- 1College of Desert Control Science and Engineering, Inner Mongolia Agricultural University, Hohhot, Inner Mongolia, China
- 2College of Forestry, Inner Mongolia Agricultural University, Hohhot, Inner Mongolia, China
Salix psammophila sand barriers are a widely used engineering measure to control quicksand in northwest China. Thus, it is important to elucidate the influence of the sand barrier decay process on soil microbial ecological stoichiometric characteristics in desert environments. In the present study, field in situ sampling and laboratory index measurements were used to evaluate and compare the performance degradation, variation in soil physical and chemical properties, and soil microbial ecological stoichiometry of sand barriers during decay. The results showed that with the worsening of the decay degree, all indexes of the decay characteristics decreased significantly, among which the flexural strength of mechanical properties decreased the most, which directly led to collapse and damage. The cellulose and lignin contents of the chemical components also exhibited varying degrees of decomposition, and the soil physical and chemical properties showed a significant increase. The changes in the microbial biomass carbon (MBC) and microbial biomass nitrogen (MBN) contents were consistent with the trend of the soil properties, and both reached their peak at 7 years. With the aggravation of decay, the stoichiometric ratios of soil microbial elements C, N, and P increased continuously. However, there was no significant increase in MBC/MBP and MBN/MBP in the early period (≤3 years) of the sand barrier establishment, but there was a significant increase in the later period (≥5 years). These results indicated that S. psammophila sand barriers mainly played the role of windbreak and sand fixation in the early period, and made soil microorganisms susceptible to phosphorus limitation in the later period. Stepwise linear regression analysis showed that MBC/MBN, MBC/MBP, and MBN/MBP were mainly affected by basic density (BD). Therefore, the sand barrier changes soil properties by degrading its own chemical components during the decay process and the loss of basic density is the main driving factor for increasing the C:N:P stoichiometry of soil microbial biomass. It can still be further promoted and used in the resource utilization process of mechanical sand barriers in the future.
1 Introduction
Desertification is a serious environmental issue that has received considerable attention across the world (Mahata and Sharma, 2021). Over the decades, in the field of desertification control, aeolian researchers have implemented various special control techniques applicable to wind and sand disaster control and have proposed ecological and engineering interventions, such as artificial vegetation and mechanical sand barriers, which provide valuable experience for the widespread application of desertification control models (You et al., 2021). Salix psammophila sand barrier is a semi-concealed mechanical sand barrier formed by artificially cutting live Salix stems into 45–50 cm long branches and inserting them vertically into the desert soil (Gao et al., 2013). Using plant stems as natural biological materials, the construction technology of S. psammophila sand barriers is simple and can be used on a large scale (Gao et al., 2013). Previous studies have shown that they can effectively contribute to the recovery of desert vegetation and change the direction and size of wind and sand flow movement (Ren et al., 2007; Liu and Bo, 2020). However, exposed to the field environment for a long time, S. psammophila sand barriers degrade naturally through biodegradation, weathering, desorption-alternation, and other biological and abiotic factors (Wang et al., 2021a). An important driving force of material decomposition and nutrient cycling is the C:N:P element stoichiometry and this process is dominated by microorganisms (Zechmeister-Boltenstern et al., 2015). Soil microbial C:N:P stoichiometry provides critical information for understanding the nutrient limitations of organisms and biological communities (Manzoni et al., 2010). Thus, how the decay process of S. psammophila sand barrier affects desert soil microbial biomass and its stoichiometry is important for understanding the recycling of nutrient elements in desert ecosystems.
Soil microorganisms play an important role in the decomposition of organic matter, utilization of plant nutrients, and driving and regulating of nutrient cycling and transformation in an ecosystem (Douterelo et al., 2010; Guo et al., 2016). They not only drive subsurface biogeochemical processes in terrestrial ecosystems (Falkowski et al., 2008) but also affect aboveground primary productivity and regulate the response of multiple ecosystem functions (Jing et al., 2015). Soil microbial activity and biomass dynamics contribute to the regulation of net soil fluxes and the amount of soil carbon and nutrients (Bauhus and Barthel, 1995; Franzluebbers et al., 1995). Although soil microbial biomass accounts for only a small fraction of soil organic matter, it contributes significantly to the cycling of C, N, and P elements in the ecosystem (Van Der Heijden et al., 2008). The ratio of C, N, and P elemental content of soil microbial biomass was used to reflect the stoichiometry of microbial biomass. Cleveland and Liptzin (2007) first used this stoichiometry to represent the response of microbial biomass C, N, and P to the soil environment, while also confirming that the microbial C:N:P ratio is well-constrained. However, microbial C:N, C:P, and N:P are influenced by biological factors, such as plant productivity and microbial community composition (Fanin et al., 2013; Chen et al., 2016). Previous studies have shown that N addition has a consistent effect on soil microbial community composition, altering the relative abundance of mycorrhizal fungi and oligotrophic bacteria (Leff et al., 2015; Sinsabaugh et al., 2015). The decay and decomposition of a S. psammophila sand barrier increased soil N availability (Gao et al., 2013), which likely caused changes in soil microbial biomass. This is because the microorganisms that decompose organic matter can store the absorbed nutrients as glycogen or polyphosphates to promote their own reproduction and growth (Kornberg 1995; Wilson et al., 2010), thereby increasing microbial biomass.
In addition, the ratio of soil microbial C:N:P varies in different environments and communities. Previous studies have shown that there are broad-scale patterns in microbial N, P, and the N:P ratio along latitude, temperature, and precipitation gradients (Li et al., 2014). However, there are relatively few studies on the mechanism of the soil microbial response to the environment in arid environments. A study of microbial stoichiometry patterns in the topsoil and subsoil along an aridity gradient showed that the soil organic carbon content and fungal to bacterial ratios had the greatest effect on the microbial C: N, C:P, and N:P ratios, while microorganisms in the topsoil were more conservative with respect to N than those in deeper soils (Liu et al., 2020). These studies confirmed that changes in substrate nutrient supply in the soil affect the microbial structure and thus control the microbial stoichiometry. The decay of S. psammophila sand barriers can release C, P, and K into the soil in a fluctuating state (Wang et al., 2021a), which directly affects the resource and nutrient conditions in the soil and changes the availability of the substrate supply. Previous studies have confirmed that the decay process of S. psammophila sand barriers affects soil microbial biomass and enzyme activities (Liang et al., 2022). However, it is not clear how microbial biomass stoichiometry correlates with decay characteristics and soil matrix properties. Therefore, there is an urgent need to clarify the effect of the decay process of S. psammophila on soil microbial biomass stoichiometry and the main driving factors, which are important for understanding the nutrient cycling processes in desert ecosystems.
In this study, we investigated the effects of the S. psammophila sand barrier decay process on soil microbial ecological stoichiometry and the main driving factors in the desert region of northwest China. In situ samples were taken from the sand barriers and soils set up in the field for a long period of time and in consecutive years. We analyzed in detail the changes in the chemical composition, physical and mechanical indexes of the sand barriers, soil physicochemical indexes, and microbial biomass C, N, and P contents. The study revealed the mechanisms whereby the chemical composition and soil properties affect microbial biomass and stoichiometry during the decay process. Three specific hypotheses were that 1) the long-term decay and decomposition of S. psammophila sand barrier are able to increase the soil microbial C, N, and P content, and there may be a peak turning point during the decomposition period; 2) The soil microbial C: N: P stoichiometric ratios will also increase with the aggravation of decay; and 3) it may be driven by indicators of the decaying nature of the sand barrier. If these results can be verified, it will provide a new theoretical basis and data support for improving the resource utilization efficiency and ecological environment of S. psammophila sand barriers in northwest China.
2 Materials and methodology
2.1 Study area
This study was carried out in the Kubuqi Desert (108°41′21″E, 40°29′34″N; 1,016 m) of Hangjin County, Ordos, Inner Mongolia, northwest China. The area falls within a temperate continental climate, controlled by westerly circulation in the upper air and influenced by monsoon circulation near the ground. It is hot and rainy in summer and cold and dry in winter, with plenty of sunshine and a large temperature difference between day and night, with an annual temperature of 6.3°C, an average wind speed of 4.4 m/s, and a frost-free period of 135 days. The average annual precipitation is 258.3 mm, mainly concentrated from July to August. The geomorphic types in the area are mobile dunes, semi-mobile dunes, and fixed dunes. The dominant natural plant species are Salix psammophila, Caragana korshinskii, Agriophyllum squarrosum, and Corispermum hyssopifolium.
2.2 Experimental design and field sampling
In the present study, all of the test plots are located next to the same road in the Kubuqi desert. These large areas of S. psammophila sand barriers are an effective measure by the local forestry department to protect the road from wind and sand hazards, and work is carried out regularly every year (Figure 1A). In our experiment, five study sites were selected to represent different types of decomposition treatments, which had been set up for 1, 3, 5, 7, and 9 years. Precipitation data is obtained from the National Earth System Science Data Center, National Science and Technology Infrastructure of China (http://www.geodata.cn) (Figure 1B). The years in which the S. psammophila sand barriers were established were divided accurately based on consultation with project implementation workers and field surveys. Sand barrier samples of different ages were located within a range of 1.2 km, and samples were collected in the same environment and topographic conditions. We conducted a basic survey of basic soil data and sampling location information (Table 1). After determination of the general situation of the sand barrier setting at the experimental site, test samples were collected and measurements were carried out using the “space instead of time–in situ sampling” method.
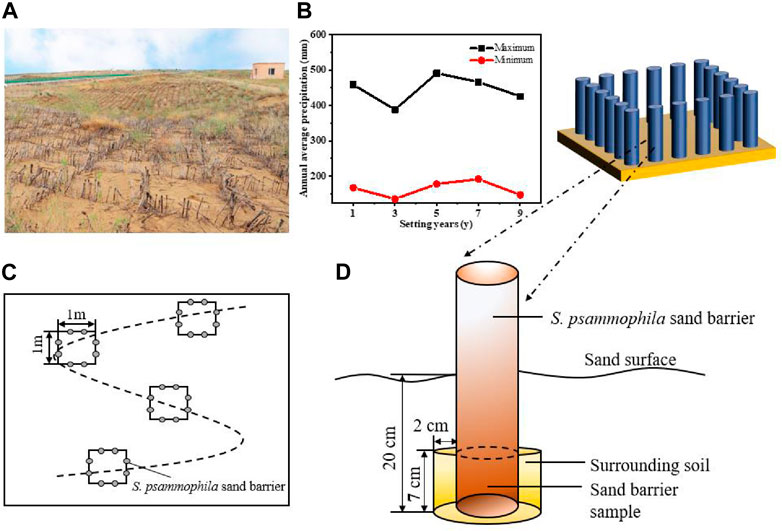
FIGURE 1. Schematic diagram of Salix psammophila sand barriers plot and sample collection. (A) Photograph of the study site; (B) Precipitation; (C) Sampling diagram; (D) Sample collection.
The test samples were derived from two parts: decaying wood from the subsurface portion of the sand barrier and the soil immediately adjacent to it. Sand barrier and soil sampling were conducted simultaneously at the five sites from May 21 to 22 in 2021. The sample sites and sampling schematic are shown in Figure 1. Before the test sample collection, there had been no precipitation event for eight consecutive days. All study sites were semi-concealed mechanical sand barriers with a 1 × 1 size grid. We randomly selected four 1 × 1 m2 grids on an “S”-shaped line at each study site, with any two grids separated by at least 5 m (Figure 1C). Two sand barrier wooden branches were selected at equal intervals on each side of the grid (east, west, south, and north), and the sample diameter was 2.0 ± 0.02 cm and the sand-buried depth was 20 ± 0.5 cm. The 7 cm branch at the bottom of the sand barrier was used as the test material (Figure 1D). A total of 160 sand barrier wood samples were used to determine basic decay characteristics, including the cellulose (Cel, %) and lignin (Lig, %) contents, basic density (BD, g·cm−3), and flexural strength (FS, MPa).
In addition, the adjacent soil corresponding to the sand barrier test material (within 2 cm) was simultaneously collected using CORNING CentriStar ™ sterile centrifuge tubes. The eight samples from the same barrier grid were homogeneously mixed into one sample, and four replicates per group were sampled. The soil was stored at 4°C and transported to the laboratory. All soil samples were evenly divided into two parts, one was used for the measurement of soil microbial biomass, and the other was air-dried naturally and passed through a 2 mm sieve to remove impurities such as residual roots and gravel, and then used for the measurement of soil organic carbon (SOC; g·kg−1), dissolved organic carbon (DOC; mg·kg−1), total nitrogen (TN; g·kg−1), available nitrogen (AN; mg·kg−1), the moisture content (MC; %), and pH.
2.3 Sand barrier and soil measurements
Each sand barrier sample was numbered and then assessed for chemical composition and physical and mechanical indexes. The samples were dried in an oven at 80°C to a constant weight and then ground into a powder and passed through a 40–60-mesh sieve. The cellulose content was determined by the nitric acid-ethanol method (Fan et al., 2013), and lignin was examined for Klason lignin using the usual technique (Sluiter et al., 2010). The BD and FS were measured according to the National Forestry Industry Standard (LY/T 2369, 2014).
Each soil sample was labeled and then analyzed for SOC by the dichromate oxidation method (Nelson and Sommers, 1983). DOC was extracted with 60 ml of 0.5 M K2SO4 after shaking for 45 min at 4,000 rpm and then measured by the wet-oxidation method (Deng et al., 2019). Soil pH was estimated on a soil: water suspension of 1:2.5 using a glass electrode meter (PHS-3C, Lexi, Shanghai, China). The MC was determined gravimetrically after oven drying the fresh soil samples to a constant weight of 105°C. The AN was determined by the continuous alkali-hydrolyzed reduction diffusing method (Stanford, 1982). The TN content was determined by the Kjeldahl method (Bremner, 1996).
2.4 Soil microbial biomass measurement
MBC and MBN were analyzed by chloroform fumigation and extraction using 0.5 mol L−1 K2SO4 (Brookes et al., 1985; Vance et al., 1987). Briefly, for each soil sample, chloroform fumigation was performed for 24 h at 25°C using pre-cultured soil (10 g, fresh weight), and the same weight of non-chloroform fumigated soil was used as a control. Then, MBC and MBN were extracted using 100 ml of 0.5 M K2SO4, and MBP was extracted using 0.5 M NaHCO3. Non-wetted soil was also extracted with 100 ml of 0.5 M NaHCO3. The calculation method was to divide the difference in the organic carbon, nitrogen, and phosphorus contents between fumigated and unfumigated soil samples by the conversion coefficient, and the values of KC, KN, and KP were 0.45, 0.54, and 0.40, respectively (Joergensen and Mueller 1996).
2.5 Statistical analysis
The decay characteristics, soil physicochemical properties, soil microbial biomass, and stoichiometry were first tested by the Shapiro–Wilk method for normal distribution, and then the non-normal distribution variables were logarithmically transformed. Then, one-way ANOVA with Duncan’s post hoc test was used to assess significant differences among decay characteristics, soil physicochemical properties, and soil microbial biomass and stoichiometric ratios under the different setting years (significance level was 0.05). Pearson correlation analyses were conducted to determine the relationships among the decay characteristics, soil physicochemical properties, soil microbial biomass, and stoichiometry. A stepwise regression model using SPSS 26.0 software (SPSS Inc., Chicago, Illinois, United States) was used to detect key factors affecting the decay characteristics of sand barriers and soil properties of soil microbial C:N:P stoichiometric ratios. The estimates in the regression coefficients were set to 95% confidence intervals for testing the significance of the regression coefficients. In addition, all of the statistical analyses were performed using SPSS 26.0 software. GraphPad Prism 8.0 (GraphPad Inc., San Diego, California, United States) and Origin 2021 software (OriginLab Inc., Northampton, Massachusetts, United States ) were used to draw graphs.
3 Results
3.1 Decay characteristics and changes in soil physicochemical properties
3.1.1 Decay characteristics
With the increase in setting time, the decay indicators of the stable sand-buried part of the S. psammophila sand barrier changed regularly (Figure 2). Cel and BD decreased significantly with time (Figures 2A,D; p < 0.05), but the Lig content did not change significantly after 3 years (Figure 2B; p > 0.05). As shown in Figure 2C, the FS showed a declining trend, and the decrease was rapid at first and then slowed, with the range of change of the mean value decreasing from 38.21 to 9.05 MPa. FS decreased the most in the first 5 years, by 58.70%, while after 5 years, there was no significant difference between groups (p > 0.05). Compared with 1 year, the average values of Cel, Lig, FS, and BD decreased by 50.79%, 44.56%, 76.32%, and 69.39%, respectively, in 9 years. Therefore, with increasing decomposition, the decay characteristics of the S. psammophila sand barriers decreased in the following order: FS > BD > Cel > Lig.
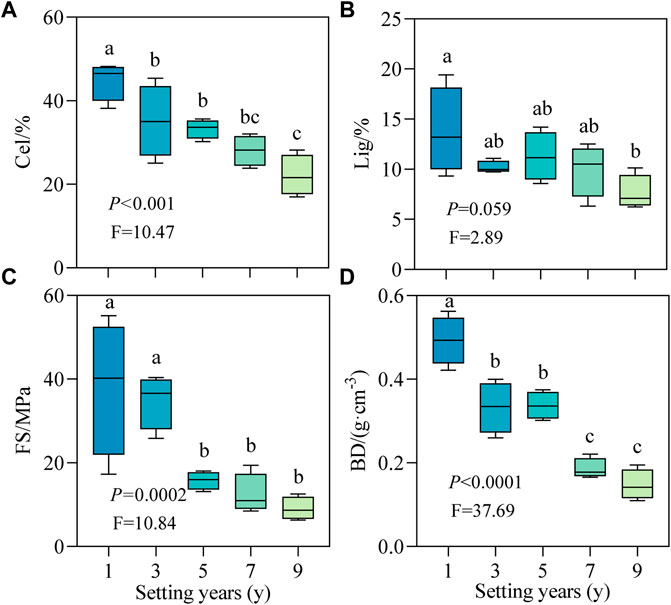
FIGURE 2. Decay characteristics of S. psammophila sand barriers under different setting years. (A) Cel, Cellulose; (B) Lig, Lignin; (C) FS, Flexural strength; (D) BD, Basic density. Different lowercase letters in the figure indicate significant differences between the sand barriers a different setting years (p < 0.05).
3.1.2 Soil physicochemical properties
To elucidate the effect of S. psammophila sand barriers on soil properties during decay, the physical and chemical indicators of soil were quantitatively analyzed. As shown in Figure 3, with the increase in setting time, the soil physical and chemical properties changed regularly due to the decomposition of the sand barrier. The changes in SOC, DOC, TN, AN, MC, and pH had the same pattern, which increased significantly with time (p < 0.05) and reached the peak value at 7 years. Compared with 1 year, the average value of each index at 7 years increased by 93.64%, 95.42%, 66.11%, 82.99%, 113.63%, and 7.13%, respectively. Therefore, the soil moisture content increased the most during the decay process of the S. psammophila sand barriers.
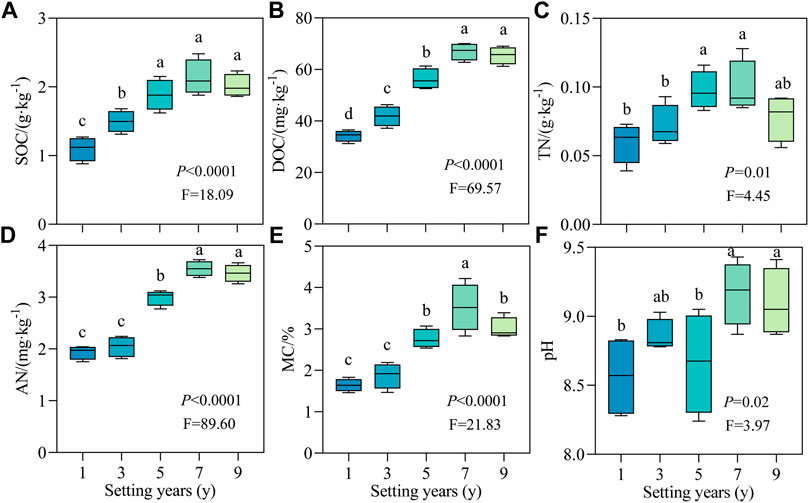
FIGURE 3. Soil physicochemical properties of S. psammophila sand barriers under different setting years. (A) SOC, Soil organic carbon; (B) DOC, Dissolved organic carbon; (C) TN, Total nitrogen; (D) AN, available nitrogen; (E) MC, Moisture content; (F) pH, pH value. Different lowercase letters in the figure indicate significant differences between the sand barriers a different setting years (p < 0.05).
3.2 Soil microbial biomass carbon, nitrogen, and phosphorus and their stoichiometric ratios
As shown in Figures 4A–C, the MBC, MBN, and MBP contents all showed an increasing trend followed by a decreasing trend with time. MBC and MBN reached the maximum value at 7 years, i.e., 60.57 and 9.28 mg/kg, respectively, while the MBP content was maximum at 5 years (8.73 mg/kg). Compared with 1 year, the MBP content at 5 years increased significantly by 53.16%. After setting for 1–9 years, MBC/MBN ratio showed an overall upward trend with a range of variation from 5.42 to 7.71 (Figure 4D). As shown in Figures 4D–F, the ratios of MBC/MBN, MBC/MBP and MBN/MBP did not change significantly in the early stage (≤3 years; p > 0.05), but increased significantly in the later stage (≥5 years; p < 0.05), and the ratios at 9 years were 1.42, 3.51, and 2.47 times those at 1 year.
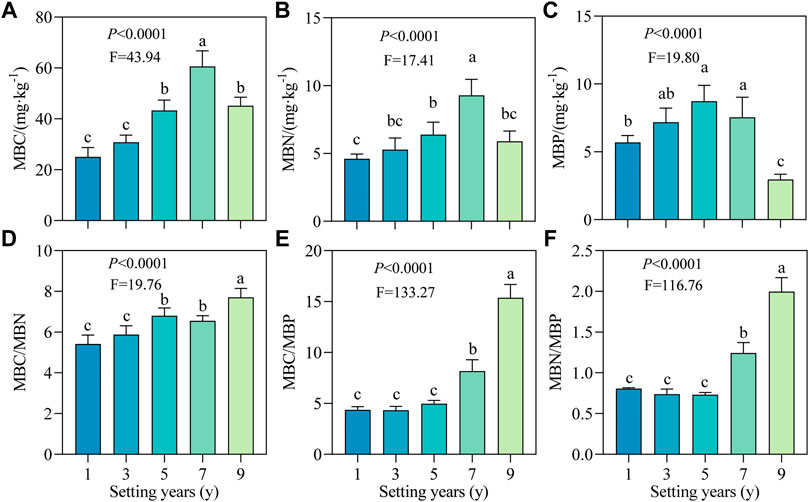
FIGURE 4. Soil microbial biomass carbon, nitrogen and phosphorus and their stoichiometric ratios of S. psammophila sand barriers under different setting years. (A) MBC, Microbial biomass carbon; (B) MBN, Microbial biomass nitrogen; (C) MBP, Microbial biomass phosphorus; (D) MBC/MBN, Soil microbial biomass C/N ratio; (E) MBC/MBP, Soil microbial biomass C/P ratio; (F) MBN/MBP, Soil microbial biomass N/P ratio. Different lowercase letters in the figure indicate significant differences between the sand barriers a different setting years (p < 0.05).
3.3 Relationship among soil microbial carbon, nitrogen, phosphorus, and soil properties and decay characteristics
As can be seen from Figure 5, there was a highly significant positive correlation between MBC and MBN (p < 0.01) and significant positive correlations with AN, pH, MC, DOC, SOC, and TN, (p < 0.05), but MBP had no significant correlation with MBC, MBN, AN, pH, MC, DOC, and SOC (p > 0.05). In addition, MBC/MBN, MBC/MBP, and MBN/MBP were significantly positively correlated with AN, MC, DOC, and SOC (p < 0.05), but significantly negatively correlated with Cel, Lig, FS, and BD (p < 0.05). The MBC/MBN, MBC/MBP, and MBN/MBP ratios were not significantly correlated with TN (p > 0.05).
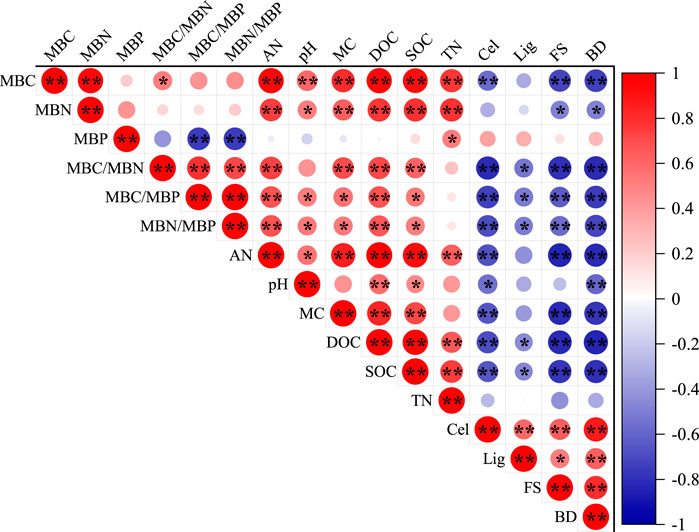
FIGURE 5. Pearson correlation analysis of the soil microbial carbon, nitrogen, phosphorus and soil properties and decay characteristics. Notes: Indicator values on the right represent the correlation coefficients. The red color represents a positive correlation, and the blue color represents a negative correlation at significance levels of *p < 0.05; **p < 0.01.
3.4 Multiple stepwise regression analysis
Stepwise regression models were constructed using soil microbial biomass and its stoichiometry with decay properties and soil physical and chemical indicators. As can be seen from Table 2, the model constructed with MBC, MBN, and MBC/MBN had the best effect, with R2 values of 0.885, 0.681, and 0.654, respectively. The results showed that the stepwise regression models of MBC/MBN, MBC/MBP, MBN/MBP, MBC, MBN, and MBP with decay characteristics and soil physical and chemical properties all reached an extremely significant level (p < 0.01). Among them, MBC/MBN, MBC/MBP, and MBN/MBP were mainly influenced by BD. MBC was mainly influenced by DOC and TN, and MBN was mainly influenced by TN and SOC, while MBP was influenced by TN and Cel.
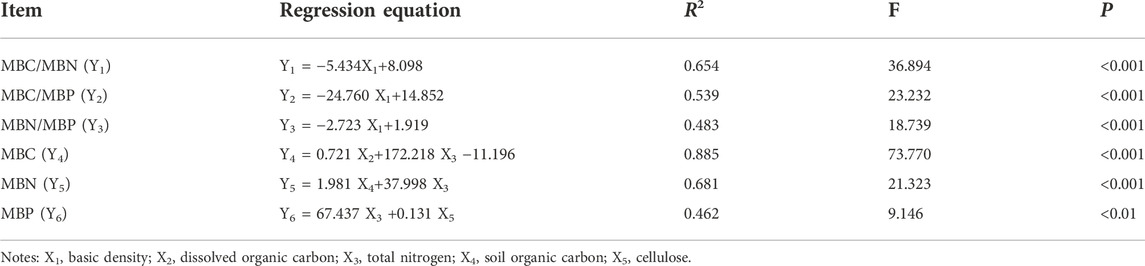
TABLE 2. Multiple stepwise regression analysis of soil microbial carbon, nitrogen and phosphorus affected by decay characteristics and soil properties.
4 Discussion
4.1 Effects of decomposition processes on decay characteristics and soil properties of S. psammophila sand barriers
The long-lasting sand-buried part of the S. psammophila sand barrier is subjected to microbial decomposition and irregular moisture absorption and desorption due to long-term exposure to the desert subsoil, resulting in the expansion and contraction of the internal microstructure of the barrier body and the loss of chemical components (Wang et al., 2021b). The results of this study showed that the contents of cellulose and lignin were significantly reduced. Compared with 1 year, the contents of cellulose and lignin decreased by 50.79% and 44.56%, respectively, in 9 years (Figures 2A,B). This is consistent with previous findings, confirming that cellulose was reduced more than lignin (Hammel, 1997). This is mainly because the structure of phenylpropane in lignin is connected by ether bonds (C-O-C) and carbon-carbon bonds (C-C), and its chemical reactions are more complex than those of degraded polysaccharides in cellulose (Zimmermann 1990; Bugg et al., 2011). The basic density of the sand barrier decreased significantly with the increase in decay, which was directly related to the flexural strength of the mechanical properties. This was caused by the breakdown of the vessels, wood fibers, and parenchyma cells caused by decay, the collapse of the cellular structure, and the contraction of the cell wall (Wang et al., 2021b). As a result, S. psammophila sand barriers are more prone to collapse and breakage when subjected to strong wind and sand flow erosion. In addition, with the increase in the number of decay years, soil SOC, DOC, TN, and AN showed a significant increasing trend, which was mainly related to the release of nutrients into the soil after the barrier body decomposed its own organic material, and its nutrient elements were released into the soil in a fluctuating state (Wang et al., 2021a). However, the soil water content increased more than the other indexes, which may be because the water absorption capacity of the sand barrier body increased sharply with the decrease of its basic density, and the higher water storage capacity maintained the surrounding soil water content.
4.2 Effects of S. psammophila sand barrier decay on soil microbial biomass
The decay process of the S. psammophila sand barriers significantly changed the basic physicochemical properties of the soil, making it easier to change the soil microbial biomass C, N, and P contents (Figure 4). The results of this study revealed that soil MBC, MBN, and MBP showed a trend of ascending and then descending with increasing decay time, which is consistent with the results of a previous study (Liang et al., 2022). On the one hand, the sand barrier may increase the content of soil DOC in the early stage of decay (Figure 3B), which can provide a better carbon source for the basic growth of microorganisms and then promote nutrient production and the basic metabolism of microorganisms. On the other hand, the dead microbial residues can release nutrients C, N, and P into the soil through soil mineralization. Therefore, the early stage of S. psammophila sand barrier decay increases soil microbial biomass. In the later stage of decay, the basic density of the sand barrier decreases, the degradation rate slows down, and the nutrients available for the normal growth of microorganisms decrease. The decrease in the substrate quality of sand barriers affects the abundance of fungi and bacterial communities (Kaiser et al., 2014), and the enhancement of the competition between species leads to the decrease in microbial species and quantity, which leads to the decrease in soil microbial biomass. In addition, studies have shown that soil SOC is a factor significantly affecting soil microbial biomass (Powlson et al., 1987; Lovell et al., 1995). Hu et al. Hu et al. (2012) showed that MBC and MBN were positively correlated with TN and SOC. This was also confirmed in the present study, where MBC, MBN, and MBP were significantly and positively correlated with SOC, TN, AN, pH, MC, and DOC (Figure 5). As a result, the soil nutrient content during the decay of S. psammophila sand barriers is an important factor affecting soil microbial biomass. This is consistent with previous research results that plantations could improve the soil nutrient status and thus increase the soil microbial biomass (Wang et al., 2020). The results of stepwise linear regression analysis showed that MBC was mainly affected by DOC and TN, while MBN was mainly affected by TN and SOC (Table 2). However, soil moisture content is an important environmental factor affecting soil microbial biomass (Baldrian et al., 2010). The DOC content is related to the role of the soil moisture content, and when soil moisture is sufficient, it can increase the ability of soil to dissolve organic carbon and stimulate the growth of microorganisms, thus increasing the MBC (Wang et al., 2020). In addition, the process of allotropic reduction of nitrate nitrogen to ammonium nitrogen by soil microorganisms reduced the loss of nitrate nitrogen and resulted in higher nitrogen retention capacity, which provided the necessary nitrogen source for microbial growth and increased the MBN content.
4.3 Effects of S. psammophila sand barrier decay on soil microbial stoichiometry
The MBC/MBN ratio is usually used to reflect the structure and status of the soil microbial community, and a higher ratio indicates the dominance of fungi in soil microorganisms, and conversely, a higher ratio of bacteria (Moore et al., 2000). The results of this study showed that MBC/MBN, MBC/MBP, and MBN/MBP did not change significantly in the early stage (≤3 years) of sand barrier setting but showed a significant increasing trend in the later stage (≥5 years). This is because the soil MBN and MBP decreased more than MBC after 7 years of sand barrier setting. The results of stepwise regression analysis showed that MBC/MBN, MBC/MBP, and MBN/MBP were mainly affected by BD, and the decrease in basic density was directly related to the loss of carbohydrate components such as cellulose and hemicellulose, which determine the modulus of the rupture index of natural materials (Hillis and Rozsa, 1984). However, the decomposition of the main chemical components directly affects the availability of nutrient elements in the soil, thereby promoting the growth of microorganisms and improving the stoichiometric ratio of soil microorganisms. Soil MBC/MBP and MBN/MBP can reflect the effectiveness of microbial nitrogen and phosphorus and can be used as indicators of the degree of plant nitrogen and phosphorus supply limitation (Tessier and Raynal, 2003). Pearson correlation analysis showed that soil MBC/MBP and MBN/MBP were correlated with SOC and DOC (Figure 5), and lower MBC/MBP and MBN/MBP indicated higher soil N and P effectiveness (Breuer et al., 2006; Jia et al., 2016). In this study, it was found that MBC/MBP and MBN/MBP significantly increased after 5 years of decay (Figures 4E,F). As a mechanical measure to assist vegetation restoration in desert ecosystems, desert vegetation may be susceptible to phosphorus limitation after 5 years. However, MBP can further replenish the soil available phosphorus pool and can alleviate the P limitation to a certain extent. In addition, we need to further investigate the potential of soil microorganisms to release P during the mineralization of organic matter in the future. If there is a greater potential for the release of P, it is also the main way to alleviate the P phosphorus limitation of vegetation.
5 Conclusion
With the increase in the S. psammophila sand barrier setting time, the flexural strength of the mechanical properties decreased the most, which directly led to collapse and damage. The changes in the soil MBC and MBN contents were consistent with the trend of the soil properties, and both reached their peak at 7 years. However, there was no significant change in MBC/MBP, and MBN/MBP in the early period (≤3 years) of the sand barrier setting, but there was a significant increase in the later period (≥5 years). These results indicated that S. psammophila sand barriers mainly played the role of windbreak and sand fixation in the early period, and made soil microorganisms susceptible to phosphorus limitation in the later period. Stepwise linear regression analysis showed that the relationship between MBC/MBN, MBC/MBP, and MBN/MBP and BD was more obvious, while MBC was significantly affected by DOC and TN, and MBN was significantly affected by TN and SOC. Therefore, the S. psammophila sand barrier changes soil properties by degrading its own chemical components during the decay process and the loss of basic density of the sand barrier is the main driving factor for increasing the C:N:P stoichiometry of soil microbial biomass. In the resource utilization process of mechanical sand barriers in the future, S. psammophila sand barriers can still be further promoted and used.
Data availability statement
The original contributions presented in the study are included in the article/Supplementary Material, further inquiries can be directed to the corresponding author.
Author contributions
YL, AR, and NW designed the study. YL and AR performed the fieldwork and the laboratory analysis. YL and NW analyzed the data and prepared the manuscript. All authors reviewed and agreed to the published version of the manuscript.
Funding
This research was supported by the Inner Mongolia Autonomous Region Science and Technology Planning Project (2022YFDZ0027), “Application and demonstration of supporting technologies for the restoration and management of wind erosion pits in Xilingol grassland.”
Conflict of interest
The authors declare that the research was conducted in the absence of any commercial or financial relationships that could be construed as a potential conflict of interest.
Publisher’s note
All claims expressed in this article are solely those of the authors and do not necessarily represent those of their affiliated organizations, or those of the publisher, the editors and the reviewers. Any product that may be evaluated in this article, or claim that may be made by its manufacturer, is not guaranteed or endorsed by the publisher.
References
Baldrian, P., Merhautová, V., Petránková, M., Cajthaml, T., and Snajdr, J. (2010). Distribution of microbial biomass and activity of extracellular enzymes in a hardwood forest soil reflect soil moisture content. Appl. Soil Ecol. 46, 177–182. doi:10.1016/j.apsoil.2010.08.013
Bauhus, J., and Barthel, R. (1995). Mechanisms for carbon and nutrient release and retention in beech forest gaps. II. The role of soil microbial biomass. Plant Soil 168/169, 585–592. doi:10.1007/bf00029372
Breuer, L., Huisman, J. A., Keller, T., and Frede, H. G. (2006). Impact of a conversion from cropland to grassland on C and Nstorage and related soil properties: Analysis of a 60-year chronosequence. Geoderma 133, 6–18. doi:10.1016/j.geoderma.2006.03.033
Brookes, P. C., Landman, A., Pruden, G., and Jenkinson, D. S. (1985). Chloroform fumigation and the release of soil nitrogen: A rapid direct extraction method to measure microbial biomass nitrogen in soil. Soil Biol. Biochem. 17 (6), 837–842. doi:10.1016/0038-0717(85)90144-0
Bugg, T. D. H., Ahmad, M., Hardiman, E. M., and Rahmanpour, R. (2011). Pathways for degradation of lignin in bacteria and fungi. Nat. Prod. Rep. 28 (12), 1883–1896. doi:10.1039/c1np00042j
Chen, Y . L., Chen, L. Y ., Peng, Y . F., Ding, J. Z., Li, F., Yang, G. B., et al. (2016). Linking microbial C : N : P stoichiometry to microbial community and abiotic factors along a 3500 km grassland transect on the Tibetan plateau. Glob. Ecol. Biogeogr. 25, 1416–1427. doi:10.1111/geb.12500
Cleveland, C. C., and Liptzin, D. (2007). C: N: P stoichiometry in soil: Is there a “redfield ratio” for the microbial biomass? Biogeochemistry 85, 235–252. doi:10.1007/s10533-007-9132-0
Deng, L., Peng, C. H., Huang, C. B., Wang, K. B., Liu, Q. Y., Liu, Y. L., et al. (2019). Drivers of soil microbial metabolic limitation changes along a vegetation restoration gradient on the Loess Plateau, China. Geoderma 353, 188–200. doi:10.1016/j.geoderma.2019.06.037
Douterelo, I., Goulder, R., and Lillie, M. (2010). Soil microbial community response to land-management and depth, related to the degradation of organic matter in English wetlands: Implications for the in situ preservation of archaeological remains. Appl. Soil Ecol. 44, 219–227. doi:10.1016/j.apsoil.2009.12.009
Falkowski, P. G., Fenchel, T., and Delong, E. F. (2008). The microbial engines that drive Earth's biogeochemical cycles. Science 320, 1034–1039. doi:10.1126/science.1153213
Fan, P., Zhou, N., Zhang, J., Lin, Y., and Zhang, L. (2013). Optimization of determination process of cellulose content with pine sawdust and nitric acid-ethanol method. Adv. Text. Technol. 21 (6), 1–5. doi:10.19398/j.att.2013.06.001
Fanin, N., Fromin, N., Buatois, B., and Hättenschwiler, S. (2013). An experimental test of the hypothesis of non-homeostatic consumer stoichiometry in a plant litter-microbe system. Ecol. Lett. 16, 764–772. doi:10.1111/ele.12108
Franzluebbers, A. J., Hons, F. M., and Zuberer, D. A. (1995). Soil organic carbon, microbial biomass, and mineralizable carbon and nitrogen in sorghum. Soil Sci. Soc. Am. J. 59, 460–466. doi:10.2136/sssaj1995.03615995005900020027x
Guo, X. P., Chen, H. Y. H., Meng, M. J., Biswas, S. R., Ye, L., and Zhang, J. (2016). Effects of land use change on the composition of soil microbial communities in a managed subtropical forest. For. Ecol. Manag. 373, 93–99. doi:10.1016/j.foreco.2016.03.048
Hammel, K. E. (1997). “Fungal degradation of lignin,” in Driven by nature: Plant litter quality and decomposition (Madison, United States: US Depatiment of Agriculture), 33–45.
Hillis, W. E., and Rozsa, A. N. (1984). High temperature and chemical effects on wood stability. Wood Sci. Technol. 19 (1), 93–102. doi:10.1007/bf00353070
Hu, Z. D., Liu, S. R., Shi, Z. M., Liu, X. L., and He, F. (2012). Variations of soil nitrogen and microbial biomass carbon and nitrogen of Quercus aquifolioides forest at different attitudes in Balangshan, Sichuan. For. Res. 25 (3), 261–268. doi:10.13275/j.cnki.lykxyj.2012.03.002
Jia, G. M., He, L., Cheng, H., Wang, S. T., Xiang, H. Y., Zhang, X. F., et al. (2016). Ecological stoichiometry characteristics of soil microbial biomass carbon, nitrogen and phosphorus under different vegetation covers in Three Gorges Reservoir Area. Res. Soil Water Conservation 23 (4), 23–27. doi:10.13869/j.cnki.rswc.20160518.001
Jing, X., Sanders, N. J., Shi, Y., Chu, H. Y., Classen, A. T., Zhao, K., et al. (2015). The links between ecosystem multifunctionality and above- and belowground biodiversity are mediated by climate. Nat. Commun. 6, 8159. doi:10.1038/ncomms9159
Joergensen, R. G., and Mueller, T. (1996). The fumigation-extraction method to estimate soil microbial biomass: Calibration of the kEN value. Soil Biol. Biochem. 28, 33–37. doi:10.1016/0038-0717(95)00101-8
Kaiser, C., Franklin, O., Dieckmann, U., and Richter, A. (2014). Microbial community dynamics alleviate stoichiometric constraints during litter decay. Ecol. Lett. 17, 680–690. doi:10.1111/ele.12269
Kornberg, A. (1995). Inorganic polyphosphate: Toward making a forgotten polymer unforgettable. J. Bacteriol. 177, 491–496. doi:10.1128/jb.177.3.491-496.1995
Leff, J. W., Jones, S. E., Prober, S. M., Barberan, A., Borer, E. T., Firn, J. L., et al. (2015). Consistent responses of soil microbial communities to elevated nutrient inputs in grasslands across the globe. Proc. Natl. Acad. Sci. U. S. A. 112, 10967–10972. doi:10.1073/pnas.1508382112
Li, P., Yang, Y. H., Han, W. X., and Fang, J. Y. (2014). Global patterns of soil microbial nitrogen and phosphorus stoichiometry in forest ecosystems. Glob. Ecol. Biogeogr. 23, 979–987. doi:10.1111/geb.12190
Liang, Y. M., Gao, Y., Wang, R. D., Duan, X. T., and Guo, X. (2022). Effects of Salix psammophila sand barriers decay on soil microbial biomass and enzyme activities. J. Soil Water Conservation 36 (2), 305–311. doi:10.13870/j.cnki.stbcxb.2022.02.039
Liu, L., and Bo, T. (2020). Effects of checkerboard sand barrier belt on sand transport and dune advance. Aeolian Res. 42, 100546. doi:10.1016/j.aeolia.2019.100546
Liu, Y., Ma, W., Kou, D., Niu, X., Wang, T., Chen, Y., et al. (2020). A comparison of patterns of microbial C: N: P stoichiometry between topsoil and subsoil along an aridity gradient. Biogeosciences 17, 2009–2019. doi:10.5194/bg-17-2009-2020
Lovell, R. D., Jarvis, S. C., and Bardgett, R. D. (1995). Soil microbial biomass and activity in long-term grassland: Effects of management changes. Soil Biol. Biochem. 27 (7), 969–975. doi:10.1016/0038-0717(94)00241-r
LY/T 2369 (2014). Testing methods for physical and mechanical properties of desert shrub. Beijing, China: Standardization Forestry Industry of China.
Mahata, S., and Sharma, V. N. (2021). The global problem of land degradation: A review. Varanasi: Banaras Hindu University.
Manzoni, S., Trofymow, J. A., Jackson, R. B., and Porporato, A. (2010). Stoichiometric controls on carbon, nitrogen, and phosphorus dynamics in decomposing litter. Ecol. Monogr. 80, 89–106. doi:10.1890/09-0179.1
Moore, J. M., Klose, S., and Tabatabai, M. A. (2000). Soil microbial biomass carbon and nitrogen as affected by cropping systems. Biol. Fertil. Soils 31, 200–210. doi:10.1007/s003740050646
Nelson, D., and Sommers, L. E. (1983). Total carbon, organic carbon and organic matter. Methods of Soil Analysis: Part 2 Chemical and Microbiological Properties 9, 539–579.
Powlson, D. S., Prookes, P. C., and Christensen, B. T. (1987). Measurement of soil microbial biomass provides an early indication of changes in total soil organic matter due to straw incorporation. Soil Biol. Biochem. 19 (2), 159–164. doi:10.1016/0038-0717(87)90076-9
Ren, Y. Y., Hu, C. Y., He, X., Li, R. F., and Zhao, G. P. (2007). The influence of Salix sandy barrier to vegetation restoration in sandy region. Res. Soil Water Conservation 14 (2), 13–15.
Sinsabaugh, R. L., Belnap, J., Rudgers, J., Kuske, C. R., Martinez, N., and Sandquist, D. (2015). Soil microbial responses to nitrogen addition in arid ecosystems. Front. Microbiol. 6, 819. doi:10.3389/fmicb.2015.00819
Sluiter, A., Hames, B., Ruiz, R., Scarlata, C., Sluiter, J., Templaton, D., et al. (2010). Determination of structural carbohydrates and lignin in biomass. Technical Report NREL/TP-510-42618. Golden, CO: National Renewable Energy Laboratory.
Stanford, G. (1982). Nitrogen in agricultural soils. Editor F. J. Stevenson (Madison, Wisconsin: Am Soc Agron), 651–688.
Tessier, J. T., and Raynal, D. J. (2003). Use of nitrogen to phosphorus ratios in plant tissue as an indicator of nutrient limitation and nitrogen saturation. J. Appl. Ecol. 40, 523–534. doi:10.1046/j.1365-2664.2003.00820.x
Van Der Heijden, M. G., Bardgett, R. D., and Van Straalen, N. M. (2008). The unseen majority: Soil microbes as drivers of plant diversity and productivity in terrestrial ecosystems. Ecol. Lett. 11, 296–310. doi:10.1111/j.1461-0248.2007.01139.x
Vance, E. D., Brookes, P. C., and Jenkinson, D. S. (1987). An extraction method for measuring soil microbial biomass C. Soil Biol. Biochem. 19, 703–707. doi:10.1016/0038-0717(87)90052-6
Wang, R. D., Gao, Y., Dang, X. H., Yang, X., Liang, Y., and Zhao, C. (2021a). Microstructure and biodegradation of long-established Salix psammophila sand barriers on sand dunes. Environ. Technol. Innovation 21, 101366. doi:10.1016/j.eti.2021.101366
Wang, R. D., Yang, X., Gao, Y., Dang, X., Liang, Y., Qi, S., et al. (2021b). Decomposition characteristics of long-established Salix psammophila sand barriers in an arid area, northwestern China. BioResources 16 (3), 5947–5963. doi:10.15376/biores.16.3.5947-5963
Wang, T., Wan, X. H., Cheng, L., Yang, J. Q., Zhang, B. B., Zou, B. Z., et al. (2020). Effects of broadleaved tree species on soil microbial stoichiometry in clear-cut patches of Cunninghamia lanceolata plantation. Chin. J. Appl. Ecol. 31 (11), 3851–3858. doi:10.13287/j.1001-9332.202011.034
Wilson, W. A., Roach, P. J., Montero, M., Baroja-Fernández, E., Munoz, F. J., Eydallin, G., et al. (2010). Regulation of glycogen metabolism in yeast and bacteria. FEMS Microbiol. Rev. 34, 952–985. doi:10.1111/j.1574-6976.2010.00220.x
You, Y., Zhou, N., and Wang, Y. (2021). Comparative study of desertification control policies and regulations in representative countries of the Belt and Road Initiative. Glob. Ecol. Conserv. 27, e01577. doi:10.1016/j.gecco.2021.e01577
Zechmeister-Boltenstern, S., Keiblinger, K. M., Mooshammer, M., Penuelas, J., Richter, A., Sardans, J., et al. (2015). The application of ecological stoichiometry to plant-microbial-soil organic matter transformations. Ecol. Monogr. 85, 133–155. doi:10.1890/14-0777.1
Keywords: Salix psammophila sand barriers, ecological restoration, decay processes, microbial biomass, stoichiometry
Citation: Liang Y, Ri A and Wang N (2022) Decay processes in Salix psammophila sand barriers increase soil microbial element stoichiomery ratios. Front. Environ. Sci. 10:1015821. doi: 10.3389/fenvs.2022.1015821
Received: 10 August 2022; Accepted: 14 November 2022;
Published: 05 December 2022.
Edited by:
Niall Patrick Hanan, New Mexico State University, United StatesReviewed by:
Lydia Bailey, Northern Arizona University, United StatesChristel Baum, University of Rostock, Germany
Copyright © 2022 Liang, Ri and Wang. This is an open-access article distributed under the terms of the Creative Commons Attribution License (CC BY). The use, distribution or reproduction in other forums is permitted, provided the original author(s) and the copyright owner(s) are credited and that the original publication in this journal is cited, in accordance with accepted academic practice. No use, distribution or reproduction is permitted which does not comply with these terms.
*Correspondence: Ana Ri, anari@emails.imau.edu.cn