- 1College of Ecology and Environment, Hainan University, Haikou, China
- 2School of Civil Engineering, Sun Yat-sen University, Guangzhou, China
- 3School of Tourism, Hainan University, Haikou, China
Different regions exhibit different response patterns of hydrological process changes under changing environments. The hydrological response mechanism of underlying surface changes in tropical rainforest regions remains uncertain, so there is an urgent need to study the causes of hydrological changes in typical tropical watersheds. The sequential clustering analysis and Pettitt-Mann-Whitney test were employed to detect abrupt change points of runoff series for the Wanquan River Basin in the tropics, and the series was then divided into pre-impact period (1967–1990) and post-impact period (1991–2014). And the Soil and Water Assessment Tool (SWAT) model was used to simulate the runoff to quantify the impact of climate change, land use change and other human activities on runoff, which the latter two collectively referred to as “human activities” in this study. The findings reveal: 1) runoff series showed an abrupt change and a downward trend around the year 1990; the SWAT model has a good simulation in the Wanquan River Basin during the pre-impact period; the coefficients of determination (R2) for the calibration and validation periods are both 0.91, and the Nash-Sutcliffe model efficiency coefficients (NSE) for the calibration and validation periods are 0.89 and 0.86, respectively. 2) There was a considerable change in land use between 1967 and 2014, as evidenced by a decrease of 29.54% in natural forest and an increase of 54.90% in rubber. These land use changes were mostly caused by the transformation of tropical rainforests into rubber forests and orchards. 3) Runoff declined in the post-impact period from the pre-impact period, with climate change, land use change, and other human activities contributing 44.05%, 10.83%, and 45.12% to the runoff change, respectively. The conversion of tropical rain forests to rubber forests and orchards has indirectly led to a reduction in runoff. These results can provide a reference for understanding the evolution of water cycle for other tropical rivers.
1 Introduction
Exploring local and global environmental changes and their impacts on the ecological environment has always received extensive attention (Jain et al., 2010; Chang et al., 2015). In a changing environment, climate change and human activities are often the two main drivers of changes in water resources. From a long-term scale, due to changes in temperature and rainfall, the effects of climate change on water resources are more noticeable, including changes in the spatial and temporal distribution of atmospheric precipitation and surface evapotranspiration (Zhou et al., 2018). On a short-term scale, human activities, including land use and large water conservancy projects, produce a more prominent impact on water resources (Vorosmarty et al., 2000). One of those impacts is land use change, which significantly influences water supplies due to human activities. Changes in land use affect the hydrological cycle of basins by changing their underlying surface covering, which in turn influences canopy interception, surface infiltration, evapotranspiration, and surface runoff (Morán-Tejeda et al., 2010). Climate change and human activities, however, have combined consequences. As a result, it is crucial to precisely quantify the impact of human activities and climate change on runoff and attribute it to various driving factors.
So far, statistical and simulated methods have been the major tools used for quantitative research into the effects of human activities and climate change on hydrological processes (DeFries and Eshleman, 2004). The regression approach is typically used in statistical methods to evaluate the impacts on runoff based on recorded meteorological and hydrological data, and subsequently to determine the regression relationship between runoff and one or more meteorological factors (Kong et al., 2016). The slope change ratio of accumulative quantity (SCRAQ) method is used to plot the multi-year cumulative runoff, precipitation, and evaporation curves, then the slope of the fitted straight lines before or after the abrupt change is determined, and finally the contributions of different factors to the runoff change is calculated (Wu et al., 2017). Although statistical methods are generally simple to apply, they require long sequences of data, and the use of multiple driving factors and uncertain data can undoubtedly affect the assessment results. In addition, statistical methods are unable to account for the effects of spatial heterogeneity, land use change, and climate change on hydrological processes (Abbaspour et al., 2007). In a nutshell, the statistical methods cannot adequately explain how and to what extent human activities affect runoff, which greatly reduces the validity of analysis results (Zhang et al., 2017).
The hydrological model has evolved over time thanks to a progressive understanding of water cycle, transitioning from a lumped to a distributed hydrological model. Lumped hydrological models commonly treats the watershed as a whole and ignore geographic variability, and many of their equations also lack obvious physical definitions (Zeng et al., 2013; Zuo et al., 2014). The benefit of distributed hydrological models is that, in addition to considering geographic heterogeneity, they can also describe the mechanisms of hydrological processes (Lin et al., 2020). On top of that, the simulation scenario may be set up flexibly. In order to assess how climate change, land use change, and other human activities affect runoff, distributed hydrological models based on physical processes have become more prevalent in recent years (Chang et al., 2015; Wang et al., 2017). Practical problems are frequently addressed with hydrological models such as TOPMODEL (Lin et al., 2010), Variable Infiltration Capacity (VIC) (Bao et al., 2012; Chang et al., 2015), and SWAT (Fukunaga et al., 2015; Mekonnen et al., 2018). Among them, the SWAT model is one of the most popular distributed hydrological models, and several studies have shown that it is well suited for application in a variety of geographical and climatic locations. It can be applied to different land management and climate change models (Lin et al., 2015; Wang et al., 2017). The SWAT model is widely applied in most rivers in China, from arid to semi-arid to wet zones. For instance, Dong et al. (2014) used the SWAT model to examine how human activities and climate change affected an arid inland watershed in northwest China. The results revealed that human activities were the primary cause of changes in runoff in the watershed, accounting for 85.7% of the runoff reduction and only 14.3% of the runoff reduction resulting from climate change. Sun et al. (2019) used the SWAT model to jointly anticipate future hydrological and soil droughts in the Yangtze River watershed. Based on SWAT model, Wu et al. (2019) examined the effect of reservoir operation on hydrological drought in the Dongjiang basin. Besides, the SWAT model also showed good applicability in tropical areas. For example, Marhaento et al. (2017) adopted SWAT to examine the impact of land use change on water balance in the tropics. SWAT models were developed by Fukunaga et al. (2015) for poor availability of data in the tropics, and produced satisfactory results.
Due to the combined effects of human activities and climate change, hydrological processes have been altered to varied degrees as economy develops. Many studies have explored the hydrological consequences of individual environmental changes, such as land use change or climate change (Pan et al., 2017; Bajracharya et al., 2018), as well as the hydrological effects of combining these changes with human activities (Nie et al., 2011; Lin et al., 2012; Sood et al., 2013). And numerous researchers have conducted in-depth research on the Pearl River Basin (Liu et al., 2010; Zhou et al., 2018), the Yellow River Basin (Zhan et al., 2014; Zhao et al., 2014; Chang et al., 2015), the Yangtze River Basin (Zhao et al., 2015; Zhang et al., 2017), arid and semi-arid regions in China, and several typical watersheds in other countries (Legesse et al., 2003; Heo et al., 2015). However, there are few studies on island rivers in the tropics, which make them particularly regional and ecologically fragile because of the abundance of tropical natural forest and artificial rubber trees in the basin (Marhaento et al., 2017). Tropical forests are vital to maintaining the health of ecosystems (Chen et al., 2016). Marhaento et al. (2017) believe that the water balance change in the Samin basin in Indonesia can be attributed to a change in land use, mostly owing to the expansion of residential area and the contraction of forest area. Op de Hipt et al. (2019) used the SHETRAN model to examine how land use and climate change affected water resources in tropical West Africa. The findings indicate that both climate change and land use change individually increase runoff as well as the combined effects. Many studies have found that different regions exhibit different response patterns of hydrological processes changes under changing environments. It is essential to undertake numerous case studies of typical places and keep exploring theoretic concepts. Nonetheless, the hydrological response mechanism of underlying surface changes in tropical rainforest regions is still uncertain. There is an urgent need to study the causes of hydrological changes in typical tropical watersheds. But there are few studies on how runoff reacts to vegetation changes in watersheds across various tropical climate zones (Zhang, 2020; Tan et al., 2021).
The Wanquan River is the third-largest river in South China’s Hainan province, and its basin is abundant with rubber plantations and tropical rainforests, giving it special geographical and ecological vulnerability features (Zhao et al., 2018). Influenced by intensive human activities, the vegetation cover in the watershed has altered drastically in recent decades. The community has been deeply concerned about an array of issues, including how the fast development in the rubber forest area would affect the hydrology process of the basin following the destruction of tropical rainforests. The analysis above suggests that one advantage of the SWAT model is an effective physical mechanism with spatially distributed parameters, so it is able to assess the impact of climate change, land use change and other human activities on runoff in the Wanquan River basin. The main uncertainty of SWAT model is related to large amounts of input data (spatial data and meteorological data), which causes unavoidable deviations from model simulation. Recognizing the above limitations, nevertheless, this study collected and analyze sufficient data to quantify the impact of climate change, land use change and other human activities on regional hydrological processes in the Wanquan river basin, which has important implications for sustainable water resources management in tropical regions. Therefore, the Wanquan River Basin is chosen as the study area in this study.
The goals of this study are: 1) to build a distributed hydrological model for the Wanquan River basin, and quantify the contributions of climate change, land use change and other human activities based on SWAT model; 2) to shed light on the mechanisms behind changes in hydrological processes as a result of tropical rainforests turning into rubber forests and other economic forests. The study can provide a basis for exploring changes in the water cycle of other tropical rivers.
2 Study area and data
The Wanquan River Basin (109°37′-110°38′E, 18°46′-19°31′N) is situated in the east-central part of Hainan Island, with a total length of 163 km. It originates from Fengmenling in the Wuzhi Mountain, passes through the cities of Qiongzhong, Wanning, and Qionghai, and flows into the South China Sea in Boao Port, and its long-term average runoff is 163.9 m3/s (Zhao et al., 2018). In order to statistically assess the effects of climate change and human activities on runoff, the upper reach of the Wanquan River Basin was chosen as a representative basin in the research area (Figure 1). The upper reach of the Wanquan River is in the middle and low mountainous areas and cover an area of 1,149 km2, where the river is meandering and fast flowing, eroding to form a gully basin. The main soil types are red loam and its derivatives. The area has a tropical monsoon and marine climate with an average annual temperature of 24°C and a precipitation of 2,300 mm. The watershed runoff is mostly replenished by precipitation and is susceptible to climate change as well as human activities (such as farmland irrigation, land use change, etc.). The Water Affairs Office of the Hainan Province provided daily runoff and precipitation data from 1967 to 2014, and the monthly observed hydrological data were measured at the Jiabao station, while daily data on solar radiation, relative humidity, wind speed, and highest and lowest temperatures for the same period were available from the National Meteorological Information Center of China (http://data.cma.cn). Meanwhile, Landsat-8 TM remote sensing images with a resolution of 30 m were downloaded from the Consultative Group on International Agricultural Research (CGIAR) Consortium for Spatial (CGIAR-CSI) (http://srtm.csi.cgiar.org), and interpreted into land use map data with a resolution of 30 m through supervised classification combined with manual visualization. The land use types were classified as rubber land, residential area, orchard, water, agricultural land and natural forest in line with the geographical location and characteristics of the study area. To be specific, rubber data were collected from the Rubber Research Institute of the Chinese Academy of Tropical Agricultural Sciences (Chen et al., 2016) for further amendment. The land use map for 2014 were validated using the 163 sample plots from the survey, and the results are shown in Table 1. Taking the land use map of 2014 as an example, the accuracy of remote sensing image interpretation in 2014 was 84.05%, and the kappa coefficient was 0.80. Finally, the land use map that meets the needs of this study was obtained, and the two land use maps for 1988 and 2014 can be observed in Figure 2. Data on soil types were available from the Data Center for Resources and Environmental Sciences, Chinese Academy of Sciences (http://www.resdc.cn). Besides, the Digital Elevation Model (DEM) with a spatial resolution of 30 m × 30 m was also downloaded from the Consultative Group on International Agricultural Research (CGIAR) Consortium for Spatial (CGIAR-CSI) (http://srtm.csi.cgiar.org).
3 Methodology
3.1 Change-point test
Sequential clustering analysis is one of the effective methods to identify the abrupt change points in hydrological series. Its crux is to deduce the optimal breakpoint, so that the sum of the squares of the deviation between the classes is minimized. And the sums of the squares of the deviation before and after the breakpoint are calculated. Details of the sequential clustering analysis method can be found in Dong et al. (2014).
Pettitt-Mann-Whitney method, one of the non-parametric test methods, has a clear physical meaning. It can identify the occurrence of a change point, and it is widely used to detect hydrological and climatic abrupt change points (Zuo et al., 2014; Bisht et al., 2017).
3.2 SWAT model
The SWAT model is a distributed hydrological model developed by the United States Department of Agriculture—Agricultural Research Service (USDA-ARS), which can simulate the basin hydrologic process as per the hydrologic cycle and water balance principles (Zhang et al., 2010). This model has the following characteristics: 1) it has an effective physical mechanism and its parameters are spatially distributed; 2) it can evaluate and predict the impact of land use changes on hydrological processes; 3) it can predict hydrological conditions without observed hydrometeorological data (Lai et al., 2006; Zhang et al., 2012). Therefore, the SWAT model is suitable for simulating surface runoff, groundwater discharge, as well as hydrological processes influenced by climate change and human activities. A detailed description of the SWAT model structure and settings can be found in the literature (Zhang et al., 2012).
SWAT-CUP (Calibration and Uncertainty Programs) was selected to calibrate and validate the SWAT model of the Wanquan River Basin. The SWAT model employs the coefficient of determination (R2) and the Nash Sutcliffe model efficiency coefficient (NSE) to evaluate its performance during calibration and validation period. R2 is obtained by linear regression method, and NSE is used to assess the fitness between the measured values and the simulated values (Lai et al., 2006).
The coefficient of determination (R2) can be defined as:
In the equation,
While the Nash Sutcliffe model efficiency coefficient (NSE) can be defined as:
In the equation,
3.3 Quantifying method
Following the identification of abrupt change point and the establishment of SWAT model, the runoff changes in the Wanquan River Basin can be attributed to climate change, land use change and other human activities. The calculation steps are as follows (Lin et al., 2012):
Step 1. Based on the abrupt change point, the runoff series can be divided into pre-impact period (natural period) and post-impact period (period with environmental changes).
Step 2. Calculate the total amount of runoff change:
where △RT is the total amount of runoff change, RHR is the observed runoff in post-impact period, and RN is the observed runoff in pre-impact period.
Step 3. Calculate the impact of climate change on runoff:
where △RC is the runoff change caused by climate change, RHN is the natural runoff during post-impact period, and RN is the observed runoff in pre-impact period.
Step 4. Calculate the impact of land use change on runoff:
Where △RL is the runoff change caused by land use change, RH2 and RH1 are simulated runoff II (corresponding to land use scenario in 2014) and simulated runoff I (corresponding to land use scenario in 1988) for post-impact period, respectively.
Step 5. Calculate the impact of other human activities on runoff △R0.
Step 6. Calculate the sum of the absolute values of runoff changes caused by climate change, land use change and other human activities △.
Step 7. Calculate the percentage of impact of land use change on runoff μl, impact of climate change on runoff μC, and impact of other human activities on runoff μR0.
4 Result
4.1 Temporal variations of runoff
The analysis of interannual and intra-annual variation of runoff from the Jiabao hydrological station in the upper reach of the Wanquan River basin was investigated over the observation period. Figure 3A illustrates the trends analysis based on linear regressions for runoff at the Jiabao station from 1967 to 2014, the results suggest that there was no significant decreasing trend in the runoff time series. The mean annual runoff from 1967 to 2014 varied from a low of 4.55 × 108 m3 in 1969 to a high of 56.78 × 108 m3, with a mean of 14.01 × 108 m3. Figure 3B shows that the intra-annual distribution of runoff varies in a unimodal distribution, peaking in October with 6.88 × 108 m3 of runoff, which accounts for 25% of total annual runoff. The typhoon season generally occurred from July to October, accounting for 67.94% of total annual runoff during the study period. A low precipitation period lasted from 1 to 3 months in the basin and accounted for less than 1% of total annual runoff during the study period.
4.2 Abrupt change point on runoff
Two statistical techniques were chosen in this study to identify the abrupt change point in order to ensure the reliability of the results. The results of the Pettitt-Mann-Whitney test are displayed in Figure 4A. The statistics U reach their highest value in 1990, indicating that this year may be the most likely change point for the runoff series between 1967 and 2014. Additionally, the sum of square of the deviation for the same series is calculated using the sequential clustering method. As seen in Figure 4B, the sum of square of the deviation is the minimum in the year 1990, further validating that year as the abrupt change point. Accordingly, the runoff series can be quantified into pre-impact period (1967–1990) and post-impact period based on the abrupt change point (1991–2014).
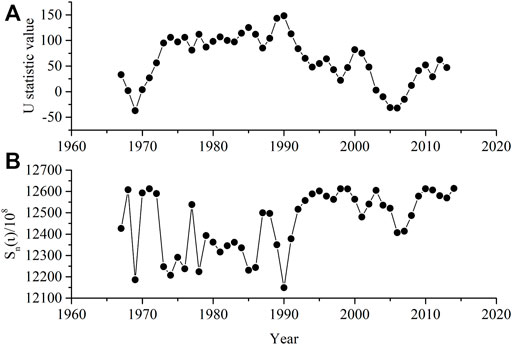
FIGURE 4. Abrupt change point detected by the sequential clustering analysis (U statistic value) and Pettitt-Mann-Whitney test (Sn(ι)).
4.3 Model calibration and validation
The SWAT model is based on the data of pre-impact period which was then divided into calibration period (1967–1978) and validation period (1979–1990). Both calibration and validation periods span 12 years, so that the results of these two periods can be compared easily. According to the parameter sensitivity analysis, seven highly sensitive parameters are used to calibrate, and the results are listed in Table 2. The most sensitive of the seven parameters is CN2, and ALPHA_BF, GW_DELAY, and GWQMN, followed by CN2, demonstrating that the runoff in the Wanquan River Basin is sensitive to surface water, groundwater exchange and surface cover. The evaluation results of calibration and validation are summarized in Table 3, where R2 and NSE are 0.91 and 0.89 in the calibration period, respectively whereas R2 and NSE are 0.91 and 0.86 in the validation period, respectively. Figure 5 presents the comparison of simulated and observed monthly runoffs for the calibration and validation periods. Both results are shown in Table 3 and Figure 5 give consistent results, which indicate that the SWAT model has achieved a good simulation. Accordingly, the SWAT model can be applied to simulate the runoff of the Wanquan River Basin.
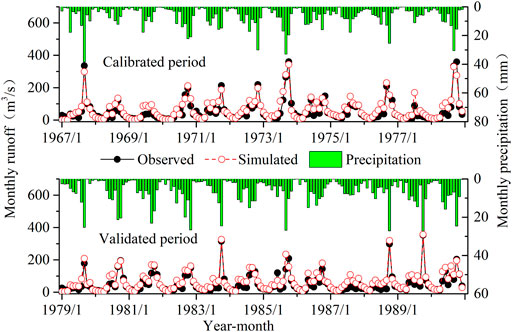
FIGURE 5. Monthly precipitation, observed runoff and simulated runoff for the calibration and validation period at the Jiabao station.
4.4 Impact of climate change and human activities on runoff change
4.4.1 Impact of land use change on runoff
The Wanquan River Basin was taken as the research object to quantitatively analyze the impact of climate change, human activities and especially land use change on runoff. In the Wanquan River Basin, the primary land use types are natural forest land, orchard and rubber, which together account for 89% of the whole basin. The conversion of land use types in the basin is the most obvious between natural forests, orchards, and rubber forests, as shown in the Sankey diagram of land use transfer matrix (see Figure 6). In addition, as shown in Table 4 and Figure 2, from 1988 to 2014, the area of rubber increased continuously from 221.58 km2 to 343.23 km2, an increase of 54.90%; the water area and the urban area continued to increase as well; the area of agriculture land decreased slightly, and the natural forest area decreased significantly, from 293.73 km2 to 206.96 km2, a decrease of 29.54%. Land use changes in the Wanquan River Basin would contribute to the change of hydrological process, including the process from runoff generation to concentration. In order to analyze the impact of land use change on runoff, the land use map of 1988 and the meteorological data from 1991 to 2014 are fed into the SWAT model, to simulate the runoff process in a natural state during the post-impact period, thus generating the results of monthly runoff RH1. In the meantime, the results of monthly runoff RH2 are produced by using the land use map of 2014 and meteorological data from 1991 to 2014. The difference in monthly runoff between varying land uses is equal to the runoff arising from land use change which decreased runoff by 0.15 × 108 m3 (see Table 5). As shown in Figure 7, the monthly runoff process attributable to land use change from 1991 to 2014 was simulated by the SWAT model. Meanwhile, it can also be noted that the greater the monthly precipitation, the smaller the impact of land use on runoff.
4.4.2 Impact of climate change on runoff
Climate change is one of the critical contributors to runoff changes. Figure 8 illustrates that the annual precipitation showed a slight upward trend in the Wanquan River basin between 1967 and 2014. The maximum rainfall occurred in 1990, greater than the average of 1,028.08 mm, while the minimum rainfall in 1977, less than the average of 1,131.37 mm. Figure 9 depicts the interannual variations of maximum and minimum temperatures in the Wanquan River Basin from 1967 to 2014. Both maximum and minimum temperatures are on the rise, and temperature variations are almost always within a small range. Apart from that, Figure 10, which demonstrates the interannual variation curve of annual evaporation for the Wanquan River Basin from 1967 to 2014, reveals a growing trend in annual evaporation throughout that period. The Wanquan River Basin’s annual evaporation increased from the lowest level of 902.09 mm in 1978 to the highest level of 1,233.6 mm in 2005. To examine the impact of climate change on runoff, the monthly runoff of RHN was simulated by the SWAT model using the 2014 land use map and the meteorological data from 1991 to 2014. The difference of the monthly runoff under different climatic conditions is equal to the runoff induced by climate change, and the runoff caused by climate change grew by 0.48 × 108 m3.
4.4.3 Quantify the impact of climate change and human activities on runoff
The calculation steps are based on Eqs. 3–10, and the change in runoff may be attributed to climate change, land use change, and other human activities. Table 5 presents the quantitative results of the impacts of climate change and human activities on runoff. The runoff falls by 0.16 × 108 m3 in the post-impact period. Climate change drives up runoff, contributing to 44.05% of the runoff change. By contrast, land use change drives down runoff, accounting for 10.83% of the runoff change. Further, other human activities also decrease runoff, representing 45.12% of the runoff change, which is extremely high. In general, human activities (including land use change and other human activities) have a slightly greater impact on runoff than climate change. Land use change on runoff variance does have an impact on runoff change depending on the degree of land use change, albeit less dramatic than other human activities and climate change. It’s also noteworthy that both climate change and human activities heavily influences runoff, of which human activities is the primary driver for runoff reduction in the Wanquan River Basin.
5 Discussion
5.1 Impacts of climate change and human activities on runoff
The runoff reduction in the Wanquan River Basin has captured much attention, and the influence of the dominant factors on runoff changes has been investigated from qualitative and quantitative perspectives (Zhao et al., 2018). Since human activities is a major contributor to runoff reduction in the Wanquan River Basin, more serious consideration should be given to both direct human impacts (such as domestic water demand, industrial and agricultural water use, and water diversion) and indirect human impacts (such as runoff change originating from the change of underlying surface characteristics) (Li et al., 2016; Wu et al., 2020).
With regard to indirect human impacts, the Wanquan River Basin is one of the major agricultural areas in Hainan province. As the local government encourage residents to plant rubber and the benefits from doing so increased, the rubber planting area rose rapidly, and other land use types including large natural forests were transformed into rubber forests. By comparing the two-stage land use map of the Wanquan River Basin, it is found that the area of rubber forests soared by 54.90% and the residential area and water area also surged, whereas the area of natural forests dropped by 29.54%, and the agriculture area and orchard reduced slightly. However, tropical fruit and tropical economic crops were still the main economic income of the Wanquan River Basin. The change in land use reveals that the natural forest had been seriously damaged, because natural forests were cut down while economic forests such as rubber, areca and fruit trees were planted in the basin. The data of the Qionghai Statistical Yearbook suggest that the trajectory of population change and land use change were preliminarily studied. Using the city of Qionghai as an illustration, the population of Hainan grew steadily since the founding of New China, particularly after the Reform and Opening-up when a large number of people moved there, leading the population of the basin to rise suddenly (see Figure 11). By comparison, the area of agricultural land continued to fall after 1980, whereas that of orchard expanded steadily until they finally levelled off. A few years later, when the vegetation cover is adequate, the canopy can intercept a considerable proportion of rain and evaporate it into the atmosphere (Chang et al., 2015). It can be seen from Figure 10 that the annual evaporation witnesses an upward trend, and that the average evaporation in the post-impact period is 66.6 mm higher than that in the pre-impact period. In general, forest can produce less runoff than other land use/cover types but more evaporation (Li et al., 2009), because the biomass per unit area of forest is much lower than that of cropland (such as orchard) (Li et al., 2020; Tan et al., 2022). Furthermore, some studies illustrate that more water is evapotranspirated by rubber plantation than by rain forest, and that rubber plantations in Xishuangbanna are called “water pumps” (Tan et al., 2011; Giambelluca et al., 2016). As a consequence, change of land use, especially the surge in the area of rubber forests, will lead to high evapotranspiration in the basin. Meanwhile, it also can change local micro-topography, improve the permeability of water in the soil, and slow down or contain runoff, thereby reducing runoff (Chang et al., 2015). Undoubtedly, the impact of land use change on water resources is a complex process (Li et al., 2016; Wu et al., 2020).
As regards direct human impacts, some reports reveal that agricultural irrigation activities would reduce the runoff in the basin. On top of that, other human activities also significantly contribute runoff reduction. For one thing, the urbanization of the Wanquan River Basin and the rapid development of agriculture have pushed up residents’ demands for more water. For another, agricultural activities directly draw water from the river channel for irrigation, especially tropical fruits and tropical economic crops that need substantial water for irrigation. The combined agricultural area and orchard area in the basin are 435.91 km2, accounting for 42.79%. The completion of the hydropower project at Hongling Reservoir, with a catchment area of 754 km2 and a total storage capacity of 704 million m3 has addressed an array of problems, such as urban and rural water supply, agricultural irrigation, power generation, and flood discharge, but also inevitably altered the hydrological process, hence reducing the runoff. More seriously, the expansion of water area will also give rise to an increase in evaporation.
In a nutshell, the runoff reduction in the Wanquan River Basin results from a combination of multiple factors, and the contribution of each factor to the runoff varies greatly. Specifically, the analysis of temperature, evaporation and precipitation demonstrates that precipitation is the most influential climate change factor on runoff. Generally, climate change and human activities are the dominant factors influencing runoff change, with the latter having a slightly greater impact than the former. Land use change is also one of the critical factors leading to the reduction of runoff. For these reasons, the respective contributions of climate change and human activities should be given considerable attention for the protection, planning, and management of water resources.
5.2 Uncertainties and limitations
Comparing the results of this study with previous studies listed in Table 6, both climate change and human activities have an impact on the reduction of runoff (Zhang et al., 2012; Tan et al., 2022). Since the impacts of human activities on runoff were not uniformly defined in previous studies, land use change and other human activities are collectively referred to as human activities. The results of this study are consistent with Yuan et al. (2015) and Zhao et al. (2018), that climate change increases runoff, while land use decreases runoff, and land use change also amplifies the effect of climate change strongly (Op de Hipt et al., 2019). In addition, human activities have gradually become the domain factors affecting runoff change, which is consistent with the results of studies in China (Zheng et al., 2009; Guo et al., 2014; Li et al., 2020; Wu et al., 2020) and other tropical regions (Marhaento et al., 2016; Sharifi et al., 2021).
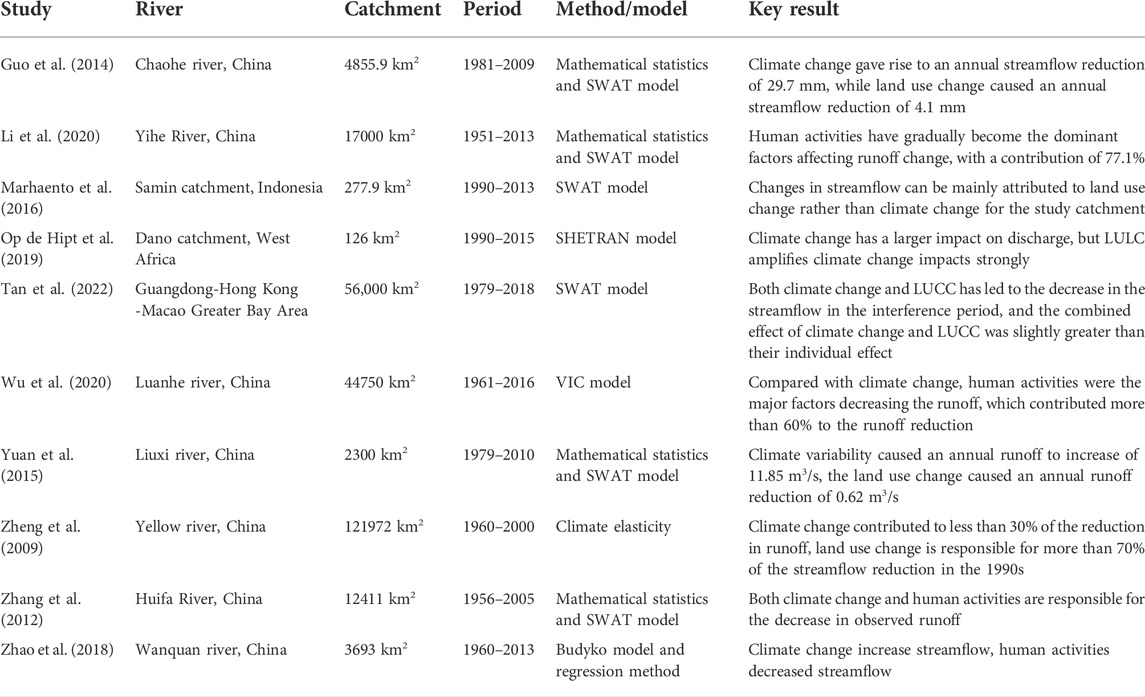
TABLE 6. Summary of relevant literature on runoff change of climate and LUCC in relation to the objectives of this study.
The major limitations in this study are mainly attributable to the SWAT model and datasets. First, the large amount of input data includes both spatial data (DEM and soil and land cover maps) and meteorological data (precipitation, temperature, wind and relative humidity). Second, the SWAT model employs numerous generalized and empirical equations to characterize complex hydrological processes, which complicates the SWAT model with so many parameters involved, therefore causing unavoidable deviations to model simulation. Finally, this study fails to individually study the impact of other human activities on runoff. Besides, the contributions of the influence factors, i.e., climate change, land use change and other human activities, are separately examined, so their interplay is ignored and should be investigated further in future studies.
6 Conclusion
The hydrological processes in the Wanquan River Basin have changed drastically due to climate change, land use change and other human activities, with the latter two collectively knowns as human activities. A quantitative analysis was leveraged to determine how these three factors influence runoff. The following are the primary conclusions: 1) The abrupt change point of runoff series from 1967 to 2014 for the Wanquan River Basin was detected, with an abrupt change taking place in 1990, and the hydrological processes in the pre- and post-impact periods differ considerably from one another. 2) The SWAT model can satisfy certain accuracy criteria and has strong applicability when used to simulate the runoff process of the Wanquan River Basin. 3) Runoff is directly caused by precipitation which is likely to may improve runoff. Human activities influence runoff changes in a significant way, and the conversion of tropical rain forests to rubber forests and orchards has indirectly led to runoff reduction. The contributions of climate change, land use change and other human activities to runoff changes were 44.05%, 10.83%, and 45.12%, respectively.
Data availability statement
The original contributions presented in the study are included in the article, further inquiries can be directed to the corresponding authors.
Author contributions
DL: Methodology, Software, Validation, Investigation, Visualization, Writing—original draft. LZ: Conceptualization, Resources, Data Curation, Methodology, Writing—review and editing. WX: Resources, Validation, Investigation. CY: Resources, Investigation, Writing—review and editing, Funding acquisition.
Funding
The study is sponsored by the National Natural Science Foundation of China (Grant No. 52069006), the Natural Science Foundation of Hainan (Grant No. ZDYF2022SHFZ060, 421RC489).
Conflict of interest
The authors declare that the research was conducted in the absence of any commercial or financial relationships that could be construed as a potential conflict of interest.
Publisher’s note
All claims expressed in this article are solely those of the authors and do not necessarily represent those of their affiliated organizations, or those of the publisher, the editors and the reviewers. Any product that may be evaluated in this article, or claim that may be made by its manufacturer, is not guaranteed or endorsed by the publisher.
References
Abbaspour, K. C., Yang, J., Maximov, I., Siber, R., Bogner, K., Mieleitner, J., et al. (2007). Modelling hydrology and water quality in the pre-alpine/alpine Thur watershed using SWAT. J. Hydrology 333, 413–430. doi:10.1016/j.jhydrol.2006.09.014
Bajracharya, A. R., Bajracharya, S. R., Shrestha, A. B., and Maharjan, S. B. (2018). Climate change impact assessment on the hydrological regime of the Kaligandaki Basin, Nepal. Sci. Total Environ. 625, 837–848. doi:10.1016/j.scitotenv.2017.12.332
Bao, Z., Zhang, J., Wang, G., Fu, G., He, R., Yan, X., et al. (2012). Attribution for decreasing streamflow of the Haihe River basin, northern China: Climate variability or human activities? J. Hydrology 460-461, 460117–461129. doi:10.1016/j.jhydrol.2012.06.054
Bisht, D. S., Chatterjee, C., Raghuwanshi, N. S., and Sridhar, V. (2017). An analysis of precipitation climatology over Indian urban agglomeration. Theor. Appl. Climatol. 133, 421–436. doi:10.1007/s00704-017-2200-z
Chang, J., Wang, Y., Istanbulluoglu, E., Bai, T., Huang, Q., Yang, D., et al. (2015). Impact of climate change and human activities on runoff in the Weihe River Basin, China. Quat. Int. 380-381, 380169–381179. doi:10.1016/j.quaint.2014.03.048
Chen, B., Li, X., Xiao, X., Zhao, B., Dong, J., Kou, W., et al. (2016). Mapping tropical forests and deciduous rubber plantations in Hainan Island, China by integrating PALSAR 25-m and multi-temporal Landsat images. Int. J. Appl. Earth Observation Geoinformation 50, 117–130. doi:10.1016/j.jag.2016.03.011
DeFries, R., and Eshleman, K. N. (2004). Land-use change and hydrologic processes: A major focus for the future. Hydrol. Process. 18, 2183–2186. doi:10.1002/hyp.5584
Dong, W., Cui, B., Liu, Z., and Zhang, K. (2014). Relative effects of human activities and climate change on the river runoff in an arid basin in northwest China. Hydrol. Process. 28, 4854–4864. doi:10.1002/hyp.9982
Fukunaga, D. C., Cecílio, R. A., Zanetti, S. S., Oliveira, L. T., and Caiado, M. A. C. (2015). Application of the SWAT hydrologic model to a tropical watershed at Brazil. Catena 125, 206–213. doi:10.1016/j.catena.2014.10.032
Giambelluca, T. W., Mudd, R. G., Liu, W., Ziegler, A. D., Kobayashi, N., Kumagai, T., et al. (2016). Evapotranspiration of rubber (Hevea brasiliensis) cultivated at two plantation sites in Southeast Asia. Water Resour. Res. 52, 660–679. doi:10.1002/2015WR017755
Guo, J., Zhang, Z., Wang, S., Peter, S., and Yao, A. (2014). Appling SWAT model to explore the impact of changes in land use and climate on the streamflow in a Watershed of Northern China. Acta Ecol. Sin. 34 (6), 1559–1567.
Heo, J., Yu, J., Giardino, J. R., and Cho, H. (2015). Water resources response to climate and land-cover changes in a semi-arid watershed, New Mexico, USA. Terr. Atmos. Ocean. Sci. 26, 463. doi:10.3319/tao.2015.03.24.01(hy)
Jain, S. K., Tyagi, J., and Singh, V. (2010). Simulation of runoff and sediment yield for a himalayan watershed using SWAT model. J. Water Resour. Prot. 02, 267–281. doi:10.4236/jwarp.2010.23031
Kong, D., Miao, C., Wu, J., and Duan, Q. (2016). Impact assessment of climate change and human activities on net runoff in the Yellow River Basin from 1951 to 2012. Ecol. Eng. 91, 566–573. doi:10.1016/j.ecoleng.2016.02.023
Lai, G., Yu, G., and Gui, F. (2006). Preliminary study on assessment of nutrient transport in the Taihu Basin based on SWAT modeling. Sci. China Ser. D 49, 135–145. doi:10.1007/s11430-006-8113-9
Legesse, D., Vallet-Coulomb, C., and Gasse, F. (2003). Hydrological response of a catchment to climate and land use changes in tropical africa: Case study South Central Ethiopia. J. Hydrology 275, 67–85. doi:10.1016/s0022-1694(03)00019-2
Li, B., Shi, X., Lian, L., Chen, Y., Chen, Z., and Sun, X. (2020). Quantifying the effects of climate variability, direct and indirect land use change, and human activities on runoff. J. Hydrology 584, 124684. doi:10.1016/j.jhydrol.2020.124684
Li, Z., Liu, W., Zhang, X., and Zheng, F. (2009). Impacts of land use change and climate variability on hydrology in an agricultural catchment on the Loess Plateau of China. J. Hydrology 377, 35–42. doi:10.1016/j.jhydrol.2009.08.007
Li, Y., Chang, J., Wang, Y., Jin, W., and Guo, A. (2016). Spatiotemporal impacts of climate, land cover change and direct human activities on runoff variations in the Wei River Basin, China. Water 8 (6), 220. doi:10.3390/w8060220
Lin, B., Chen, X., Yao, H., Chen, Y., Liu, M., Gao, L., et al. (2015). Analyses of landuse change impacts on catchment runoff using different time indicators based on SWAT model. Ecol. Indic. 58, 55–63. doi:10.1016/j.ecolind.2015.05.031
Lin, K., He, Y., and Chen, X. (2012). Identifying the quantitative effect of climate change and human activity on runoff in the Dongjiang River basin. Shuili Xuebao (J. Hydraul. Eng.) 43 (11), 1312–1321. doi:10.13243/j.cnki.slxb.2012.11.001
Lin, K., Zhang, Q., and Chen, X. (2010). An evaluation of impacts of DEM resolution and parameter correlation on TOPMODEL modeling uncertainty. J. Hydrology 394, 370–383. doi:10.1016/j.jhydrol.2010.09.012
Lin, P., Shi, P., Yang, T., Xu, C.-Y., Li, Z., and Wang, X. (2020). A statistical vertically mixed runoff model for regions featured by complex runoff generation process. Water 12, 2324. doi:10.3390/w12092324
Liu, D., Chen, X., Lian, Y., and Lou, Z. (2010). Impacts of climate change and human activities on surface runoff in the Dongjiang River basin of China. Hydrol. Process. 24, 1487–1495. doi:10.1002/hyp.7609
Li., Z., Xu, Y., Sun, Y., Wu, M., and Zhao, B. (2020). Urbanization-driven changes in land-climate dynamics: A case study of haihe river basin, China. Remote Sens. 12, 2701. doi:10.3390/rs12172701
Marhaento, H., Booij, M. J., and Hoekstra, A. Y. (2016). Attribution of changes in stream flow to land use change and climate change in a mesoscale tropical catchment in Java, Indonesia. Hydrology Res. 48, 1143–1155. doi:10.2166/nh.2016.110
Marhaento, H., Booij, M. J., Rientjes, T. H. M., and Hoekstra, A. Y. (2017). Attribution of changes in the water balance of a tropical catchment to land use change using the SWAT model. Hydrol. Process. 31, 2029–2040. doi:10.1002/hyp.11167
Mekonnen, D. F., Duan, Z., Rientjes, T., and Disse, M. (2018). Analysis of combined and isolated effects of land-use and land-cover changes and climate change on the upper Blue Nile River basin’s streamflow. Hydrol. Earth Syst. Sci. 22, 6187–6207. doi:10.5194/hess-22-6187-2018
Morán-Tejeda, E., Ceballos-Barbancho, A., and Llorente-Pinto, J. M. (2010). Hydrological response of Mediterranean headwaters to climate oscillations and land-cover changes: The mountains of Duero River basin (Central Spain). Glob. Planet. Change 72, 39–49. doi:10.1016/j.gloplacha.2010.03.003
Nie, W., Yuan, Y., Kepner, W., Nash, M. S., Jackson, M., and Erickson, C. (2011). Assessing impacts of Landuse and Landcover changes on hydrology for the upper San Pedro watershed. J. Hydrology 407, 105–114. doi:10.1016/j.jhydrol.2011.07.012
Op de Hipt, F., Diekkruger, B., Steup, G., Yira, Y., Hoffmann, T., Rode, M., et al. (2019). Modeling the effect of land use and climate change on water resources and soil erosion in a tropical West African catch-ment (Dano, Burkina Faso) using SHETRAN. Sci. Total Environ. 653, 431–445. doi:10.1016/j.scitotenv.2018.10.351
Pan, S., Liu, D., Wang, Z., Zhao, Q., Zou, H., Hou, Y., et al. (2017). Runoff responses to climate and land use/cover changes under future scenarios. Water 9, 475. doi:10.3390/w9070475
Sharifi, A., Mirabbasi, R., Ali Nasr-Esfahani, M., Torabi Haghighi, A., and Fatahi Nafchi, R. (2021). Quantifying the impacts of anthropogenic changes and climate variability on runoff changes in central plateau of Iran using nine methods. J. Hydrology 603, 127045. doi:10.1016/j.jhydrol.2021.127045
Sood, A., Muthuwatta, L., and McCartney, M. (2013). A SWAT evaluation of the effect of climate change on the hydrology of the Volta River basin. Water Int. 38, 297–311. doi:10.1080/02508060.2013.792404
Sun, F., Mejia, A., Zeng, P., and Che, Y. (2019). Projecting meteorological, hydrological and agricultural droughts for the Yangtze River basin. Sci. Total Environ. 696, 134076. doi:10.1016/j.scitotenv.2019.134076
Tan, M. L., Tew, Y. L., Chun, K. P., Samat, N., Shaharudin, S. M., Mahamud, M. A., et al. (2021). Improvement of the esa CCI land cover maps for water balance analysis in tropical regions: A case study in the muda river basin, Malaysia. J. Hydrology Regional Stud. 36, 100837. doi:10.1016/j.ejrh.2021.100837
Tan, X., Liu, S., Tian, Y., Zhou, Z., Wang, Y., Jiang, J., et al. (2022). Impacts of climate change and land use/cover change on regional hydrological processes: Case of the guangdong-Hong Kong-Macao greater bay area. Front. Environ. Sci. 9. doi:10.3389/fenvs.2021.783324
Tan, Z.-H., Zhang, Y.-P., Song, Q.-H., Liu, W.-J., Deng, X.-B., Tang, J.-W., et al. (2011). Rubber plantations act as water pumps in tropical China. Geophys. Res. Lett. 38. doi:10.1029/2011GL050006
Vorosmarty, C. J., Green, P., Salisbury, J., and Lammers, R. B. (2000). Global water resources: Vulnerability from climate change and population growth. Science 289, 284–288. doi:10.1126/science.289.5477.284
Wang, Y., Zhang, Q., Wang, J., and Zhang, L. (2017). Appling SWAT model to explore the impacts of land use and climate changes on the hydrological characteristics in Taohe River Basin. J. Desert. Res. 37 (1), 175. doi:10.7522/j.issn.1000-694X.2015.00189
Wu, J., Chen, X., Yu, Z., Yao, H., Li, W., and Zhang, D. (2019). Assessing the impact of human regulations on hydrological drought development and recovery based on a ‘simulated-observed’ comparison of the SWAT model. J. Hydrology 577, 123990. doi:10.1016/j.jhydrol.2019.123990
Wu, L., Wang, S., Bai, X., Luo, W., Tian, Y., Zeng, C., et al. (2017). Quantitative assessment of the impacts of climate change and human activities on runoff change in a typical karst watershed, SW China. Sci. Total Environ. 601 (602), 1449–1465. doi:10.1016/j.scitotenv.2017.05.288
Wu, L., Zhang, X., Hao, F., Wu, Y., Li, C., and Xu, Y. (2020). Evaluating the contributions of climate change and human activities to runoff in typical semi-arid area, China. J. Hydrology 590, 125555. doi:10.1016/j.jhydrol.2020.125555
Yuan, Y., Zhang, Z., and Meng, J. (2015). Impact of changes in land use and climate on the runoff in Liuxihe Watershed based on SWAT model. Chin. J. Appl. Ecol. 26 (4), 989–998.
Zeng, S., Xia, J., and Du, H. (2013). Separating the effects of climate change and human activities on runoff over different time scales in the Zhang River basin. Stoch. Environ. Res. Risk Assess. 28, 401–413. doi:10.1007/s00477-013-0760-8
Zhan, C. S., Jiang, S. S., Sun, F. B., Jia, Y. W., Niu, C. W., and Yue, W. F. (2014). Quantitative contribution of climate change and human activities to runoff changes in the Wei River basin, China. Hydrol. Earth Syst. Sci. 18, 3069–3077. doi:10.5194/hess-18-3069-2014
Zhang, A., Zhang, C., Fu, G., Wang, B., Bao, Z., and Zheng, H. (2012). Assessments of impacts of climate change and human activities on runoff with SWAT for the huifa river basin, northeast China. Water Resour. manage. 26, 2199–2217. doi:10.1007/s11269-012-0010-8
Zhang, H., Wang, B., Liu, D. L., Zhang, M., Leslie, L. M., and Yu, Q. (2020). Using an improved SWAT model to simulate hydrological responses to land use change: A case study of a catchment in tropical Australia. J. Hydrology 15, 124822. doi:10.1016/j.jhydrol.2020.124822
Zhang, X., Srinivasan, R., and Liew, M. V. (2010). On the use of multi-algorithm, genetically adaptive multi-objective method for multi-site calibration of the SWAT model. Hydrol. Process. 24, 955–969. doi:10.1002/hyp.7528
Zhang, Y., Zhong, P., Chen, J., Bing, J., Xu, D., and Wang, M. (2017). Impacts of climate change and human activities on the three gorges reservoir inflow. Water 9, 957. doi:10.3390/w9120957
Zhao, G., Tian, P., Mu, X., Jiao, J., Wang, F., and Gao, P. (2014). Quantifying the impact of climate variability and human activities on streamflow in the middle reaches of the Yellow River basin, China. J. Hydrology 519, 387–398. doi:10.1016/j.jhydrol.2014.07.014
Zhao, Y., Zou, X., Gao, J., Xu, X., Wang, C., Tang, D., et al. (2015). Quantifying the anthropogenic and climatic contributions to changes in water discharge and sediment load into the sea: A case study of the Yangtze River, China. Sci. Total Environ. 536, 803–812. doi:10.1016/j.scitotenv.2015.07.119
Zhao, Y., Zou, X., Liu, Q., and Chen, Y. (2018). Impacts of climate variability and human activities on streamflow in the Wanquan river basin along the east coast of hainan island, southern China. J. Coast. Res. 35, 410–419. doi:10.2112/JCOASTRES-D-18-00005.1
Zheng, H., Zhang, L., Zhu, R., Liu, C., Sato, Y., and Fukushima, Y. (2009). Responses of streamflow to climate and land surface change in the headwaters of the Yellow River Basin. Water Resour. Res. 45. doi:10.1029/2007WR006665
Zhou, Y., Lai, C., Wang, Z., Chen, X., Zeng, Z., Chen, J., et al. (2018). Quantitative evaluation of the impact of climate change and human activity on runoff change in the Dongjiang river basin, China. Water 10. doi:10.3390/w10050571
Keywords: climate change, human activities, land use change, runoff, Wanquan River Basin
Citation: Li D, Zhu L, Xu W and Ye C (2022) Quantifying the impact of climate change and human activities on runoff at a tropical watershed in South China. Front. Environ. Sci. 10:1023188. doi: 10.3389/fenvs.2022.1023188
Received: 22 August 2022; Accepted: 18 October 2022;
Published: 04 November 2022.
Edited by:
Venkatramanan Senapathi, Alagappa University, IndiaReviewed by:
Sivakumar Karthikeyan, Alagappa University, IndiaVasanthavigar Murugesan, Alagappa University, India
Yifei Zhao, Nanjing Normal University, China
Copyright © 2022 Li, Zhu, Xu and Ye. This is an open-access article distributed under the terms of the Creative Commons Attribution License (CC BY). The use, distribution or reproduction in other forums is permitted, provided the original author(s) and the copyright owner(s) are credited and that the original publication in this journal is cited, in accordance with accepted academic practice. No use, distribution or reproduction is permitted which does not comply with these terms.
*Correspondence: Changqing Ye, yechangqing2001@hotmail.com
†These authors have contributed equally to this work