Corrigendum: Biodegradation of 2-4-6 trichlorophenol by sequencing batch reactors (SBR) equipped with a rotating biological bed and operated in an anaerobic-aerobic condition
- Environmental Health Engineering Department, School of Public Health, Urmia University of Medical Science, Urmia, Iran
2-4-6 Trichlorophenol (TCP) is toxic, carcinogenic, and resistant to biodegradation. In this study, a rotating biological bed (RBB) was used to improve the efficiency of Sequencing batch reactors (SBR), and it was operated in sequential anaerobic and aerobic conditions. Biofilm growth on media of rotating biological bed was also confirmed using Scanning Electron Microscope (SEM). In this study, the effect of 2-4-6 trichlorophenol concentration (5–430 mg/L), hydraulic retention time (HRT) (12–30 h), the number of operating cycles per day (6–12 cycles/d), the type of combination of anaerobic and aerobic processes and the presence of a rotating biological bed and its rotation were studied. SBR equipped with a rotating biological bed (SBR-RBB) with the sequential anaerobic-aerobic operation in optimal operating conditions (TCP: 430 mg/L, cycles/d: 8, and HRT: 6 h) can remove nearly 100% of TCP and more than 95% of TP and COD. The role of the presence of an RBB in removing TCP, TP, and COD was 7, 20, and 23%, respectively. The role of rotation of RBB also was 23%, 10, 21, and 62%, respectively. So, SBR-RBB, with the sequential anaerobic-aerobic operation, was able to remove higher concentrations of TCP (430 mg/L) in a shorter HRT (6 h) with higher efficiency (nearly 100%) compared to previous studies. Therefore, for the first time in this study, the biological treatment of 430 mg/L of TCP is reported by a biological process.
Introduction
Phenol and phenolic compounds are resistant, toxic compounds with bactericidal and fungicidal properties (Aghapour et al., 2013a; Aghapour et al., 2013b). Chlorophenols result from combining phenol with chlorine and are usually more resistant and stable than phenol and are one of the serious environmental concerns (Mondal et al., 2011; Arora and Bae, 2014). The biodegradability of chlorophenols depends on the position and number of chlorine groups substituted in the aromatic composition of phenol; with increasing the number of substituted chlorines in the aromatic ring, the toxicity of these compounds increases, and their degradability decreases (Mondal et al., 2011). Industrial wastewaters mainly release chlorophenols (Li et al., 2011; Mondal et al., 2011). 2-4-6 Trichlorophenol (TCP) is one of the most toxic, resistant, and widely used chlorophenols. It enters the environment through wastewater from wood, pharmaceutical, military, petrochemical, textile, bleach, pesticides, herbicides, fungicides, insecticides, and disinfectant compounds (Han et al., 2015; Barik and Gogate, 2018). It is also considered one of the by-products of chlorination of drinking water (Nyström et al., 1992; Milia et al., 2016; Pan et al., 2017). The International Agency for Research on Cancer (IARC) places this substance in the B2 carcinogen (possibly carcinogenic to humans) (Halappa Gowda et al., 1985; Vainio and Bianchini, 2002; Huff, 2012).
Different methods have been studied to remove TCP from water and wastewater (Gaya et al., 2010; Joseph et al., 2011; Ding et al., 2016; Olu-Owolabi et al., 2017; Liang et al., 2022). To degrade and remove TCP from aquatic environments, it is necessary to use efficient, economical, and environmentally friendly treatment methods such as biological treatment processes (Aghapour et al., 2013a; Aghapour et al., 2013b; Aghapour et al., 2015; Azubuike et al., 2016; Salgot et al., 2016; Khorsandi et al., 2018; Khorsandi et al., 2020; Rezaei et al., 2022b). In biological processes, biomass can acclimate and degrade organic compounds due to the production of different enzymes (Aghapour et al., 2013a; Aghapour et al., 2013b; Aghapour et al., 2015; Khorsandi et al., 2018; Khorsandi et al., 2020; Rezaei et al., 2022b). Compared to other ways, biological processes require less cost, more straightforward operation, and better and more reliable performance (El-Naas et al., 2017). So, the study with stabilized microbial species shows that sequential anaerobic-aerobic processes effectively degrade TCP (Gardin et al., 2001). The SBR with a combined anaerobic-aerobic operation process is also considered a suitable decomposition process for removing industrially resistant compounds such as chlorinated aromatic compounds (Chan et al., 2009; Aghapour et al., 2013a; Aghapour et al., 2013b; Aghapour et al., 2015; Khorsandi et al., 2018; Khorsandi et al., 2020; Rezaei et al., 2022b). Due to the high toxicity of 2-4-6 Trichlorophenol, it is necessary to conduct additional studies on the biodegradation of this compound.
A review of scientific literature shows that anaerobic processes are more successful in dechlorinating organic compounds than aerobic processes (Chan et al., 2009). However, due to the slight difference between the oxidation-reduction potential of the electron donor (one part of the substrate) and the electron acceptor (another part of the substrate) in anaerobic processes, the rate of biodegradation reactions in anaerobic processes is usually lower than aerobic process. Therefore, after the initial dechlorination in the anaerobic step, the biodegradation of the produced aromatic intermediate compounds in these conditions usually requires more retention time (Metcalf et al., 1991).
Given the high rate of aerobic reactions and the high ability of aerobic processes to biodegrade aromatic compounds, these processes are usually superior to anaerobic processes (Aghapour et al., 2013a; Aghapour et al., 2013b; Pantea et al., 2013; Metcalf et al., 2014; Aghapour et al., 2015; Khorsandi et al., 2018; Khorsandi et al., 2020; Rezaei et al., 2022b). Therefore, combining anaerobic-aerobic processes for dehalogenation and biodegradation of TCP can be more effective than separate aerobic and anaerobic processes.
SBR is one of the most important and usable processes in treating industrial wastewater containing toxic and degradable compounds (Metcalf et al., 1991; Aghapour et al., 2013a; Aghapour et al., 2013b; Aghapour et al., 2015; Khorsandi et al., 2018; Khorsandi et al., 2020; Rezaei et al., 2022b). The operation of SBR includes four phases of feeding, reaction, settling, and decant, and it is possible to operate it as a combined anaerobic-aerobic in its reaction phase (Metcalf et al., 1991). Due to the high toxicity and resistance of TCP to biodegradation, it is necessary to increase the resistance of the biological treatment system to shocks caused by the entry of toxic and resistant substances. The rotating biological bed (RBB) increases the transfer rate of the substrate to the biomass and biofilm due to the rotation inside the bioreactor. On the other hand, it increases the resistance of the biological treatment system to the shock of toxic substances due to the high diversity of biological species grown on media and their cooperation with suspended biological agents in the bioreactor (Aghapour et al., 2013b; Barwal and Chaudhary, 2014). Therefore, this study aimed to evaluate the efficiency of SBR-RBB and operated in a sequential anaerobic and aerobic condition for the biodegradation of TCP.
Materials and methods
Materials
For the preparation of synthetic wastewater, TCP (99% MERK) was used as a substrate, and K2HPO4, KH2PO4, NH4Cl, and (NH4)2HPO4 as nutrients required for biomass with Merck brand (99%) were used. The stock solution of TCP was used to feed the bioreactor with different concentrations of TCP. To supply the nutrients (N and P) required for biomass, the stock solution of nutrients with COD: N:P ratio equal to 100:5:1 was used (Aghapour et al., 2013a; Aghapour et al., 2013b; Aghapour et al., 2015; Khorsandi et al., 2018; Khorsandi et al., 2020; Rezaei et al., 2022b). The stock of nutrient solution used in this study was prepared and used by dissolving 5 g of potassium phosphate, 15 g of potassium dihydrogen, 120 g of ammonium chloride, and 12 g of diammonium phosphate in 1 L of tap water (Aghapour et al., 2013a; Aghapour et al., 2013b; Aghapour et al., 2015; Khorsandi et al., 2018; Khorsandi et al., 2020; Rezaei et al., 2022b).
To identify the biofilm morphology formed on the 2H media in the SBR-RBB, a scanning electron microscope (SEM) with the Philips brand (model: XL30). The 2H media were removed from RBB under optimal conditions and dried at 25°C for 24 h. The biofilms attached to the surfaces of 2H media were then imaged using SEM (Aghapour et al., 2013b).
Acclimation
This study used activated sludge from Urmia municipal wastewater treatment plant (biolak process) as the primary biomass with an MLSS concentration of about 5,000 mg/L. The biomass Acclimation was performed separately in anaerobic and aerobic SBR equipped with a rotating biological bed with a volume of 3.5 L. Due to TCP’s high toxicity and resistance to biodegradation, an initial concentration of 3.5 mg/L was used to acclimate biomass in aerobic and anaerobic processes. The operating conditions of bioreactors for sludge acclimation are given in Table 1. The acclimatized sludge was transferred to the SBR equipped with a rotating biological bed and operated in sequential anaerobic and aerobic conditions.
Experimental setup
The bioreactor was made on a laboratory scale in the form of a cube with dimensions of 18 × 18 × 25 cm and 8 L (Figure 1). For biofilm growth on media, 30% of the practical volume of the bioreactor was filled with 2H media (HDPE-2H). The 2H media was housed inside a cubic lattice package and rotated inside the bioreactor with a vertical axis by a gearbox motor at 25 rpm. A mixer with a diameter of 10 cm and a height of 2 cm was used at the bottom of the media package to mix the sludge deposited in the bottom of the bioreactor. An air pump (BUYO) was used to supply the air and oxygen needed for the bioreactor. Due to the blockage of the reactor, a one-way gas outlet pipe was installed in the upper part of the bioreactor to remove the gases produced by the bioreactor. The operating conditions of the bioreactor are given in Table 1.
Before any change in operating conditions and studying the effect of another parameter on the bioreactor’s efficiency, the bioreactor’s efficiency should have reached steady-state conditions. Steady-state conditions in this study are conditions in which the efficiency of the bioreactor is less than 3% in 1 week (Aghapour et al., 2013a; Aghapour et al., 2013b; Aghapour et al., 2015; Khorsandi et al., 2018; Khorsandi et al., 2020; Rezaei et al., 2022b).
Analysis
TCP concentration in the samples was determined using Agilent 1206 HPLC with C18 column (4.6 μm × 100 mm × 3.5 μm) and UV detector at 290 nm. The mobile phase was a mixture of methanol/water/acetic acid in a 1/29/70 with a flow rate of 1 ml/min, and the concentration was measured at room temperature (Li et al., 2011). Due to the possibility of converting TCP to other phenolic compounds, the total phenolic compounds (TP) were determined using the standard method 5220 D (Mondal et al., 2011). COD concentration was determined by standard methods 5530 D (2). The MLSS concentration was measured using the thermal gravimetric method, the 2540 D method, standard method book (Mondal et al., 2011). All samples were analyzed after filtration with a membrane filter (Whatman) with a pore size of 0.45 μm (Aghapour et al., 2013a; Aghapour et al., 2013b; Aghapour et al., 2015; Khorsandi et al., 2018; Khorsandi et al., 2020; Rezaei et al., 2022b).
Results and discussion
Acclimation
Due to the predominance of aerobic species in the activated sludge of municipal wastewater treatment plants (biolak process), to strengthen anaerobic species and enhance biofilm growth on media, in this study, separate anaerobic and aerobic bioreactors were used for biomass acclimation (Aghapour et al., 2013a; Aghapour et al., 2013b; Aghapour et al., 2015; Khorsandi et al., 2018; Khorsandi et al., 2020; Rezaei et al., 2022a; Rezaei et al., 2022b). To acclimate the biomass in each bioreactor, synthetic wastewater containing TCP with a concentration of 3.5 mg/L was used. Figure 2 (a and b) show the results related to the biomass acclimation in separate anaerobic and aerobic bioreactors.
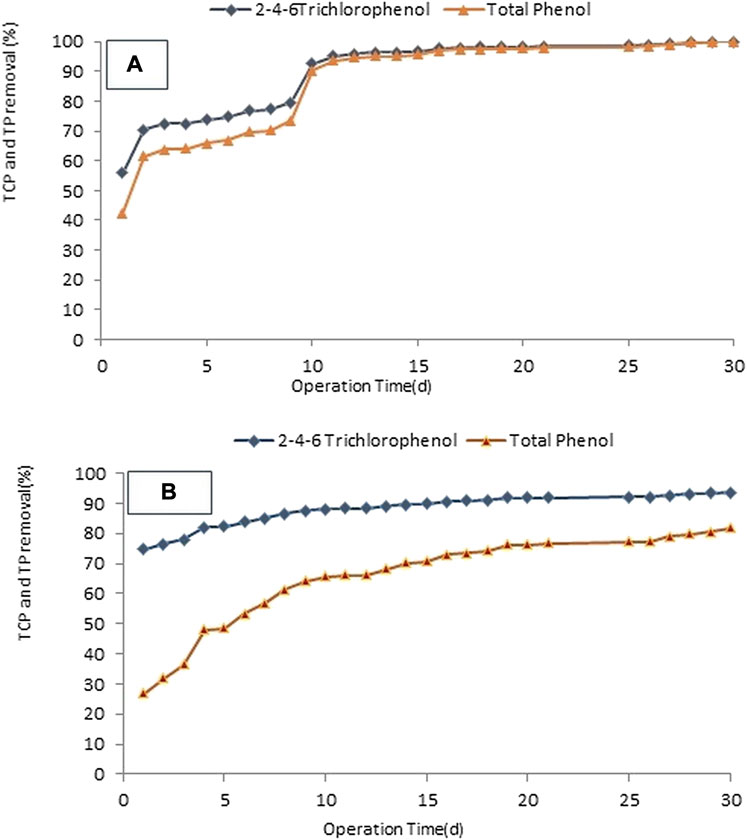
FIGURE 2. TCP and TP removal during the Acclimation phase. (A,B). Biomass acclimation in separate anaerobic and aerobic bioreactors.
As shown in Figure 2, the anaerobic and aerobic bioreactors successfully acclimated to a 3.5 mg/L of TCP concentration and reached a steady-state condition after 1 month. At this step, microorganisms acquire the enzymes needed for the dehalogenation and biodegradation of TCP (Li et al., 2011; Mondal et al., 2011). The mean removal efficiencies of TCP and TP at the steady-state condition of the acclimation step were 93% and 73% in anaerobic bioreactors and 99% and 99% in aerobic bioreactors, respectively. As shown in Figure 2, the efficiency of the anaerobic bioreactor in the dechlorination and degradation of TCP is higher than TP. The low biodegradation efficiency of TP can be related to the lower rate of anaerobic reactions compared to aerobic reactions. Previous studies indicate that the degradation of phenolic compounds occurs at a high rate during the aerobic process (Aghapour et al., 2013a; Aghapour et al., 2013b; Arora and Bae, 2014; Aghapour et al., 2015; Khorsandi et al., 2018; Khorsandi et al., 2020; Rezaei et al., 2022b). Therefore, this study will use a combination of anaerobic and aerobic processes for the biodegradation of TCP. In biological processes, the duration of the biomass acclimation period varies from a few days to several months, depending on the type of compounds in the incoming wastewater (Mondal et al., 2011; Aghapour et al., 2013a; Aghapour et al., 2013b; Aghapour et al., 2015; Han et al., 2015; Barik & Gogate, 2018; Khorsandi et al., 2018; Khorsandi et al., 2020; Rezaei et al., 2022a; Rezaei et al., 2022b). In this study, the biomass in each bioreactor was acclimated to TCP after 30 days. A study was not found on acclimating biological mass to 2-4-6 trichlorophenol in biological processes. However, the duration of acclimation of microorganisms under anaerobic conditions with 2-chlorophenol was 23 days (Nyström et al., 1992), and another study was reported to be about 4 months (Milia et al., 2016). The toxicity of chlorophenol compounds usually increases with increasing the number of chlorine atoms attached to the aromatic ring (Pan et al., 2017); it can be concluded that the biomass’s acclimation has taken place in a shorter time due to the high toxicity of 2-4-6 trichlorophenol compared to the above compound. The low acclimation time in this study could be due to the use of an RBB and the growth of biological agents with high diversity and number in the biofilm formed on the media, increasing their contact with inlet wastewater.
The effect of TCP concentration
After complete biomass acclimation to TCP, TCP-acclimated sludge was transferred to SBR -RBB with the sequential anaerobic and aerobic operation. Due to the complete acclimation of the biomass to TCP and the production of enzymes required for biodegradation in separate bioreactors, the concentration of TCP starts from 5 mg/L. It gradually increases to 10, 20, 50, 100, 200, 300, and 430 mg/L. The results of the effect of TCP concentration on bioreactor efficiency are presented in Figure 3.
As shown in Figure 3, with increasing TCP concentration, the bioreactor efficiency in removing TCP, TP, and COD decreased rapidly but, after a few days, gradually increased and reached a steady-state condition. The most significant decrease in bioreactor efficiency was observed at a TCP concentration of 10 mg/L. In 1 day, the removal efficiency of TCP and TP decreased from 99% to 96%–97% and 89%, respectively. Then it gradually increased and reached a steady-state condition for about 5 days. However, at concentrations of 10–200 mg/L, the efficiency of the bioreactor did not change much. By increasing the concentration from 200 to 300 and 430 mg/L, due to the increased toxicity and inhibitory effect of TCP on the growth and metabolism of the biomass, the bioreactor efficiency decreased and gradually increased and reached a steady-state condition. It is related to the reinforcement of biological agents of wastewater treatment in terms of type and number created by increasing the biofilm with a rotating biological bed (Aghapour et al., 2013a; Aghapour et al., 2013b; Aghapour et al., 2015; Khorsandi et al., 2018; Khorsandi et al., 2020; Rezaei et al., 2022a; Rezaei et al., 2022b). Also, the bioreactor operation under sequential anaerobic and aerobic conditions has led to the growth of resistant biological species with a high potential for biological degradation of TCP(Huff, 2012).
A comparison of the results of this study with the combined anaerobic UASB and aerobic process shows that the maximum tolerable concentration of TCP is 100 mg/L (Ding et al., 2016). In another study using an acidified UASB reactor, the maximum treatable concentration of TCP was determined to be 70 mg/L (Díaz-Báez and Valderrama-Rincon, 2017). Also, the removal efficiency of 2-4 dichlorophenol in anaerobic-aerobic processes with series operations with a concentration of 100 mg/L has been reported to be 86% (Olu-Owolabi et al., 2017). Therefore, SBR-RBB can biologically degrade higher concentrations (430 mg/L) of TCP and meet environmental standards (TP = 1 mg/L).
The effect of hydraulic retention time
The hydraulic retention time (HRT) of the bioreactor is considered an essential design and effective parameter in the construction costs of the treatment plant (Aghapour et al., 2013a; Aghapour et al., 2013b; Aghapour et al., 2015; Khorsandi et al., 2018; Khorsandi et al., 2020; Rezaei et al., 2022a; Rezaei et al., 2022b). In this study, HRT started from 30 h and was gradually reduced to 25, 20, 16, and 12 h after reaching steady-state conditions. The results related to the effect of HRT are given in Figure 4.
As shown in Figure 4, by reducing HRT, the efficiency of the bioreactor in removing TCP, TP, and COD decreased and, after a few days, gradually increased and reached a steady-state condition. The mean removal efficiencies of TCP and TP in steady-state conditions and with an HRT of 30, 25, 20, 16, and 12 h are almost 100%, and for COD related to TCP were determined 95, 95, 95, 95, and 94%. Due to the high efficiency and ability of the bioreactor to biodegrade TCP by reducing the HRT, the removal efficiency of TCP and TP did not change much, and only the COD removal efficiency associated with TCP decreased and gradually increased after a few days and has reached a steady-state condition (Aghapour et al., 2013a; Aghapour et al., 2013b; Aghapour et al., 2015; Khorsandi et al., 2018; Khorsandi et al., 2020; Rezaei et al., 2022a; Rezaei et al., 2022b).
Effect of the number of operation cycles per day
The selected process in this study was quickly able to withstand the shocks, and the reduction of HRT did not cause much change in its efficiency. Therefore, to determine the maximum capability of this bioreactor, the number of cycles of operation of the bioreactor was increased from four cycles per day to 6, 8, 10, and 12 cycles/d, respectively. The results related to the effect of the number of operation cycles on bioreactor efficiency are given in Figure 5.
As shown in Figure 5, as the number of operating cycles increases and the duration of the anaerobic and aerobic steps decreases, the HRT of the bioreactor decreases from 12 h to 8, 6, 4.8, and 4 h. In the number of cycles of 6, 8, 10, and 12 cycles/d (equivalent to HRT of 8, 6, 4.8, and 4 h), in steady-state conditions, the average removal efficiencies of bioreactor are 100%, 100%, 100% and 97% for TCP, 100%, 100%, 99% and 91% for TP and 94%, 93%, 93% and 76% for COD, respectively.
Optimum HRT has been reported in the biodegradation of TCP in the combined anaerobic UASB and aerobic process for 11 days. Also, for the degradation of 2-4 dichlorophenol by an anaerobic-aerobic process, an HRT of 26.4 h has been reported (Olu-Owolabi et al., 2017). A comparison of the results of this study with previous studies shows that the SBR-RBB can purify much higher concentrations of TCP (430 mg/L) at a much lower HRT (6 h) than previous studies.
Identification of biofilm morphology formed on the 2H media in RBB
The SEM technique was used to investigate the surface morphology of the biofilm formed on the 2H media. Images of fresh 2H media and 2H media with biofilm are presented in Figure 6. Comparing fresh media surface and biofilm media images shows that the fresh media surface is uneven and has fine pores. At the same time the 2H media surface used in the bioreactor has a biofilm without pores and is almost smooth and cloudy, confirming the creation of biofilm on 2H media (Aghapour et al., 2013b; Aghapour et al., 2015; Khorsandi et al., 2020).
Effect of anaerobic and aerobic process sequence on SBR-RBB
To determine the effect of anaerobic and aerobic process sequences on the removal of TCP, TP, and COD, the efficiency of the bioreactor in anaerobic-aerobic operation was compared with aerobic-anaerobic operating conditions. After each anaerobic step, the bioreactor output was sampled to determine the role of aerobic and anaerobic processes in each operating condition. The removal rate of TCP, TP, and COD was measured. The results are shown in Figure 7.
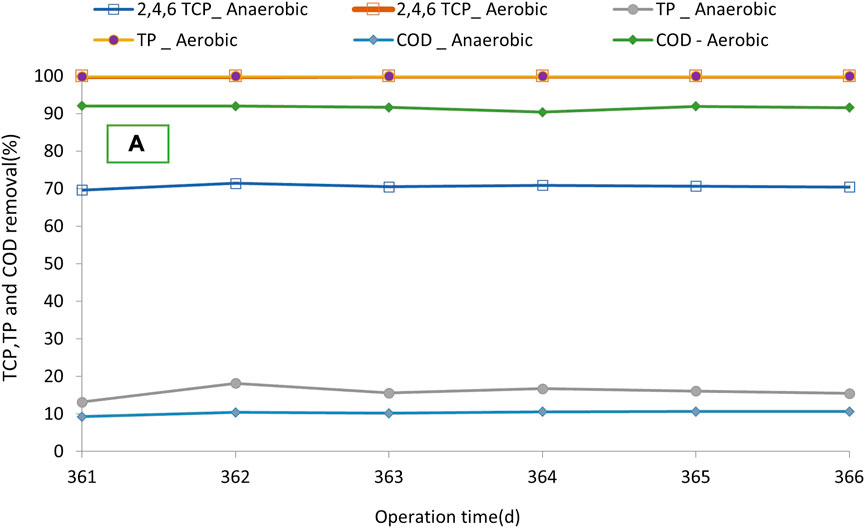
FIGURE 7. Effect of sequence anaerobic and aerobic conditions on SBR-RBB. (A) Anaerobic and Aerobic conditions sequence, B: Aerobic and Anaerobic conditions sequence.
Results related to the operation of the SBR-RBB as anaerobic-aerobic condition and the precedence of the anaerobic step over the aerobic step in Part A of Figure 7 and the results related to the operation of SBR-RBB as aerobic-anaerobic condition and the priority of the aerobic step over the anaerobic step is given in Part B of Figure 7. As shown in Figure 6, if the anaerobic step precedes the aerobic step, the SBR-RBB is more efficient in removing TCP, TP, and COD. The efficiency of SBR-RBB in both operating modes and the separate role of each anaerobic and aerobic process are summarized in Figure 8.
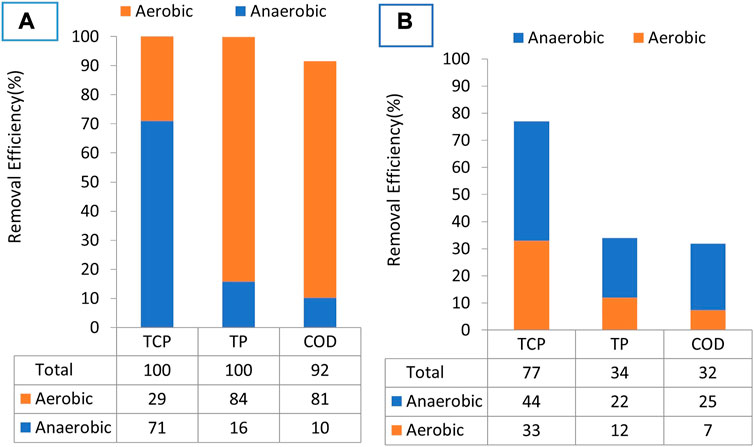
FIGURE 8. summarizes the effect of the sequence of anaerobic and aerobic conditions on SBR-RBB. (A) Average removal of TCP, TP, and COD in the anaerobic and Aerobic sequence. (B) Average removal of TCP, TP, and COD in the aerobic and Anaerobic sequence.
According to Section A of Figures 7, 8, in the operation of the bioreactor with the anaerobic-aerobic sequence, due to the priority of the anaerobic step and high TCP dechlorination rate in the anaerobic step, the efficiency of the treatment system in removing TCP (71%) is always higher than the removal of TP (16%) and COD (10%) (Gaya et al., 2010). During the dehalogenation step, the chlorine atoms bonded to carbons 6, 2, and four are removed from TCP, converted to 2-4 dichlorophenols, four monochlorophenol, and finally phenol. Therefore, in the dehalogenation step, the TP concentration does not decrease much despite the decrease in TCP concentration (Song et al., 2018). Also, due to the presence of intermediates from the dechlorination of TCP, the efficiency of COD is not significantly reduced.
In the aerobic step, adding oxygen to the phenolic compounds produced in the anaerobic step is first converted to catechol and then to 2-hydroxy munconic semialdehyde. Finally, during the tricarboxylic acid cycle (TCA) or Krebs cycle, they become the final products of biological reactions. So, in the aerobic step, the removal efficiency of TP (84%) and COD (81%) increased more than in the anaerobic step (Li et al., 2011; Azubuike et al., 2016). Thus, the bioreactor can remove about 100% of TCP and TP and 92% of COD when used with anaerobic-aerobic sequences. These results are consistent with previous studies on the high rate of dechlorination of chlorophenols in anaerobic processes compared to aerobic processes. By dechlorination in the anaerobic step, TCP is converted to intermediate compounds with high biodegradability, such as phenol, and due to the high rate of biodegradation of intermediates produced in the aerobic process, the efficiency of the SBR-RBB increases. Thus, it can be concluded that the anaerobic process is more efficient in the dechlorination and conversion of TCP to phenolic compounds, and the aerobic process is more efficient in the biodegradation of phenolic compounds.
According to Section B of Figures 7, 8, in the operation of the bioreactor with aerobic-anaerobic sequence, due to the priority of the aerobic step, the efficiency of the bioreactor in removing TCP (77%), TP (34%), and COD (32%) are much lower than operating conditions with anaerobic-aerobic sequence. The low efficiency of the bioreactor under the aerobic-anaerobic sequence is related to the degradation pathway of the TCP in the aerobic step. TCP dechlorination and TP degradation are not performed separately in the aerobic step. The main pathway of degradation of TCP in the aerobic phase (1 hour) consists of two steps. In the first step, the enzyme TCP hydroxylase converts it to 2,6-Dichlorohydroquinone by replacing the OH with the chlorine atom bonded carbon four of the phenol ring in the TCP compound. Therefore, a part of chlorination is performed at this step. In the second step, the enzyme chlorohydroquinone1,2-dioxygenase breaks it down by adding oxygen to the bond of 1-2 phenol rings. Therefore, due to the breakdown of the phenol ring by oxygenase enzymes, the concentration of TP in the bioreactor is also reduced (Salgot et al., 2016). However, due to the short time in the aerobic step, biodegradation reactions are not complete, and therefore the removal of COD (7%) is also low. During the anaerobic phase, dehalogenation of the remaining TCP and degradation of intermediates produced in the aerobic step is performed. So, it can be concluded that the efficiency of the SBR-RBB is significantly reduced in operation with aerobic and anaerobic sequences. Therefore, it is better to use anaerobic-aerobic sequences operation in biological treatment systems used to biodegrade TCP and other halogenated aromatic compounds to achieve high efficiency at low HRT.
The effect of a rotating biological bed
To investigate the effect of rotation of the biological bed on the bioreactor efficiency with the anaerobic-aerobic sequence, the electromotor of the mixer was turned off, and the biological bed was operated as a fixed bed. According to Figure 9 (Section A), in these conditions (days 377–394), the removal efficiency of TCP and TP from 100% and the removal efficiency of COD from 90.5% decreased to 93, 80, and 67%. After this step (on days 395–401), the electromotor was activated, and the system was put into steady-state operation again (Section B). Therefore, the role of the rotation of the biological bed in removing TCP, TP, and COD was 7, 20, and 23%, respectively.
To investigate the effect of the RBB on the efficiency of the bioreactor under optimal operating conditions (concentration 430 mg/L and hydraulic retention time of 6 h), the media inside the rotating package were taken out of the bioreactor, and the bioreactor was used only with suspended biomass. According to Figure 9 (Section C), in these conditions, the removal efficiency of TCP and TP decreased from about 100% and the removal efficiency of COD from 90% to 79.4, 37.6, and 28.8%. Therefore, the role of the rotating biological bed in removing TCP, TP, and COD was 10, 21, and 62%, respectively. Comparing the effect of rotation and the presence of a rotating biological bed, it can be concluded that rotation and the presence of a rotating biological bed, due to the increase in the transfer rate of the substrate to the biofilm, have the most significant effect on COD removal.
Based on the obtained results, upgrading SBR with an RBB and operation with anaerobic and aerobic sequences is the main factor in increasing the rate of biodegradation and the efficiency of the bioreactor in the biodegradation and mineralization of TCP. The study results are consistent with the study of equipping the SCR (continuous-inflow reactor) with a rotating biological bed (Aghapour et al., 2013b).
The study limitation
One of the important limitations of this study was the impossibility of measuring the concentration of COD in the effluent from the bioreactor, due to its low concentration, at TCP input concentrations lower than 10 mg/L. Also, the bad smell of TCP composition was one of the other important limitations of this study. It was eliminated in optimal operating conditions (anaerobic/aerobic conditions) due to its rapid dehalogenation in anaerobic conditions.
Conclusion
The following conclusions are drawn based on the results obtained from the biological degradation of 2-4-6 trichlorophenol:
- The time required for aerobic and anaerobic biomass acclimation to 2-4-6 trichlorophenol at a concentration of 3.5 mg/L and HRT of 24 h was about 1 month.
- The optimal concentration of 2-4-6 trichlorophenol and HRT in this hybrid process was determined to be 430 mg/L and 6 h, respectively.
- The best way to combine biological processes to achieve high efficiency and capacity of the bioreactor in the biodegradation of 2-4-6 trichlorophenol is to arrange anaerobic and aerobic processes.
- The presence of the biological bed and its rotation in the bioreactor had a very influential role in the efficiency and capacity of the bioreactor for the biodegradation of trichlorophenol.
Therefore, Due to the high capacity of this hybrid process in accepting high concentrations of trichlorophenol with low HRT and almost complete removal of this compound, it can be used as a helpful technique in biodegradation and mineralization of complex chlorinated organic compounds.
Data availability statement
The raw data supporting the conclusions of this article will be made available by the authors, without undue reservation.
Author contributions
All authors listed have made a substantial, direct, and intellectual contribution to the work and approved it for publication.
Funding
This manuscript is part of the results of a master’s thesis entitled “ investigation of the efficiency of a modified Sequencing Batch Reactor (SBR) in biodegradation of 2-4-6 trichlorophenol as a toxic compound in aqueous solutions ” with the research project code of 1395-01-34-2289 at the Urmia University of Medical Sciences.
Acknowledgments
This paper is a part of the results of a master’s thesis in Environmental Health Engineering. We thank Urmia University of Medical Sciences for financing the research.
Conflict of interest
The authors declare that the research was conducted in the absence of any commercial or financial relationships that could be construed as a potential conflict of interest.
Publisher’s note
All claims expressed in this article are solely those of the authors and do not necessarily represent those of their affiliated organizations, or those of the publisher, the editors and the reviewers. Any product that may be evaluated in this article, or claim that may be made by its manufacturer, is not guaranteed or endorsed by the publisher.
References
Aghapour, A. A., Moussavi, G., and Yaghmaeian, K. (2013a). Biological degradation of catechol in wastewater using the sequencing continuous-inflow reactor (SCR). J. Environ. Health Sci. Eng. 11 (1), 3. doi:10.1186/2052-336x-11-3
Aghapour, A. A., Moussavi, G., and Yaghmaeian, K. (2015). Degradation and COD removal of catechol in wastewater using the catalytic ozonation process combined with the cyclic rotating-bed biological reactor. J. Environ. Manag. 157, 262–266. doi:10.1016/j.jenvman.2015.02.036
Aghapour, A. A., Moussavi, G., and Yaghmaeian, K. (2013b). Investigating the performance of a novel cyclic rotating-bed biological reactor compared with a sequencing continuous-inflow reactor for biodegradation of catechol in wastewater. Bioresour. Technol. 138, 369–372. doi:10.1016/j.biortech.2013.03.133
Arora, P. K., and Bae, H. (2014). Bacterial degradation of chlorophenols and their derivatives. Microb. Cell Fact. 13 (1), 31. doi:10.1186/1475-2859-13-31
Azubuike, C. C., Chikere, C. B., and Okpokwasili, G. C. (2016). Bioremediation techniques-classification based on site of application: Principles, advantages, limitations and prospects. World J. Microbiol. Biotechnol. 32 (11), 180. doi:10.1007/s11274-016-2137-x
Barik, A. J., and Gogate, P. R. (2018). Hybrid treatment strategies for 2,4,6-trichlorophenol degradation based on combination of hydrodynamic cavitation and AOPs. Ultrason. Sonochemistry 40, 383–394. doi:10.1016/j.ultsonch.2017.07.029
Barwal, A., and Chaudhary, R. (2014). To study the performance of biocarriers in moving bed biofilm reactor (MBBR) technology and kinetics of biofilm for retrofitting the existing aerobic treatment systems: A review. Rev. Environ. Sci. Biotechnol. 13 (3), 285–299. doi:10.1007/s11157-014-9333-7
Chan, Y. J., Chong, M. F., Law, C. L., and Hassell, D. G. (2009). A review on anaerobic-aerobic treatment of industrial and municipal wastewater. Chem. Eng. J. 155 (1), 1–18. doi:10.1016/j.cej.2009.06.041
Díaz-Báez, M. C., and Valderrama-Rincon, J. D. (2017). Rapid restoration of methanogenesis in an acidified UASB reactor treating 2,4,6-trichlorophenol (TCP). J. Hazard. Mater. 324, 599–604. doi:10.1016/j.jhazmat.2016.11.031
Ding, Y., Sun, W., Cao, L., and Yang, J. (2016). A spontaneous catalytic membrane reactor to dechlorinate 2,4,6-TCP as an organic pollutant in wastewater and to reclaim electricity simultaneously. Chem. Eng. J. 285, 573–580. doi:10.1016/j.cej.2015.10.034
El-Naas, M. H., Mousa, H. A., and Gamal, M. E. (2017). “Microbial degradation of chlorophenols,” in Microbe-induced degradation of pesticides (Berlin, Germany: Springer), Heidelberg, Germany 23–58. doi:10.1007/978-3-319-45156-5_2
Gardin, H., Lebeault, J., and Pauss, A. (2001). Degradation of 2,4,6-trichlorophenol (2,4,6-TCP) by co-immobilization of anaerobic and aerobic microbial communities in an upflow reactor under air-limited conditions. Appl. Microbiol. Biotechnol. 56 (3), 524–530. doi:10.1007/s002530000577
Gaya, U. I., Abdullah, A. H., Hussein, M. Z., and Zainal, Z. (2010). Photocatalytic removal of 2,4,6-trichlorophenol from water exploiting commercial ZnO powder. Desalination 263 (1), 176–182. doi:10.1016/j.desal.2010.06.055
Halappa Gowda, T. P., Lock, J. D., and Kurtz, R. G. (1985). A comprehensive study of risk assessment for a hazardous compound of public health concern. Water Air Soil Pollut. 24 (2), 189–206. doi:10.1007/bf00285444
Han, S., Li, X., Wang, Y., and Chen, S. (2015). Graphene oxide-based fluorescence molecularly imprinted composite for recognition and separation of 2,4,6-trichlorophenol. RSC Adv. 5 (3), 2129–2136. doi:10.1039/c4ra11029c
Huff, J. (2012). Long-term toxicology and carcinogenicity of 2,4,6-trichlorophenol. Chemosphere 89 (5), 521–525. doi:10.1016/j.chemosphere.2012.05.015
Joseph, C. G., Puma, G. L., Bono, A., Taufiq-Yap, Y. H., and Krishnaiah, D. (2011). Operating parameters and synergistic effects of combining ultrasound and ultraviolet irradiation in the degradation of 2,4,6-trichlorophenol. Desalination 276 (1), 303–309. doi:10.1016/j.desal.2011.03.068
khan, M. Z., Mondal, P. K., Sabir, S., and Tare, V. (2011). Degradation pathway, toxicity and kinetics of 2, 4, 6-trichlorophenol with different co-substrate by aerobic granules in SBR. Bioresour. Technol., 102(13), 7016–7021. doi:10.1016/j.biortech.2011.04.057
Khorsandi, H., Ghochlavi, N., and Aghapour, A. A. (2018). Biological degradation of 2,4,6-trichlorophenol by a sequencing batch reactor. Environ. Process. 5 (4), 907–917. doi:10.1007/s40710-018-0333-4
Khorsandi, H., Gholizadeh, M., and Aghapour, A. A. (2020). Catechol biodegradation by a novel hybrid anoxic biofilter. Environ. Technol. 41 (6), 777–784. doi:10.1080/09593330.2018.1510434
Li, J., Cai, W., and Zhu, L. (2011). The characteristics and enzyme activities of 4-chlorophenol biodegradation by Fusarium sp. Bioresour. Technol. 102 (3), 2985–2989. doi:10.1016/j.biortech.2010.10.006
Liang, Y., Zhao, T., Xiao, B., Xiong, J., Wang, S., and Zhu, H., (2022). 2,4,6-Trichlorophenol degradation mechanism and microbial community analysis in an intimately coupled visible-light photocatalysis and biodegradation system. J Chem. Tech Biotech 97 (9), 2547–2556. doi:10.1002/jctb.7127
Metcalf, E., Abu-Orf, M., Bowden, G., Burton, F. L., Pfrang, W., and Stensel, H. D., , and AECOM. (2014). Wastewater engineering: Treatment and resource recovery. New York: McGraw Hill Education.
Metcalf, L., Eddy, H. P., and Tchobanoglous, G. (1991). Wastewater engineering: Treatment, disposal, and reuse. New York: McGraw-Hill.
Milia, S., Porcu, R., Rossetti, S., and Carucci, A. (2016). Performance and characteristics of aerobic granular sludge degrading 2,4,6-trichlorophenol at different volumetric organic loading rates. Clean. Soil Air Water 44 (6), 615–623. doi:10.1002/clen.201500127
Nyström, A., Grimvall, A., Krantz-Rüilcker, C., Sävenhed, R., and Åkerstrand, K. (1992). Drinking water off-flavour caused by 2, 4, 6-trichloroanisole. Water Sci. Technol. 25 (2), 241–249. doi:10.2166/wst.1992.0058
Olu-Owolabi, B. I., Alabi, A. H., Diagboya, P. N., Unuabonah, E. I., and Düring, R.-A. (2017). Adsorptive removal of 2, 4, 6-trichlorophenol in aqueous solution using calcined kaolinite-biomass composites. J. Environ. Manag. 192, 94–99. doi:10.1016/j.jenvman.2017.01.055
Pan, Y., Wang, Y., Li, A., Xu, B., Xian, Q., and Shuang, C., (2017). Detection, formation and occurrence of 13 new polar phenolic chlorinated and brominated disinfection byproducts in drinking water. Water Res. 112, 129–136. doi:10.1016/j.watres.2017.01.037
Pantea, E. V., Tamara, R., Carmen, G., and Blaj, I. (2013). comparison of efficiency of different type systems for wastewater treatment. Analele Universităţii din Oradea, Fascicula Protecţia Mediului, 21.
Rezaei, R., Aghapour, A. A., Chavshin, A. R., and Bargeshadi, R. (2022a). Biodegradation of the atenolol and nitrogen removal using the sequencing batch biofilm reactor. Bioresour. Technol. Rep. 18, 101109. doi:10.1016/j.biteb.2022.101109
Rezaei, R., Aghapour, A. A., and Khorsandi, H. (2022b). Investigating the biological degradation of the drug β-blocker atenolol from wastewater using the SBR. Biodegradation. 33. doi:10.1007/s10532-022-09979-w
Salgot, M., Oron, G., Cirelli, G. L., Dalezios, N. R., Díaz, A., and Angelakis, A. N. (2016). Criteria for wastewater treatment and reuse under water scarcity. Boca Raton FL, USA: CRC Press.
Song, J., Chen, L., Chen, H., Sheng, F., Xing, D., and Li, L., (2018). Characterization and high-throughput sequencing of a trichlorophenol-dechlorinating microbial community acclimated from sewage sludge. J. Clean. Prod. 197, 306–313. doi:10.1016/j.jclepro.2018.06.061
Keywords: 2-4-6 trichlorophenol, biodegradation, biofilm, wastewater, toxic compound
Citation: Ghochlavi N, Aghapour AA and Khorsandi H (2022) Biodegradation of 2-4-6 trichlorophenol by sequencing batch reactors (SBR) equipped with a rotating biological bed and operated in an anaerobic-aerobic condition. Front. Environ. Sci. 10:1015790. doi: 10.3389/fenvs.2022.1015790
Received: 10 August 2022; Accepted: 05 October 2022;
Published: 19 October 2022.
Edited by:
Mubarak Mujawar, University of Technology Brunei, BruneiReviewed by:
Mansooreh Dehghani, Shiraz University of Medical Sciences, IranSudipti Arora, Dr. B. Lal Institute of Biotechnology, India
Copyright © 2022 Ghochlavi, Aghapour and Khorsandi. This is an open-access article distributed under the terms of the Creative Commons Attribution License (CC BY). The use, distribution or reproduction in other forums is permitted, provided the original author(s) and the copyright owner(s) are credited and that the original publication in this journal is cited, in accordance with accepted academic practice. No use, distribution or reproduction is permitted which does not comply with these terms.
*Correspondence: Ali Ahmad Aghapour, YWFhZ2hhcG91ckBnbWFpbC5jb20=