- 1Botany and Ecology Department, Federal University of Mato Grosso (UFMT), Cuiabá, Brazil
- 2Pantanal Research Center (UFMT), Cuiabá, Brazil
- 3Department of Ecology, Universidad de Alicante, Alicante, Spain
- 4Multidisciplinary Institute for Environmental Studies (MIES), Universidad de Alicante, Alicante, Spain
- 5Department of Ecology and Zoology, Federal University of Santa Catarina, Florianópolis, Brazil
- 6Department of Sanitary and Environmental Engineering, Federal University of Mato Grosso (UFMT), Cuiabá, Brazil
- 7Argentina The National Institute of Limnology (INALI; CONICET-UNL), Ciudad Universitaria, Santa Fe, Argentina
Microplastics (<5 mm) can have negative and deleterious environmental effects. However, the scarce information on the levels of microplastics and the factors underlying their input in wetlands, limits the understating of the vulnerability of these ecosystems to microplastics. To contribute closing this important information gap, the concentration (items/m−2), size and the variables related to the distribution of microplastics in fluvial sediments of the Cuiabá River, a tributary of the Pantanal, the world largest flooded Savanna and a UNESCO World Heritage were investigated. The mean microplastic level was 576.8 ± 577.8 items/m−2 of sediments which were mainly fragments (309.0 ± 319.6 items/m−2) and fibers (267.8 ± 339.9 items/m−2). The maximum level was 1938.8 items/m−2 found in the urban stretch of the sister cities of Cuiabá and Várzea Grande, the largest urban agglomeration in the study area. Unexpectedly, the composition of the microplastic with highest average level (240.7 ± 310.4 items/m−2), a blue synthetic fiber, could not be unequivocally determined by RAMAN spectrometry. Its complex composition includes Pb, Li and Nb, suggesting it may derive from electronic waste. Polypropylene (159.2 ± 133.4 items/m−2) and Polyvinyl Chloride (104.6 ± 217.2 items/m−2) were the second and third polymers with higher average levels. The average size of microplastics particles in sampling sites ranged from 0.18 mm to 1.74 mm. Microplastic concentration was positively related to urban areas. Similarly, microplastics particles size decreased with distance from urban areas. These results suggest that urban areas along the Cuiabá River, with poor waste management, are a major source of microplastic pollution in the Pantanal floodplain and that electronic waste might contribute significantly to this contamination. This study highlights the importance of adequate waste management in cities to limit microplastic contamination in areas of ecological importance, such as the Pantanal.
Introduction
In many parts of the world the destination of solid waste varies. It can be adequately disposed in sanitary landfill, or controlled landfills, inadequately in dumps or simply throwed out wherever the locals see fits (Geyer et al., 2017; Abrelpe, 2020; Wayman and Niemann, 2021). Solid waste, when improperly discarded, can reach rivers and be transported downstream (Geyer et al., 2017; Schmidt et al., 2017; Emmerik et al., 2019). As a result, aquatic environments may be contaminated by particles of anthropogenic origin with complex composition, including polymers and metals, such as paint particles, cellulose, dyed particles, rubber, and plastics (Fendall and Sewell, 2009; Naidu et al., 2016). Brazil is the largest producer of thermoplastic resins and processed plastics in South America, reaching up to 15.5 million tons in 2018 (Abiplast, 2019). From the non-organic solid waste generated, plastic is the most abundant, followed by, paper, textiles, leather, rubber, tailings and electronics (Abrelpe, 2020).
Microplastics are plastic particles smaller than 5 mm, which can be classified as primary or secondary (Arthur et al., 2009; Cole et al., 2011). Primary microplastics are polymers produced on an industrial scale and specifically in these sizes, such as small microspheres and glitters (Horton and Dixon, 2018). Secondary microplastics are formed by the fragmentation of larger plastics, which occur by biotic or abiotic processes (Andrady, 2011).
There are several factors associated with the amount of microplastic particles in aquatic systems, such as human population density in the drainage basin, and agricultural, industrial activities and mainly the sewage treatment plants (Peng et al., 2017; Blettler et al., 2018; De Falco et al., 2018; Townsend et al., 2019; Park et al., 2020). The transport and distribution of these particles can be influenced by hydrological events (Hurley et al., 2018), seasonal variations (Xia et al., 2021), river flow, as well as particle size (Nizzetto et al., 2016) and density (He et al., 2020). Processes such as aggregation to biofilms (Chen et al., 2019) or encapsulation in fecal plots, also play a role in their transport, deposition in sediments or availability to organisms (Cole et al., 2016; Moreschi et al., 2020). Additionally, atmospheric transport can also be a relevant driver of microplastics distribution in aquatic environments (Horton and Dixon, 2018), even at locations distant from microplastic sources (Allen et al., 2019; Klein and Fischer, 2019; Bullard et al., 2021).
Although many studies have focused on microplastics contamination of water column, little is known about the microplastics contamination in sediments (but see Yao et al., 2019 for a review). This research-bias may lead to incomplete picture about the microplastic contamination of aquatic environments, as sediments can act as sink of microplastics, especially the particles with a density higher than that of the overlying water compartments (Jambeck et al., 2015; Van Cauwenberghe et al., 2015; Woodall et al., 2014). Therefore, sediments can regulate the distribution of microplastics within the aquatic environments. Studies of microplastics in sediments of freshwater environments have indicated that microplastics can be very abundant in urban rivers with high human population density and large commercial and industrial areas (Shruti et al., 2019; He et al., 2020). Microplastics found in these sediments are mainly of secondary origin, arising from the fragmentation of larger plastics or fibers from synthetic clothes washing (Blair et al., 2019). In Amazonian rivers in Brazil, for example, fiber microplastics predominated, especially in the vicinity of the city of urban areas (Gerolin et al., 2020).
In wetlands, the concentration of microplastics may depend on habitat type (Helcoski et al., 2020), population density (Scheurer and Bigalke 2018), or degree of industrialisation (Townsend et al., 2019). Although studies on the presence and distribution of microplastics are growing in wetlands, they are mainly focused on coastal wetlands, being specially scarce in inland wetlands (Qian et al., 2021). Inland wetlands differ from coastal ones in hydrology and vegetation assemblages and provide important ecosystem services such as water storage, sediment retention, among others (Nunes da Cunha et al., 2007; Junk et al., 2014). The Pantanal, a UNESCO World Heritage, is the largest American continental wetland and it is a largely suffering from anthropogenic pressures (Junk et al., 2006; Alho et al., 2019). Microplastics are an emerging environmental threat, however, in the Pantanal, only one study on microplastics in the water column was found in the literature (De Faria et al., 2021). To the best of our knowledge, this is the first study on microplastic contamination in the Pantanal sediments.
The aim of this study was to characterize the level, size, and composition of microplastic in the sediment of the margins of Cuiabá River, one of the main tributaries of the Pantanal. Specifically the study aimed at: i-determining how the microplastic level, composition and size were influenced by the proximity of human settlements of different areas and population along the Cuiabá River, ii-assessing whether the distance from the largest urban area (Cuiabá metropolitan region) was a preponderant factor to explain concentration, composition and size of microplastics; and iii-understanding whether the land use (urban, peri-urban rural) was a relevant factor to explain variations in microplastic levels and composition.
Materials and methods
Study area
This study was carried out on the fluvial beaches of the Cuiabá River (Figure 1), one of the main tributaries of the Pantanal (Girard, 2011). The Cuiabá River basin is located in the State of Mato Grosso (Brazil), and has great environmental and economic importance, covering an area of 22,851.1 km2 (Chiranda et al., 2016). Along its length, the Cuiabá River passes through the metropolitan area of Cuiabá (∼864,000 inhabitants). In this metropolitan area, ca. 50% of the wastewater is neither collected nor treated (De Faria et al., 2021). Downstream of this dense urban area, the river crosses the cities of Santo Antônio de Leverger (18,000 inhabitants), with only 10.4% of the wastewater being treated (De Faria et al., 2021) and Barão de Melgaço (8,164 inhabitants), before continuing its course in the Pantanal floodplains.
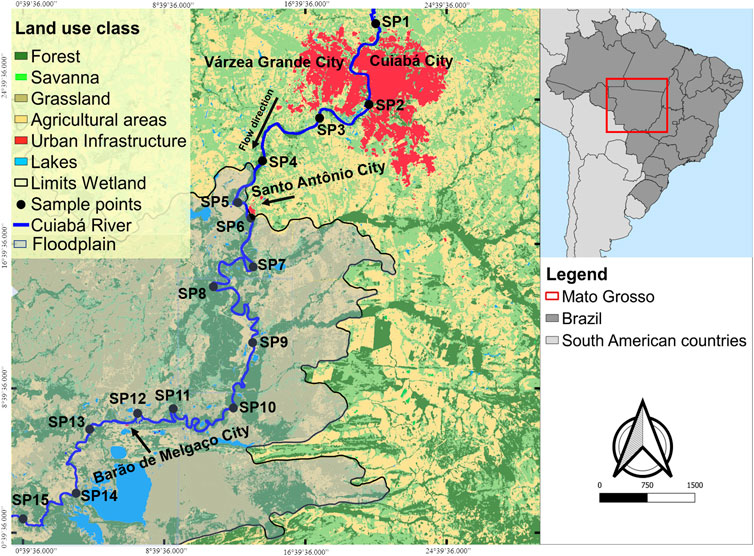
FIGURE 1. Cuiabá River map representing locating sampling sites and the main land use. Sites one to four located on the Plateau highlands and sites 5–15 are located on the Pantanal floodplain lowlands. The black line delimits the Plateau- Floodplain boundary. The floodplain (wetlands) area is shaded and lies at the west of this limit as shown on the map. Cuiabá River flows from NE to SW. Sp–Sampling sites.
The Pantanal is a large floodplain of approximately 160,000 km2 inserted in the Upper Paraguay River basin (Junk et al., 2006). It is located on a tectonic fault characterized by a graben in which sediments have been deposited since the beginning of the Tertiary (Irion et al., 2011). The sediments can be found in different proportions such as fine and coarse sand, silt and clay (Ussami et al., 1999). The study area is mainly made up of two major physiographic regions: ‘Plateau’ or highlands (altitudes from 250 to 750 m) surrounding the ‘Planície’ or lowlands (altitudes from 100 to 180 m) which is the floodplain and wetlands area called the Pantanal (Girard, 2011). The north-south and east-west gradients of the Pantanal plain are of the order of 10 cm per km and when rivers coming from the highlands enter it, the speed of water flowing in these channels is dramatically reduced (Girard, 2011). The region is marked by well-defined rainy and dry seasons that cause periods of floods and droughts (Junk et al., 2006). The main rivers that flow into the Pantanal are: the Paraguay River (annual mean discharge = 482 m³/s), the Cuiabá River (386 m2/s), the São Lourenço River (344 m³/s), the Taquari River (322 m³/s), the Miranda River (101 m³/s) and the Aquidauana River (128 m³/s). In the Cuiabá River basin, the flooding period coincides with the rainy season and takes place from December to April while the drought occurs from June to September (Girard, 2011). The sediment sampled in this study were eroded in the higher part of the surrounding highland and transported to the Pantanal by the rivers flowing there. Ground water in the study area was previously investigated by Girard et al. (2003). It is confined below clay–silt layers and flow from the river towards the floodplain where it sustains channel flow and to maintains soil humidity in depressed areas during the dry period.
Sampling sites
Sediment samples were taken from emerged fluvial beaches on rivers banks, in early August 2020, during the dry season, when these areas were exposed and more easily accessed. A total of 15 sites were sampled in triplicate, totaling 45 samples collected along the course of the Cuiabá River (Figure 1), from the metropolitan area of Cuiabá (in the highlands area) to the interior of the Pantanal, more than 190 km downriver from the capital. One site was located just upstream of the densely populated Cuiabá metropolitan area, another into this metropolitan area, a third one immediately downstream of the densely populated area. Close to the two urban agglomerations located in the Pantanal, namely Santo Antônio and Barão de Melgaço, sites were located immediately upstream and downstream of these cities. The distribution of the other eight sampling sites covered stretches of river between and downstream from these cities (Figure 1). Note that sites one to four are along a reach of the Cuiabá where river slope and water velocity are higher than in the Pantanal lowlands. The other sites are in the Pantanal floodplain were slope and velocity are lower (Girard, 2011). Samples 4, 5, and 6 were collected in the transition zone between the high and lowlands.
For sediment collection, a 25 cm × 25 cm (0.0625 m2) wooden quadrat and a stainless-steel shovel was used and a depth of 3 cm of sediments was taken from surface (Klein et al., 2015). At each sampling site, both the quadrat and the stainless-steel shovel were washed with distilled water, to avoid cross contamination between the sampling sites. Afterwards, samples were transported to the Chemistry Laboratory of the Federal University of Mato Grosso.
To determine the types of land use and occupation at a sampling site the public use data provided by MAPIBIOMAS (2021) (https://mapbiomas.org/) was used and treated with the QGIS 3.10 software. Land use and occupation determination were defined using a 3 km radius circle centered on the sampling site, for an area about 28 km2 (see Table 1 in supplementary material). This criterion was selected to avoid overlapping areas. The land use type and occupation were delimited in that perimeter using a mask in the raster image. This classification resulted in the following categories: urban, agricultural, (which includes pasture and agricultural land uses), forest formation, grassland formation and savannah. The relative area of each of these land use categories was expressed in km2.
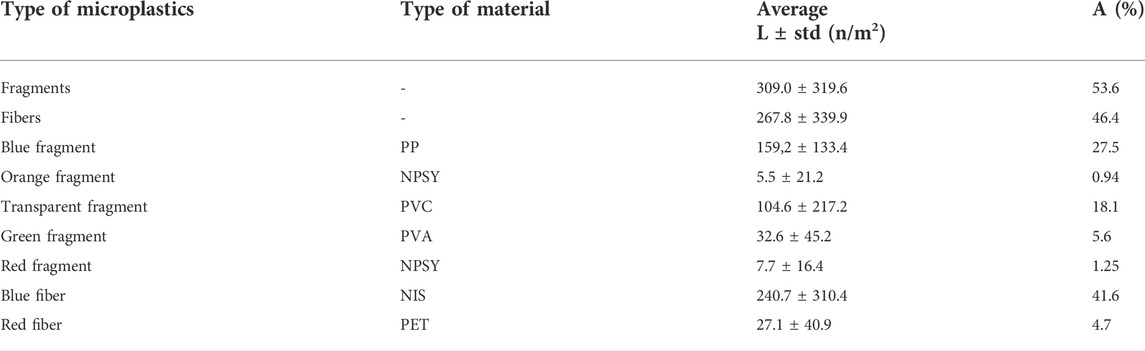
TABLE 1. Microplastics in the study area. PP–Polypropylene, NPSY–Naplesyl, PVC–Polyvinyl Chloride, PVA–Polyvinyl alcohol, NIS–Non-identified Synthetic, PET–Polyethylene terephthalate. L stands for levels and std for standard deviation. A is the relative abundance for the study area.
Sample treatment and microplastic identification
Samples were processed adapting the protocol suggested by Masura et al. (2015) for microplastics, which consists in isolating these by first digesting the organic matter and then separating the mineral fraction by gravimetry. Properly labeled sediment samples were weighed and oven-dried at a temperature of 60°C for 24 h. And then weighed again. Subsequently, 250-g sediment aliquots were taken for microplastic analysis.
For organic matter digestion, sediment was transferred to a 1 L beaker properly decontaminated and washed with distilled water. Aliquots were subjected to a 30% solution of hydrogen peroxide until there was no more visible reaction with the organic matter. On average, 100 ml hydrogen peroxide were used in each sample. This process lasted an average of 24 h, varying more or less, depending on the type and amount of organic matter in the sediment.
For the separation of microplastic from the sediment, the protocols of Frias et al. (2018) for microplastics were adopted. In this technique, a NaCl solution (360 g, d = 1.2 g/cm³) was added to each sample covering three times the volume of sediment in the beaker. The solution was then stirred for 1 min and filtered after resting for 1 h. Since the density of the vast majority of plastics is less than 1.2 g/cm−³, they separated from the sediment by gravimetry and concentrated in the supernatant. Then, the supernatant was filtered through filtration equipment containing a glass fiber microfilter (GF-4 Macherey-Nagel) 47 mm in diameter and 1.4 micron pores. This procedure was repeated three times for each sample.
Contamination prevention and assessment
To prevent contamination in collections and the laboratory, clothes made from synthetic fibers were avoided, in addition to the mandatory use of a cotton lab coat. All lab equipment was washed with distilled water and all containers used in sample processing were made of glass, and those being handled were kept covered with aluminum foil to avoid air contamination Frias et al. (2018). In addition, Petri dishes containing filters identical to those used to separate the microplastics were distributed throughout the laboratory so that the laboratory air contamination could be evaluated. Sample blanks were processed in the same way as sediments, which allowed the assessment of contamination not only by air, but also by the solutions used in the treatment of sediments. Three blanks were run at each time samples were processed.
Microscopic analysis
All the filters were analyzed with a binocular magnifying glass with the aid of a transparent circular template divided into eight sections that were placed at the top of the Petri dish. Each filter was analyzed section by section and for each possible microplastics, one picture has been made. Regarding the classification of morphology, colors and polymers, microplastics were classified according to Frias et al. (2018). Microplastics identified at this step were counted and regrouped according to their type and color. The particle size was measured by analyzing the pictures of each microplastic confirmed by RAMAN (Chen et al., 2019; Prata et al., 2019; De Faria et al., 2021).
RAMAN analysis and microplastic concentration
RAMAN spectrometry (NRS-5100, Jasco, Madrid, Spain equipped with LMU-20X-UVB lens) was used to determine the composition of selected microplastics representing the groups of microplastics previously identified. The laser excitation frequency and intensity used were 784.79 nm and 11.8 mW, respectively. RAMAN spectra were recorded with a charge-coupled device camera (UV-NIR range; 1,024 × 255 pixels) electrically cooled to 70°C. RAMAN spectra were obtained between 162 and 1,886 cm−1 with a spectral resolution of 2.47 cm−1.
The RAMAN spectra found were compared to the spectral library (Open Specy) (Cowger et al., 2020). The identification of the material composing the microplastics was considered conclusive when the correlation between the spectrum of the particle under analysis was correlated with the reference spectrum of the Open Specy Library with a Pearson r ≥ 0.7. For highly degraded particles, the database SloPP-E was used (Munno et al., 2020).
Particles that were not identified as synthetic by RAMAN were excluded from microplastic counts. The results are expressed either in levels or abundances. The level (L) of particles for each sample was obtained by:
where n is the number of synthetic particles in the 250 g aliquot and W is the weight (g) of the dry sample obtained in 0.0625 m2. L is expressed in items/m−2.
Data analysis
First, a descriptive analysis of the data was carried out to analyze the relative abundances of each particle type, colors, amount of microplastics identified at each sampling site, their concentration and the particle sizes.
To determine which environmental variables best correlated to the total concentration, fiber concentration, fragment concentration and sizes of microplastics, a multiple regression was performed. Initially, the variables that were considered relevant were forest area, savannah area, grassland area, urban area, agricultural area and river path distance (see Supplementary Table S1 for the results of this classification). Before multiple regression analysis, collinear variables (r > 0.6) were removed. Grassland and savannah formations were removed following this criterion. The multiple regression model of the total concentration of microplastics for the analysis was as follows: total density ∼ urban areas + agricultural areas + forest + distance of potential source (metropolitan region). The same model was performed for fiber concentration, fragment concentration and microplastic sizes. A Breusch-Pagan test was performed to check homoscedasticity and a Shapiro-Wilk test was performed in the residuals of the regression model to check for normality. When normality assumption was not met, the data were transformed following the Box-Cox technique. This technique identifies adequate data normalization. In this case, for total density (λ = 0) a Log10 transformation was used, and fiber density (λ = 0.5), a square root transformation was used. The microplastic sizes (mm) followed a normal distribution and were standardized by the mean and plotted with standard error.
As the previous analysis reduced land use to only urban and non-urban (agricultural) areas, it was further subdivided following the classification of the Brazilian Geography Institute (IBGE, 2000) and classified as urban (high habitational density), peri-urban (lower habitational area) and rural (mainly agricultural). The non-metric multidimensional scaling (NMDS) analysis was then applied to the sampling sites according to the composition of microplastic based on the Bray-Curtis distance.
One PERMANOVA analysis was performed to test whether the polymer composition differed among urban, peri-urban and rural areas. Multivariate analyses were performed by means of R software (R CORE TEAM, 2020) and the ‘Vegan’ package (Oksanen et al., 2013). All statistical tests were conducted with a significance level of α = 0.05.
Results
Concentrations, types, colors, sizes and composition
In this study, an average of 576.8 ± 557.8 items/m−2 was found in fluvial beaches, from which 309.0 ± 319.6 items/m−2 were fragments and 267.8 ± 339.6 items/m−2 were fibers. Fragments represented 53.6% of all counted particles and were encountered at all sampling sites. Fibers were absent only at sampling site 14 (Table 1 and Supplementary Table S2) and made up 46.4% of all particles. Example of microparticles of this study can be seen in Supplementary Figure S1 in the supplementary material.
In general, as can be seen in Table 1, the blue synthetic fibers, which had RAMAN spectra that did not match any type of known material of the library used (Cowger et al., 2021; non-identified blue fibers synthetic–NIS, 240.7 ± 310.4 items/m−2) and the blue fragments (Polypropylene - PP, 159.2 ± 133.4 items/m−2) were dominant. Example of NIS and PP can be visualized in Supplementary Figure S1A and Supplementary Figure S1C in the supplementary material, respectively.
NIS highest levels (1174.2 items/m−2) were found at sampling site 2, which is located in the river stretch that crosses the Cuiabá metropolitan urban area, and at sampling site 5 (651.5 items/m−2), which is the first point in the Pantanal lowlands, at the border with the highlands area (Figures 1, 2). PP was found at all sampling sites with the highest level at site 5 (488.6 items/m−2). Polyvinyl chloride particles (PVC, transparent fragments, (Supplementary Figure S1F), highest level was found at sampling site 11 (839.9 items/m−2) in the Pantanal floodplain, close-by to an open-air dump containing construction waste. Note that dumping is frequent along the Cuiabá River. However, they were absent at sampling sites 1, 4, 7–9, and 12–15. Polyvinyl alcohol particles (PVA, green fragments) were found at sites 2–8 and 10, that is, they had little expression in the Pantanal. Polyethylene terephthalate (PET, red fibers, Supplementary Figure S1B) were only present in sites 1, 2, 4–6 and 10, i.e., almost absent in the Pantanal lowlands. Naples ink particles (NPSY, Supplementary Figure S1E) were present in small amounts only in sites 3, 4, 8, and 9. The RAMAN spectra for each type of identified or non-identified polymers are given in the supplementary material (Supplementary Figure S2, S3).
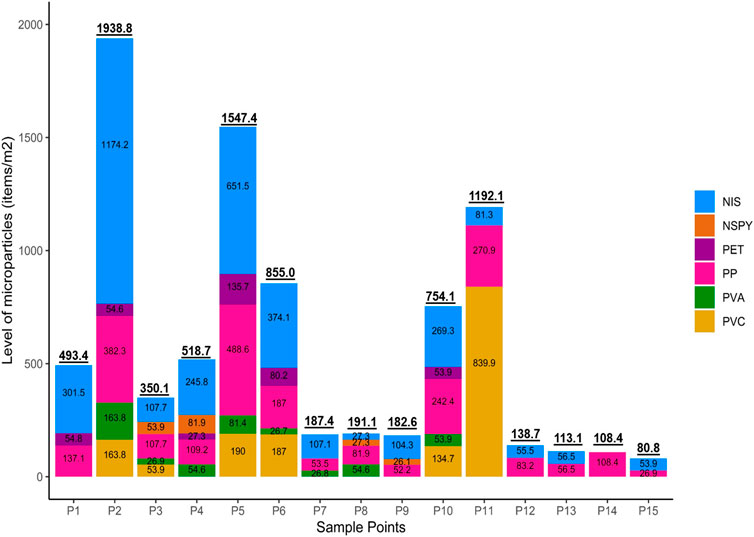
FIGURE 2. Microplastic composition at each sampling site. NIS–Non-identified synthetic; NPSY–Ink particles; PET - Polyethylene terephthalate; PP–Polypropylene; PVA - Polyvinyl alcohol; PVC - Polyvinyl Chloride. The numbers in the bars are the level of a specific type of microparticle. The numbers on top of the bars are the total level of microplastic at that site. Sites one to four are located in the Plateau highlands. Sites 5 to 15 are located in the Pantanal floodplain (See Figure 1).
The maximum levels of anthropogenic microparticles (identified polymers and NIS) were found in the urban stretch of the Cuiabá River (1938.8 items/m−2), followed by sampling site 5 (1547.5 items/m−2) and sampling site 11 (1192.1 items/m−2). The lowest level (80.8 items/m−2) was found inside the Pantanal at sampling site 15 (Figure 2).
Mean microparticles sizes were in general larger in the highlands than in the Pantanal floodplain. In the highlands, the sizes of the microplastic particles are greater than 1 mm except at sampling site 3 (just downstream of the metropolitan area, Figure 1). The largest mean size found on the plateau was at sampling site 1 (1.74 mm ± 1.38 mm). In the Pantanal floodplain, nine of the 11 mean particle size were below 1 mm. The mean sizes greater than 1 mm were found at sampling sites 6 (1.36 ± 0.86) and 5 (1.16 mm ± 1.05 mm), just downstream of the Cuiabá River at the entry in the floodplain, where the river water velocity is known to decrease. The smallest mean sizes in the study area were found in the Pantanal floodplain at sampling sites 14 (0.18 mm ± 0.07 mm) and 12 (0.46 mm ± 0.47 mm).
Relationships between microparticles composition and environment variables
Among the variables initially used for the multiple linear regression (forest area, savannah area, grassland area, urban area, agricultural area and river path distance), only the urban area (km2) within the 3 km radius circle centered on the sampling site was significantly related to the total concentration of microparticles (items/m−2) and total fiber concentration (items/m−2). Regarding the size of microparticles, only the distance from the metropolitan region was significantly related. The coefficient of determination showed that the total concentration of microparticles increased as a function of the urban area (R2 = 0.29, p = 0.03, Figure 3A). The same followed for total fiber concentration, which also increased with the urban area (R2 = 0.56, p = 0.001, Figure 3B). The size of the particles (mm) decreased with increasing distance from the metropolitan area of the city of Cuiabá and Várzea Grande, (R2 = 0.55, p = 0.001, Figure 3C).
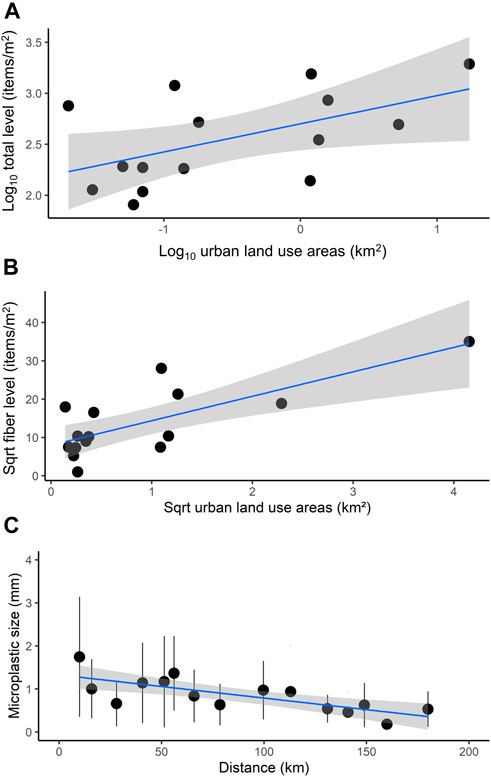
FIGURE 3. Statistically significant microplastics relationships with environmental variables. (A) Microplastic level in function of urban land use areas - log10 transformation (figure a) R2 = 0.29 (p = 0.03). (B) Fiber level in function of urban land use areas - sqrt transformation (figure b) R2 = 0.56 (p = 0.001). (C) Microplastic size in relation to the distance from the Metropolitan region (figure c) R2 = 0.55 (p = 0.001). Urban land use areas are from Supplementary Table S1 (see Sampling Sites in Materials and Methods).
The PERMANOVA analysis demonstrated that polymer composition was not statistically different among the urban areas, peri-urban areas and rural areas (R2 = 0.18; p = 0.26).
Discussion
The mean microparticles levels found in this study were higher than those found in the sediment of other river basins located in other regions of the world (Table 2). For example, the levels of microparticles along the La Plata River in Argentina (Pazos et al., 2021), the beaches in Dubai (Aslam et al., 2020), and of Vembanad Lake, in southern India (Sruthy and Ramasamy, 2017) are quite lower. The high microparticles level found in Pantanal is similar to the one found in a remote lake in Tibet, China (Zhang et al., 2016). However, they were lower than the microparticle levels recorded on the shores of one of the largest Argentinian lakes on the Paraná River floodplain, the Setúbal Lake (Blettler et al., 2017), the shores of the Paraná River in Argentina (Blettler et al., 2019), and the North Shore of Lake Garda, a subalpine Italian lake (Imhof et al., 2013). The population density and/or land use in these basins worldwide do not seem to influence the microplastic levels because sites like Argentina, India, Italia, and Dubai are more densely populated than the fluvial beaches of the Cuiabá River (Pantanal) and they are located mostly in urban and peri-urban locations while the sites of this study are mostly non-urban. Furthermore, some of these sites have lower or higher microplastics concentrations regardless of human population size. The only similar level in these examples, comes from a site in Tibet far more remote and low-density population than in this study.
In contrast, the total concentration of anthropogenic microparticles and fibers (items/m−2) was found to increase with the urban area. Although the metrics to express anthropogenic areas are different, previous studies have shown that human population density and land use area influence the concentrations of microplastics (Yonkos et al., 2014; Fan et al., 2019). Other more recent studies have also associated higher concentrations close to urban areas than in natural or agricultural areas on the Arvand River (Soltani et al., 2022). In this study, secondary microplastics that are characteristically influenced by urban areas were mainly encountered, as also found on the St. Lawrence River in Canada (Crew et al., 2020). In other regions of the world microplastics were related to the fishing activities, tourism or social development (Fan et al., 2019; Li et al., 2022).
In wetlands, the concentration of microplastics is also related to the increase in human population (Scheurer and Bigalke, 2018), or the proportion of industrial areas (Townsend et al., 2019). Furthermore, the concentration of microplastics in the urban river and lakes may tend to decrease with the distance from large urban centers, and may be related to the strong anthropic activity (Wang et al., 2017; Luo et al., 2019). Despite of these urbanization-mediated input microplastics, in this study, the composition of anthropogenic microparticles did not change between urban and rural areas. For instance, blue fibers and fragments are found in almost all the sampling sites. These findings may indicate that the secondary microplastics came from the same parent material both in urban and rural areas or these particles can also be transported by air (Horton and Dixon, 2018).
Larger microplastics tend to settle at low flows, unlike smaller particles that are more easily transported by water over longer distances (Nizzetto et al., 2016; Tibbetts et al., 2018). The sediment transport in the Cuiabá River is initially influenced by the morphological characteristics of the river, such as sinuosity, seasonal flows and the interaction with other tributaries (Hamilton, 2002; Pupim, 2014). In this study, larger synthetic particles were not only observed at sampling sites closer to urban areas but were also found in the highlands-lowlands transition sites (sites four–6). There, particles were larger than at sites immediately up or downriver. The highlands-lowlands transition is typically marked by a topographic depression and flattening typically associated with a drop in current velocity and greater sediment deposition when compared to the plateau region (Assine et al., 2015). This change in hydrodynamic conditions would force the settling of larger microplastic particles as we observed. Conversely, in the floodplain, particles sizes are generally finer, probably due to the fact that in the Pantanal the current speed reduces drastically and, like the sediments, the finer microplastics tend to be deposited (De Faria et al., 2021).
Blue fibers were the most frequent microparticles in the study area (found in 14 of the 15 sampling sites) and those with higher concentrations. Visually they were characterized as microplastics, but surprisingly, their RAMAN analysis revealed that their spectra did not correspond to a plastic polymer spectrum listed in Open Specy. Recently, anthropogenic particles with spectra not related to plastics have been reported and described in the water and sediment of Lake Simcoe, Canada (Felismino et al., 2021) or in Antarctica (Fragão et al., 2021). In this study, the Open Specy spectra that correlated better with the blue fiber spectrum were those of PbO, synthetic rhodostannite (Cu2FeSn3S8), and lithium triniobate (LiNbO3) (Supplementary Figure S3, supplementary material). These three compounds or similar compounds (such as Cu2FeSnS4) are associated with electronic devices production (Pugh et al., 2013; Baláž et al., 2017; Sumets et al., 2017). These are largely inappropriately disposed and end up in landfills or nature (Wong et al., 2007; Li and Achal, 2020; Patil and Ramakrishna, 2020). The fate of e-waste in Brazil is still not clearly defined, which can impair its collection and logistics (Echegaray and Valeria, 2017). Reuse or informal collection is the most likely handling of electronic waste, as only a few companies are specialized in recycling electronic devices in Brazil (Reis et al., 2012).
Shredded scraps of e-waste are commonly of made of heterogeneous and fibrous material, like the blue NIS found in this study. Most of the nonmetals are thermoset resins and reinforcing materials. Many e-waste particles are of varying rod-like and irregular shapes. They contain a substantial amount of nonmetallic Br and Si, along with metallic components such as Cu, Al, Sb, Fe, and Sn (Dragomir et al., 2021; Murali et al., 2022). The only other fibrous polymer is a red PET which was found in low levels mainly in the highlands or highlands-lowlands transition. It is commonly associated with wastewater from the washing of synthetic textiles (Browne et al., 2011).
Blue PP fragments were the second most frequent type of particle being in all sampling sites. PP is a hard sturdy material which is likely to produce fragment and is mainly present in food packaging, plastic containers, tubes and automotive components (Plastic Europe, 2020). It is also encountered in electronics such as in computers (Maddah, 2016). In a macroplastic study, PP has been associated to bottle cups, straws, and lolly sticks found on beaches (Frigione et al., 2021).
Polyvinyl Chloride (PVC) transparent fragments was the third most frequent type of particle and was distributed in the highlands and highlands-lowlands transition, but was markedly found in site 11 in the floodplain (Figure 2). PVC is present in construction materials, tubes and recreational materials (Plastic Europe, 2020). According to Abiplastic (2019), these were the plastics with the highest consumption in Brazil, where PP represented 21.6% and PVC, 13.6% of sold plastics. Hard transparent PVC is mainly found in the form of tubes and pipes that are used for construction. Soft fragments may come from PVC film which is commonly used to wrap food. The dumping sites along the Cuiabá River may be underpinning such PVC contamination.
PVA green fragments were also found in this study and are also characteristic of urban areas (Table, 1). PVA is used in the industrial, commercial, medicine and food sectors. It has been applied in the industrial, commercial, medical, and food sectors and has been used to produce many end products, such as lacquers, resins, surgical threads, and food packaging materials (Baker et al., 2012; Gaaz et al., 2015), the most likely source of the PVA microfragments in the study area.
Finally, Naplesyl fragments associated to paint particles were the less frequent type found (Table 1). They were present at sampling sites 3, 4, 8 and 9. These are particles that were probably derived from boats, just as in marine environments (Gaylarde et al., 2021), since there is a predominance of fishing trade along the Cuiabá River (Wantzen et al., 2008). In Jinhae Bay, South Korea, the predominance of more than 50% of paint particles is related to microplastics in the samples was reported (Song et al., 2015). Other studies report these paint particles, as in Hudson Bay and Canadian Arctic waters (Huntington et al., 2020) or in surface waters of the Chesapeake Bay (Bikker et al., 2020).
Conclusion
• This study reports the first estimation of anthropogenic microparticles in sediments in the Pantanal, which are found in relatively high concentrations in the Pantanal when compared to other water bodies worldwide;
• The proximity of human settlements can determine microplastic level, composition and size and the distance from the largest urban area (Cuiabá metropolitan region) is the main factor underpinning the size of microplastics;
• Microplastics pollution in sediments of Cuiabá River is dominated by secondary fibers and fragment. Also, over 40% of the microplastics were made of and undetermined material or combination of polymers/metals such as toxic Pb and Li and were associated with e-waste which can have negative drawbacks to the environment;
• Some of the microplastics found in the floodplain probably reached the Pantanal through the river flow, but local activities, such as construction or navigation, may also contribute to contamination of sediments by microplastics.
The concentrations of microplastics in sediments of the Pantanal raise a warning about the poor waste management and the potential environmental consequences as the increasing influx of microsynthetics from human activities may lead some organisms, vulnerable to this pollution type to disappear (Naidoo and Glassom, 2019; Barboza et al., 2020). Thus direct and indirect impacts on biodiversity and ecosystem functioning are expected as a result of their accumulation in sediments of aquatic environments (Huang et al., 2021).
Data availability statement
The original contributions presented in the study are included in the article/supplementary material, further inquiries can be directed to the corresponding author.
Author contributions
AL: writing, original draft, methodology, formal analysis, conceptualization, investigation. PG: writing—review and editing, conceptualization, investigation, supervision. CS: writing—review and editing, conceptualization, investigation, methodology. AC: writing—review and editing, conceptualization. EF: writing—review and editing, conceptualization. BR: writing—review and editing, conceptualization. DS: writing—review and editing, conceptualization. MB: writing —review and editing, conceptualization, methodology.
Acknowledgments
We aknowledge fellowships from the Brazilian Coordination for the Improvement of Higher Education Personnel (CAPES). We also wish to thank the Pantanal Research Centre (CPP) for help with logistics. We also acknowledge the Federal University of Mato Grosso (UFMT). We are thankful to Dr Adelina of the Morfology and Animal Histology Laboratory for sharing microscopes that were used to determined microplastics. We thank Nuria Casado-Coy for her assistance in the analysis of samples.
Conflict of interest
The authors declare that the research was conducted in the absence of any commercial or financial relationships that could be construed as a potential conflict of interest.
Publisher’s note
All claims expressed in this article are solely those of the authors and do not necessarily represent those of their affiliated organizations, or those of the publisher, the editors and the reviewers. Any product that may be evaluated in this article, or claim that may be made by its manufacturer, is not guaranteed or endorsed by the publisher.
Supplementary material
The Supplementary Material for this article can be found online at: https://www.frontiersin.org/articles/10.3389/fenvs.2022.1017480/full#supplementary-material
References
Alho, C. J. R., Mamede, S. B., Benites, M., Andrade, B. S., and Sepúlveda, J. J. O. (2019). Threats to the biodiversity of the Brazilian pantanal due to land use and occupation. Ambient. Soc. 22. doi:10.1590/1809-4422ASOC201701891VU2019L3AO
Allen, S., Allen, D., Phoenix, V. R., Roux, G. Le, Jiménez, P. D., Simonneau, A., et al. (2019). Atmospheric transport and deposition of microplastics in a remote mountain catchment. Nat. Geosci. 12, 339–344. doi:10.1038/s41561-019-0335-5
Andrady, A. L. (2011). Microplastics in the marine environment. Mar. Pollut. Bull. 62, 1596–1605. doi:10.1016/j.marpolbul.2011.05.030
Arthur, C., Baker, J., and Bamford, H. (2009). Proceedings of the International research Workshop on the Occurrence , effects , and fate of microplastic marine Debris. NOAA Technical Memorandum.
Aslam, H., Ali, T., Mortula, M. M., and Attaelmanan, A. G. (2020). Evaluation of microplastics in beach sediments along the coast of Dubai, UAE. Mar. Pollut. Bull. 150, 110739. doi:10.1016/j.marpolbul.2019.110739
Assine, M. L., Merino, E. R., Pupim, F. N., Warren, L. V., Guerreiro, R. L., and McGlue, M. M. (2015). Geology and Geomorphology of the pantanal basin. Handb. Environ. Chem. 37, 23–50. doi:10.1007/698_2015_349
Baker, M. I., Walsh, S. P., Schwartz, Z., and Boyan, B. D. (2012). A review of polyvinyl alcohol and its uses in cartilage and orthopedic applications. J. Biomed. Mat. Res. 9999B, 1451–1457. doi:10.1002/jbm.b.32694
Baláž, P., Baláž, M., Sayagués, M. J., Škorvánek, I., Zorkovská, A., Dutková, E., et al. (2017). Mechanochemical Solvent-Free synthesis of Quaternary Semiconductor Cu-Fe-Sn-S Nanocrystals. Nanoscale Res. Lett. 12, 256. doi:10.1186/s11671-017-2029-5
Barboza, L. G. A., Lopes, C., Oliveira, P., Bessa, F., Otero, V., Henriques, B., et al. (2020). Microplastics in wild fish from North East Atlantic Ocean and its potential for causing neurotoxic effects, lipid oxidative damage, and human health risks associated with ingestion exposure. Sci. Total Environ. 717, 134625. doi:10.1016/j.scitotenv.2019.134625
Bikker, J., Lawson, J., Wilson, S., and Rochman, C. M. (2020). Microplastics and other anthropogenic particles in the surface waters of the Chesapeake Bay. Mar. Pollut. Bull. 156, 111257. doi:10.1016/j.marpolbul.2020.111257
Blair, R. M., Waldron, S., Phoenix, V. R., and Gauchotte-Lindsay, C. (2019). Microscopy and elemental analysis characterisation of microplastics in sediment of a freshwater urban river in Scotland, UK. Environ. Sci. Pollut. Res. 26, 12491–12504. doi:10.1007/s11356-019-04678-1
Blettler, M. C. M., Abrial, E., Khan, F. R., Sivri, N., and Espinola, L. A. (2018). Freshwater plastic pollution: Recognizing research biases and identifying knowledge gaps. Water Res. 143, 416–424. doi:10.1016/j.watres.2018.06.015
Blettler, M. C. M., Garello, N., Ginon, L., Abrial, E., Espinola, L. A., and Wantzen, K. M. (2019). Massive plastic pollution in a mega-river of a developing country: Sediment deposition and ingestion by fish (Prochilodus lineatus). Environ. Pollut. 255, 113348. doi:10.1016/j.envpol.2019.113348
Blettler, M. C. M., Ulla, M. A., Rabuffetti, A. P., and Garello, N. (2017). Plastic pollution in freshwater ecosystems: Macro-meso-and microplastic debris in a floodplain lake. Environ. Monit. Assess. 189, 581. doi:10.1007/s10661-017-6305-8
Browne, M. A., Crump, P., Niven, S. J., Teuten, E., Tonkin, A., Galloway, T., et al. (2011). Accumulation of microplastic on Shorelines Woldwide : Sources and sinks. Environ. Sci. Technol. 45, 9175–9179. doi:10.1021/es201811s
Bullard, J. E., Ockelford, A., Brien, P. O., and Mckenna, C. (2021). Preferential transport of microplastics by wind. Atmos. Environ. X. 245, 118038. doi:10.1016/j.atmosenv.2020.118038
Chen, X., Xiong, X., Jiang, X., Shi, H., and Wu, C. (2019). Sinking of floating plastic debris caused by biofilm development in a freshwater lake. Chemosphere 222, 856–864. doi:10.1016/j.chemosphere.2019.02.015
Chiranda, R., Colpini, C., and Soares, T. S. (2016). Caracterização da Bacia Hidrográfica do Rio Cuiabá. Adv. For. Sci. 3, 13–20. doi:10.34062/afs.v3i1.2995
Cole, M., Lindeque, P., Halsband, C., and Galloway, T. S. (2011). Microplastics as contaminants in the marine environment : A review. Mar. Pollut. Bull. 62, 2588–2597. doi:10.1016/j.marpolbul.2011.09.025
Cole, M., Lindeque, P. K., Fileman, E., Clark, J., Lewis, C., Halsband, C., et al. (2016). Microplastics alter the properties and sinking Rates of Zooplankton Faecal Pellets. Environ. Sci. Technol. 50, 3239–3246. doi:10.1021/acs.est.5b05905
Crew, A., Gregory-Eaves, I., and Ricciardi, A. (2020). Distribution, abundance, and diversity of microplastics in the upper St. Lawrence River. Environ. Pollut. 260, 113994. doi:10.1016/j.envpol.2020.113994
De Falco, F., Gullo, M. P., Gentile, G., Di Pace, E., Cocca, M., Gelabert, L., et al. (2018). Evaluation of microplastic release caused by textile washing processes of synthetic fabrics. Environ. Pollut. 236, 916–925. doi:10.1016/j.envpol.2017.10.057
De Faria, É., Girard, P., Nardes, C. S., Moreschi, A., Christo, S. W., Ferreira Junior, A. L., et al. (2021). Microplastics pollution in the south American pantanal. Case Stud. Chem. Environ. Eng. 3, 100088. doi:10.1016/j.cscee.2021.100088
Dragomir, M. L., Cadar, R. D., and Boitor, R. M. (2021). Using E-waste in asphalt mixtures – A laboratory investigation. IOP Conf. Ser. Mat. Sci. Eng. 1138, 012022. doi:10.1088/1757-899x/1138/1/012022
Echegaray, F., and Valeria, F. (2017). Assessing the intention-behavior gap in electronic waste recycling : The case of Brazil. J. Clean. Prod. 142, 180–190. doi:10.1016/j.jclepro.2016.05.064
Emmerik, T. V., Tramoy, R., Calcar, C. V., Alligant, S., Treilles, R., Tassin, B., et al. (2019). Seine plastic debris transport Tenfolded during increased river discharge. Front. Mar. Sci. 6, 1–7. doi:10.3389/fmars.2019.00642
Fan, Y., Zheng, K., Zhu, Z., Chen, G., and Peng, X. (2019). Distribution, sedimentary record, and persistence of microplastics in the Pearl River catchment, China. Environ. Pollut. 251, 862–870. doi:10.1016/j.envpol.2019.05.056
Felismino, M. E. L., Helm, P. A., and Rochman, C. M. (2021). Microplastic and other anthropogenic microparticles in water and sediments of Lake Simcoe. J. Gt. Lakes. Res. 47, 180–189. doi:10.1016/j.jglr.2020.10.007
Fendall, L. S., and Sewell, M. A. (2009). Contributing to marine pollution by washing your face : Microplastics in facial cleansers. Mar. Pollut. Bull. 58, 1225–1228. doi:10.1016/j.marpolbul.2009.04.025
Fragão, J., Bessa, F., Otero, V., Barbosa, A., Sobral, P., Waluda, C. M., et al. (2021). Microplastics and other anthropogenic particles in Antarctica: Using penguins as biological samplers. Sci. Total Environ. 788, 147698. doi:10.1016/j.scitotenv.2021.147698
Frias, J., Pagter, E., Nash, R., and O’Connor, I. (2018). Standardised protocol for monitoring microplastics in sediments. JPI-Oceans Bas. Proj. 33. doi:10.13140/RG.2.2.36256.89601/1
Frigione, M., Marini, G., and Pinna, M. (2021). A thermal analysis-based approach to identify different waste macroplastics in beach litter: The case study of aquatina di frigole natura 2000 site (it9150003, Italy). Sustainability 13, 3186. doi:10.3390/su13063186
Gaaz, T. S., Sulong, A. B., Akhtar, M. N., Kadhum, A. A. H., Mohamad, A. B., Al-Amiery, A. A., et al. (2015). Properties and applications of polyvinyl alcohol, halloysite nanotubes and their nanocomposites. Molecules 20, 22833–22847. doi:10.3390/molecules201219884
Gaylarde, C. C., Neto, B., and Monteiro, E. (2021). Paint fragments as polluting microplastics : A brief review. Mar. Pollut. Bull. 162, 111847–847. doi:10.1016/j.marpolbul.2020.111847
Gerolin, C. R., Pupim, F. N., Sawakuchi, A. O., Grohmann, C. H., Labuto, G., and Semensatto, D. (2020). Microplastics in sediments from Amazon rivers, Brazil. Sci. Total Environ. 749, 141604. doi:10.1016/j.scitotenv.2020.141604
Geyer, R., Jambeck, J. R., and Law, K. L. (2017). Production , use , and fate of all plastics ever made. Sci. Adv. 3, 1700782. doi:10.1126/sciadv.1700782
Girard, P., Da Silva, C. J., and Abdo, M. (2003). River - Groundwater interactions in the Brazilian pantanal. The case of the Cuiabá River. J. Hydrol. X. 283, 57–66. doi:10.1016/S0022-1694(03)00235-X
Girard, P. (2011). “Hydrology of surface and groundwaters in the Pantanal floodplains,” in The Pantanal: Ecology, biodiversity and sustainable management of a large neotropical seasonal wetland (Pensoft), 103–126.
Hamilton, S. K. (2002). Hydrological controls of ecological structure and function in the pantanal wetland (Brazil). Ecohydrol. South Am. Rivers Wetl. (6), 133–158.
He, B., Goonetilleke, A., Ayoko, G. A., and Rintoul, L. (2020). Abundance, distribution patterns, and identification of microplastics in Brisbane River sediments, Australia. Sci. Total Environ. 700, 134467. doi:10.1016/j.scitotenv.2019.134467
Helcoski, R., Yonkos, L. T., Sanchez, A., and Baldwin, A. H. (2020). Wetland soil microplastics are negatively related to vegetation cover and stem density. Environ. Pollut. 256, 113391. doi:10.1016/j.envpol.2019.113391
Horton, A. A., and Dixon, S. J. (2018). Microplastics: An introduction to environmental transport processes. WIREs Water 5, 1–10. doi:10.1002/wat2.1268
Huang, Y., Li, W., Gao, J., Wang, F., Yang, W., Han, L., et al. (2021). Effect of microplastics on ecosystem functioning: Microbial nitrogen removal mediated by benthic invertebrates. Sci. Total Environ. 754, 142133. doi:10.1016/j.scitotenv.2020.142133
Huntington, A., Corcoran, P. L., Jantunen, L., Thaysen, C., Bernstein, S., Stern, G. A., et al. (2020). A first assessment of microplastics and other anthropogenic particles in Hudson Bay and the surrounding eastern Canadian Arctic waters of Nunavut. FACETS 5, 432–454. doi:10.1139/facets-2019-0042
Hurley, R., Woodward, J., and Rothwell, J. J. (2018). Microplastic contamination of river beds significantly reduced by catchment-wide flooding. Nat. Geosci. 11, 251–257. doi:10.1038/s41561-018-0080-1
IBGE (2000). Sinopse Preliminar do Censo Demográfico. Rio de Janeiro, RJ: Instituto Brasileiro de Geografia e Estatística - IBGE.
Imhof, H. K., Ivleva, N. P., Schmid, J., Niessner, R., and Laforsch, C. (2013). Contamination of beach sediments of a subalpine lake with microplastic particles. Curr. Biol. 23, R867–R868. doi:10.1016/j.cub.2013.09.001
Irion, G., Buchas, H., Junk, W., Nunes da Cinha, C., De Moraes, J. O., and Kasbohm, J. (2011). “Aspects of geological and sedimentological evolution of the Pantanal plain,” in The Pantanal: Ecology, biodiversity and sustainable management of a large neotropical seasonal wetland. Editors W. J. Junk, C. J. da Silva, C. Nunes da Cunha, and K. M. Wantzen (Pensoft), 870.
Junk, W. J., da Cunha, C. N., Wantzen, K. M., Petermann, P., Strüssmann, C., Marques, M. I., et al. (2006). Biodiversity and its conservation in the pantanal of Mato Grosso, Brazil. Aquat. Sci. 68, 278–309. doi:10.1007/s00027-006-0851-4
Junk, W. J., Piedade, M. T. F., Lourival, R., Wittmann, F., Kandus, P., Lacerda, L. D., et al. (2014). Brazilian wetlands: Their definition, delineation, and classification for research, sustainable management, and protection. Aquat. Conserv. 24, 5–22. doi:10.1002/aqc.2386
Klein, M., and Fischer, E. K. (2019). Microplastic abundance in atmospheric deposition within the Metropolitan area of Hamburg , Germany. Sci. Total Environ. 685, 96–103. doi:10.1016/j.scitotenv.2019.05.405
Klein, S., Worch, E., and Knepper, T. P. (2015). Occurrence and Spatial distribution of microplastics in river shore sediments of the Rhine-main area in Germany. Environ. Sci. Technol. 49, 6070–6076. doi:10.1021/acs.est.5b00492
Li, B., Wan, H., Cai, Y., Peng, J., Li, B., Jia, Q., et al. (2022). Human activities affect the multidecadal microplastic deposition records in a subtropical urban lake, China. Sci. Total Environ. 820, 153187. doi:10.1016/j.scitotenv.2022.153187
Li, W., and Achal, V. (2020). Environmental and health impacts due to e-waste disposal in China – a review. Sci. Total Environ. 737, 139745. doi:10.1016/j.scitotenv.2020.139745
Luo, W., Su, L., Craig, N. J., Du, F., Wu, C., and Shi, H. (2019). Comparison of microplastic pollution in different water bodies from urban creeks to coastal waters. Environ. Pollut. 246, 174–182. doi:10.1016/j.envpol.2018.11.081
Maddah, H. A. (2016). Polypropylene as a Promising plastic : A review. Acad. Accel. world’s Res. 6, 1–11. doi:10.5923/j.ajps.20160601.01
MapBiomas Project (2020). Collection of the Annual Series of Land Use and Land Cover Maps of Brazil. http://mapbiomas.org/ (Accessed April 20, 2021).
Masura, J., Baker, J., Foster, G., and Arthur, C. (2015). Laboratory methods for the analysis of microplastics in the marine environment: Recommendations for quantifying synthetic particles in waters and sediments. Siver Spring: MD, NOAA Mar. Debris Div, 31. doi:10.25607/OBP-604
Moreschi, A. C., Callil, C. T., Christo, S. W., Ferreira Junior, A. L., Nardes, C., de Faria, É., et al. (2020). Filtration, assimilation and elimination of microplastics by freshwater bivalves. Case Stud. Chem. Environ. Eng. 2, 100053. doi:10.1016/j.cscee.2020.100053
Munno, K., De Frond, H., O’Donnell, B., and Rochman, C. M. (2020). Increasing the Accessibility for characterizing microplastics: Introducing new application-based and spectral Libraries of plastic particles (SLoPP and SLoPP-E). Anal. Chem. 92, 2443–2451. doi:10.1021/acs.analchem.9b03626
Murali, A., Sarswat, P. K., Benedict, J., Plummer, M. J., Shine, A. E., and Free, M. L. (2022). Determination of metallic and polymeric contents in electronic waste materials and evaluation of their hydrometallurgical recovery potential. Int. J. Environ. Sci. Technol. 19, 2295–2308. doi:10.1007/s13762-021-03285-3
Naidoo, T., and Glassom, D. (2019). Decreased growth and survival in small juvenile fish, after chronic exposure to environmentally relevant concentrations of microplastic. Mar. Pollut. Bull. 145, 254–259. doi:10.1016/j.marpolbul.2019.02.037
Naidu, R., Andres, V., Espana, A., Liu, Y., and Jit, J. (2016). Emerging contaminants in the environment: Risk-based analysis for better management. Chemosphere 154, 350–357. doi:10.1016/j.chemosphere.2016.03.068
Nizzetto, L., Bussi, G., Futter, M. N., Butterfield, D., and Whitehead, P. G. (2016). A theoretical assessment of microplastic transport in river catchments and their retention by soils and river sediments. Environ. Sci. Process. Impacts 18, 1050–1059. doi:10.1039/C6EM00206D
Nunes da Cunha, C., Junk, W. J., and Leitão-Filho, H. F. (2007). Woody vegetation in the pantanal of Mato Grosso, Brazil: A preliminary typology. Amazonia 3/4, 159–184.
Park, T.-J., Lee, S.-H., Lee, M.-S., Lee, J.-K., Park, J.-H., and Zoh, K.-D. (2020). Distributions of microplastics in surface water, fish, and sediment in the vicinity of a sewage treatment plant. Water 12, 3333. doi:10.3390/w12123333
Patil, R. A., and Ramakrishna, S. (2020). A comprehensive analysis of e-waste legislation worldwide. Environ. Sci. Pollut. Res. 27, 14412–14431. doi:10.1007/s11356-020-07992-1
Pazos, R. S., Amalvy, J., Cochero, J., Pecile, A., and Gómez, N. (2021). Temporal patterns in the abundance, type and composition of microplastics on the coast of the Río de la Plata estuary. Mar. Pollut. Bull. 168, 112382–382. doi:10.1016/j.marpolbul.2021.112382
Peng, G., Zhu, B., Yang, D., Su, L., Shi, H., and Li, D. (2017). Microplastics in sediments of the Changjiang estuary, China. Environ. Pollut. 225, 283–290. doi:10.1016/j.envpol.2016.12.064
Plastics Europe (2020). Plastics – The facts 2020 - an analysis of European plastics production, demand and waste data. Plastics Europe.
Prata, J. C., Reis, V., Matos, J. T. V., da Costa, J. P., Duarte, A. C., and Rocha-Santos, T. (2019). A new approach for routine quantification of microplastics using Nile Red and automated software (MP-VAT). Sci. Total Environ. 690, 1277–1283. doi:10.1016/j.scitotenv.2019.07.060
Pugh, D., Bloor, L., Moniz, S., and Carmalt, C. J. (2013). “Precursor Chemistry – main group metal Oxides,” in Comprehensive Inorganic Chemistry II (Elsevier), 1021–1052. doi:10.1016/B978-0-08-097774-4.00134-0
Pupim, N. (2014). Geomorfologia e paleo-hidrologia dos megaleques dos rios Cuiabá e São Lourenço, quaternário da Bacia do Pantanal. Available at: http://hdl.handle.net/11449/123676.
Qian, J., Tang, S., Wang, P., Lu, B., Li, K., Jin, W., et al. (2021). From source to sink: Review and prospects of microplastics in wetland ecosystems. Sci. Total Environ. 758, 143633. doi:10.1016/j.scitotenv.2020.143633
Reis, C., Oliveira, D., Moura, A., and Engel, A. (2012). Collection and recycling of electronic scrap : A worldwide overview and comparison with the Brazilian situation. Waste Manag. 32, 1592–1610. doi:10.1016/j.wasman.2012.04.003
Scheurer, M., and Bigalke, M. (2018). Microplastics in Swiss floodplain soils. Environ. Sci. Technol. 52, 3591–3598. doi:10.1021/acs.est.7b06003
Schmidt, C., Krauth, T., and Wagner, S. (2017). Export of plastic debris by rivers into the Sea. Environ. Sci. Technol. 51, 12246–12253. doi:10.1021/acs.est.7b02368
Shruti, V. C., Jonathan, M. P., Rodriguez-Espinosa, P. F., and Rodríguez-González, F. (2019). Microplastics in freshwater sediments of Atoyac river basin, Puebla city, Mexico. Sci. Total Environ. 654, 154–163. doi:10.1016/j.scitotenv.2018.11.054
Soltani, N., Keshavarzi, B., Moore, F., Busquets, R., Nematollahi, M. J., Javid, R., et al. (2022). Effect of land use on microplastic pollution in a major boundary waterway: The Arvand River. Sci. Total Environ. 830, 154728. doi:10.1016/j.scitotenv.2022.154728
Song, Y. K., Hong, S. H., Jang, M., Han, G. M., and Shim, W. J. (2015). Occurrence and distribution of microplastics in the Sea surface Microlayer in Jinhae Bay , South Korea. Arch. Environ. Contam. Toxicol. 69, 279–287. doi:10.1007/s00244-015-0209-9
Sruthy, S., and Ramasamy, E. V. (2017). Microplastic pollution in Vembanad Lake, Kerala, India: The first report of microplastics in lake and estuarine sediments in India. Environ. Pollut. 222, 315–322. doi:10.1016/j.envpol.2016.12.038
Sumets, M. P., Dybov, V. A., and Ievlev, V. M. (2017). LiNbO3 films: Potential application, synthesis techniques, structure, properties. Inorg. Mat. 53, 1361–1377. doi:10.1134/S0020168517130015
Tibbetts, J., Krause, S., Lynch, I., and Sambrook Smith, G. (2018). Abundance, distribution, and drivers of microplastic contamination in urban river environments. Water 10, 1597. doi:10.3390/w10111597
Townsend, K. R., Lu, H.-C., Sharley, D. J., and Pettigrove, V. (2019). Associations between microplastic pollution and land use in urban wetland sediments. Environ. Sci. Pollut. Res. 26, 22551–22561. doi:10.1007/s11356-019-04885-w
Ussami, N., Shiraiwa, S., and Dominguez, J. M. L. (1999). Basement reactivation in a sub-Andean foreland flexural bulge: The Pantanal wetland, SW Brazil. Tectonics 18, 25–39. doi:10.1029/1998TC900004
Wang, W., Ndungu, A. W., Li, Z., and Wang, J. (2017). Microplastics pollution in inland freshwaters of China: A case study in urban surface waters of Wuhan, China. Sci. Total Environ. 575, 1369–1374. doi:10.1016/j.scitotenv.2016.09.213
Wantzen, K. M., Nunes, C., Junk, W. J., Girard, P., Rossetto, O. C., Penha, J. M., et al. (2008). Towards a sustainable management concept for ecosystem services of the Pantanal wetland. Ecohydrol. Hydrobiology 8, 115–138. doi:10.2478/v10104-009-0009-9
Wayman, C., and Niemann, H. (2021). The fate of plastic in the ocean environment-a minireview. Environ. Sci. Process. Impacts 23, 198–212. doi:10.1039/d0em00446d
Wong, C. S. C., Wu, S. C., Duzgoren-aydin, N. S., Aydin, A., and Wong, M. H. (2007). Trace metal contamination of sediments in an e-waste processing village in China. Environ. Pollut. 145, 434–442. doi:10.1016/j.envpol.2006.05.017
Xia, F., Yao, Q., Zhang, J., and Wang, D. (2021). Effects of seasonal variation and resuspension on microplastics in river sediments. Environ. Pollut. 286, 117403. doi:10.1016/j.envpol.2021.117403
Yonkos, L. T., Friedel, E. A., Perez-Reyes, A. C., Ghosal, S., and Arthur, C. D. (2014). Microplastics in Four Estuarine Rivers in the Chesapeake Bay, U.S.A. Environ. Sci. Technol. 48, 14195–14202. doi:10.1021/es5036317
Keywords: microplastics, anthropogenic particles, freshwater, land use, emerging contaminants, floodplain
Citation: Camargo ALG, Girard P, Sanz-Lazaro C, Silva ACM, de Faria É, Figueiredo BRS, Caixeta DS and Blettler MCM (2022) Microplastics in sediments of the Pantanal Wetlands, Brazil. Front. Environ. Sci. 10:1017480. doi: 10.3389/fenvs.2022.1017480
Received: 12 August 2022; Accepted: 20 September 2022;
Published: 12 October 2022.
Edited by:
Peiyue Li, Chang’an University, ChinaCopyright © 2022 Camargo, Girard, Sanz-Lazaro, Silva, de Faria, Figueiredo, Caixeta and Blettler. This is an open-access article distributed under the terms of the Creative Commons Attribution License (CC BY). The use, distribution or reproduction in other forums is permitted, provided the original author(s) and the copyright owner(s) are credited and that the original publication in this journal is cited, in accordance with accepted academic practice. No use, distribution or reproduction is permitted which does not comply with these terms.
*Correspondence: Alan Lacerda Gomes Camargo, YWxhbi5sYWNlcmRhLmJsZWZvckBnbWFpbC5jb20=