- 1Laboratory of Genomic and Bioinformatics, Center of Genomics and System Biology, Institute of Biological Sciences, Federal University of Pará, Belém, Brazil
- 2Institute of Biological Sciences, Federal University of Minas Gerais, Belo Horizonte, Brazil
- 3Federal Rural University of the Amazon, Paragominas, Brazil
- 4Evandro Chagas Institute - IEC/SVS/MS, Department of Arbovirology and Hemorrhagic Fevers, Ananindeua, Brazil
The tropical Amazon has a unique biodiversity that has been affected by the development of pastures and economically important crops, such as soybeans. In the Amazon soil, the communities of microorganisms are diverse and act in different biogeochemical activities relevant to their adaptation to the environment. The assessment of changes in soil microorganism communities is essential to consider the impact of agribusiness action in one of the wealthiest regions in diversity in the world. Thus, the soil microbial diversity of the Amazon forest, the north region of Brazil, was evaluated regarding the influence of soybean farming with regions with periods of two and 14 years of exploitation, with regions of pasture and forest area, through the metagenomics approach with new generation sequencing technology, in addition, it was considered chemical characteristics such as pH value, organic matter content, macronutrients, micronutrients, and cations. High microbial diversity was identified at all collection sites and, despite this, bacterial, archaeal, and virus communities were very diverse between sites, with higher identification of Enterobacter cloacae and species of Pseudomonas, Pseudoplusia includens, Methanosarcina barkeri in the farmed and pasture, whose microbial diversity is influenced by the presence of cations and the interaction of organic matter with clay. It was evident that there is a change in the communities of native microorganisms for others adapted in the areas that had their vegetal cover eliminated.
1 Introduction
Brazil has 49% of its territory represented by the coverage of the Amazon rainforest (Kaschuk et al., 2011), but that in recent times has been reduced by the increase in agricultural activities. Currently, the disposition of these territories for pasture activities is 48.6% (172.3 Mha). For cultivation, it is 21.6% (76.7 Mha), of which 5.8% is used for soybean plantations (20.6 Mha) (Bordonal et al., 2018; Rajão et al., 2020; Leite-Filho et al., 2021). Soybean cultivation is vital in Brazil, with the South and Midwest regions as the most prominent producers (Colussi and Schnitkey, 2021; Cattelan and Dall’Agnol, 2018); however, the north region, which comprises part of the tropical Amazon, has a relevant role in agricultural production (Battisti et al., 2018; Lima et al., 2019). In this context, the production of transgenic soy is observed, whose development requires the application of different chemical compounds to prepare the soil and control pests (Alves et al., 2014).
Ecosystem changes are related to the reduction of macro and microdiversity, influence climate change, and variations in biogeochemical processes (Gardner et al., 2019; Tahat et al., 2020). Soil microorganisms develop most geochemical processes, such as organic carbon cycling, nutrient disposal, production and consumption of greenhouse gases, CO2, N2O, and CH4 (Jansson and Hofmockel, 2020), and strengthening the immune response and plant growth (Döring et al., 2020; Fitzpatrick et al., 2020).
Bacterial communities are the most dominant in the soil, but archaea, viruses, and fungi are present to a lesser extent, which coexist and interact with each other to maintain ecosystem functions (Naik et al., 2020). Likewise, the high value of diversity and the varied composition of microbial communities can be used as an indicator of soil quality and health (Chaparro et al., 2012; Wang et al., 2019). In this scenario, the adverse effects of contemporary agronomic practices, including conventional crops, use of machinery, application of pesticides, chemical fertilizers, and monocultures, among others, can alter macrobiological and microbiological diversity, modifying the native composition of different microorganisms that reside in a given ecosystem (Li et al., 2018; Chen et al., 2019; Saleem et al., 2019).
In this sense, changes in diversity and composition can favor the increase of opportunistic pathogens for the different organisms that inhabit this place, affecting the health of plants, animals, and humans; Most of the changes involve the relative abundance of different taxonomic groups, such as Acidobacteria, Bacteroidetes, Nitrospirae, Deltaproteobacteria and Proteobacteria (Colin et al., 2017; Lladó et al., 2018). Several of these organisms are usually associated with the development of diseases that affect humans, but in the soil, they are growth promoters and antagonistic against plant pathogens, such as the genus Escherichia, Burkholderia, Enterobacter, Pseudomonas, Ralstonia, Serratia, Agrobacterium, Pantoea, Herbaspirillum, Ochrobactrum, and Stenotrophomonas (Berg et al., 2014; Lladó et al., 2018).
In the different ecosystems, they are of complex composition and interaction; in the case of soil, microorganisms are in symbiosis, and the imbalance of this relationship can affect different levels of the health of plants, animals, humans, and the environment (van Bruggen et al., 2019; Brevik et al., 2020; Garcia et al., 2020). The use of next-generation sequencing allowed the metagenomic analysis, representing the most significant number of organisms in different habitats; these analyzes made it possible to show with better sensitivity the effects on microdiversity in the environment in time and space, highlighting the associations of different factors (e.g., Physical chemicals, heavy materials, phenolic compounds, among others) that interact with microbial communities (Nesme et al., 2016; Saleem et al., 2019). In this sense, different works describe the composition and diversity of the microbiome, the effect on the plant, and its influence on the culture (Liu et al., 2013; Souza et al., 2016; Behnke et al., 2021; Castellano-Hinojosa and Strauss, 2021). Changes in ecosystems by the development of cultures can alter the diversity and composition of microorganisms, favoring the selection of microbial communities that can harm humans. Thus, this work aims to describe the taxonomic and functional changes in the metagenomes of Amazonian soils after ecosystem disturbances for the development of pasture and transgenic soybean monoculture (in 2 and 14-year-old plantations) to elucidate the possible changes in the monocultures and the potential implications for ecosystem and environmental health.
2 Materials and methods
2.1 Description of the study sites
The study site is in the Amazon city of Paragominas-PA. The area is being explored for 35 years for intensive grain farming and beef cattle (Rodrigues et al., 2003). The predominant soil is Oxisol (Soil Survey Staff, 2014). The weather is Aw (Alvares et al., 2013) humid and hot tropical. The soil samples were collected at the beginning of February 2020, the plants were in the stage of the third and fourth fully developed trifoliate leaf. The information about the sites was described by those responsible for the farm in Table 1, four collection sites were separated, being secondary forest, pasture, soybean crop of 2 and 14 years.
2.2 Sample
Soil samples were collected in a flat, uniform area at a depth of up to 20 cm “topsoil” (Bach et al., 2018). At each location, three points were collected, a linear transect of 200 m was drawn, and at each point, five equidistant samples (50 m) were collected with the help of a Dutch auger that it was sterilized at each change of location (Carvalho et al., 2016), these samples were mixed at each point, obtaining a total of twelve samples divided into a part for chemical and molecular analysis. The fresh soil samples taken for molecular evaluation were stored in sterile falcon tubes with a capacity of 15 ml and deposited in liquid nitrogen immediately. Samples for chemical characterization were placed in clean plastic bags of approximately 500 g and kept at room temperature until analysis.
2.3 Soil chemical characterization
All analysis methods were performed as described by the Raij et al. (2001). Initially, the samples were dehydrated in an oven with air circulation at a temperature of 40 °C; then, they were sieved with a 2 mm mesh sieve. The pH was determined with the treatment of 0.01 M calcium chloride (CaCl2) and the content of organic matter (OM) by the colorimetric method. The levels of macronutrients such as phosphorus (P), potassium (K), and micronutrients such as boron (B), copper (Cu), iron (Fe), manganese (Mn), and zinc (Zn) were determined by atomic absorption spectrophotometry. The cations were extracted with the potassium chloride solution by the ion exchange process for the determination of the exchangeable cations aluminum (Al3+), calcium (Ca2+), and magnesium (Mg2+) for the determination of potassium, calcium, and magnesium, were used the EDTA titration method.
2.4 Total community DNA extraction
The samples were initially pre-treated with sodium phosphate buffer solution (500 mM Na2HPO4 and 500 mM NaH2PO4; pH 7.2) due to the high clay content. In a micro tube, 800 mg of sample were added to 1.2 ml of phosphate buffer solution, which was then mixed at 250 RPM for 5 min under a horizontal shaker. Immediately afterward, the microtube was incubated at 4°C for 3 min, later it was mixed for 5 min at 250 RPM and vortexed for 15 s, and centrifuged at 500 RPM for 10 min. The supernatant was transferred into a new tube (previously weighed), centrifuged at 10,000 RPM for 10 min, and the pellet was considered and stored at 4 °C. This procedure was repeated until 250 mg of soil was obtained (Högfors-Rönnholm et al., 2018).
The extraction of genetic material was performed with the DNA isolation kit Power Soil DNeasy (Qiagen, USA). Subsequently, the DNA concentrations were measured in the NanoDrop® spectrophotometer (Thermo Scientific), and the samples with concentrations ≥50 ng ml-1 and with purity indices (PI) between 1.8–2.0 (Figueroa et al., 2017) quality were evaluated by horizontal electrophoresis on 1% agarose gel with 0.5ug uL-1 ethidium bromide and stored at -20 °C for later use.
2.5 Sequencing and analysis of the metagenome community
For the construction of DNA libraries, the samples were treated according to the procedure with protocols from the manufacturer Nextera XT DNA Library (Illumina). Sequencing was performed with the NextSeq 550 System High-Output kit for a read size of 2 × 150 bp. The platform used was the Illumina NextSeq 500/550 HighOutput, with the entire procedure performed according to the manufacturer’s protocol at Instituto Evandro Chagas.
The analysis of the quality of the readings of each sequencing was visualized through the software FASTQC Version 0.11.9 (Andrew, 2019) and, later, the bases that had a quality value below PHRED 20 had their low-quality ends removed and filtered, using the Trimmomatic tool (Bolger et al., 2014). With the readings processed, the Kraken2 program (Wood et al., 2019) was used to analyze the diversity profile between each point to identify the domains of bacteria, archaea, viruses, protozoa, and fungi. Finally, for the visualization and comparative analysis of the reading sets of each of the replicas, the Paviam program was used (Breitwieser and Salzberg, 2020).
For the analysis of the diversity of the microorganisms identified, the program MicrobiomeAnalyst was used (Dhariwal et al., 2017; Chong et al., 2020). The data obtained in the analysis with Kraken2 were filtered to consider organisms with a minimum frequency (count) of 20%; later, the abundance was normalized with a trimmed mean of M-values (TMM). The alpha diversity indices were Rich, Chao1, Simpson, and Shannon, and ANOSIM was used to analyze the beta diversity. Previously, the OTU (operational taxonomic unit) was filtered to reduce noise, excluding those rare OTUs (occurring in less than three samples in total) and low abundance (less than three sequences per OTU).
2.6 Functional gene analysis
From the reading processed by Trimmomatic, the readings of each replica were assembled by the Megahit program (Li et al., 2015) with characteristic presets for mounting data from complex metagenomic environments such as soil (meta-large parameter); and the resulting contigs were analyzed using the Metaquast software (Mikheenko et al., 2016) to assess the quality of contigs generated after assembly. After that, the very short contigs, with sizes smaller than 350bp, were removed to decrease the chances of identifying false positives. Then the generated contigs were submitted to the public Meta Genome Rapid Annotation database using Subsystem Technology (MG-RAST) (Meyer et al., 2008); subsequently, functional genes were identified using the SEED Subsystems database, with an alignment of 90%, size 20 bp, e-value of 10−5.
2.7 Statistical analysis
The normality test was performed with Shapiro Wilk, with a significance of α = 0.05. The data obtained by chemical analysis (pH, organic matter, macro, micronutrients, and cations) were analyzed with ANOVA or Kruskal Wallis with the PAST program (Hammer et al., 2001).
Redundancy analysis (RDA) was used to evaluate the diversity index associated with chemical characteristics. In the case of functional genes, a treatment of relative abundances was carried out with the Orange Data Mine program (Demsar et al., 2013).
3 Results
3.1 Soil chemical characteristics
The chemical characteristics of the soil found in each location were different; in the case of pH, they were acidic, with significant differences (p values <0.05), the forest site with the lowest values compared to the others, but the site with tillage showed higher values due to the systematic use of fertilization and addition of lime. The organic matter content in the forest and pasture site was high and significant. There was a quantitative reduction of 56% and 52% in organic matter content at the 2-year-old and 14-year-old soybean sites compared to the forest site. The sites of pasture and cultured of soybean with 2 and 14 years showed high values of available phosphorus and micronutrients compared to the forest site, possibly resulted from the annual addition of chemical fertilizers. Regarding the concentrations of metals, high and significant values were observed in all places for B, Mn, and Zn. Cation concentration was significantly different between sites for H+Al (Table 2).
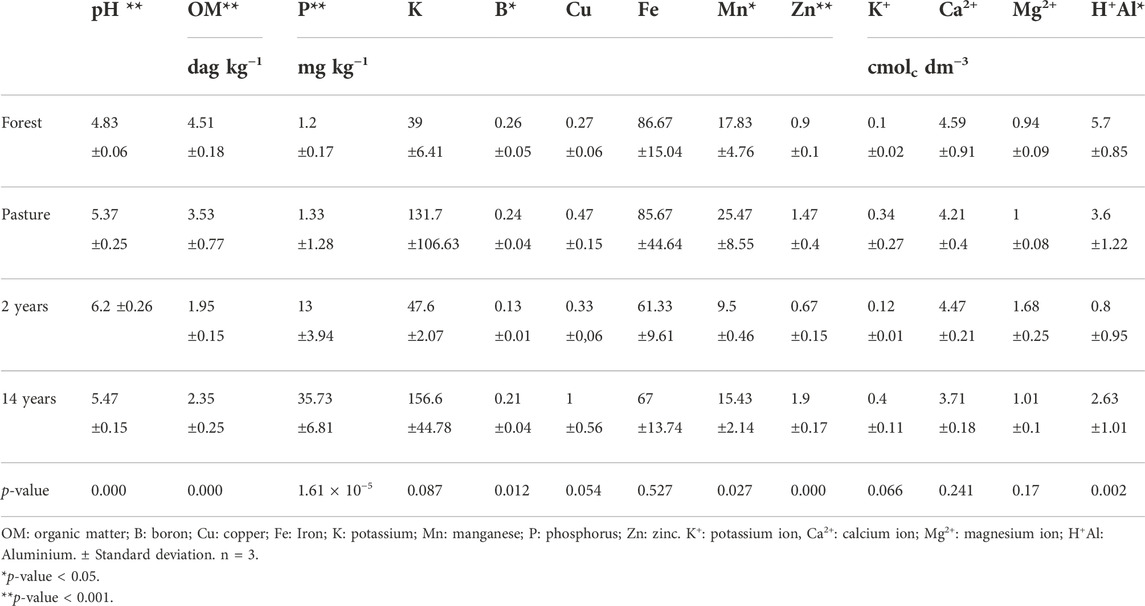
TABLE 2. Values of chemical analysis pH, organic matter, available macro, micronutrient, and soil cations at each site.
3.2 Composition of microorganism communities
Up to 6,153,723 readings were used to classify, of which they were used for the bacterial domain with up to 5,760,000 readings (96%), followed by the eukaryote domain with up to 128,601 readings, archaeas with up to 74,993 readings, and viruses with up to 2001 readings (supplementary figure 1).
The composition of the microorganism communities identified in the different sites varied in taxonomic classifications. According to the phylum, in bacteria, there was a more significant predominance of Proteobacteria and Actinobacteria, especially in the forest, representing 65% (Figures 1A–D), but without significant differences between the sites (Kruskal–Wallis; χ2 = 0.492; p-value = 0.921). In archaea, close percentages were observed (Kruskal–Wallis; χ2 = 0.189; p-value = 0.979), with a predominance of Euryarchaeota (82%) being more representative in the pasture site (Figures 1E–I). For viruses, they were similar in all locations (ANOVA; F = 1.34 × 10−30; p-value = 1), but a higher percentage of Uroviricota was observed in the pasture site, with 44% (Figure 1J-M). As for fungi, the most abundant phylum was Ascomycota, with 88% (figure 1N–Q).
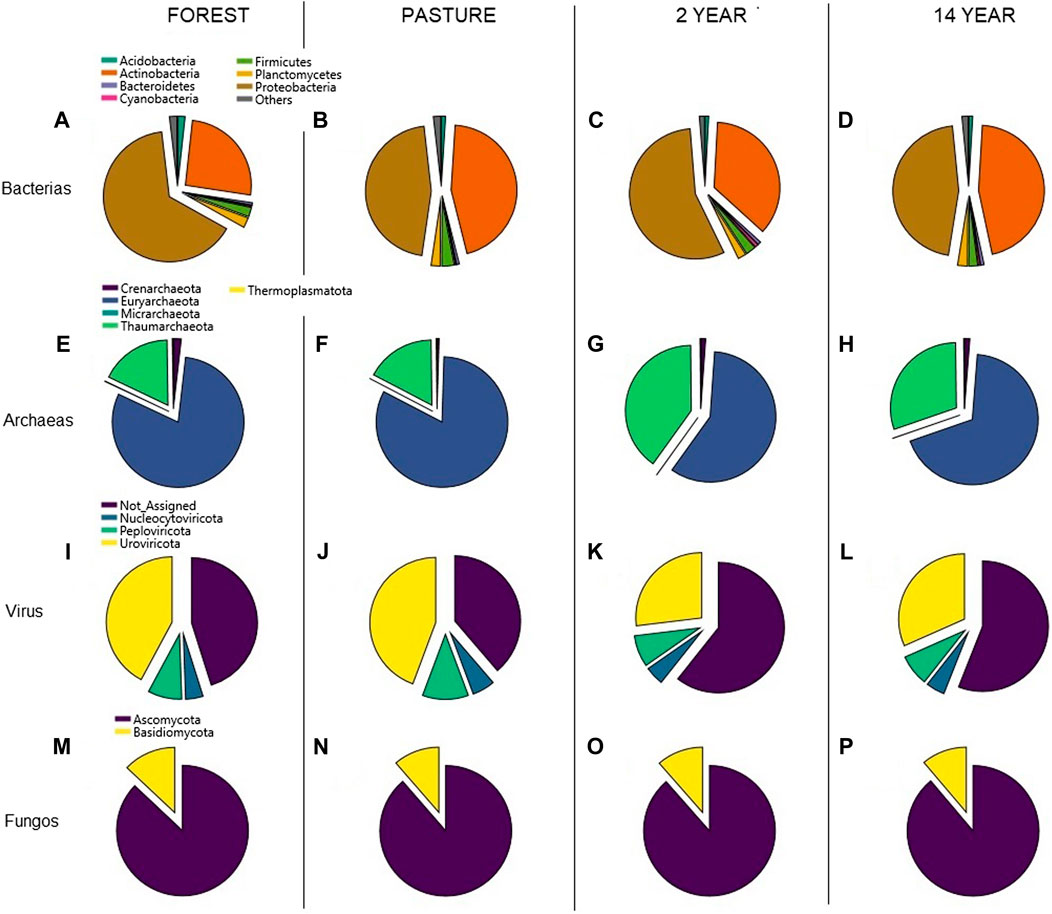
FIGURE 1. Abundance of the different phyla of the microorganisms identified in the forest sites corresponds to (A,E,I,M); pasture corresponds to (B,F,J,N); 2 years corresponds to (C,G,K,O); 14 years corresponds to (D,H,L,P).
Regarding the most predominant families, the bacteria Bradyrhizobiaceae, Enterobacteriaceae, Micromonosporaceae, Mycobacteriaceae, Nocardiodaceae, Pseudomonaceae, and Streptosporangiaceae were identified with greater abundance. In pasture and crop sites, an increase of up to 10% in the Enterobacteriaceae and Pseudomonaceae families was observed (supplementary figure 2A). For archaea, a different composition was observed between the sites, and the most abundant were Nitrososphaeraceae, Nitrialbaceae, Halorubraceae, Halobacteriaceae, Haloferacaceae, Haloarculaceae, with Methanosarcinaceae being more representative in the areas with crop and pasture (supplementary figure 2B). In the viruses, the presence of Siphoviridae in all sites was notable in the pasture site, and the presence of Demerecviridae in the forest site and the Bauloviridae family in the cultivated areas were also evidenced (supplementary figure 2C). Finally, as for the fungi, a similar composition was observed between the sites of the Chaetomiaceae, Glomerellaceae, Pyriculariaceae, Mycosphaerellaceae, and others (supplementary figure 2D).
The compositions of the genus identified in the different sites for bacteria with a different composition for the forest and the presence in greater abundance of Bradyrhizobium and Streptomyces are observed in all sites; relevance was evidenced in the sites of cultures of Enterobacter and Pseudomonas, in the pasture site in addition to of the above, Staphylococcus, Brucella and Klebsiella among others stand out (Figure 2A). In the case of archaea, the composition in the pasture site was different from the other sites, with a greater abundance of Methanosarcina, Methanocella, Candidatus Nitrosasphaera, and Nitrososphaera, especially in the forest site (Figure 2B). In viruses, the composition was grouped between the forest-pasture and crop sites with the highest abundance of unclassified genera and the presence of Alphabaculovirus and Timquatrovirus from the cultures and pasture sites (Figure 2C). Fungal species composition differed slightly in the forest site compared to the other cases (Figure 2D).
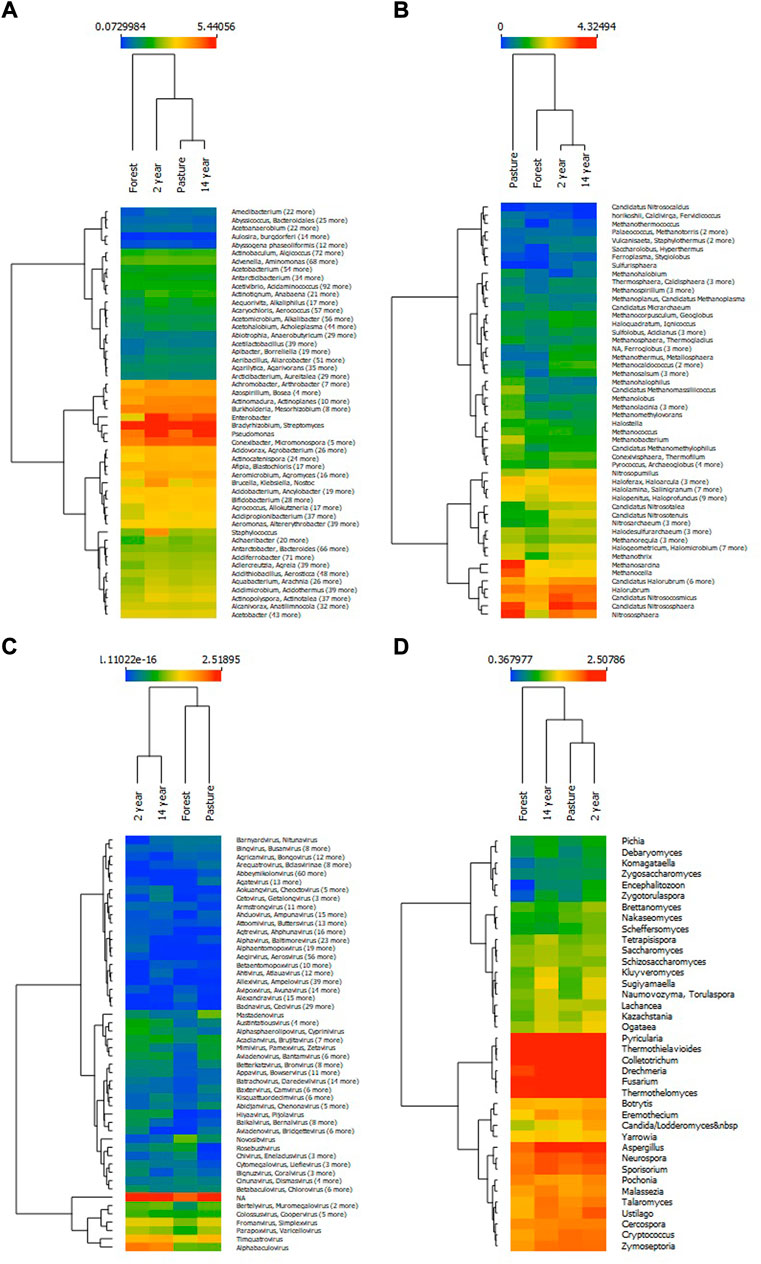
FIGURE 2. Heat map of genus abundance at each site of organisms (A) bacteria, (B) archaea, (C) virus and (D) fungi, grouped using the k-means algorithm.
The most representative species of microorganisms, starting from bacteria, are Bradyrhizobium sp and Rhodopseudomonas palusti in the forest site, and Streptomyces sp, Enterobacter cloacae and Pseudomonas aeruginosa species are more evident in the cultivated areas (Figure 3A). For archaea, the abundance of Methanosarcina barkeri, Nitrososphaera viennensis, M. mazei, and Candidatus Nitrosocosmicus evergladensis in the pasture site was more relevant; in the forest, Candidatus Nitrosocosmicus oleophilus, Halorubrum sp and Haloborum sp are relevant, in the case of the cultivated sites Candidatus Nitrosocos micus oleophilus and Nistrososphaera vienensis were more evident (Figure 3B), as for the viruses, different species of Pandoravirus sp and Caulobacter phage were identified in the forest, in the pasture site, the Ruegeria phage and Mycobacterium phage were more evident, as for the cultivated areas, the most predominant species were Pseudoplusia includens in addition to the above (Figure 3C). The microbial composition related to fungi showed similar proportions at different sites, with the most relevant species being of Thermothielaviodes terretris, Colletotrichum higginsianum, Drechmeria coniospora, and Thermothelomyce thermophilus (Figure 3D).
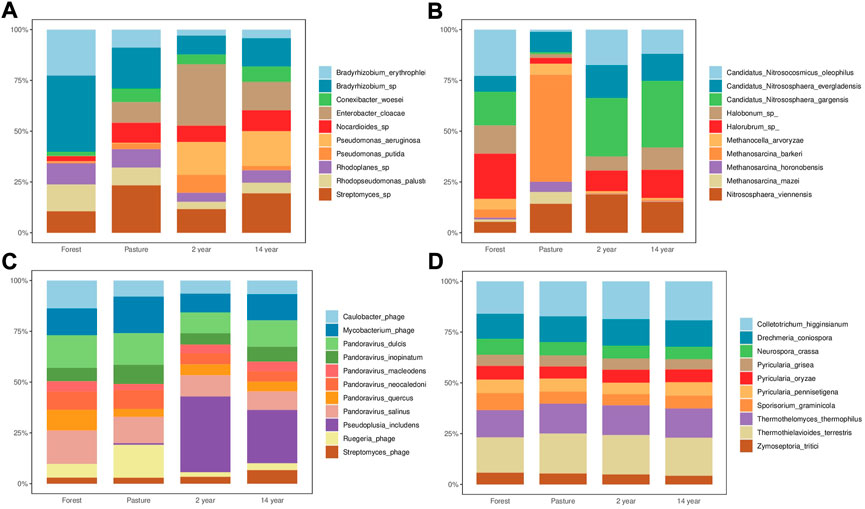
FIGURE 3. Abundance of the main species at each site of the organisms (A) bacteria, (B) archaea, (C) virus and (D) fungi.
3.3 Analysis of microbiological diversity
The analysis of diversity indices calculated in all sites showed no significant differences in the richness of microorganisms; in the bacteria domain, 5,619 taxas were identified in the pasture site that had the higher values of that domain, followed by the culture sites (2 and 14 years). In archaea, values between 251 and 281 taxas were identified in forest and pasture areas. In the case of viruses and fungi, lower counts were identified but they were higher in the culture and pastage sites. The estimate of the Chao one index was evidenced in bacteria of up to 99% of identified taxa, followed by fungi with up to 100%; in the case of archaea, values between 83 and 93% in viruses were lower compared to the previous between 79 and 88%. Simpson’s index was similar in different organisms, and representing high heterogeneity between sites; the values were higher in bacteria and fungi; in the case of archaea with the pasture site with lower values and in viruses in the 2-year site (Table 3).
Shannon’s index analysis showed high values in all areas; especially in bacteria; and the values for the other organisms were similar; in the case of archaea, there were significant differences between the sites (ANOVA; F = 24.006; p-value = 0.000), with the lowest values in the pasture site and the virus at the 2-year site (Table 3). The beta diversity index was evaluated with the analysis of ANOSIM, and a more significant difference in the composition of communities was evidenced in archaea, followed by viruses (Table 3). The principal coordinate analyzes (PCoA) of the replicas between the different organisms showed clusters of these for each site; in the case of archaeal, the replicas of the pasture site were grouped separately from the others (supplementary figure 3B). Contrary to what was observed with viruses, which showed a separation of sites with and without tillage (supplementary figure 3C), for bacteria and fungi, the replicas did not show different clusters (supplementary figures 3A and D).
The RDA analysis for each microorganism and the association of the physicochemical analysis, in the case of bacteria, the variables present an 84.84% variation between them, the H diversity in the forest and are associated with the content of MO, Mn, B, and cation Ca2+, but not associated with iron content (Figure 4A). In archaea, the variation of the variables is 90.21%, and the pasture site is more represented by the association of the variables of Mn, K, Zn, and K+ cation (Figure 4B). In the case of viruses and fungi the variation of the variables was less than 50% (Figures 4C,D).
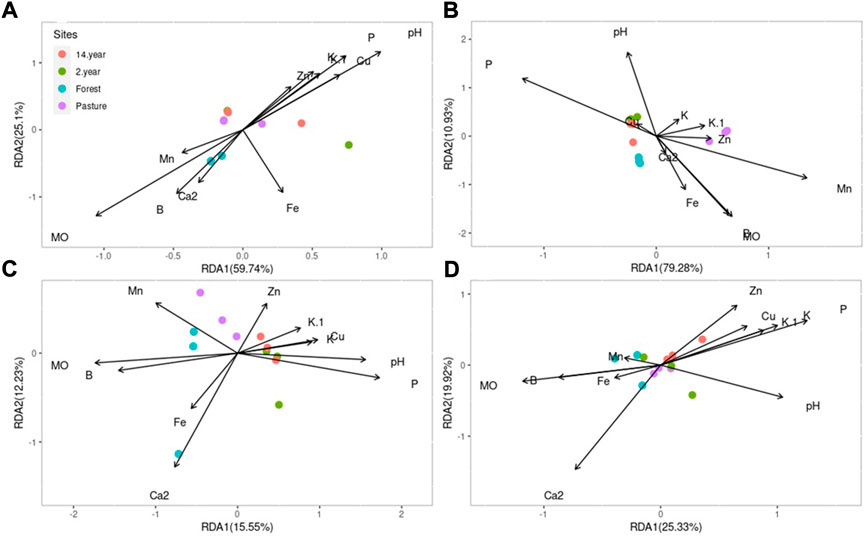
FIGURE 4. RDA analysis of chemical variables and Shannon’s alpha diversity index at all sites each site of the organisms (A) bacteria, (B) archaea, (C) virus and (D) fungi.
3.4 Analysis of functional gene annotation
The analysis of the composition of the CDS according to level 1 of the SEED subsystem database for the different sites showed variations in relative abundance but without significant differences between them (Kruskal–Wallis; χ2 = 5.823; p-value = 0.12). The forest site compared to the pasture and crop sites showed a difference in the composition of the genes; in addition, the grouping by relative abundance and the design of the genes revealed the presence of three groups: low, moderate, and high relative abundance of the genes. In the low proportion group, the genes associated with photosynthesis, dormancy, and secondary metabolism were identified; in the moderate abundance group, genes related to metabolism of fatty acids, lipids and isoprenoids, sulfur, nitrogen, and aromatic compounds, cell regulation and division, in addition to the presence of mobile elements; with the exception of the genes for iron and potassium metabolism that were in the low-abundance group for the forest. In the group with the high relative abundance, genes related to cellular homeostasis, stress, carbohydrate metabolism, metabolism of proteins, DNA and RNA were found. A critical point observed in the forest site was the low abundance of genes involved with virulence, diseases, and defense, cell wall, capsule, and membrane transport in contrast to the other sites (Figure 5).
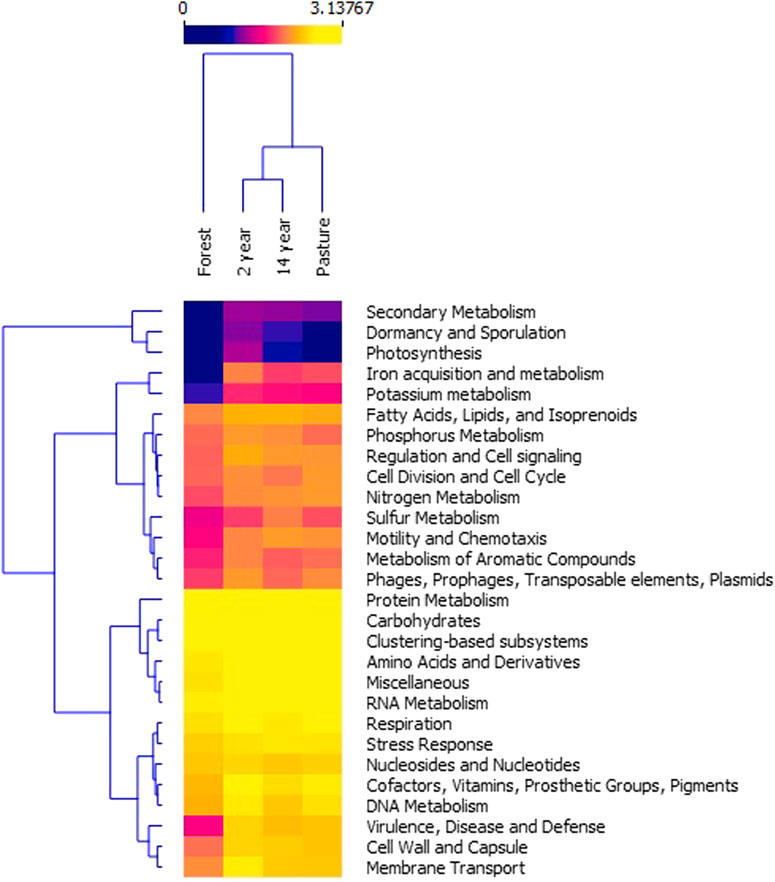
FIGURE 5. Heatmap of the logarithm of the relative abundance of the functional annotations identified in the sites, using level 1 of the SEED Subsystems database. Permission to reuse and Copyright.
4 Discussion
4.1 Chemical characteristics and microbial communities
Soil is a complex matrix due to its chemical composition, the presence of different organisms, environmental conditions, contaminants, and other factors that influence biodiversity and co-exist for the development of biogeochemical processes for the maintenance of the ecosystem (Evans and Wallenstein, 2014; Maron et al., 2018; Lacerda-Júnior et al., 2019). The soils of the Amazon show particular chemical characteristics in each region (Souza et al., 2016; Costa et al., 2020); in the case of the municipality of Paragominas, the soil is characterized by a considerable content of clay (approx. 70%) which would induce the provision of nutrients (Ferraz et al., 2013; Laurent et al., 2017), by the interactions of organic matter with soil minerals, called organo-minerals and provide the generation of microaggregates, consisting mainly of bacteria, followed by viruses, archaea, and fungi (Cuadros, 2017; Mandakovic et al., 2018; Totsche et al., 2018; Kome et al., 2019; Quesada et al., 2020).
On the other hand, the different practices that are carried out for land use in intensive agriculture contribute to the alteration and reduction of the reserve of organic matter and the diversity of the communities of organisms that reside in the soil (Carvalho et al., 2016; Santos, 2019; García et al., 2020), At the site where the samples were collected, the forest was initially removed, and limestone, chemical fertilizers, and pesticides were applied for the cultivation of transgenic soybeans, thus, the long-term application of chemical fertilizers alters the chemical characteristics and reduces the availability of some nutrients (Gupta et al., 2022). As a result, the presence of these compounds in cultivated soil decreases microbial activity, in contrast to what happens in sites with high vegetation cover (Bevivino et al., 2014; Kim et al., 2020), which would explain the greater abundance and diversity of microorganisms in the forest compared to pasture and crop soils, a decrease and displacement of bacterial communities were evidenced and these microorganisms pose adaptation mechanisms against disturbances (Liu et al., 2013; Sun and Badgley, 2019; Moroenyane et al., 2021).
Soil microbial communities are dynamic and sensitive to biotic and abiotic changes, but it is still possible to evidence the presence of different most relevant taxonomic groups in each site; in the forest, the dominant phyla were Proteobacteria, followed by Actinobacteria, which are usually found in these tropical regions (Sugiyama et al., 2014; Lin et al., 2019), in the case of pasture and culture sites with transgenic soybean, the increased abundance of the Actinobacteria phylum, might be involved with changes in soil pH (Navarrete et al., 2015a; Navarrete et al., 2015b; Durrer et al., 2021), in pastures, in addition to the microorganisms mentioned, the Firmicutes phylum is frequently reported in this type of grass places (Lladó et al., 2018; Santos, 2019).
In the case of the genus, the presence of bacteria that promote plant growth was relevant, especially in the forest site; in the other places, bacteria were also identified that are involved in the cultivation of soy, corn, sugarcane (Sugiyama et al., 2014; Khalifa et al., 2016), particularly in the genera identified in soybean crops, the genus of Enterobacter, Acinetobacter, Pseudomonas, Ochrobactrum, Rhizobium, Bradyrhizobium, Klebsiella and Bacillus have been frequently reported to perform different functions, such as nitrogen fixation, phosphorus mobilization, salt stress tolerance, phytohormone production, and production of defenses against pathogens (Mahartha and Suprapta, 2018; Zhao et al., 2018). Nevertheless, some of these species are considered opportunistic pathogens for humans and animals and have clinical importance due to their presence of multi-resistant antibiotic genes (De Oliveira et al., 2020; Girlich et al., 2021; Martins et al., 2021); in this work the increase in the abundance of the species Enterobacter cloacae and Pseudomonas aeruginosa in cultivated and pasture soils was relevant, in the case of cultivated sites no organic fertilizer was applied, and the increase in these pathogens could be related to the presence of glyphosate used in the cultivation of transgenic soybeans (Kepler et al., 2020).
On the other hand, the composition of the archaea diversity was relevant to microorganisms involved in the nitrogen cycle and methane production (Lehtovirta-Morley et al., 2016; Kroeger et al., 2021; Zhou et al., 2021), these were dominant in pasture and cultural sites. However, it was evidenced that particular communities in the pasture site, such as M. barkeri and M. horonobensis, could be considered bioindicators for this type of site. In addition, the presence of haloarchaea suggests the relevance of using chemical fertilizers for the accumulation of salts (Zhalnina et al., 2013; Torregrosa-Crespo et al., 2018; Behnke et al., 2021), but in the forest site, the presence of these organisms could be associated with the naturally high levels of iron and potassium salts.
The most relevant viruses identified on cultivated areas were species of Pseudoplusia includens, which are pathogens of the insects Chrysodeixis includen, these are parasites of the soybean crop (Alexandre et al., 2010; Craveiro et al., 2015; Harrison et al., 2019) the presence of these viruses can be considered as biopesticides and/or pest controllers in crops. Pandoraviruses, which were identified in the four sites, are giant viruses and are present in high diversity in soil and water, and can infect protozoa and amoebas (Abergel et al., 2015; Aherfi et al., 2016; Colson et al., 2017; Andradedos et al., 2018; Schulz et al., 2018; Akashi and Takemura, 2019; Tokarz-DeptułaBeata et al., 2019). Also, analysis of the composition of functional genes in these sites at levels 2 and 3 showed a greater relative abundance of genes involved in the lytic cycle, so it could be a greater abundance of prophages in the forest and phages than in the sites with culture (data not shown).
In the case of the fungal species, the composition of the communities found in this study was similar in all sites, but in other studies, heterogeneity was evidenced in places with and without cultivation, with saprophytic and pathogenic fungi being found (Souza et al., 2016; Bach et al., 2018; Checinska Sielaff et al., 2018) this could be an influence of the reduced number of species identified for this type of organisms in contrast to the others. The most abundant fungal species in this study are known for their lignocellulolytic capacity (Velasco et al., 2020) it was also; identified the presence of fungi capable of developing the disease in the plant, such as anthracnose (O’Connell et al., 2012; Yan et al., 2018), as well as the presence of organisms of the genus Fusarium, Aspergillus, Neurospora among others (Huang et al., 2017; Panelli et al., 2017; Behnke et al., 2021). Thus, soil management to eliminate vegetation cover through the fire, as was initially carried out in cultivation and pasture sites, would increase the presence of fungi, as well as Neurospora crassa, which can affect crop development and human health (Kuo et al., 2015; Lebrigand et al., 2016; Nourrisson et al., 2017).
The disturbances caused by the management of the culture affect the composition of the microorganisms and favor their changes, increasing the selection of organisms with better adaptation to the type of culture (Calderón et al., 2018). The composition of the different microbial communities was divergent between the sites. The forest site was most affected by the displacement of these native communities and the increase of other multi-species associations that would be adapted to these sites.
4.2 Chemical factors and microbial diversity
The type of soil management influences the microbiome composition in cultures, but the different microorganisms were responses vary (Longley et al., 2020; Köberl et al., 2020). The chemical fertilizers and pesticides used for this soybean seed influence the structure of the bacterial community (Zeng et al., 2019; Li et al., 2020; Chen X. et al., 2021); in soils with longer crop grown periods, bacterial and fungal diversity were generally low (Hamid et al., 2017; Dube et al., 2019).
The use of Shannon’s alpha diversity index (H) can be considered a sensitive indicator to assess anthropogenic disturbances in contrast to others (Bobul’Ská et al., 2021; Lan et al., 2017), as observed in this work, tracking the oscillations of this microbial diversity index due to the effect of different chemical factors could be an essential tool to assess the impact of soil management.
Likewise, pH was one of the main factors associated with changes in bacterial and fungal communities. (Lin et al., 2019; Chen Y.-P. et al., 2021). On the other hand, it was evidenced that the function of the virus communities would be related to the electrical conductivity of the soil; it is strongly associated with their dynamics with the regulation of bacterial communities (Seaton et al., 2022), the measurement could be considered in future works of this fact and the diversity of the phages.
In this work, the organic matter did not have a strong influence on the alpha diversity index, as reported by Lin et al. (2019) could be due to the richness in biodiversity of the place, in addition to the interaction of organic matter with soil minerals with the help of cations, favoring the preservation of the diversity of microorganisms (Aboudi Mana et al., 2017; Totsche et al., 2018).
Additionally, in this type of soil, cations can favor the adaptation of halotolerant organisms and help increase virus diversity (Kome et al., 2019; Rieke et al., 2022). Because according to Hua et al. (2021), the presence of phages can contribute to the increase of extracellular DNA and, together with the presence of phosphorus salts in the soil, influence the diversity of these microbial communities.
On the other hand, in our work, negative associations between alpha diversity indices and copper and potassium content were not evidenced since the accumulation of these elements is toxic to some microorganisms (Li et al., 2021; Salah et al., 2021), in the case of native organisms residing in the forest, as well as the diversity of bacteria that were affected with the levels of these elements, but it was not observed with the iron content.
However, chemical fertilizers are added to the crop sites and are correlated with the stability of microbial diversity; fungi were more sensitive than bacteria (Wipf et al., 2021). Using these compounds increases the levels of K and P in the soil, which are necessary for the growth of the organisms found on these sites (Chen Y.-P. et al., 2021), at work were especially suitable for archaea, fungi and viruses.
4.3 Characterization of functional genes
The high relative abundance of genes associated with metabolism reflects the metabolic redundancy in the different sites, which contributes to the preservation of the functionality of bacterial communities in the ecosystem (Maron et al., 2018; Jiao et al., 2019; Chen Y.-P. et al., 2021), despite the difference in the gene composition of the forest with the other sites. In the soil of the Amazon, due to the particularity of the ecosystem, the principal activity is the metabolism of carbohydrates, such as beta-glycosides (Bobul’Ská et al., 2021).
In the group with the highest relative abundance of functional genes, pasture and crop sites with genes associated with virulence and defense could be a response to stress and adaptation by microorganisms. In this way, a point to be considered is the accumulation of salts and other compounds by the use of chemical products, favoring the increase of these resistance mechanisms, as well as the ABC membrane transport proteins for the importation of available substrates, such as inorganic and organic ions, mono and oligosaccharides, amino acids and peptides (Liu et al., 2013; Ramesh et al., 2014; Chen et al., 2018; Ji et al., 2020). In the case of genes associated with iron and potassium metabolism, at the forest site, they were low at the other sites; thus, we can suggest that there is the presence of other components and/or mechanisms capable of protecting microorganisms from high levels of these elements. Just like that, the complex composition and interaction of organisms in this ecosystem limits the increase of these genes, but this mechanism is still not well understood (Doonan et al., 2020).
In forest soil, bacterial populations are mostly oligotrophic with a low replication rate (r-strategist), and in pasture and crop soils, the presence of copiotrophic populations increases, capable of increasing metabolic and replication rates (k-strategist) (Maron et al., 2018; Dube et al., 2019; Lacerda-Júnior et al., 2019), which would be represented in the difference in the relative abundance of genes associated with DNA metabolism between the sites.
Likewise, in the group of genes with moderate abundance, the presence of functions related to secondary metabolisms, such as those associated with the metabolism of nitrogen, aromatic compounds, and sulfur, in addition to the presence of mobile genetic elements, was highlighted. Sites, which reflect the versatility of genomes for the development of different functional mechanisms (Fuentes-Lara et al., 2019; Pedrinho et al., 2019), are responsible for nitrogen fixation and nutrient uptake from the soil (VanInsberghe et al., 2015; Araujo et al., 2017; Leite et al., 2018; Martins da Costa et al., 2018; Htwe et al., 2019).
A better understanding of agricultural management and the influence on soil microbial communities will help direct a microbiota to a desired beneficial state, which can be leveraged to improve the development of region-appropriate agricultural practices and, more satisfactorily, crop performance (Fernandez-Gnecco et al., 2022). The effects of organic farming/fertilization, cropping systems, crop rotation, and correction additives should be considered in future studies to improve favorable microbiomes (Chen et al., 2018). Techniques such as reforestation and crop rotation can be an excellent alternative to help maintain the health of the soil, in addition to contributing to the reduction of pathogenic organisms (Rascovan et al., 2016; Curd et al., 2018; Jiao et al., 2018; Neupane et al., 2021).
5 Conclusion
The clayey soils of the Amazon presented a characteristic behavior in the forest, pasture, and soybean fields, and it was observed that the formation of microaggregates by the organo-mineral interaction allows the accumulation of organic matter and favors the maintenance of microbial diversity. Soil management through practices such as the addition of lime, chemical fertilizers, and the use of pesticides in the samples in this study did not show a negative influence on the alpha diversity index of microbial communities, but it is suggested that cations and pH in these types of soils are more relevant than others. The compositions of the most pertinent microorganisms were the archaea communities that were dominant in the pasture site and the predominance of bacterial communities of Enterobacter cloacae and species of Pseudomonas, Pseudoplusia includens, Methanosarcina barkeri in pasture and crop sites. The complexity of interactions in the forest reflects soil quality and human health protection since species considered pathogenic in humans were identified in lower abundance. Finally, we can infer that the electrodynamics of some ions in these soils can influence the biological dynamics and should be better clarified.
Data availability statement
The datasets presented in this study can be found in online repositories. The names of the repository/repositories and accession number(s) can be found below: https://www.ncbi.nlm.nih.gov/, PRJNA841936.
Author contributions
OC performs the collection of samples, laboratory analysis, analysis of results and writing. CD and RR perform the analysis of bioinformatic data, ES collaborates in the collection of samples and chemical analysis, CS helps with laboratory methods, SD and AR perform sequencing analyses. AC structure the article and help with the writing.
Acknowledgments
Our thanks for the funded National Research Council (CNPq), Alliance Program for Education and Training—PAEC-OEA-GCUB 2017, within the scope of the Cooperation Agreement between the Organization of American States (OAS) and the Coimbra Group of Brazilian Universities (CGUB); L'Oréal Brasil-UNESCO-ABC “For Women in Science”. We thank the PROPESP/UFPA (Pró-Reitoria de Pesquisa e Pós-Graduação/Universidade Federal do Pará for the financial support on this work.
Conflict of interest
The authors declare that the research was conducted in the absence of any commercial or financial relationships that could be construed as a potential conflict of interest.
Publisher’s note
All claims expressed in this article are solely those of the authors and do not necessarily represent those of their affiliated organizations, or those of the publisher, the editors and the reviewers. Any product that may be evaluated in this article, or claim that may be made by its manufacturer, is not guaranteed or endorsed by the publisher.
Supplementary material
The Supplementary Material for this article can be found online at: https://www.frontiersin.org/articles/10.3389/fenvs.2022.1024610/full#supplementary-material
References
Abergel, C., Legendre, M., and Claverie, J.-M. (2015). The rapidly expanding universe of giant viruses: Mimivirus, Pandoravirus, Pithovirus and Mollivirus. FEMS Microbiol. Rev. 39, 779–796. doi:10.1093/femsre/fuv037
Aboudi Mana, S. C., Hanafiah, M. M., and Chowdhury, A. J. K. (2017). Environmental characteristics of clay and clay-based minerals. Geol. Ecol. Landscapes 1, 155–161. doi:10.1080/24749508.2017.1361128
Aherfi, S., Colson, P., La Scola, B., and Raoult, D. (2016). Giant viruses of amoebas: An update. Front. Microbiol. 7, 349. doi:10.3389/fmicb.2016.00349
Akashi, M., and Takemura, M. (2019). Co-isolation and characterization of two pandoraviruses and a mimivirus from a riverbank in Japan. Viruses 11, 1123. doi:10.3390/v11121123
Alexandre, T. M., Ribeiro, Z. M. A., Craveiro, S. R., Cunha, F., Fonseca, I. C. B., Moscardi, F., et al. (2010). Evaluation of seven viral isolates as potential biocontrol agents against Pseudoplusia includens (Lepidoptera: Noctuidae) caterpillars. J. Invertebr. Pathology 105, 98–104. doi:10.1016/j.jip.2010.05.015
Alvares, C. A., Stape, J. L., and Sentelhas, P. C. (2013). Ko¨ppen’s climate classification map for Brazil. Meteorol. Z. 19 . doi:10.1127/0941-2948/2013/0507
Alves, L. W. R., Carvalho, E. J. M., and Silva, L. G. T. (2014). Boletim de Pesquisa e Desenvolvimento 9. Diagnóstico agrícola do município de Paragominas, PA, 28.
Andrade, A. C., dos, S. P., Arantes, T. S., Rodrigues, R. A. L., Machado, T. B., Dornas, F. P., et al. (2018). Ubiquitous giants: A plethora of giant viruses found in Brazil and Antarctica. Virol. J. 15, 22. doi:10.1186/s12985-018-0930-x
Andrew, S. (2019). FastQC. Babraham Bioinforma. Available at: http://www.bioinformatics.babraham.ac.uk/projects/fastqc/.
Araujo, R. S., Cruz, S. P. da, Souchie, E. L., Martin, T. N., Nakatani, A. S., Nogueira, M. A., et al. (2017). Preinoculation of soybean seeds treated with agrichemicals up to 30 Days before sowing: Technological innovation for large-scale Agriculture. Int. J. Microbiol. 2017, 1–11. doi:10.1155/2017/5914786
Bach, E. M., Williams, R. J., Hargreaves, S. K., Yang, F., and Hofmockel, K. S. (2018). Greatest soil microbial diversity found in micro-habitats. Soil Biol. Biochem. 118, 217–226. doi:10.1016/j.soilbio.2017.12.018
Battisti, R., Sentelhas, P. C., Pascoalino, J. A. L., Sako, H., de Sá Dantas, J. P., and Moraes, M. F. (2018). Soybean yield gap in the areas of yield contest in Brazil. Int. J. Plant Prod. 12, 159–168. doi:10.1007/s42106-018-0016-0
Behnke, G. D., Kim, N., Zabaloy, M. C., Riggins, C. W., Rodriguez-Zas, S., and Villamil, M. B. (2021). Soil microbial indicators within rotations and tillage systems. Microorganisms 9, 1244. doi:10.3390/microorganisms9061244
Berg, G., Erlacher, A., Smalla, K., and Krause, R. (2014). Vegetable microbiomes: Is there a connection among opportunistic infections, human health and our ‘gut feeling. Microb. Biotechnol. 7, 487–495. doi:10.1111/1751-7915.12159
Bolger, A. M., Lohse, M., and Usadel, B. (2014). Trimmomatic: A flexible trimmer for Illumina sequence data. Bioinformatics 30, 2114–2120. doi:10.1093/bioinformatics/btu170
Bordonal, R. de O., Carvalho, J. L. N., Lal, R., de Figueiredo, E. B., de Oliveira, B. G., and La Scala, N. (2018). Sustainability of sugarcane production in Brazil. A review. Agron. Sustain. Dev. 38, 13. doi:10.1007/s13593-018-0490-x
Breitwieser, F. P., and Salzberg, S. L. (2020). Pavian: Interactive analysis of metagenomics data for microbiome studies and pathogen identification. Bioinformatics 36, 1303–1304. doi:10.1093/bioinformatics/btz715
Brevik, E. C., Slaughter, L., Singh, B. R., Steffan, J. J., Collier, D., Barnhart, P., et al. (2020). Soil and human health: Current status and future needs. Air, Soil Water Res. 13, 117862212093444. doi:10.1177/1178622120934441
Calderón, K., Philippot, L., Bizouard, F., Breuil, M.-C., Bru, D., and Spor, A. (2018). Compounded disturbance chronology modulates the resilience of soil microbial communities and N-cycle related functions. Front. Microbiol. 9, 2721. doi:10.3389/fmicb.2018.02721
Carvalho, T. S., Jesus, E. da C., Barlow, J., Gardner, T. A., Soares, I. C., Tiedje, J. M., et al. (2016). Land use intensification in the humid tropics increased both alpha and beta diversity of soil bacteria. Ecology 97, 2760–2771. doi:10.1002/ecy.1513
Castellano-Hinojosa, A., and Strauss, S. L. (2021). Insights into the taxonomic and functional characterization of agricultural crop core rhizobiomes and their potential microbial drivers. Sci. Rep. 11, 10068. doi:10.1038/s41598-021-89569-7
Cattelan, A. J., and Dall’Agnol, A. (2018). The rapid soybean growth in Brazil. OCL 25, D102. doi:10.1051/ocl/2017058
Chaparro, J. M., Sheflin, A. M., Manter, D. K., and Vivanco, J. M. (2012). Manipulating the soil microbiome to increase soil health and plant fertility. Biol. Fertil. Soils 48, 489–499. doi:10.1007/s00374-012-0691-4
Checinska Sielaff, A., Upton, R. N., Hofmockel, K. S., Xu, X., Polley, H. W., and Wilsey, B. J. (2018). Microbial community structure and functions differ between native and novel (exotic-dominated) grassland ecosystems in an 8-year experiment. Plant Soil 432, 359–372. doi:10.1007/s11104-018-3796-1
Chen, H., Xia, Q., Yang, T., and Shi, W. (2018). Eighteen-year farming management moderately shapes the soil microbial community structure but promotes habitat-specific taxa. Front. Microbiol. 9, 1776. doi:10.3389/fmicb.2018.01776
Chen, Q.-L., Cui, H.-L., Su, J.-Q., Penuelas, J., and Zhu, Y.-G. (2019). Antibiotic resistomes in plant microbiomes. Trends Plant Sci. 24, 530–541. doi:10.1016/j.tplants.2019.02.010
Chen, X., Wicaksono, W. A., Berg, G., and Cernava, T. (2021a). Bacterial communities in the plant phyllosphere harbour distinct responders to a broad-spectrum pesticide. Sci. Total Environ. 751, 141799. doi:10.1016/j.scitotenv.2020.141799
Chen, Y.-P., Tsai, C.-F., Rekha, P. D., Ghate, S. D., Huang, H.-Y., Hsu, Y.-H., et al. (2021b). Agricultural management practices influence the soil enzyme activity and bacterial community structure in tea plantations. Bot. Stud. 62, 8. doi:10.1186/s40529-021-00314-9
Chong, J., Liu, P., Zhou, G., and Xia, J. (2020). Using MicrobiomeAnalyst for comprehensive statistical, functional, and meta-analysis of microbiome data. Nat. Protoc. 15, 799–821. doi:10.1038/s41596-019-0264-1
Colin, Y., Nicolitch, O., Van Nostrand, J. D., Zhou, J. Z., Turpault, M.-P., and Uroz, S. (2017). Taxonomic and functional shifts in the beech rhizosphere microbiome across a natural soil toposequence. Sci. Rep. 7, 9604. doi:10.1038/s41598-017-07639-1
Colson, P., La Scola, B., and Raoult, D. (2017). Giant viruses of amoebae: A journey through innovative research and paradigm changes. Annu. Rev. Virol. 4, 61–85. doi:10.1146/annurev-virology-101416-041816
Colussi, J., and Schnitkey, G. (2021). New soybean record: Historical growing of production in Brazil. FarmcdocDaily 11, 4.
Costa, A. C. S. da, Souza, I. G. de, Canton, L. C., Gil, L. G., and Figueiredo, R. (2020). Contribution of the chemical and mineralogical properties of sandy-loam tropical soils to the cation exchange capacity. Rev. Bras. Ciência do Solo 44, e0200019. doi:10.36783/18069657rbcs20200019
Craveiro, S. R., Inglis, P. W., Togawa, R. C., Grynberg, P., Melo, F. L., Ribeiro, Z. M. A., et al. (2015). The genome sequence of Pseudoplusia includens single nucleopolyhedrovirus and an analysis of p26 gene evolution in the baculoviruses. BMC Genomics 16, 127. doi:10.1186/s12864-015-1323-9
Cuadros, J. (2017). Clay minerals interaction with microorganisms: A review. Clay Min. 52, 235–261. doi:10.1180/claymin.2017.052.2.05
Curd, E. E., Martiny, J. B. H., Li, H., and Smith, T. B. (2018). Bacterial diversity is positively correlated with soil heterogeneity. Ecosphere 9. doi:10.1002/ecs2.2079
De Oliveira, D. M. P., Forde, B. M., Kidd, T. J., Harris, P. N. A., Schembri, M. A., Beatson, S. A., et al. (2020). Antimicrobial resistance in ESKAPE pathogens. Clin. Microbiol. Rev. 33, 001811-19–e219. doi:10.1128/CMR.00181-19
Demsar, J., Curk, T., Erjavec, A., Demsar, J., Curk, T., Erjave, A., et al. (2013). Orange: Data mining toolbox in Python. J. Mach. Learn. Res. 4, 5.
Dhariwal, A., Chong, J., Habib, S., King, I. L., Agellon, L. B., and Xia, J. (2017). MicrobiomeAnalyst: A web-based tool for comprehensive statistical, visual and meta-analysis of microbiome data. Nucleic Acids Res. 45, W180–W188. doi:10.1093/nar/gkx295
Doonan, J. M., Broberg, M., Denman, S., and McDonald, J. E. (2020). Host–microbiota–insect interactions drive emergent virulence in a complex tree disease. Proc. R. Soc. B 287, 20200956. doi:10.1098/rspb.2020.0956
Döring, T. F., Rosslenbroich, D., Giese, C., Athmann, M., Watson, C., Vágó, I., et al. (2020). Disease suppressive soils vary in resilience to stress. Appl. Soil Ecol. 149, 103482. doi:10.1016/j.apsoil.2019.103482
Dube, J. P., Valverde, A., Steyn, J. M., Cowan, D. A., and van der Waals, J. E. (2019). Differences in bacterial diversity, composition and function due to long-term agriculture in soils in the eastern free state of South Africa. Diversity 11, 61. doi:10.3390/d11040061
Durrer, A., Gumiere, T., Rumenos Guidetti Zagatto, M., Petry Feiler, H., Miranda Silva, A. M., Henriques Longaresi, R., et al. (2021). Organic farming practices change the soil bacteria community, improving soil quality and maize crop yields. PeerJ 9, e11985. doi:10.7717/peerj.11985
Evans, S. E., and Wallenstein, M. D. (2014). Climate change alters ecological strategies of soil bacteria. Ecol. Lett. 17, 155–164. doi:10.1111/ele.12206
Fernandez-Gnecco, G., Covacevich, F., Consolo, V. F., Behr, J. H., Sommermann, L., Moradtalab, N., et al. (2022). Effect of long-term agricultural management on the soil microbiota influenced by the time of soil sampling. Front. Soil Sci. 2, 837508. doi:10.3389/fsoil.2022.837508
Ferraz, A. P. F., Souza, R. M. S., de, E. S., Antonino, A. C. D., and Pragana, B. (2013), Efeito da umidade e densidade do solo na resistência a penetração de um solo de textura Areia Franca. Florianópolis SC: XXIV Congresso Brasileiro de Ciências do Solo.
Figueroa, D., Duval, P., Calle, R., Xiomara, D., Alegría, C., Aliaga, Á., et al. (2017). Establecimiento y evaluación de dos métodos de pre tratamiento de muestras de suelo para la extracción de ADN para el estudio de la diversidad bacteriana. Rev. Con-Ciencia 5, 11–25.
Fitzpatrick, C. R., Salas-González, I., Conway, J. M., Finkel, O. M., Gilbert, S., Russ, D., et al. (2020). The plant microbiome: From ecology to reductionism and beyond. Annu. Rev. Microbiol. 74, 81–100. doi:10.1146/annurev-micro-022620-014327
Fuentes-Lara, L., Medrano-Macías, J., Pérez-Labrada, F., Rivas-Martínez, E., García-Enciso, E., González-Morales, S., et al. (2019). From elemental sulfur to hydrogen sulfide in agricultural soils and plants. Molecules 24, 2282. doi:10.3390/molecules24122282
Garcia, S. N., Osburn, B. I., and Jay-Russell, M. T. (2020). One health for food safety, food security, and sustainable food production. Front. Sustain. Food Syst. 4, 1. doi:10.3389/fsufs.2020.00001
Gardner, C. J., Bicknell, J. E., Baldwin-Cantello, W., Struebig, M. J., and Davies, Z. G. (2019). Quantifying the impacts of defaunation on natural forest regeneration in a global meta-analysis. Nat. Commun. 10, 4590. doi:10.1038/s41467-019-12539-1
Girlich, D., Ouzani, S., Emeraud, C., Gauthier, L., Bonnin, R. A., Le Sache, N., et al. (2021). Uncovering the novel Enterobacter cloacae complex species responsible for septic shock deaths in newborns: A cohort study. Lancet Microbe 2, e536–e544. doi:10.1016/S2666-5247(21)00098-7
Hamid, M. I., Hussain, M., Wu, Y., Zhang, X., Xiang, M., and Liu, X. (2017). Successive soybean-monoculture cropping assembles rhizosphere microbial communities for the soil suppression of soybean cyst nematode. FEMS Microbiol. Ecol. 93, fiw222. doi:10.1093/femsec/fiw222
Hammer, O., Harper, D. A. T., and Ryan, P. D. (2001). Past: Paleontological statistics software package for education and data analysis. Palaeontol. Electron. 4, 9. Available at: http://palaeo-electronica.org/2001_1/past/issue1_01.htm.
Harrison, R. L., Rowley, D. L., and Popham, H. J. R. (2019). A novel Alphabaculovirus from the soybean looper, Chrysodeixis includens, that produces tetrahedral occlusion bodies and encodes two copies of he65. Viruses 11, 579. doi:10.3390/v11070579
Högfors-Rönnholm, E., Christel, S., Engblom, S., and Dopson, M. (2018). Indirect DNA extraction method suitable for acidic soil with high clay content. MethodsX 5, 136–140. doi:10.1016/j.mex.2018.02.005
Htwe, A. Z., Moh, S. M., Soe, K. M., Moe, K., and Yamakawa, T. (2019). Effects of biofertilizer produced from Bradyrhizobium and Streptomyces griseoflavus on plant growth, nodulation, nitrogen fixation, nutrient uptake, and seed yield of mung bean, cowpea, and soybean. Agronomy 9, 77. doi:10.3390/agronomy9020077
Hua, Z., Ouellette, M., Makkay, A. M., Papke, R. T., and Zhaxybayeva, O. (2021). Nutrient supplementation experiments with saltern microbial communities implicate utilization of DNA as a source of phosphorus. ISME J. 15, 2853–2864. doi:10.1038/s41396-021-00960-8
Huang, N., Wang, W., Yao, Y., Zhu, F., Wang, W., and Chang, X. (2017). The influence of different concentrations of bio-organic fertilizer on cucumber Fusarium wilt and soil microflora alterations. PLoS ONE 12, e0171490. doi:10.1371/journal.pone.0171490
Jansson, J. K., and Hofmockel, K. S. (2020). Soil microbiomes and climate change. Nat. Rev. Microbiol. 18, 35–46. doi:10.1038/s41579-019-0265-7
Ji, C., Liu, Z., Hao, L., Song, X., Wang, C., Liu, Y., et al. (2020). Effects of Enterobacter cloacae HG-1 on the nitrogen-fixing community structure of wheat rhizosphere soil and on salt tolerance. Front. Plant Sci. 11, 1094. doi:10.3389/fpls.2020.01094
Jiao, S., Chen, W., Wang, J., Du, N., Li, Q., and Wei, G. (2018). Soil microbiomes with distinct assemblies through vertical soil profiles drive the cycling of multiple nutrients in reforested ecosystems. Microbiome 6, 146. doi:10.1186/s40168-018-0526-0
Jiao, S., Chen, W., and Wei, G. (2019). Resilience and assemblage of soil microbiome in response to chemical contamination combined with plant growth. Appl. Environ. Microbiol. 85, 025233-18–e2618. doi:10.1128/AEM.02523-18
Kaschuk, G., Alberton, O., and Hungria, M. (2011). Quantifying effects of different agricultural land uses on soil microbial biomass and activity in Brazilian biomes: Inferences to improve soil quality. Plant Soil 338, 467–481. doi:10.1007/s11104-010-0559-z
Khalifa, A. Y. Z., Alsyeeh, A.-M., Almalki, M. A., and Saleh, F. A. (2016). Characterization of the plant growth promoting bacterium, Enterobacter cloacae MSR1, isolated from roots of non-nodulating Medicago sativa. Saudi J. Biol. Sci. 23, 79–86. doi:10.1016/j.sjbs.2015.06.008
Kim, N., Zabaloy, M. C., Guan, K., and Villamil, M. B. (2020). Do cover crops benefit soil microbiome? A meta-analysis of current research. Soil Biol. Biochem. 142, 107701. doi:10.1016/j.soilbio.2019.107701
Kome, G. K., Enang, R. K., Tabi, F. O., and Yerima, B. P. K. (2019). Influence of clay minerals on some soil fertility attributes: A review. OJSS 09, 155–188. doi:10.4236/ojss.2019.99010
Kroeger, M. E., Meredith, L. K., Meyer, K. M., Webster, K. D., de Camargo, P. B., de Souza, L. F., et al. (2021). Rainforest-to-pasture conversion stimulates soil methanogenesis across the Brazilian Amazon. ISME J. 15, 658–672. doi:10.1038/s41396-020-00804-x
Kuo, H.-C., Hui, S., Choi, J., Asiegbu, F. O., Valkonen, J. P. T., and Lee, Y.-H. (2015). Secret lifestyles of Neurospora crassa. Sci. Rep. 4, 5135. doi:10.1038/srep05135
Lacerda-Júnior, G. V., Noronha, M. F., Cabral, L., Delforno, T. P., de Sousa, S. T. P., Fernandes-Júnior, P. I., et al. (2019). Land use and seasonal effects on the soil microbiome of a Brazilian dry forest. Front. Microbiol. 10, 648. doi:10.3389/fmicb.2019.00648
Laurent, F., Poccard-Chapuis, R., Plassin, S., and Pimentel Martinez, G. (2017). Soil texture derived from topography in North-eastern Amazonia. J. Maps 13, 109–115. doi:10.1080/17445647.2016.1266524
Lebrigand, K., He, L. D., Thakur, N., Arguel, M.-J., Polanowska, J., Henrissat, B., et al. (2016). Comparative genomic analysis of Drechmeria coniospora reveals core and specific genetic requirements for fungal endoparasitism of nematodes. PLoS Genet. 12, e1006017. doi:10.1371/journal.pgen.1006017
Lehtovirta-Morley, L. E., Ross, J., Hink, L., Weber, E. B., Gubry-Rangin, C., Thion, C., et al. (2016). Isolation of ‘ Candidatus Nitrosocosmicus franklandus’, a novel ureolytic soil archaeal ammonia oxidiser with tolerance to high ammonia concentration. FEMS Microbiol. Ecol. 92, fiw057. doi:10.1093/femsec/fiw057
Leite, J., Passos, S. R., Simões-Araújo, J. L., Rumjanek, N. G., Xavier, G. R., and Zilli, J. É. (2018). Genomic identification and characterization of the elite strains Bradyrhizobium yuanmingense BR 3267 and Bradyrhizobium pachyrhizi BR 3262 recommended for cowpea inoculation in Brazil. Braz. J. Microbiol. 49, 703–713. doi:10.1016/j.bjm.2017.01.007
Leite-Filho, A. T., Soares-Filho, B. S., Davis, J. L., Abrahão, G. M., and Börner, J. (2021). Deforestation reduces rainfall and agricultural revenues in the Brazilian Amazon. Nat. Commun. 12, 2591. doi:10.1038/s41467-021-22840-7
Li, D., Liu, C.-M., Luo, R., Sadakane, K., and Lam, T.-W. (2015). Megahit: An ultra-fast single-node solution for large and complex metagenomics assembly via succinct de Bruijn graph. Bioinformatics 31, 1674–1676. doi:10.1093/bioinformatics/btv033
Li, H., Penttinen, P., Mikkonen, A., Stoddard, F. L., and Lindström, K. (2020). Response of soil bacterial community diversity and composition to time, fertilization, and plant species in a sub-boreal climate. Front. Microbiol. 11, 1780. doi:10.3389/fmicb.2020.01780
Li, J., Gan, G., Chen, X., and Zou, J. (2021). Effects of long-term straw management and potassium fertilization on crop yield, soil properties, and microbial community in a rice–oilseed rape rotation. Agriculture 11, 1233. doi:10.3390/agriculture11121233
Li, J., Wu, X., Gebremikael, M. T., Wu, H., Cai, D., Wang, B., et al. (2018). Response of soil organic carbon fractions, microbial community composition and carbon mineralization to high-input fertilizer practices under an intensive agricultural system. PLoS ONE 13, e0195144. doi:10.1371/journal.pone.0195144
Lima, M. J. A. de, Oliveira, E. C. de, Sampaio, L. S., Fraisse, C. W., and Souzadede, P. J. O. P. (2019). Agrometeorological analysis of the soybean potentiality in an Amazonian environment. Pesqui. Agropecu. Trop. 49, e54595. doi:10.1590/1983-40632019v4954595
Lin, Y.-T., Lin, Y.-F., Tsai, I. J., Chang, E.-H., Jien, S.-H., Lin, Y.-J., et al. (2019). Structure and diversity of soil bacterial communities in offshore islands. Sci. Rep. 9, 4689. doi:10.1038/s41598-019-41170-9
Liu, W.-Y., Wong, C.-F., Chung, K. M.-K., Jiang, J.-W., and Leung, F. C.-C. (2013). Comparative genome analysis of Enterobacter cloacae. PLoS ONE 8, e74487. doi:10.1371/journal.pone.0074487
Lladó, S., López-Mondéjar, R., and Baldrian, P. (2018). Drivers of microbial community structure in forest soils. Appl. Microbiol. Biotechnol. 102, 4331–4338. doi:10.1007/s00253-018-8950-4
Longley, R., Noel, Z. A., Benucci, G. M. N., Chilvers, M. I., Trail, F., and Bonito, G. (2020). Crop management impacts the soybean (Glycine max) microbiome. Front. Microbiol. 11, 1116. doi:10.3389/fmicb.2020.01116
Mahartha, K. A., and Suprapta, D. N. (2018). Efficacy of Enterobacter cloacae KtB3 to control damping-off disease on soybean caused by sclerotium rolfsii. Int. J. Agric. Biol. 20, 6.
Mandakovic, D., Rojas, C., Maldonado, J., Latorre, M., Travisany, D., Delage, E., et al. (2018). Structure and co-occurrence patterns in microbial communities under acute environmental stress reveal ecological factors fostering resilience. Sci. Rep. 8, 5875. doi:10.1038/s41598-018-23931-0
Maron, P.-A., Sarr, A., Kaisermann, A., Lévêque, J., Mathieu, O., Guigue, J., et al. (2018). High microbial diversity promotes soil ecosystem functioning. Appl. Environ. Microbiol. 84, 1–13. doi:10.1128/AEM.02738-17
Martins da Costa, E., Azarias Guimarães, A., Soares de Carvalho, T., Louzada Rodrigues, T., de Almeida Ribeiro, P. R., Lebbe, L., et al. (2018). Bradyrhizobium forestalis sp. nov., an efficient nitrogen-fixing bacterium isolated from nodules of forest legume species in the Amazon. Arch. Microbiol. 200, 743–752. doi:10.1007/s00203-018-1486-2
Martins, N. B., Ferreira, L. A. R., Queiroz, C. L., Buiatte, A. B. G., Correia Lima, A. M., De Souza, R. R., et al. (2021). Caseous stomatitis caused by Pseudomonas aeruginosa in Boa constrictor amarali. Acta Sci. Vet. 49. doi:10.22456/1679-9216.105257
Meyer, F., Paarmann, D., D’Souza, M., Olson, R., Glass, E., Kubal, M., et al. (2008). The metagenomics RAST server – A public resource for the automatic phylogenetic and functional analysis of metagenomes. BMC Bioinforma. 9, 386. doi:10.1186/1471-2105-9-386
Mikheenko, A., Saveliev, V., and Gurevich, A. (2016). MetaQUAST: Evaluation of metagenome assemblies. Bioinformatics 32, 1088–1090. doi:10.1093/bioinformatics/btv697
Moroenyane, I., Tremblay, J., and Yergeau, É. (2021). Soybean microbiome recovery after disruption is modulated by the seed and not the soil microbiome. Phytobiomes J. 5, 418–431. doi:10.1094/PBIOMES-01-21-0008-R
Naik, K., Mishra, S., Srichandan, H., Singh, P. K., and Choudhary, A. (2020). Microbial formulation and growth of cereals, pulses, oilseeds and vegetable crops. Sustain. Environ. Res. 30, 10. doi:10.1186/s42834-020-00051-x
Navarrete, A. A., Tsai, S. M., Mendes, L. W., Faust, K., de Hollander, M., Cassman, N. A., et al. (2015a). Soil microbiome responses to the short-term effects of Amazonian deforestation. Mol. Ecol. 24, 2433–2448. doi:10.1111/mec.13172
Navarrete, A. A., Venturini, A. M., Meyer, K. M., Klein, A. M., Tiedje, J. M., Bohannan, B. J. M., et al. (2015b). Differential response of Acidobacteria subgroups to forest-to-pasture conversion and their biogeographic patterns in the western Brazilian Amazon. Front. Microbiol. 6, 1443. doi:10.3389/fmicb.2015.01443
Nesme, J., Achouak, W., Agathos, S. N., Bailey, M., Baldrian, P., Brunel, D., et al. (2016). Back to the future of soil metagenomics. Front. Microbiol. 7, 73. doi:10.3389/fmicb.2016.00073
Neupane, A., Bulbul, I., Wang, Z., Lehman, R. M., Nafziger, E., and Marzano, S.-Y. L. (2021). Long term crop rotation effect on subsequent soybean yield explained by soil and root-associated microbiomes and soil health indicators. Sci. Rep. 11, 9200. doi:10.1038/s41598-021-88784-6
Nourrisson, C., Garcia-Hermoso, D., Morio, F., Kauffmann-Lacroix, C., Berrette, N., Bonhomme, J., et al. (2017). Thermothelomyces thermophila human infections. Clin. Microbiol. Infect. 23, 338–341. doi:10.1016/j.cmi.2016.10.025
O’Connell, R. J., Thon, M. R., Hacquard, S., Amyotte, S. G., Kleemann, J., Torres, M. F., et al. (2012). Lifestyle transitions in plant pathogenic Colletotrichum fungi deciphered by genome and transcriptome analyses. Nat. Genet. 44, 1060–1065. doi:10.1038/ng.2372
Panelli, S., Capelli, E., Comandatore, F., Landinez-Torres, A., Granata, M. U., Tosi, S., et al. (2017). A metagenomic-based, cross-seasonal picture of fungal consortia associated with Italian soils subjected to different agricultural managements. Fungal Ecol. 30, 1–9. doi:10.1016/j.funeco.2017.07.005
Pedrinho, A., Mendes, L. W., Merloti, L. F., da Fonseca, M. de C., Cannavan, F. de S., and Tsai, S. M. (2019). Forest-to-pasture conversion and recovery based on assessment of microbial communities in Eastern Amazon rainforest. FEMS Microbiol. Ecol. 95, fiy236. doi:10.1093/femsec/fiy236
Quesada, C. A., Paz, C., Oblitas Mendoza, E., Phillips, O. L., Saiz, G., and Lloyd, J. (2020). Variations in soil chemical and physical properties explain basin-wide Amazon forest soil carbon concentrations. SOIL 6, 53–88. doi:10.5194/soil-6-53-2020
Raij, B. V., Andrade, J. d., Cantarella, H., and Quaggio, A. J. (2001). Análise Química para Avaliação da Fertilidade de Solos Tropicais. Campinas: Instituto Agronômico.
Rajão, R., Soares-Filho, B., Nunes, F., Börner, J., Machado, L., Assis, D., et al. (2020). The rotten apples of Brazil’s agribusiness. Science 369, 246–248. doi:10.1126/science.aba6646
Ramesh, A., Sharma, S. K., Yadav, N., and Joshi, O. P. (2014). Phosphorus mobilization from native soil P-pool upon inoculation with phytate-mineralizing and phosphate-solubilizing Bacillus aryabhattai isolates for improved P-acquisition and growth of soybean and wheat crops in microcosm conditions. Agric. Res. 3, 118–127. doi:10.1007/s40003-014-0105-y
Rascovan, N., Carbonetto, B., Perrig, D., Díaz, M., Canciani, W., Abalo, M., et al. (2016). Integrated analysis of root microbiomes of soybean and wheat from agricultural fields. Sci. Rep. 6, 28084. doi:10.1038/srep28084
Rodrigues, T. E., Silvadas Chagas, R., da Silva Lima, J. M., GamaFerreira, J. R., ValenteAzevedo, M., et al. (2003). Carcterização e Calssificação dos solos do Município de Paragominas, Estado do Pará. 1st ed. Belem, PA: Embrapa Amazônia Oriental.
Salah, I., Parkin, I. P., and Allan, E. (2021). Copper as an antimicrobial agent: Recent advances. RSC Adv. 11, 18179–18186. doi:10.1039/D1RA02149D
Saleem, M., Hu, J., and Jousset, A. (2019). More than the sum of its parts: Microbiome biodiversity as a driver of plant growth and soil health. Annu. Rev. Ecol. Evol. Syst. 50, 145–168. doi:10.1146/annurev-ecolsys-110617-062605
Santos, D. G. dos (2019). De genomas a comunidades: Descrevendo alterações na comunidade bacteriana envolvida na oxidação do metano em solos da amazônia. PhD thesis. Piracicaba (SP): University of São Paulo. doi:10.11606/T.11.2019.tde-05082019-112352
Schulz, F., Alteio, L., Goudeau, D., Ryan, E. M., Yu, F. B., Malmstrom, R. R., et al. (2018). Hidden diversity of soil giant viruses. Nat. Commun. 9, 4881. doi:10.1038/s41467-018-07335-2
Soil Survey Staff (2014). Keys to soil taxonomy. 12th ed. Washington, DC: USDA-Natural Conservation Service.
Souza, R. C., Mendes, I. C., Reis-Junior, F. B., Carvalho, F. M., Nogueira, M. A., Vasconcelos, A. T. R., et al. (2016). Shifts in taxonomic and functional microbial diversity with agriculture: How fragile is the Brazilian Cerrado? BMC Microbiol. 16, 42. doi:10.1186/s12866-016-0657-z
Sugiyama, A., Ueda, Y., Zushi, T., Takase, H., and Yazaki, K. (2014). Changes in the bacterial community of soybean rhizospheres during growth in the field. PLoS ONE 9, e100709. doi:10.1371/journal.pone.0100709
Sun, S., and Badgley, B. D. (2019). Changes in microbial functional genes within the soil metagenome during forest ecosystem restoration. Soil Biol. Biochem. 135, 163–172. doi:10.1016/j.soilbio.2019.05.004
Tahat, M. M., Alananbeh, K. M., Othman, Y. A., and Leskovar, D. I. (2020). Soil health and sustainable agriculture. Sustainability 12, 4859. doi:10.3390/su12124859
Tokarz-DeptułaBeata, B., Niedźwiedzka-Rystwej1, P., Czupryńska, P., and Deptuła, W. (2019). Protozoal giant viruses: Agents potentially infectious to humans and animals. Virus Genes 55, 574–591. doi:10.1007/s11262-019-01684-w
Torregrosa-Crespo, J., Bergaust, L., Pire, C., and Martínez-Espinosa, R. M. (2018). Denitrifying haloarchaea: Sources and sinks of nitrogenous gases. FEMS Microbiol. Lett. 365. doi:10.1093/femsle/fnx270
Totsche, K. U., Amelung, W., Gerzabek, M. H., Guggenberger, G., Klumpp, E., Knief, C., et al. (2018). Microaggregates in soils. J. Plant Nutr. Soil Sci. 181, 104–136. doi:10.1002/jpln.201600451
van Bruggen, A. H. C., Goss, E. M., Havelaar, A., van Diepeningen, A. D., Finckh, M. R., and Morris, J. G. (2019). One Health - cycling of diverse microbial communities as a connecting force for soil, plant, animal, human and ecosystem health. Sci. Total Environ. 664, 927–937. doi:10.1016/j.scitotenv.2019.02.091
VanInsberghe, D., Maas, K. R., Cardenas, E., Strachan, C. R., Hallam, S. J., and Mohn, W. W. (2015). Non-symbiotic Bradyrhizobium ecotypes dominate North American forest soils. ISME J. 9, 2435–2441. doi:10.1038/ismej.2015.54
Velasco, J., Oliva, B., Gonçalves, A. L., Lima, A. S., Ferreira, G., França, B. A., et al. (2020). Functional characterization of a novel thermophilic exo-arabinanase from Thermothielavioides terrestris. Appl. Microbiol. Biotechnol. 104, 8309–8326. doi:10.1007/s00253-020-10806-6
Wang, Z., Liu, Y., Zhao, L., Zhang, W., and Liu, L. (2019). Change of soil microbial community under long-term fertilization in a reclaimed sandy agricultural ecosystem. PeerJ 7, e6497. doi:10.7717/peerj.6497
Wipf, H. M.-L., Xu, L., Gao, C., Spinner, H. B., Taylor, J., Lemaux, P., et al. (2021). Agricultural soil management practices differentially shape the bacterial and fungal microbiomes of Sorghum bicolor. Appl. Environ. Microbiol. 87, AEM.02345-20–20. doi:10.1128/AEM.02345-20
Wood, D. E., Lu, J., and Langmead, B. (2019). Improved metagenomic analysis with Kraken 2. Genome Biol. 20, 257. doi:10.1186/s13059-019-1891-0
Yan, Y., Yuan, Q., Tang, J., Huang, J., Hsiang, T., Wei, Y., et al. (2018). Colletotrichum higginsianum as a model for understanding host–pathogen interactions: A review. Int. J. Mol. Sci. 19, 2142. doi:10.3390/ijms19072142
Zeng, Y., Abdo, Z., Charkowski, A., Stewart, J. E., and Frost, K. (2019). Responses of bacterial and fungal community structure to different rates of 1, 3-dichloropropene fumigation. Phytobiomes J. 3, 212–223. doi:10.1094/PBIOMES-11-18-0055-R
Zhalnina, K., de Quadros, P. D., Gano, K. A., Davis-Richardson, A., Fagen, J. R., Brown, C. T., et al. (2013). Ca. Nitrososphaera and Bradyrhizobium are inversely correlated and related to agricultural practices in long-term field experiments. Front. Microbiol. 4, 104. doi:10.3389/fmicb.2013.00104
Zhao, L., Xu, Y., and Lai, X. (2018). Antagonistic endophytic bacteria associated with nodules of soybean ( Glycine max L.) and plant growth-promoting properties. Braz. J. Microbiol. 49, 269–278. doi:10.1016/j.bjm.2017.06.007
Keywords: amazon soil, clayey soils, microbiological diversity, metagenome, chemical characteristic
Citation: Cardenas-Alegria OV, Dantas CWD, Silva Guedes Lobato EM, Da Silva SP, Moraes CdS, Cruz ACR, Ramos RTJ and Carneiro AR (2022) Influence of soil management for soybean production under microbial diversity in amazon soils. Front. Environ. Sci. 10:1024610. doi: 10.3389/fenvs.2022.1024610
Received: 22 August 2022; Accepted: 31 October 2022;
Published: 18 November 2022.
Edited by:
Jehangir Bhadha, University of Florida—Everglades Research & Education Center, United StatesReviewed by:
Ademir Araujo, Federal University of Piauí, BrazilNan Xu, University of Florida, United States
Copyright © 2022 Cardenas-Alegria, Dantas, Silva Guedes Lobato, Da Silva, Moraes, Cruz, Ramos and Carneiro. This is an open-access article distributed under the terms of the Creative Commons Attribution License (CC BY). The use, distribution or reproduction in other forums is permitted, provided the original author(s) and the copyright owner(s) are credited and that the original publication in this journal is cited, in accordance with accepted academic practice. No use, distribution or reproduction is permitted which does not comply with these terms.
*Correspondence: Oscar Victor Cardenas-Alegria, b3NjYXIuYWxlZ3JpYUBpY2IudWZwYS5icg==