity of AMP deaminase is 87.4% elevated during the active period so that ammonia production is increased by 214% and it does not become a risk to snail in aquatic habitat. This event implies the transition of ammonotelism to uricotelism during the aestivation condition (Moorthy et al., 1982). Therefore, adenosine deaminase and AMP deaminase play an important role in converting excretory products from ammonia to uric acid, thus protecting the animal from ammonia toxicity and desiccation during dormant periods.
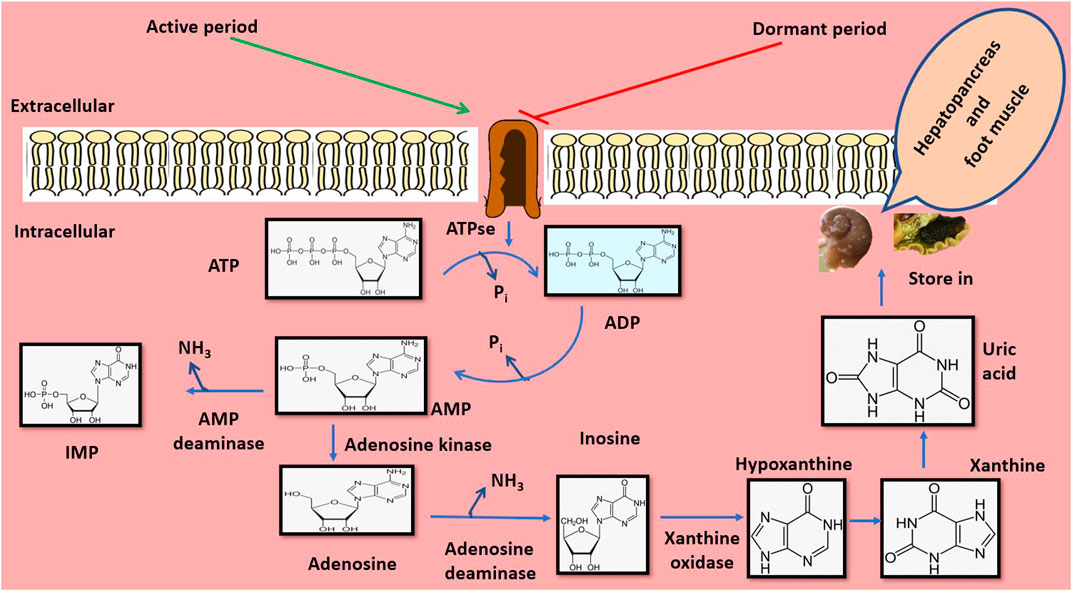
FIGURE 6. Environmental induced alteration of excretory pattern from ammonotelism to uricotelism. During the winter and summer seasons, when temperatures are extremely low and extremely high, respectively, the organism has a tendency to change its habitat from aquatic to terrestrial during the dormant period. During the active period, ATP serves as an activator for ammonia formation by converting into AMP. AMP is then transformed into IMP and inosine through the release of ammonia by AMP deaminase and adenosine deaminase enzymes. During dormant periods, the ATPase activity is blocked, so the splitting of ATP is also depleted, which in turn decreases the ammonia concentration, and the organism escapes from ammonia toxicity. Therefore, during dormant periods, the excretory products shift from ammonia to uric acid, which is stored in the hepatopancreas and foot muscle.
The metabolic activities also influence the alteration of excretory patterns during dormant periods. According to a report by Chaturvedi and Agarwal (1983), excretion of ammonia is restricted exclusively during active phases in the case of P. globosa due to the near absence of metabolic activity and preference for dry habitats during the dormant stages (Chaturvedi and Agarwal, 1983). During hibernation, this transition mode of the excretory system in P. globosa is transformed from ammonotelism to uricotelism. It could occur through Kreb’s cycle as ammonia is more toxic than uric acid, but the chance of elimination of this excretory product under the terrestrial mode of habituation becomes less feasible (Saxena 1956a). Moreover, uric acid acts as an excretory product that helps the animal conservation of water. Thus, the switching of excretory products is beneficial to this organism which prevents and saves the snail from desiccation and the toxic effects of ammonia during such dormant periods.
6.3 Organ-specific accumulation of excretory products
Many organs play crucial roles in accumulating excretory products during dormant periods. The hepatopancreas acts as the urea-generating site in P. globosa, probably due to the involvement of the ornithine cycle in it. During dormant stages, this metabolic waste cannot be excreted due to cease of excretion activities (Chaturvedi and Agrawal, 1979). About 97 and 138% elevated accumulation of uric acid in hepatopancreas and foot muscles, respectively, are documented during aestivation, which is analogous to such an event in insects (Athawale and Reddy 2000). Therefore, the foot muscle of P. globosa serves as a potential accumulation site during the dormant period. The membrane system of secretory cells of salivary glands in P. globosa is stretched during the active period to produce and store many secretory products. This metabolic process is ceased during aestivation, indicating the inactivation of the salivary gland during aestivation (Bhattacharyya et al., 2012). Therefore, the role of the hepatopancreas, foot muscle, and salivary gland is crucial in secreting and accumulating excretory products such as urea and uric acid by a few of the above-selected organs.
6.4 Physiological adaptation
During aestivation, many biochemical activities are also altered to compensate for the effects of lowered metabolic responses during dormant phases (Figure 7). The activities of glucogenic aminotransferases such as aspartate aminotransferase and alanine aminotransferase found in the hepatopancreas of P. globosa are elevated during an active period in comparison to that of aestivation period. It indicates the cessation of these enzymes during aestivation, which slows down amino acid metabolism (Reddy et al., 1990). Injection of sterol obtained from the cerebral ganglia of aestivated snail to an active one causes the depletion of oxygen consumption in the latter case (Meenakshi 1956). It is corroborated by the data that during the starvation period, the activities of respiratory and mitochondrial enzymes in Pila, such as succinic dehydrogenase, cytochrome C oxidase, and glutamic dehydrogenase are 54%, 59%, and 62% decreased, respectively, due to the action of sterols (Raghupathiramireddy 1967). The absence of glucose content is also found in P. globosa during the dormant period (Saxena 1956a). It may be due to glucose conversion to glycogen or non-carbohydrate substances like fat. Thus, most of the physiological activities are halted to overcome the energy expenditure of this organism during the dormant period.
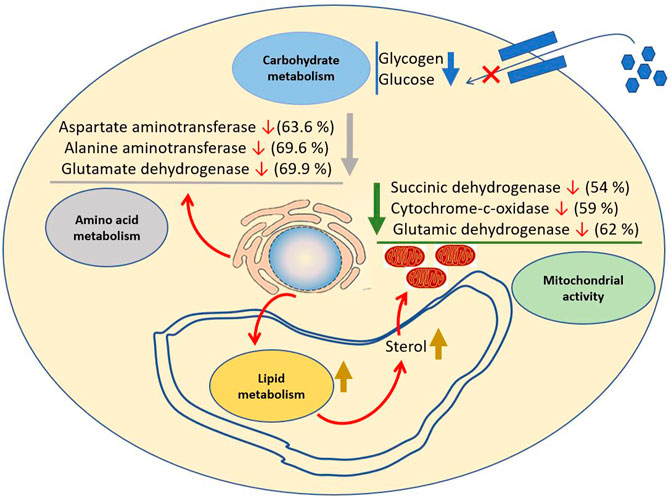
FIGURE 7. Environmental induced alteration of metabolism of P. globosa. Sterol plays a major role in metabolism during the dormant period. The sterol concentration suddenly rises during the dormant period which decreases the activity of respiratory and mitochondrial enzymes such as succinate dehydrogenase, cytochrome-c-oxidase, and glutamic dehydrogenase. Similarly, sterol also decreases amino acid as well as carbohydrate metabolism.
6.5 Alteration of carbohydrate metabolism
The carbohydrate metabolism and energy level are also altered in response to dormant periods in many ectotherms. A decrease in the level of ATPase activity during aestivation is found to be associated with an 80% increase in the ATP accumulation level in the snail as compared to the normal period. In turn, it lowers glycolytic activity by inhibiting phosphofructokinase enzyme through allosteric inhibition. It happens because the recovery event from aestivation is a process of energy expenditure, not energy saving (Raghupathiramireddy and Swami 1967). Similarly, the observed 34.7% and 70.6% decrease in glycogen and glucose levels, respectively, in ganglia (cerebral, pleuropedal, and visceral) of aestivated P. globosa indicates that carbohydrate is used as a source of energy during aestivation (Singh, 1976). However, the absence of glucose content in P. globosa by Saxena (1956b) may be due to the conversion of glucose to glycogen or non-carbohydrate substances like fat. However, fat may compensate for the lack of energy sources during dormant periods. This adaptive mechanism compensates for energy expenditure from carbohydrate sources during the dormant phases.
6.6 Role of fat during the dormant period
The role of fat is crucial during the dormant period. During aestivation, the iodine index or iodine absorption value mediated by ascorbic acid and fat is 81% elevated due to the increment of antioxidant enzymes in P. globosa (Krishnamoorthy 1968). Enzyme hydrolysis of methyl ester salicylate is increased, and olive and coconut oil are decreased during aestivation in P. globosa, indicating more hydrolysis of unsaturated fatty acid compared to saturated fatty acid during such periods (Krishnamoorthy and Brahmanandam 1970). Injection of sterol obtained from the cerebral ganglia of aestivated P. virens to an active one decreases oxygen consumption (Meenakshi 1956). The depletion of oxygen consumption suggests the 54%–62% inhibition of respiratory and mitochondrial enzyme activities due to the action of sterols during the starvation period (Raghupathiramireddy 1967). Therefore, sterol plays a crucial role during dormant periods by controlling oxygen consumption and respiratory and mitochondrial enzyme activities.
6.7 Adaptation in energy expenditure
In P. globosa, energy cost is dependent on the surrounding habitat. The energy expenditure decreases when the water level lowers in the habitat. For example, about 14.7 kcal g−1day−1energy expenditure under the lower water level in its habitat is recorded in this species, while the value is found to rise to 67.4 kcal g−1day−1 when the water level rises (Haniffa 1980a). Similarly, the energy budget of P. globosa is 1.5–3 times elevated during starvation conditions, while absorption efficiency and conversion efficiency are influenced by both feeding and available food levels by 93=–76 and 6%–8%, respectively (Haniffa 1987). The energy in this snail is utilized or released via aerobic as well as anaerobic metabolic processes during the aestivation period (Haniffa 1978). Therefore, the energy expenditure is less in the organism during the dormant phases of its life, and the demanding value of energy is elevated during post-dormant periods and is mostly modulated changes in the habitats of P. globosa.
Interestingly, the energy source content varies between sexes, and the body pattern becomes favorable for females compared to males in P. globosa. The fat and carbohydrate content of P. globosa are found to be significantly higher, accounting for 7 and 36%, respectively, in larger females compared to that of the same-sized male counterpart (Nargis et al., 2012). It shows the female-biased higher energy source that may play a crucial role in the development of females. The growth rate and body weight of Plia sp. Have a negative correlation with each other, as studied by Haniffa (1980b). It has been calculated that its body growth rate is reduced by 14.13% when its body weight is increased and increased during achieving adult stages (Haniffa 1980b). It indicates the existing relation between energy expenditure and body pattern, especially with the body growth of this snail. The above information suggests a higher energy source requirement in females than males in P. globosa. Overall, P. globosa shows adaptative nature in response to abiotic stressors by altering redox regulation, excretory system, and metabolism such as carbohydrate, fat, and energy.
7 Behavioral responses of P. globosa to
The snail P. globosa is semi-sessile in nature and its movement and other responses against the ecotoxic changes in the environment may be considered as excellent behavioral indices to study. Such studies are well recorded in other land snails such as Helix pomacella, Eobania vermiculata, H. melanostoma, and H. asemnis (Şereflişanand and Duysak, 2021). These authors studied that epiphragm layer plays an important role in above snails for their sustainable life cycle maintenance and most importantly to develop the capacity for ecological tolerances. The rate of mineral deposition on epiphragm layer formation governs their behavioral pattern including hypometabolism during the dormant phases of life. Studies on the activeness or any behavioral changes including locomotion, direction of locomotion like upward or downward movement, choosing the vegetation and habitat, opercular movement, sensing by the tentacles to any toxic changes especially to pollutants and their concentration in environment by the snail P. globosa could open a new window for environmental monitoring science Table 4.
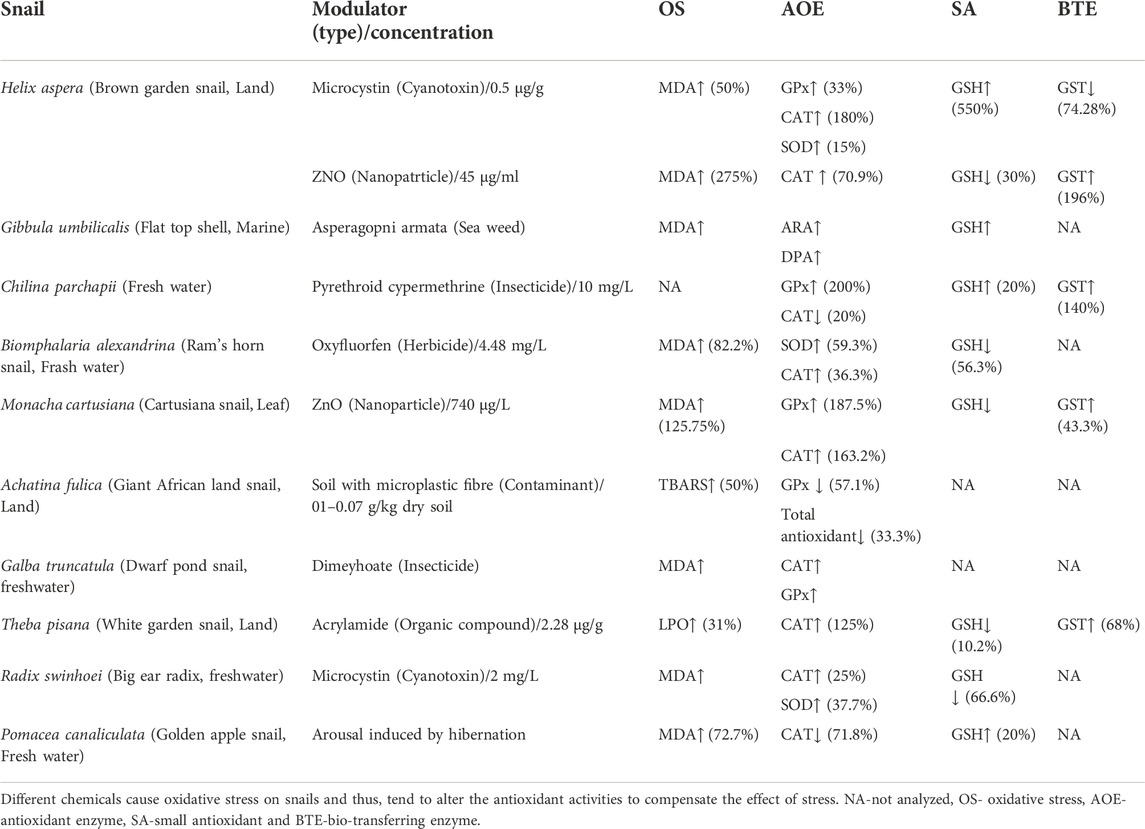
TABLE 4. Modulator induced oxidative stress of different snails. NA- Not analyzed. OS- oxidative stress.
8 Recent biotechnological findings in P. globosa
Nayak and Misra (2019) proposed that the shell of P. globosa can be used as a nanocrystalline hydroxyapatite precursor (HAp). They have confirmed using ICP-OES study that the shell has high calcium carbonate content (94.76%). While FTIR, SEM, XRD, XPS, BET, and FESEM studies revealed the presence of high-quality of HAp equivalent to natural apatite. It indicates that P. globosa shell can be used in the production of economically feasible as well as eco-friendly ceramic products without supplanting additives. The zero point of charge, natural pH, and dielectric characteristics confirmed that it could be used in the fabrication of electrical apparatus and orthopedic applications (Parveen et al., 2020). The physical and chemical characteristics of this species also revealed that instead of discarding their shell as aquaculture waste products, it can be used for bioremediation and pharmaceutical applications and as a source of lime (Parveen et al., 2020). Extract of P. globosa inhibits differentiation of osteoclasts through downregulation of nuclear factor ĸB and activated T-cells c1 signaling pathway. It indicates that it can be used in various treatments, especially against osteolytic bone diseases (Pandiarajan et al., 2019). The elegantly reviewed information about P. globosa and elucidation of its ecotoxic responses can be useful for environmental chemistry and environmental pollution and also be helpful for its conservation (Figure 8).
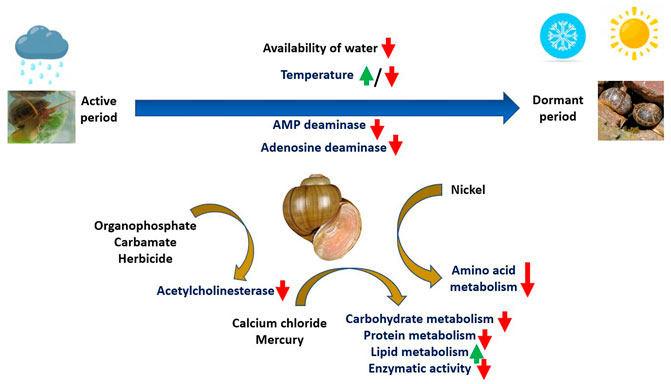
FIGURE 8. Biochemical, physiologic and environmental responses of the snail P. globosa in a grassland ecosystem. Various environmental stimuli in the grassland ecosystem are responsible for inducing biochemical and molecular responses in the snail during the active and inactive phases of life cycle. The responses start with ammino-acid catabolism to several enzymatic responses, as shown in the figure.
9 Conclusion
We reviewed the species P. globosa especially from its ecotoxicology aspects, using major databases such as PubMed, AGRICOLA, and Google search engine. Overall, the literature illustrates different aspects of P. globosa, especially its alteration of physiological and biochemical responses against the environmental induced dormant periods and under exposure to biotic and abiotic stressors, including pollutants. The molecular alteration from ammonotelic to uricotelic nature is observed in nature during its dormant periods, such as hibernation and aestivation. Various heavy metals, such as nickel and mercury found, induce stress factors in the snail. Especially the protein content is downregulated by nickel. Since this organism is semi-sessile, ectothermic, and amphibious in nature, the studied parameters such as alleviated body carbohydrate and protein contents during aestivation and hibernation, respectively, alleviated activity of adenosine monophosphate deaminase, adenosine deaminase, succinic dehydrogenase, cytochrome-c-oxidase, glutamate dehydrogenase and acetylcholinesterase under exposure to environmental pollutants such as phorate, formothion, trichlorfon, aldicarb and butachlor may be noticeably used as biomarkers of environmental pollution. More studies on elucidating its ecological roles and ecotoxic responses can be useful for monitoring and studying environmental chemistry, pollution, and ecotoxicology (Figure 8).
10 Future prospective
Various aspects of the snail P. globosa have been explored for a broad range of purposes, with a particular emphasis on its behavioral, metabolic, and cellular responses to ecotoxic natural and anthropogenic insults. This organism is mainly available in submerged and terrestrial grassland and hugely in cultivated lands. Because it is a semi-sessile ectotherm, it is possible to use this snail as a model organism to investigate the potentially harmful effects of various chemicals, including the fertilizers used on the aforementioned lands. The magnitude of responses of particular enzyme(s) such as acetylcholinesterase and the biotransformation enzyme glutathione-S-transferase against the dose of particular pollutants could provide usuful information. Adding the behavioral changes such as opercular movement, locomotion etc. could fortify the value of such studies. Its evolutionary adaptations at the molecular level during dormant phases, such as aestivation and hibernation, are proposed to be studied. Finally, having human consumption value, the nutritional profile of this organism may provide an option for a new venture on its food value.
11 Limitation of this study
We declare that we have collected all possible published information regarding the species “Pila globosa”, especially in relation to its ecotoxicology and ecophysiology. Including the published 41 articles in PubMed and about 80 articles in the Google search engine in English on the species in relation to its environmental physiology or toxicology is itself a limitation to making this review comprehensive. However, this demonstrates the paucity of studies on this species.
Author contributions
Conceptualization; data curation; formal analysis; funding acquisition; investigation; methodology; project administration; resources; software; supervision; validation; visualization; roles/writing—original draft; Writing—review and editing by BP, concept, writing—original draft; writing—review and editing by FP and DS, writing and revision by SP, KD, and LS.
Acknowledgments
Encouragements and laboratory facilities provided by the honorable Director, College of Basic Science and Humanities, Bhubaneswar, and the honorable Vice Chancellor, Odisha University of Agriculture and Technology Bhubaneswar, and the Central Instrumentation facility are duly acknowledged. Schemes (number ECR/2016/001984 from Science Engineering Research Board, DST, Govt. of India and 1188/ST, Bhubaneswar, dated 01.03.17, ST- (Bio)-02/2017 from Department of Biotechnology, DST, Govt. of Odisha, India) to BP are acknowledged.
Conflict of interest
The authors declare that the research was conducted in the absence of any commercial or financial relationships that could be construed as a potential conflict of interest.
Publisher’s note
All claims expressed in this article are solely those of the authors and do not necessarily represent those of their affiliated organizations, or those of the publisher, the editors and the reviewers. Any product that may be evaluated in this article, or claim that may be made by its manufacturer, is not guaranteed or endorsed by the publisher.
References
Afaq-Husain, S., and Shameel, M. (2021). The structure and reproduction of a new species Helminthocladia nizamuddinii (Nemaliales-Rhodophyta) from the Coast of Pakistan. Bot. Mar. 34 (2), 81–90. doi:10.1515/botm.1991.34.2.81
Ajesh, K., and Sreejith, K. (2014). Disease of the shells of Indian apple snails (Ampullariidae: Pila globosa). Ruthenica 24 (1), 31–33.
Ali, D., Ibrahim, K. E., Hussain, S. A., and Abdel-Daim, M. M. (2021). Role of ROS generation in acute genotoxicity of azoxystrobin fungicide on freshwater snail Lymnaea luteola L. Environ. Sci. Pollut. Res. 28 (5), 5566–5574. doi:10.1007/s11356-020-10895-w
Anjaneyulu, G. (1967). Amphistome (trematoda: Digenea) from the gut of the snail Pila globosa swainson. Ind. J. Helminthol. 19 (1), 23–26.
Athawale, M. S., and Reddy, S. (2000). Storage excretion in the Indian apple snail, Pila globosa (Swainson), during aestivation. Indian J. Exp. Biol. 40 (11), 1304–1306.
Baby, R. L., Hasan, I., Kabir, K. A., and Naser, M. N. (2010). Nutrient analysis of some commercially important molluscs of Bangladesh. J. Sci. Res. 2 (2), 390–396. doi:10.3329/jsr.v2i2.3362
Bal, A., Panda, F., Pati, S. G., Das, K., Agrawal, P. K., and Paital, B. (2021a). Modulation of physiological oxidative stress and antioxidant status by abiotic factors especially salinity in aquatic organisms. Comp. Biochem. Physiology Part C Toxicol. Pharmacol. 241, 108971. doi:10.1016/j.cbpc.2020.108971
Bal, A., Pati, S. G., Panda, F., Mohanty, L., and Paital, B. (2021b). Low salinity induced challenges in the hardy fish Heteropneustes fossilis; future prospective of aquaculture in near coastal zones. Aquaculture 543, 737007. doi:10.1016/j.aquaculture.2021.737007
Bhattacharya, P., Swarnakar, S., Mukhopadhyay, A., and Ghosh, S. (2016). Exposure of composite tannery effluent on snail, Pila globosa: A comparative assessment of toxic impacts of the untreated and membrane treated effluents. Ecotoxicol. Environ. Saf. 126, 45–55. doi:10.1016/j.ecoenv.2015.12.021
Bhattacharyya, K. N., Chaki, K. K., Sarkar, A. K., and Misra, K. K. (2012). Ultrastructure of the salivary gland cells in active and aestivated mollusk, Pila globosa (gastropoda: Orthogastropoda: Ampularidae). Proc. Zool. Soc. 65 (1), 64–69. doi:10.1007/s12595-012-0023-6
Booij, L., Tremblay, R. E., Szyf, M., and Benkelfat, C. (2015). Genetic and early environmental influences on the serotonin system: Consequences for brain development and risk for psychopathology. J. Psychiatry Neurosci. 40 (1), 5–18. doi:10.1503/jpn
Chaturvedi, M. L., and Agarwal, R. A. (1983). Ammonia excretion in snails Viviparus bengalensis (Lamark) and Pila globosa (Swainson) during active and dormant periods. Int. Rev. Ges. Hydrobiol. Hydrogr. 68 (4), 599–602. doi:10.1002/iroh.19830680413
Chaturvedi, M. L., and Agarwal, R. A. (1979). Excretion, accumulation and site of synthesis of urea in the snail Pila globosa during active and dormant periods. J. Physiol. 75 (3), 233–237.
Das, R., Islam, M. S., Moniruzzaman, M., and Ahmed, K. K. U. (2020). Natural breeding of freshwater apple snail Pila globosa (Swainson) in pond and aquarium. Bangladesh J. Fish. 32 (1), 45–54. doi:10.52168/bjf.2020.32.06
David, M., Mushigeri, S. B., and Prashanth, M. S. (2003). Nickel induced changes on some aspects of protein metabolism in the tissues of Pila globosa. J. Environ. Biol. 24 (1), 69–75.
Haniffa, M. A. (1987). Effect of starvation on food utilization in the freshwater snail Pila globosa (Swainson). Proc. Indian. Acad. Sci. 96 (2), 135–140. doi:10.1007/BF03179399
Haniffa, M. A. (1978). Energy loss in an aestivating population of the tropical snail Pila globosa. Hydrobiologia 61 (2), 169–182. doi:10.1007/BF00018748
Haniffa, M. A. (1980a). Influence of crowding and water level on food utilization in the freshwater snail Pila globosa (Swainson). Indian J. Exp. Biol. 18 (1), 71–73.
Haniffa, M. A. (1980b). Studies on life span, growth increments and energy budget of the freshwater snail Pila globosa (Swainson) in an artificial pond. Proc. Indian. Acad. Sci. 89 (3), 275–286. doi:10.1007/BF03179169
Hu, Z., Tong, Q., Chang, J., Yu, J., Li, S., Niu, H., et al. (2021). Gut bacterial communities in the freshwater snail Planorbella trivolvis and their modification by a non-herbivorous diet. PeerJ 9, e10716. doi:10.7717/peerj.10716
Huq, K. A., Hossain, M. I., and Huda, M. N. (2002). Abundance and feeding ecology of freshwater apple Pila globosa (Swinson) in bell ecosystem of Gopalgonj University. Univ. J. Zool. Rajshahi Univ. 21, 35–36. doi:10.3329/ujzru.v21i0.60
Jahan, M. S., Islam, M. R., Rahman, M. R., and Alam, M. M. (2007). Induced breeding of Pila globosa (swainson 1822) (gastropoda: Prosobranchia) for commercial farming. Univ. J. Zool. Rajshahi Univ. 26, 35–39. doi:10.3329/ujzru.v26i0.695
Kahlon, S. K., Sharma, G., Julka, J. M., Kumar, A., Sharma, S., and Stadler, F. J. (2018). Impact of heavy metals and nanoparticles on aquatic biota. Environ. Chem. Lett. 16, 919–946. doi:10.1007/s10311-018-0737-4
Karimi, B., Maron, P. A., Chemidlin-Prevost, B. N., Bernard, N., Gilbert, D., and Ranjard, L. (2017). Microbial diversity and ecological networks as indicators of environmental quality. Environ. Chem. Lett. 15, 265–281. doi:10.1007/s10311-017-0614-6
Kasiotis, K. M., and Emmanouil, C. (2015). Advanced PAH pollution monitoring by bivalves. Environ. Chem. Lett. 13, 395–411. doi:10.1007/s10311-015-0525-3
Kathade, S., Aswani, M., and Nirichan, B. (2020). Probiotic characterization and cholesterol assimilation ability of Pichia kudriavzevii isolated from the gut of the edible freshwater snail "Pila globosa. Egypt. J. Aquat. Biol. Fish. 24 (7), 23–39. doi:10.21608/ejabf.2020.119039
Krishnamoorthy, R. V., and Brahmanandam, V. (1970). Hepatopancreatic lipolytic activity in the aestivated pond snail, Pila globosa. Indian J. Exp. Biol. 8 (2), 145–146.
Krishnamoorthy, R. V. (1968). Hepatopancreatic unsaturated fatty acids during aestivation of the snail, Pila globosa. Comp. Biochem. Physiol. 24 (1), 279–282. doi:10.1016/0010-406X(68)90976-6
Kumud, M., and Minakshi, S. (1994). pH in the digestive system of some gastropod molluscs. Curr. Sci. 66 (9), 682–683.
Lal, M. B., and Agarwal, R. A. (1968a). A saline medium for maintaining isolated heart of Pila globosa, swainson. Proc. Indian Acad. Sci. -. Sect. B 67 (1), 1–7. doi:10.1007/BF03051678
Lal, M. B., and Agarwal, R. A. (1968b). Effect of 5-hydroxytryptamine and related compounds on the isolated heart of Pila globosa (gastropoda: Mollusca). Proc. Indian Acad. Sci. 67 (5), 195–200. doi:10.1007/BF03053901
Lal, M. B., and Saxena, B. B. (1952). Uricotelism in the common Indian apple-snail, Pila globosa (Swainson). Nature 170 (4337), 1024. doi:10.1038/1701024a0
Mahilini, H. M., and Rajendran, A. (2008). Categorization of hemocytes of three gastropod species Trachea vittata (Muller), Pila globosa (Swainson) and Indoplanorbis exustus (Dehays). J. Invertebr. Pathol. 97 (1), 20–26. doi:10.1016/j.jip.2007.07.007
Meenakshi, V. R. (1964). Aestivation in the Indian apple snail Pila-I. Adaptation in natural and experimental conditions. Comp. Biochem. Physiol. 11 (4), 379–386. doi:10.1016/0010-406X(64)90004-0
Meenakshi, V. R. (1956). Studies on the physiology of Pila virens (Lamarck) with special reference to aestivation. India: Dissertation, Annamalai University.
Metcalf, R. L. (1971). “The chemistry and biology of pesticides,” in Pesticides in the environment (New York: Dekker), 2–140.
Migaszewski, Z. M., Lamothe, P. J., Crock, J. G., Galuszka, A., and Dolegowska, S. (2011). The role of sample preparation in interpretation of trace element concentration variability in moss bioindication studies. Environ. Chem. Lett. 9, 323–329. doi:10.1007/s10311-010-0282-2
Moorthy, K. S., Reddy, B. K., Chetty, C. S., and Swami, K. S. (1982). Changes in activity levels of AMP deaminase and adenosine deaminase in the Indian apple snail, Pila globosa (Swainson) during starvation and aestivation stress. Experientia 38 (10), 1204–1205. doi:10.1007/BF01959741
Murty, A. S. (1973). Life cycle of Pseudodiplodiscoides pilai (Trematoda: Diplodiscidae) from the gut of the apple snail, Pila globosa (Swainson). J. Parasitol. 59 (2), 323–326. doi:10.2307/3278826
Murty, A. S. (1970). Pseudodiplodiscoides pilai gen. et sp. nov. (Trematoda: Diplodiscidae) from the apple snail Pila globosa (Swainson) in Andhra Pradesh. Zool. Anz 185 (3/4).
Nahid, S. A. A., Henriksson, P. J. G., and Wahab, M. A. (2013). Value-chain analysis of freshwater apple snail (Pila globosa) used for on-farm feeds in the freshwater prawn farming sector in Bangladesh. Int. J. Agril. Res. Innov. Tech. 3 (2), 22–30. doi:10.3329/ijarit.v3i2.17840
Nakao, M., and Sasaki, M. (2021). Trematode diversity in freshwater snails from a stopover point for migratory waterfowls in Hokkaido, Japan: An assessment by molecular phylogenetic and population genetic analyses. Parasitol. Int. 83, 102329. doi:10.1016/j.parint.2021.102329
Naresh, K. N., Krupanidhi, S., and Rajan, S. S. (2013). Purification, spectroscopic characterization and o-diphenoloxidase activity of hemocyanin from a freshwater gastropod: Pila globosa. Protein J. 32 (5), 327–336. doi:10.1007/s10930-013-9490-5
Nargis, A., Ali, S. H., Hasan, M. R., and Rahman, M. R. (2012). Seasonal variations in the chemical composition of apple snail, Pila globosa. Bangladesh J. Sci. Ind. Res. 47 (1), 89–92. doi:10.3329/bjsir.v47i1.10730
Nargis, A., Talukder, D., Parmanik, S. H. A., and Hasan, M. R. (2011). Nutritional value and physico-chemical characteristics of apple snail Pila globosa (swainson) and Lymnaea luteola lamark. Bangladesh J. Sci. Ind. Res. 46 (4), 539–542. doi:10.3329/bjsir.v46i4.9604
Nayak, B., and Misra, P. K. (2019). Recognition of the surface characteristics and electrical properties of a nanocrystalline hydroxyapatite synthesized from Pila globosa shells for versatile applications. Mat. Chem. Phys. 230, 187–196. doi:10.1016/j.matchemphys.2019.03.068
Niranjan, A. R. S., and Roy, S. K. (1982). The use of stomach cytase from the water snail Pila globosa in root tip squashes of ferns. Stain Technol. 57 (3), 188–190. doi:10.3109/10520298209066614
Paital, B., and Chainy, G. B. (2012). Biology and conservation of the genus Scylla in India subcontinent. J. Environ. Biol. 33 (5), 871–879.
Paital, B., and Chainy, G. B. N. (2010). Antioxidant defenses and oxidative stress parameters in tissues of mud crab (Scylla serrata) with reference to changing salinity. Comp. Biochem. Physiology Part C Toxicol. Pharmacol. 151, 142–151. doi:10.1016/j.cbpc.2009.09.007
Paital, B., and Chainy, G. B. N. (2013b). Modulation of expression of SOD isoenzymes in mud crab (Scylla serrata): Effects of inhibitors, salinity and season. J. Enzyme Inhib. Med. Chem. 28, 195–204. doi:10.3109/14756366.2011.645239
Paital, B., and Chainy, G. B. N. (2013a). Seasonal variability of antioxidant biomarkers in mud crabs (Scylla serrata). Ecotoxicol. Environ. Saf. 87, 33–41. doi:10.1016/j.ecoenv.2012.10.006
Paital, B., Panda, S. K., Hati, A. K., Mohanty, B., Mohapatra, M. K., Kanungo, S., et al. (2016). Longevity of animals under reactive oxygen species stress and disease susceptibility due to global warming. World J. Biol. Chem. 7 (1), 110–127. doi:10.4331/wjbc.v7.i1.110
Panda, F., Pati, S. G., Bal, A., Das, K., Samanta, L., and Paital, B. (2021a). Control of invasive apple snails and their use as pollutant ecotoxic indicators: A review. Environ. Chem. Lett. 19, 4627–4653. doi:10.1007/s10311-021-01305-9
Panda, F., Pati, S. G., Bal, A., Mathur, S., Nirmaladevi, R., and Paital, B. (2021b). Temporal morphometric analyses of Pila globosa in India for its use in aquaculture and food industry. JoBAZ. 82 (1), 17–19. doi:10.1186/s41936-021-00216-z
Panda, F., Pati, S. G., Bal, A., Samanta, L., and Paital, B. (2022b). Tissue specific redox regulatory system in semi-sessile amphibious Indian apple snail Pila globosa for future ecotoxic studies. Proc. Nat. Acd Sci. B. accepted.
Panda, F., Pati, S. G., Samanta, L., and Paital, B. (2022a). Seasonal variation of water quality modulated redox regulatory system in the apple snail Pila globosa and its use as bioindicator species in freshwater ecosystems across India. Water 14, 3275. doi:10.3390/w14203275
Pandiarajan, S., Samuel, S., Loganathan, T., Jaganathan, S. S., Krishnamurthi, T., Sarangapani, R., et al. (2019). Pila globosa snail extract inhibits osteoclast differentiation via downregulation of nuclear factor κB and nuclear factor of activated T-Cells c1 signaling pathways. Pharmacogn. Mag. 15 (64), 298. doi:10.4103/pm.pm_39_19
Parveen, S., Chakraborty, A., Chanda, D. K., Pramanik, S., Barik, A., and Aditya, G. (2020). Microstructure analysis and chemical and mechanical characterization of the shells of three freshwater snails. ACS Omega 5 (40), 25757–25771. doi:10.1021/acsomega.0c03064
Pati, S. G., Panda, F., Samanta, L., and Paital, B. (2022). Spatio-temporal changes in oxidative stress physiology parameters in apple snail Pila globosa as a function of soil Mg, Ca, organic carbon and aquatic physico-chemical factors. Environ. Geochem. Health. doi:10.1007/s10653-022-01376-9
Pöykiö, R., and Perämäki, P. (2003). Acid dissolution methods for heavy metals determination in pine needles. Environ. Chem. Lett. 1, 191–195. doi:10.1007/s10311-003-0038-3
Prasuna, C. L., Narasimhulu, K. V., Gopal, N. O., Rao, J. L., and Rao, T. V. R. K. (2004). The microstructures of biomineralized surfaces: A spectroscopic study on the exoskeletons of fresh water (apple) snail, Pila globosa. Spectrochimica Acta Part A Mol. Biomol. Spectrosc. 60 (10), 2305–2314. doi:10.1016/j.saa.2003.12.004
PubMed (2022a). Search term “apple snail”. Available at: https://pubmed.ncbi.nlm.nih.gov/?term=apple+snail (Accessed on 03 06, 2022).
PubMed (2022b). Search term “Pila globosa”. Available at: https://pubmed.ncbi.nlm.nih.gov/?term=pila+globosa (Accessed on 03 06, 2022).
Raghupathiramireddy, S. (1967). Respiratory enzymes during aestivation of the Indian apple-snail Pila globosa. Life Sci. 6 (4), 341–345. doi:10.1016/0024-3205(67)90002-1
Raghupathiramireddy, S., and Swami, K. S. (1967). Adenine nucleotides and adenosine triphosphatase activity during aestivation of the Indian apple snail Pila globosa. Can. J. Biochem. 45 (4), 603–607. doi:10.1139/o67-069
Rajyalakshmi, T., Srinivas, T., Swamy, K. V., Prasad, N. S., and Mohan, P. M. (1996). Action of the herbicide butachlor on cholinesterases in the freshwater snail Pila globosa (Swainson). Drug Chem. Toxicol. 19 (4), 325–331. doi:10.3109/01480549608998241
Rao, M. G., and Kumar, N. N. (1982). The tannery industrial effluent effect on succinate dehydrogenase activity pattern in a freshwater snail, Pila globosa. Proc. Ani. Sci. 91 (5), 427–431. doi:10.1007/BF03186139
Reddy, G. R., Babu, G. R. V., and Chetty, C. S. (1990). Metabolic consequences of glucogenic transamination in aestivating snail, Pila globosa (swainson): Neuroendocrine involvement. Biochem. Int. 20 (2), 287–290.
Sahib, I. K., and Rao, K. R. (1988). Studies on some kinetic parameters of aminotransferases in tissues of the snail, Pila globosa (Swainson) during malathion intoxication. Acta Physiol. hung. 71 (1), 31–34.
Saxena, B. B. (1956a). Excretory constituents of the blood of Pila globosa (swainson). Arch. Int. Physiol. Biochim. 64 (4), 578–582. doi:10.3109/13813455609145434
Saxena, B. B. (1956b). Nutritive constituents of the blood of Pila globosa (Swainson). Naturwissenschaften 43 (9), 201–202. doi:10.1007/BF00632954
Şereflişan, H., and Duysak, O. (2021). Hibernation period in some land snail species (gastropoda: Helicidae): Epiphragmal structure and hypometabolic behaviors. Turk. JAF. Sci. Tech. 9 (1), 166–171. doi:10.24925/turjaf.v9i1.166-171.3777
Sigurnjak, M., Cvetnić, M., Miloloža, M., Kučić Grgić, D., Markić, M., Kušić, H., et al. (2021). Modeling the toxicity of pollutants mixtures for risk assessment: A review. Environ. Chem. Lett. 19, 1629–1655. doi:10.1007/s10311-020-01107-5
Sil, M., Aravind, N. A., and Karanth, K. P. (2020). Into-India or out-of-India? Historical biogeography of the freshwater gastropod genus Pila (caenogastropoda: Ampullariidae). Biol. J. Linn. Soc. Lond. 129 (3), 752–764. doi:10.1093/biolinnean/blz171
Singh, I. (1976). Neurochemical correlates of aestivation: Changes in the levels of glycogen and glucose in the cerebral, pleuropedal and visceral ganglia of the Indian apple snail, Pila globosa. Proc. Indian Acad. Sci. -. Sect. B 84 (2), 56–59. doi:10.1007/BF03045582
Singh, O., and Agarwal, R. A. (1979). Effects of certain carbamate and organophosphorous pesticides on isolated organs of Pila globosa (Gastropoda). Toxicol. Appl. Pharmacol. 50 (3), 485–492. doi:10.1016/0041-008X(79)90402-2
Singh, O., and Agarwal, R. A. (1978). Pharmacological studies on the molluscicidal activity of phorate on Pila globosa (Gastropoda). Acta Pharmacol. Toxicol. (Copenh). 42 (5), 365–370. doi:10.1111/j.1600-0773.1978.tb02218.x
Singh, O., and Agarwal, R. A. (1981). Toxicity of certain pesticides to two economic species of snails in northern India. J. Econ. Entomol. 74 (5), 568–571. doi:10.1093/jee/74.5.568
Sivaramakrishna, B., and Radhakrishnaiah, K. (2000). Mercury induced alterations in the energetics of hepatopancreas of two freshwater molluscs, Pila globosa and Lamellidens marginalis. Trace Met. Environ. 4, 389–409. doi:10.1016/S0927-5215(00)80017-X
Sivaramakrishna, B., Radhakrishnaiah, K., and Suresh, A. (1991). Assessment of mercury toxicity by the changes in oxygen consumption and ion levels in the freshwater snail, Pila globosa, and the mussel, Lamellidens marginalis. Bull. Environ. Contam. Toxicol. 46 (6), 913–920. doi:10.1007/BF01689738
Sousa, A. C. A., Pastorinho, M. R., Takahashi, S., and Shinsuke, T. (2014). History on organotin compounds, from snails to humans. Environ. Chem. Lett. 12, 117–137. doi:10.1007/s10311-013-0449-8
Stankovic, S., and Jovic, M. (2012). Health risks of heavy metals in the mediterranean mussels as seafood. Environ. Chem. Lett. 10, 119–130. doi:10.1007/s10311-011-0343-1
Stankovic, S., Kalaba, P., and Stankovic, A. R. (2014). Biota as toxic metal indicators. Environ. Chem. Lett. 12, 63–84. doi:10.1007/s10311-013-0430-6
Swarnakar, S., Chowdhury, P. S., and Sarkar, M. (1991). N-Glycolylneuraminic acid specific lectin from Pila globosa snail. Biochem. Biophys. Res. Commun. 178 (1), 85–94. doi:10.1016/0006-291X(91)91783-9
Keywords: bioindicator species, ecotoxic studies, pesticide, Pila sp., acetylcholinesterase, environmental effects, environmental pollution monitoring, snail biology
Citation: Panda F, Pati SG, Das K, Samanta L, Sahoo DK and Paital B (2022) Biochemical and molecular responses of the freshwater snail Pila sp. to environmental pollutants, abiotic, and biotic stressors. Front. Environ. Sci. 10:1033049. doi: 10.3389/fenvs.2022.1033049
Received: 31 August 2022; Accepted: 09 November 2022;
Published: 21 November 2022.
Edited by:
Hai Ocean Xu, Jiangsu University, ChinaReviewed by:
Frederick Ato Armah, University of Cape Coast, GhanaAbubakari Zarouk Imoro, University for Development Studies, Ghana
Francis Addy, University for Development Studies, Ghana
Copyright © 2022 Panda, Pati, Das, Samanta, Sahoo and Paital. This is an open-access article distributed under the terms of the Creative Commons Attribution License (CC BY). The use, distribution or reproduction in other forums is permitted, provided the original author(s) and the copyright owner(s) are credited and that the original publication in this journal is cited, in accordance with accepted academic practice. No use, distribution or reproduction is permitted which does not comply with these terms.
*Correspondence: Biswaranjan Paital, Ymlzd2FyYW5qYW5wYWl0YWxAZ21haWwuY29t; Dipak Kumar Sahoo, ZGlwYWtzYWhvbzExQGdtYWlsLmNvbQ==ZHNhaG9vQGlhc3RhdGUuZWR1