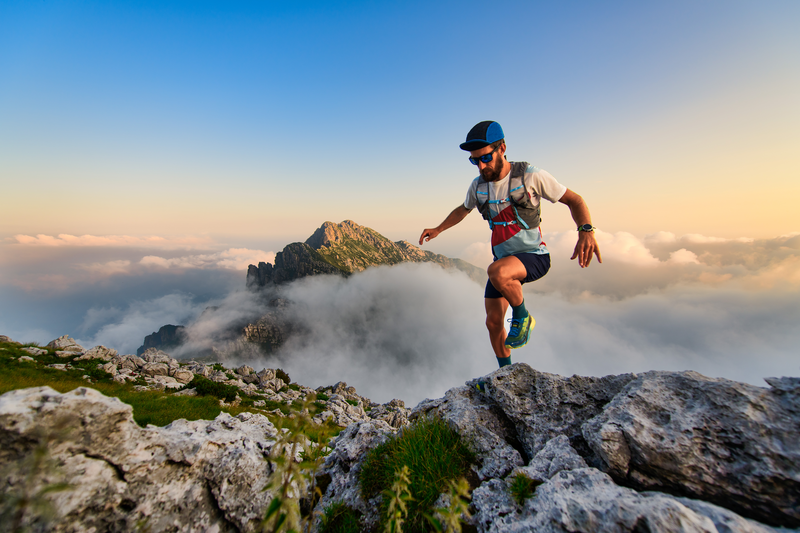
95% of researchers rate our articles as excellent or good
Learn more about the work of our research integrity team to safeguard the quality of each article we publish.
Find out more
ORIGINAL RESEARCH article
Front. Environ. Sci. , 16 January 2023
Sec. Toxicology, Pollution and the Environment
Volume 10 - 2022 | https://doi.org/10.3389/fenvs.2022.1013878
This article is part of the Research Topic Biogeochemistry of Metals in Contaminated Environments View all 9 articles
The alkaline rocks are known for enriching rare lithophilic elements, including lithium, uranium, and tin, which negatively impact aquatic life. This study offers an intensive investigation of the influence of alkaline rocks on Nile Tilapia (Oreochromis niloticus). The variation in blood profile, the induction of antioxidant enzymes, morphological erythrocyte, and histological structure have been conducted for the fish after 15 days of exposure to alkaline rocks powder with a dose of 100 μg/L. As a result, there was a pronounced decrease in blood profiles, such as platelets and white blood cell counts. There was a failure in the liver and kidney functions. Moreover, it shows an increase in superoxide dismutase (SOD) and catalase (CAT) activities as antioxidant biomarkers. Also, exposure to alkaline rocks induced DNA mutation and erythrocyte distortion. We concluded that the bulk alkaline rocks induced changes in the hemato-biochemical and antioxidant parameters of Nile tilapia. Additionally, exposure to bulk alkaline rock compounds also caused poikilocytosis and nuclear abnormalities of RBCs. This draws our attention to the seriousness of climatic changes, the erosion of rocks, and their access to water.
Alkaline rocks are characterized by the presence of feldspathoids, alkaline pyroxenes, amphiboles, or all of them combined. One of the main reasons that has attracted petrologists to conduct intensive studies of alkaline rocks is their enrichment in rare lithophilic elements, such as Be, Nb, Ta, Zr, Hf, Ga, Li, Rb, Th, U, and rare earth elements (REE). However, alkaline rocks are of minimal distribution around the world. In North America, alkaline rocks cover an area of less than 0.05% of the total area of igneous rocks. In Europe, the ratio is likely to be smaller than 1% (Harangi et al., 2003). In Egypt, alkaline rocks are of relatively limited distribution, particularly in the southeastern desert. They are represented mainly by ring complexes, some dykes, plugs, volcanic flows, and, comparatively, small alkaline granitic, syenitic bodies, or both. The detailed geology, petrology, geochemistry, and geochronology of the El Kahfa ring complex have been treated by several authors (El Ramly et al., 1969; Serencsits et al., 1981; El Ramly and Hussein 1985; Bishady et al., 2016; Hegazy et al., 2016). El Ramly et al. (1969) inferred that the El Kahfa ring complex intruded into metavolcanic and epidiorite rocks. The El Kahfa ring complex comprises an incomplete ring and a small central stock. The incomplete ring comprises inner and outer rings and consists of alkaline syenites, quartz-bearing syenites, and alkali gabbro. The central stock is formed from small bodies of volcanic rocks and mainly of alkali gabbro, which is cut by small bodies of alkaline syenite that grade into nepheline syenite. These rocks are enriched in the Lithophile Elements (LILE) (Rb and Ba) and the high-field strength element (HFSE) (Zr, Hf, Ta, and Nb) (Pfander et al., 2007).
The importance of REEs in a wide range of technologies arises from their natural availability. One of the most common sources of REE is its dispersion from indigenous rocks, which may cause water contamination. Thus, contamination may contribute to their release into surrounding ecosystems and aquatic organisms (Malhotra et al., 2020). Rock deterioration along the series of mountains of the Red Sea region can increase the exposure of terrestrial and aquatic organisms to high levels of REEs. There is a shortage in studying the effects of REEs on aquatic biota, given the limited number of reported studies on the toxicity of REEs on the aquatic environment and organisms. Hence, the impact of REE toxicity on aquatic organisms should be thoroughly discussed, considering growth inhibition, embryotoxicity, cytogenetic effects, and organ-specific toxicity. Aquatic biota was reported as a significant models for studying the toxic influence of REEs and improving their impacts on human health (Malhotra et al., 2020).
Moreover, there are some studies in which REEs have been shown to have potential cytotoxicity and genotoxicity properties (Franchi et al., 2015) on animals models, such as zebrafish (He et al., 2016) and rats (Pagano et al., 2015; Kim et al., 2017). Nile tilapia (Oreochromis niloticus) is considered a standard aquatic animal model for examining toxicity, especially in acute toxicity studies. Juveniles of tilapia are established as one of the standard models used for toxicity investigation due to their high sensitivity to low-level environmental pollutants (Lan et al., 2021). Different blood cell indices, including hemotoxic and biochemical indices, are used as significant biomarkers of the systemic response to environmental stress in fishes (Mekkawy et al., 2011; Abou Khalil et al., 2017; Sayed and Soliman 2018). These indices were selected because they are the first route for pollutants and the most sensitive tissue to detect side effects and pathology. Besides nuclear damage, apoptosis, and micronuclei abnormalities, fish erythrocyte distortion was considered in toxicological studies as a sign of pollution (Mekkawy et al., 2011; Sayed et al., 2016a; Sayed et al., 2019; Talapatra and Banerjee, 2005). Based on this point of view, this work aims to explore the effects of igneous rock powder on Nile tilapia juveniles using hematobiochemical parameters, erythrocyte alterations, and antioxidant biomarkers as endpoints.
Alkaline rocks [alkaline syenites (samples I (high Pb and U) and II (low Pb and U)], trachyte (sample III), and quartz-syenites (sample IV) were collected from the Gabal El Kahfa ring complex, which is located in the Southeastern Desert of Egypt, approximately 100 km from the Red Sea coast. It is bounded by longitudes 34°36′ to 34°40′ E and latitudes 24°04′ to 24°10′ N (Figure 1).
Major element chemical analyses of four representative rock samples of alkaline syenites and trachyte from the El Kahfa ring complex were done using the XRF technique and ICP-MS.
An X-ray diffractometer (XRD, Philips PW 2103, Netherland, CuKα = 1.54056 Å as the radiation source) was employed to analyze the crystal structures and phase compositions of the samples. Diffraction patterns were investigated within the 2θ range of 4–80°.
Infrared spectra were recorded using a Nicolet FTIR spectrophotometer (model 6700) in the wavenumber range of 4,000–400 cm−1. Measurements were carried out by pelletizing the samples with KBr.
The morphology and microstructures of the samples were evaluated using a scan electron microscope (SEM, EVO 15, ZEISS, Germany).
Noncontaminated young juveniles of the N. tilapia (O. niloticus) fish that were free of external parasites were collected from the Fish Biology and Pollution Laboratory at Assiut University, Egypt. The young juveniles with an average weight of about 3 g were kept in water tanks under conductivity of 260.3 μS/cm, pH of 7.4, and dissolved oxygen of 6.8 mg/L, 25.5°C. Moreover, fish were fed 3% of their body weight on commercial diets daily with 12:12 light as a photoperiod for 2 weeks.
The fish were divided into four groups. Each group contained six fish in each replicate (three experimental replicates). Control group was feed normal in clean water, the 2nd group exposed to 100 μg/L from alkaline syenites [sample I (high Pb and U)], 3rd group exposed to 100 μg/L from alkaline syenites [sample II (low Pb and U)], 4th group exposed to 100 μg/L from trachyte (sample III), and 5th group exposed to 100 μg/L from quartz-syenites (sample IV) for 15 days. Every day, half of the capacity of the tanks was replaced with fresh-dosed water. The dose was selected according to the toxic dose studied by Joonas et al. (2017).
At the end of the exposure, fish from each group were randomly selected and anesthetized using ice to lessen stress (Wilson et al., 2009). Then, blood samples were collected from the caudal veins into heparinized and non-heparinized tubes for hematobiochemical parameter measurements. Likewise, blood smears on clean glass slides were prepared (triplicate slides from each fish/nine slides from each group).
The counting of the red blood cells (RBCs) and total white blood cells (WBCs) was conducted according to Stevens. (1997). The microhematocrit method of Hesser. (1960) was used to detect hematocrit (HCT). Hemoglobin was measured according to Lee et al. (1999). RBC indices were calculated according to the formula suggested therein. Blood in the non-heparinized tubes was allowed to clot at 4°C. Then, the blood was separated through centrifugation at 5,000 rpm at 4°C for 20 min to isolate the serum. Biochemical parameters [e.g., glucose, albumin, total protein, globulin, creatinine, aspartic aminotransferase (AST), alanine aminotransferase (ALT), urea, and uric acid] were estimated using kits (Biodiagonstic, Egypt).
The blood smears were fixed in absolute methanol, air-dried, and stained with 6% Giemsa stain according to the method proposed by Hamed et al. (2019b). According to staining quality, slides were selected, coded, randomized, and scored by one person according to Al-Sabti and Metcalfe. (1995) and Schmid. (1975) using an Omax microscope with a 14 MP USB Digital Camera (CS-M837ZFLR-C140U, A35140U3; China).
Three fish from each group were randomly selected and anesthetized using ice to lessen stress (Wilson et al., 2009). The serum was separated to measure enzyme activity. Antioxidants kits from Biodiagnostic Company, Cairo, Egypt were used to estimate total antioxidant capacity (TAC), malondialdehyde (MDA), total peroxides (TPX), superoxide dismutase (SOD), and catalase (CAT) according to the procedure of (Nishikimi et al., 1972; Ohkawa et al., 1979; Aebi 1984; Koracevic et al., 2001; Waseem and Parvez 2013), respectively.
The homogeneity of variance was assumed for raw data. Moreover, in the absence of interactions, the pattern of variations was recorded by one-way ANOVA considering Tukey-HSD test for multiple comparisons using IBM-SPSS package version 21 (IBM-SPSS, 2012) at 0.05 significance.
The Research and Ethical Committee of the Faculty of Science at Assiut University approved the experimental design of this study.
The contents of the major elements, trace elements, and REEs of the samples are tabulated in Table 1. It was observed that silica (SiO2) and alumina (Al2O3) were the major components of all the samples, with percentages above 72.95%. Other components, such as TiO2, Fe2O3, FeO, MnO, MgO, CaO, Na2O, K2O, and P2O5, were also variable. Still, the percentages of these components were lower than 8%. Sample III had the highest silica content, at about 75.34%, while sample II contained the highest alumina content, at approximately 14.77%. Meanwhile, the sample I had the highest hematite (Fe2O3), and sample VI had the highest CaO content (3.76%). A total of 31 trace elements were found, but seven of them, including Ti, Cr, Zn, Sr, Zr-RFA, Cs, and Ba, had significant contents over 100 ppm. The trace element with the highest content was Ti, with 6.65 ppm found in sample I. Sample II had the highest Cs content (987.8 ppm), while sample III had the highest Cr content (231 ppm). Furthermore, 14 REEs were also detected in the samples, but only three, including La, Pr, and Nd, had significant contents over 40 ppm. Sample II had the highest La (193 ppm) and Pr (83.3 ppm) contents, while sample I had the highest Nd content (49.7 ppm).
The XRD patterns of the samples are shown in Figure 2. All samples had reflections at 2ϴ values of 20.7°, 26.4°, 36.4°, 39.3°, 40.1°, 42.3°, 50.1°, 54.8°, 59.8°, 67.9° and 75.6°, indicating the presence of quartz (COD card no 00-901-1493) as the main component, which was in agreement with that observed from the XRF data. At the same time, the peaks observed at 2ϴ of 13.7°, 21.9°, 23.4°, 24.1°, 25.4°, 27.6°, and 30.1° were characteristics of albite (COD card no. 00-900-9663). It was noted that sample III had the highest peak intensities, reflecting its high crystallinity. In contrast, sample IV had the lowest intensities of the XRD peaks, indicating that it had a low crystallinity. The average crystallite sizes were calculated using the Scherrer equation (Said et al., 2014) and were found to be 18.7 nm, 25.3 nm, 19.5 nm, and 15.8 nm for samples I, II, III, and IV, respectively.
The FTIR spectra of the samples are shown in Figure 3. All had similar FTIR bands, except for sample IV, which had some slightly different bands. All samples had several bands at 3435 cm−1, 1140 cm−1, 1040 cm−1, 1051 cm−1, 1021 cm−1, 777 cm−1, 725 cm−1, 694 cm−1, 648 cm−1, 586 cm−1, 532 cm−1, 463 cm−1, and 425 cm−1. The band located at 3435 cm−1 can be assigned to the stretching vibration of water molecules (Lyalina et al., 2019). The stretching vibrations of Si-O were detected at 1140 cm−1 and 1096 cm−1, while the bands observed at 1036 cm−1 and 1015 cm−1 were the stretching vibrations of Al-O (Wang et al., 2009). The bands found at 777 cm−1, 694 cm−1, and 648 cm−1 could be attributed to the stretching vibration of Si-Si, and the bending vibration of O-Si-O, respectively (Xu and van Deventer, 2003). The asymmetric and symmetric stretching vibrations of M-O (M = Al, Si) were observed at 725 cm−1 and 425 cm−1, respectively (Ma et al., 2015). The band located at 586 cm−1 could be due to the O-Si-(Al)-O bending vibration (Ramasamy et al., 2009). Meanwhile, the stretching and bending modes of the Fe-O bond in hematite were observed at 533 cm−1 and 463 cm−1, respectively (Tadic et al., 2019). The FTIR spectrum of sample VI had two additional bands at 3546 cm−1 and 1428 cm−1, corresponding to the stretching vibrations of OH groups (Lyalina et al., 2019) and the symmetric stretching vibration of unidentate carbonate (Imtiaz et al., 2013). The bands observed in samples III and IV were sharper than in samples I and II. This happened because samples III and IV had a higher SiO2 content found in the XRF data (Table 1).
The SEM micrographs of samples II and IV are shown in Figure 4. The two samples had almost the same morphology. A mixture of blocky and flakey structures was observed, with an average size of about 1.2 μm and 0.6 μm, respectively. Many fractures and pores were also observed.
The levels of all hematological parameters of O. niloticus with 15 days of alkaline rock exposure are recorded in Table 2. As mentioned in Table 2, the specimens exposed to alkaline rock had a pronounced decrease in their platelets. The count levels of WBCs, RBCs, blood hemoglobin concentration (Hb), and hematocrit (Ht) were significantly different from those in the control group (p < 0.05). Mean corpuscular volume (MCV), mean corpuscular hemoglobin (MCH), mean corpuscular hemoglobin concentration (MCHC), lymphocytes, and monocytes had a significant increase in some treatment groups compared to the control group (p < 0.05). On the other hand, there were no significant variations in neutrophil cells in any of the groups (Table 2). Alterations in erythrocytes had a significant (p < 0.05) increase in rock-exposed groups.
TABLE 2. Effect of alkaline rock powder exposure for 15 days on the hematological parameters of juveniles of Tilapia (Oreochromis niloticus).
The levels of kidney function (creatinine), liver function (AST, ALT, and ALP), and other biochemical parameters (e.g., glucose, total protein, albumin, globulin, and A/G ratio) of O. niloticus specimens exposed to alkaline rocks for 15 days are shown in Table 3. In comparison to the control group, the levels of these parameters on the rocks-exposed fish had a significant increase (p < 0.05) in all treatment groups in a dose-dependent manner (Table 3).
TABLE 3. The effects of alkaline rock powder exposure for 15 days on the biochemical parameters of juveniles of Tilapia (Oreochromis niloticus).
The activities of SOD, CAT, total peroxides (TPX), malondialdehyde (MDA), total antioxidant capacity (TAC), and oxidative stress index (OSI) of O. niloticus specimens exposed to alkaline rocks for 15 days are shown in Table 4. Compared to the control group, the activities of MDA, SOD, and CAT of the rocks-exposed fish exhibited a significant (p < 0.05) increase in all treatment groups in a dose-dependent manner. The activities of TAC, TPX, and OSI had a significant increase (p < 0.05) only in a high concentration of two samples (100 μg/L) (Table 4).
TABLE 4. Effects of alkaline rock powder exposure for 15 days on the antioxidant parameters of the juveniles of Tilapia (Oreochromis niloticus).
The erythrocytes of specimens in the control group had a well-shaped and normal size with their central nucleus, as shown in Figure 5A. However, the fish exposed to alkaline rock at a dose of 100 μg/L for 15 days had blood smears with poikilocytosis of the erythrocytes, as indicated in Figures 5B–F. This is confirmed by the presence of spinocyte, crenated, acanthocyte, kidney-shaped, and sickle cells. We also observed changes in RBCs containing tear-drop cells, lobed-nuclei cells, eccentric-nuclei cells, microcytes, and schistocytes.
FIGURE 5. Represented blood smears from juvenile Tilapia, Oreochromis niloticus. (A) Control; (B–F) exposed to 100 μg/L alkaline rocks samples for 15 days; (RBCs) red blood cells; (Ac) acanthocytes (Cr) crenated cells; (Sk) Sickle cells; (Tr) teardrop--like cells; (Shc) Schistocyte; (Amc) Amoeboid cells, H and E stain, scale bar: 20 μm.
Rare earth elements can be reused and recycled through various technologies to minimize environmental impacts; however, there is insufficient data about their biological, bioaccumulation, and health effects (Romero-Freire et al., 2019). With rare earth elements’ increasing use, environmental toxicity is becoming a concern, which may further harm human health. REEs’ toxic effects are not fully investigated due to a lack of detailed reports (Malhotra et al., 2020). Trace elements and REEs usually sink into the aquatic environment as dissolved phases. They can be absorbed in sediments as well as aquatic plants and animals, resulting in multiple intake routes of inhalation, occupational exposure, and iatrogenic exposure. Research on the toxicity, mobility, and biomagnification of REEs in water bodies can be conducted using aquatic biota, particularly fish. Among different indicators, bioindicators, such as the hematological and biochemical parameters of fish, can be used to justify aspects of water, including its quality (Saravanan et al., 2011). The decrease in the RBCs, Hb, HCT, and PL values of tilapia fish exposed to bulk alkaline rocks we observed can be attributed to the suppression of erythropoiesis (the progress of development by erythropoietin in the kidney), hemosynthesis, osmoregulatory dysfunction, or accession of the erythrocyte destruction rate of the hematopoietic organ, which may be caused by pollutants action (Oluah et al., 2020). Moreover, the decrease in PL values can be ascribed to the acceleration in RBC hemolysis and inhibition of erythropoiesis to the (Woryi et al., 2020). Furthermore, the significant reduction in RBCs resulting from the existence of many types of reactive oxygen species (ROS) (Dar and Borana 2014). These damaged RBCs may cause hypoxia, a reduction in HCT values, and Hb contents of the rock-exposed fishes (Abdel-Khalek et al., 2016). Such hypoxia suppresses aerobic glycolysis, which reduces the energy required for Hb production (Joshi et al., 2002). Even more, the detected lower values of HCT can be attributed to hemodilution and disrupted osmoregulation due to gill destruction (Kori-Siakpere et al., 2008).
The results of this study can be compared with other reported effects on fish under different stressors. For instance, the exposure of Nile tilapia to CuSO4 and CuO nanoparticles can negatively influence hematological parameters (Soliman et al., 2021). Also, exposure to microplastics affects early juveniles blood parameters (Hamed et al., 2019b; Hamed et al., 2020). Alkaladi et al. (2015) and his research team reported a drop in the total count of WBCs of O. niloticus as a result of exposure to the of bulk alkaline, which may infect the lymphoid tissues of fish (Mukherjee and Sinha 1993). Furthermore, Nile tilapia and catfish exposure to nickel can reduce WBCs and affect the immune system (Ololade and Oginni 2010). Similarly, bulk alkaline rocks can decrease the WBC count from the accumulation of contaminates in the tissues.
Moreover, exposure to bulk alkaline rocks significantly influences other biochemical parameters, including liver functions, creatinine, glucose, total protein, albumin, globulin, and albumin to globulin (A/G) ratio depending on the dose rate. Higher enzyme levels are detected from the blood samples test, such as lactic dehydrogenase, AST, ALT, creatine kinase, and alkaline phosphatase, which correspond to damage to cell membranes (Banaee 2013). Such results are in agreement with other reported findings (Gill et al., 1992; Kazlauskienė and Stasiunaitė 1999; Monteiro et al., 2005; Heydarnejad et al., 2013; Pretto et al., 2014; Abdel-Khalek et al., 2015; Hamed et al., 2019a; Hamed et al., 2019b; Osman et al., 2019; Sayed et al., 2019; Soliman et al., 2019; Sayed et al., 2021a; Sayed et al., 2021b; Soliman et al., 2021; Hamed et al., 2022; Sayed et al., 2022). The variation in glucose levels is related to the glycogenolysis and gluconeogenesis processes occurring in the liver and kidney (Oner et al., 2008). Javed et al. (2017) showed that fish exposed to copper contamination might need to increase their protein levels to induce their immune system and tissue repair. In agreement with this work, the variation in the biochemical parameters has also been observed in goby (Pomatoschistus microps) after exposure to nickel contaminations (dos Santos Norberto 2014). However, the glomerular filtration rate and kidney dysfunction biomarker can be indicated by detecting creatinine and uric acid values (Banaee 2013; Abdel-Khalek et al., 2016).
Based on the results found by Hook et al. (2014), different aquatic environmental stressors are detectable using antioxidant biomarkers. In this study, there were significant increases in SOD, CAT, TPX, MDA, TAC, and OSI. Inflation in SOD and CAT after exposure to bulk alkaline rocks corresponds to the generation of ROS in fish. Such high SOD and CAT activities indicate that the liver is more likely to suffer from oxidative stress than other organs (Atli et al., 2006). This result is in coincidence with the reports published by Safari and his team (Safari 2016) that show that exposure to heavy metals can induce stress detection from the expression of genes encoding SOD and CAT to the detoxification of ROS (Rastgoo and Alemzadeh 2011). Similar results were detected in different types of fish, such as sturgeon, murrel, and tilapia (Atli and Canli 2007; Dabas et al., 2012; Safari 2016). OSI is an indicator that can be used to investigate the interaction between different free radicals and the antioxidant system (Sayed et al., 2016a). Therefore, OSI has been investigated in different animal models, such as arsenite toxicity in goldfish (Bagnyukova et al., 2007) and methyltestosterone effects in N. Tilapia (O. niloticus) (Sayed et al., 2016a). Our result was suitable in compliance with the results found by Hamed et al. (2020) that the disclosure of microplastics may induce antioxidant damage in N. Tilapia (O. niloticus). The examined behaviors of antioxidant enzyme activity were in agreement with the results reported previously in studies involving different species exposed to heavy metal (Pedrajas et al., 1995; Paris-Palacios et al., 2000; Vieira et al., 2009; Wang et al., 2009; Tang et al., 2013; Hamed et al., 2019a; Sayed et al., 2019; Soliman et al., 2019; Sayed et al., 2021a; Sayed et al., 2021b; Soliman et al., 2021; Sayed et al., 2022). It has been reported in another study that the most significant finding was that the REE accumulated in zebra tissue after REE exposure was highly correlated to cytochrome c oxidase (CO1) and superoxide dismutase (SOD) were increased, while gene expressions of catalase (CAT) and glutathione-S-transferase (GST) were decreased (Hanana et al., 2017).
We investigated morphological erythrocyte amendment and nuclear distortions directed by the bulk alkaline rocks (Figure 5). As shown in Figure 5, there was an alternation in erythrocytes in the rock-exposed groups compared to the control. Comparable observations were recorded under the exposure of various aquatic species to other contaminants, such as copper sulfate and copper oxide nanoparticles (Soliman et al., 2021) and lead nitrate (Hamed et al., 2019b). Also, the rise in the morphological distortions of erythrocytes in catfish under disclosure to UVA was investigated (Osman et al., 2019). Even more, exposure to heavy can cause damage and mutation in DNA, reflecting in the quality of micronucleus and other alterations in erythrocytes, as previously studied (Osman et al., 2019; Soliman et al., 2019; Sayed et al., 2021a; Sayed et al., 2021b; Hamed et al., 2021; Soliman et al., 2021; Hamed et al., 2022). In another study conducted by Trifuoggi et al. (2017), a group of chlorides of 7 REEs were tested on two sea urchin species showed developmental defects and cytogenetic anomalies. Also, Kurvet et al. (2017) reported in his research that all studied soluble REEs were toxic to bacteria and protozoa. The mecahnism of the cell damge can expalined as During the action, REE might compete with Ca2+ for binding sites in biological systems, inhibiting calcium channels in cell membranes and affecting the work of cells and tissues (Dave and Xiu 1991).
In conclusion, the bulk alkaline rocks induced changes in biochemical, antioxidant, and hematological parameters in Nile tilapia. Exposure to the bulk alkaline rock compounds also caused poikilocytosis and nuclear abnormalities of RBCs. This draws our attention to the seriousness of climatic changes, the potential erosion of rocks, their access to water, and consequent effects on aquatic biota.
The raw data supporting the conclusion of this article will be made available by the authors, without undue reservation.
The animal study was reviewed and approved by Assiut University.
Experimental design: ES and AS. Experiment and analysis: ES, MH, AE, MA, and AS. Data interpretation: ES, MH, AE MA, and AS. Writing and revision: ES, MH, AE, MA, and AS. All authors read and approved the final manuscript.
The authors declare that the research was conducted in the absence of any commercial or financial relationships that could be construed as a potential conflict of interest.
All claims expressed in this article are solely those of the authors and do not necessarily represent those of their affiliated organizations, or those of the publisher, the editors and the reviewers. Any product that may be evaluated in this article, or claim that may be made by its manufacturer, is not guaranteed or endorsed by the publisher.
Abdel-Khalek, A. A., Badran, S. R., and Marie, M. A. S. (2016). Toxicity evaluation of copper oxide bulk and nanoparticles in Nile tilapia, Oreochromis niloticus, using hematological, bioaccumulation and histological biomarkers. Fish. Physiol. Biochem. 42 (4), 1225–1236. doi:10.1007/s10695-016-0212-8
Abdel-Khalek, A. A., Kadry, M. A., Badran, S. R., and Marie, M-A. S. (2015). Comparative toxicity of copper oxide bulk and nano particles in nile tilapia; Oreochromis niloticus: Biochemical and oxidative stress. J. Basic & Appl. Zoology 72, 43–57. doi:10.1016/j.jobaz.2015.04.001
Abou Khalil, N. S., Abd-Elkareem, M., and Sayed, A. H. (2017). Nigella sativa seed protects against 4-nonylphenol-induced haematotoxicity in Clarias gariepinus (Burchell, 1822): Oxidant/antioxidant rebalance. Aquac. Nutr. 23 (6), 1467–1474. doi:10.1111/anu.12522
Al-Sabti, K., and Metcalfe, C. D. (1995). Fish micronuclei for assessing genotoxicity in water. Mutat. Research/Genetic Toxicol. 343 (2–3), 121–135. doi:10.1016/0165-1218(95)90078-0
Alkaladi, A., El-Deen, N. A. N., Afifi, M., and Zinadah, O. A. A. (2015). Hematological and biochemical investigations on the effect of vitamin E and C on Oreochromis niloticus exposed to zinc oxide nanoparticles. Saudi J. Biol. Sci. 22 (5), 556–563. doi:10.1016/j.sjbs.2015.02.012
Atli, G., Alptekin, Ö., Tükel, S., and Canli, M. (2006). Response of catalase activity to Ag+, Cd2+, Cr6+, Cu2+ and Zn2+ in five tissues of freshwater fish Oreochromis niloticus. Comp. Biochem. Physiology Part C Toxicol. Pharmacol. 143 (2), 218–224. doi:10.1016/j.cbpc.2006.02.003
Atli, G., and Canli, M. (2007). Enzymatic responses to metal exposures in a freshwater fish Oreochromis niloticus. Comp. Biochem. Physiology Part C Toxicol. Pharmacol. 145 (2), 282–287. doi:10.1016/j.cbpc.2006.12.012
Bagnyukova, T. V., Luzhna, L. I., Pogribny, I. P., and Lushchak, V. I. (2007). Oxidative stress and antioxidant defenses in goldfish liver in response to short-term exposure to arsenite. Environ. Mol. Mutagen. 48 (8), 658–665. doi:10.1002/em.20328
Banaee, M. (2013). “Physiological dysfunction in fish after insecticides exposure,” in Insecticides. Editor T. Stanislav (Rijeka: IntechOpen). Ch. 4.
Bishady, A., El-Sherif, A., and Darwish, M. (2016). Petrological and geochemical constraints on the evolution of el-kahfa alkaline ring complex, south eastern Desert, Egypt. J. Geol. Resour. Eng. 6, 283–304.
Dabas, A., Nagpure, N., Kumar, R., Kushwaha, B., Kumar, P., and Lakra, W. (2012). Assessment of tissue-specific effect of cadmium on antioxidant defense system and lipid peroxidation in freshwater murrel, Channa punctatus. Fish. Physiol. Biochem. 38 (2), 469–482. doi:10.1007/s10695-011-9527-7
Dar, Z., and Borana, K. (2014). Effect of sub lethal doses of copper sulphate on certain haematological parameters of common carp, Cyprinus carpio. Int. J. Recent Sci. Res. 5 (2), 332–335.
Dave, G., and Xiu, R. (1991). Toxicity of mercury, copper, nickel, lead, and cobalt to embryos and larvae of zebrafish, Brachydanio rerio. Arch. Environ. Contam. Toxicol. 21 (1), 126–134. doi:10.1007/bf01055567
dos Santos Norberto, R. S. (2014). Toxic effects of nickel alone and in combination with microplastics on early juveniles of the common goby (Pomatoschistus microps). Porto, Portugal: FCT, University of Porto.
El Ramly, M., Budanov, V., Armanious, L., and Dereniuk, N. (1969). The three ring complexes of gabal El Kahfa, GabalNigrub El fogani and gabal El naga, south eastern Desert of Egypt. Geol. Surv. Egypt 52, 39.
El Ramly, M., and Hussein, A. (1985). The ring complexes of the Eastern Desert of Egypt. J. Afr. Earth Sci. 3 (1-2), 77–82. doi:10.1016/0899-5362(85)90024-7
Franchi, L. P., Manshian, B. B., de Souza, T. A., Soenen, S. J., Matsubara, E. Y., Rosolen, J. M., et al. (2015). Cyto-and genotoxic effects of metallic nanoparticles in untransformed human fibroblast. Toxicol. Vitro 29 (7), 1319–1331. doi:10.1016/j.tiv.2015.05.010
Gill, T. S., Tewari, H., and Pande, J. (1992). Short-and long-term effects of copper on the rosy barb (Puntius conchonius Ham.). Ecotoxicol. Environ. Saf. 23 (3), 294–306. doi:10.1016/0147-6513(92)90079-i
Hamed, M., Monteiro, C. E., and Sayed, A. E-D. H. (2022). Investigation of the impact caused by different sizes of polyethylene plastics (nano, micro, and macro) in common carp juveniles, Cyprinus carpio L., using multi-biomarkers. Sci. Total Environ. 803, 149921. doi:10.1016/j.scitotenv.2021.149921
Hamed, M., Osman, A. G. M., Badrey, A. E. A., Soliman, H. A. M., and Sayed, A. E-D. H. (2021). Microplastics-induced eryptosis and poikilocytosis in early-juvenile nile Tilapia (Oreochromis niloticus). Front. Physiol. 12, 742922. doi:10.3389/fphys.2021.742922
Hamed, M., Soliman, H. A. M., Osman, A. G. M., and Sayed, A. E-D. H. (2020). Antioxidants and molecular damage in Nile Tilapia (Oreochromis niloticus) after exposure to microplastics. Environ. Sci. Pollut. Res. 27 (13), 14581–14588. doi:10.1007/s11356-020-07898-y
Hamed, M., Soliman, H. A. M., Osman, A. G. M., and Sayed, A. E-D. H. (2019a). Assessment the effect of exposure to microplastics in nile Tilapia (Oreochromis niloticus) early juvenile: I. Blood biomarkers. Chemosphere 228, 345–350. doi:10.1016/j.chemosphere.2019.04.153
Hamed, M., Soliman, H. A. M., and Sayed, A. E-D. H. (2019b). Ameliorative effect of Spirulina platensis against lead nitrate–induced cytotoxicity and genotoxicity in catfish Clarias gariepinus. Environ. Sci. Pollut. Res. 26 (20), 20610–20618. doi:10.1007/s11356-019-05319-3
Hanana, H., Turcotte, P., André, C., Gagnon, C., and Gagné, F. (2017). Comparative study of the effects of gadolinium chloride and gadolinium – based magnetic resonance imaging contrast agent on freshwater mussel, Dreissena polymorpha. Chemosphere 181, 197–207. doi:10.1016/j.chemosphere.2017.04.073
Harangi, S., Tonarini, S., Vaselli, O., and Piero, M. (2003). Geochemistry and petrogenesis of Early Cretaceous alkaline igneous rocks in Central Europe: Implications for a long-lived EAR-type mantle component beneath Europe. Acta Geol. Hung. 46, 77–94. doi:10.1556/ageol.46.2003.1.6
He, A., Kwatra, S. G., Zampella, J. G., and Loss, M. J. (2016). Nephrogenic systemic fibrosis: Fibrotic plaques and contracture following exposure to gadolinium-based contrast media. BMJ Case Rep. 2016, bcr2016214927. doi:10.1136/bcr-2016-214927
Hegazy, H., Arzamastsev, A., and Saad, E. (2016). Geology, petrography and geochemistry of el-kahfa ring complex, south eastern Desert. Int. J. Geophys. Geochem. 3, 25–37.
Hesser, E. F. (1960). Methods for routine fish hematology. Progressive Fish-Culturist 22, 164–171. doi:10.1577/1548-8659(1960)22[164:mfrfh]2.0.co;2
Heydarnejad, M. S., Khosravian-hemami, M., Nematollahi, A., and Rahnama, S. (2013). Effects of copper at sublethal concentrations on growth and biochemical parameters in rainbow trout (Oncorhynchus mykiss). Int. Rev. Hydrobiol. 98 (2), 71–79. doi:10.1002/iroh.201201443
Hook, S. E., Gallagher, E. P., and Batley, G. E. (2014). The role of biomarkers in the assessment of aquatic ecosystem health. Integr. Environ. Assess. Manag. 10 (3), 327–341. doi:10.1002/ieam.1530
Imtiaz, A., Farrukh, M. A., Khaleeq-ur-rahman, R., and Adnan, R. (2013). Micelle-assisted synthesis of Al2O3⋅CaO nanocatalyst: Optical properties and their applications in photodegradation of 2,4,6-trinitrophenol. Sci. World J. 2013, 641420. doi:10.1155/2013/641420
Javed, M., Ahmad, M., Usmani, N., and Ahmad, M. (2017). Multiple biomarker responses (serum biochemistry, oxidative stress, genotoxicity and histopathology) in Channa punctatus exposed to heavy metal loaded waste water. Sci. Rep. 7 (1), 1675–1711. doi:10.1038/s41598-017-01749-6
Joonas, E., Aruoja, V., Olli, K., Syvertsen-Wiig, G., Vija, H., and Kahru, A. (2017). Potency of (doped) rare Earth oxide particles and their constituent metals to inhibit algal growth and induce direct toxic effects. Sci. Total Environ. 593-594, 478–486. doi:10.1016/j.scitotenv.2017.03.184
Joshi, P., Harish, D., and Bose, M. (2002). Effect of lindane and malathion exposure to certain blood parameters in a fresh water teleost fish Clarias batrachus. Pollut. Res. 21 (1), 55–57.
Kazlauskienė, N., and Stasiūnaitė, P. (1999). The lethal and sublethal effect of heavy metal mixture on rainbow trout (Oncorhynchus mykiss) in its early stages of development. Acta Zool. Litu. 9 (2), 47–55. doi:10.1080/13921657.1999.10512287
Kim, Y-S., Lim, C-H., Shin, S-H., and Kim, J-C. (2017). Twenty-eight-day repeated inhalation toxicity study of nano-sized neodymium oxide in male Sprague-Dawley rats. Toxicol. Res. 33 (3), 239–253. doi:10.5487/tr.2017.33.3.239
Koracevic, D., Koracevic, G., Djordjevic, V., Andrejevic, S., and Cosic, V. (2001). Method for the measurement of antioxidant activity in human fluids. J. Clin. pathology 54 (5), 356–361. doi:10.1136/jcp.54.5.356
Kori-Siakpere, O., Ubogu, E. O., Kurvet, I., Juganson, K., Vija, H., Sihtmäe, M., et al. (2008). Sublethal haematological effects of zinc on the freshwater fish, Heteroclarias sp.(Osteichthyes: Clariidae). Mater. Toxic. Nine (Doped) Rare Earth Metal Oxides Respective Individ. Metals Aquatic Microorg. Vibrio fischeri Tetrahymena thermophila 710 (127), 754. doi:10.3390/ma10070754
Kurvet, I., Juganson, K., Vija, H., Sihtmäe, M., Blinova, I., Syvertsen-Wiig, G., et al. (2017). Toxicity of nine (Doped) rare earth metal oxides and respective individual metals to aquatic microorganisms Vibrio fischeri and Tetrahymena thermophila. Materials (Basel) 10 (7), 754. doi:10.3390/ma10070754
Lan, S. M., Briggs, T-M. D., Obanya, H. E., Amaeze, N. H., and Otitoloju, A. A. (2021). Toxicity Evaluation of Pharmaceutical Wastewater to the Nile Tilapia (<i&gt;Oreochromis niloticus&lt;/i&gt;). J. Environ. Prot. (Irvine, Calif. 12 (04), 296–309. doi:10.4236/jep.2021.124019
Lee, G. R., Foerster, J., Lukens, J., Paraskevas, F., Greer, J. P., and Rodgers, G. M. (1999). Wintrobe's clinical hematology 10th. Bethseda, Maryland: Lippincort Williams and Wilkins.
Lyalina, L. M., Kadyrova, G. I., Selivanova, E. A., Zolotarev Jr., A. A., Savchenko, Y. E., and Panikorovskii, T. L. (2019). On Composition of Meliphanite from Nepheline Syenite Pegmatite of the Sakharjok Massif, Kola Peninsula. Geol. Ore Depos. 61, 671–679. doi:10.1134/S1075701519070092
Ma, X., Yang, J., Ma, H., Liu, C., and Zhang, P. (2015). Synthesis and characterization of analcime using quartz syenite powder by alkali-hydrothermal treatment. Microporous Mesoporous Mater. 201, 134–140. doi:10.1016/j.micromeso.2014.09.019
Malhotra, N., Hsu, H. S., Liang, S. T., Roldan, M. J. M., Lee, J. S., Ger, T. R., et al. (2020). An updated review of toxicity effect of the rare Earth elements (REEs) on aquatic organisms. Animals 10 (9), 1663.
Mekkawy, I. A., Mahmoud, U. M., and Sayed, A. H. (2011). Effects of 4-nonylphenol on blood cells of the African catfish Clarias gariepinus (Burchell, 1822). Tissue Cell. 43 (4), 223–229. doi:10.1016/j.tice.2011.03.006
Monteiro, S. M., Mancera, J. M., Fontaínhas-Fernandes, A., and Sousa, M. (2005). Copper induced alterations of biochemical parameters in the gill and plasma of Oreochromis niloticus. Comp. Biochem. Physiology Part C Toxicol. Pharmacol. 141 (4), 375–383. doi:10.1016/j.cbpc.2005.08.002
Mukherjee, J., and Sinha, G. (1993). Cadmium toxicity on haematological and biochemical aspects in an Indian freshwater major carp, Labeo rohita(Hamilton). J. Freshw. Biol. 5 (3), 245–251.
Nishikimi, M., Appaji Rao, N., and Yagi, K. (1972). The occurrence of superoxide anion in the reaction of reduced phenazine methosulfate and molecular oxygen. Biochem. Biophysical Res. Commun. 46 (2), 849–854. doi:10.1016/s0006-291x(72)80218-3
Ohkawa, H., Ohishi, N., and Yagi, K. (1979). Assay for lipid peroxides in animal tissues by thiobarbituric acid reaction. Anal. Biochem. 95 (2), 351–358. doi:10.1016/0003-2697(79)90738-3
Ololade, I., and Oginni, O. (2010). Toxic stress and hematological effects of nickel on African catfish, Clarias gariepinus, fingerlings. J. Environ. Chem. Ecotoxicol. 2 (2), 014–019.
Oluah, N. S., Aguzie, I. O., Ekechukwu, N. E., Madu, J. C., Ngene, C. I., and Oluah, C. (2020) Hematological and immunological responses in the African catfish Clarias gairepinus exposed to sublethal concentrations of herbicide Ronstar®. Ecotoxicol. Environ. Saf. 201(110824): 110824, doi:10.1016/j.ecoenv.2020.110824
Öner, M., Atli, G., and Canli, M. (2008). Changes in serum biochemical parameters of freshwater fish Oreochromis niloticus following prolonged metal (Ag, Cd, Cr, Cu, Zn) exposures. Environ. Toxicol. Chem. 27 (2), 360–366. doi:10.1897/07-281r.1
Osman, A., Hamed, M., and Sayed, A. (2019). Protective role of Spirulina platensis against UVA-induced haemato-biochemical and cellular alterations in Clarias gariepinus. J. Photochem. Photobiol. B Biol. 191, 59–64. doi:10.1016/j.jphotobiol.2018.12.013
Pagano, G., Aliberti, F., Guida, M., Oral, R., Siciliano, A., Trifuoggi, M., et al. (2015). Rare Earth elements in human and animal health: State of art and research priorities. Environ. Res. 142, 215–220. doi:10.1016/j.envres.2015.06.039
Paris-Palacios, S., Biagianti-Risbourg, S., and Vernet, G. (2000). Biochemical and (ultra) structural hepatic perturbations of Brachydanio rerio (Teleostei, Cyprinidae) exposed to two sublethal concentrations of copper sulfate. Aquat. Toxicol. 50 (1-2), 109–124. doi:10.1016/s0166-445x(99)00090-9
Pedrajas, J., Peinado, J., and Lopez-Barea, J. (1995). Oxidative stress in fish exposed to model xenobiotics. Oxidatively modified forms of Cu, Zn-superoxide dismutase as potential biomarkers. Chemico-Biological Interact. 98 (3), 267–282. doi:10.1016/0009-2797(95)03651-2
Pfänder, J., Münker, C., Stracke, A., and Mezger, K. (2007). Nb/Ta and Zr/Hf in ocean island basalts — implications for crust–mantle differentiation and the fate of Niobium. Earth Planet. Sci. Lett. 254, 158–172. doi:10.1016/j.epsl.2006.11.027
Pretto, A., Loro, V. L., Silva, V. M. M., Salbego, J., de Menezes, C. C., Souza, CdF., et al. (2014). Exposure to sublethal concentrations of copper changes biochemistry parameters in silver catfish, Rhamdia quelen, Quoy & Gaimard. Bull. Environ. Contam. Toxicol. 92 (4), 399–403. doi:10.1007/s00128-014-1215-8
Ramasamy, V., Rajkumar, P., and Ponnusamy, V. (2009). Depth wise analysis of recently excavated Vellar river sediments through FTIR and XRD studies. Indian J. Phys. 83, 1295–1308. doi:10.1007/s12648-009-0110-3
Rastgoo, L., and Alemzadeh, A. (2011). Biochemical responses of Gouan ('Aeluropus littoralis') to heavy metals stress. Aust. J. Crop Sci. 5 (4), 375–383.
Romero-Freire, A., Joonas, E., Muna, M., Cossu-Leguille, C., Vignati, D. A. L., and Giamberini, L. (2019). Assessment of the toxic effects of mixtures of three lanthanides (Ce, Gd, Lu) to aquatic biota. Sci. Total Environ. 661, 276–284. doi:10.1016/j.scitotenv.2019.01.155
Safari, R. (2016). Toxic effects of Cadmium on antioxidant defense systems and lipid peroxidation in Acipenser persicus (Borodin, 1897). Int. J. aquatic Biol. 3 (6), 425–432.
Said, A. E.-A. A., Abd El-Wahab, M. M., and El-Aal, M. A. (2014). The catalytic performance of sulfated zirconia in the dehydration of methanol to dimethyl ether. J. Mol. Catal. A Chem. 394, 40–47. doi:10.1016/j.molcata.2014.06.041
Saravanan, M., Kumar, K. P., and Ramesh, M. (2011). Haematological and biochemical responses of freshwater teleost fish Cyprinus carpio (Actinopterygii: Cypriniformes) during acute and chronic sublethal exposure to lindane. Pesticide Biochem. physiology 100 (3), 206–211. doi:10.1016/j.pestbp.2011.04.002
Sayed, A., Khalil, N. A., Sayed, A. E-D. H., AbdAllah, E. A., and Hamed, M. (2016a). Oxidative stress induction in monosex nile Tilapia (Oreochromis niloticus, linnaeus, 1758): A field study on the side effects of methyltestosterone) hepato-nephrotoxicity in late juvenile of Oreochromis niloticus exposed to gibberellic acid: Ameliorative effect of spirulina platensis. J Aquac Res Dev. Biochem. physiology 7167 (3), 104600. doi:10.1016/j.pestbp.2020.104600
Sayed, A. E-D. H., Hamed, M., Badrey, A. E. A., and Soliman, H. A. M. (2021a). Bioremediation of hemotoxic and oxidative stress induced by polyethylene microplastic in Clarias gariepinus using lycopene, citric acid, and chlorella. Comp. Biochem. Physiology Part C Toxicol. Pharmacol. 250, 109189. doi:10.1016/j.cbpc.2021.109189
Sayed, A. E-D. H., Hamed, M., Soliman, H. A. M., and Authman, M. M. N. (2022). The protective role of lycopene against toxic effects induced by the herbicide Harness® and its active ingredient acetochlor on the African catfish Clarias gariepinus (Burchell, 1822). Environ. Sci. Pollut. Res. 29 (10), 14561–14574. doi:10.1007/s11356-021-16518-2
Sayed, A. E-D. H., Hamed, M., and Soliman, H. A. M. (2021b). Spirulina platensis alleviated the hemotoxicity, oxidative damage and histopathological alterations of hydroxychloroquine in catfish (Clarias gariepinus). Front. Physiol. 12, 683669. doi:10.3389/fphys.2021.683669
Sayed, A. H., Mahmoud, U. M., and Mekkawy, I. A. (2016b). Erythrocytes alterations of monosex tilapia (Oreochromis niloticus, Linnaeus, 1758) produced using methyltestosterone. Egypt. J. Aquatic Res. 42 (1), 83–90. doi:10.1016/j.ejar.2015.10.004
Sayed, A. H., Soliman, H. A. M., and Mitani, H. (2019). UVA-induced neurotoxicity in Japanese medaka (Oryzias latipes). Photochem. Photobiol. Sci. 18 (1), 71–79. doi:10.1039/c8pp00169c
Sayed, A. H., and Soliman, H. A. M. (2018). Modulatory effects of green tea extract against the hepatotoxic effects of 4-nonylphenol in catfish (Clarias gariepinus). Ecotoxicol. Environ. Saf. 149, 159–165. doi:10.1016/j.ecoenv.2017.11.007
Schmidt, W. (1975). The micronucleus test. Mutat. Research/Environmental Mutagen. Relat. Subj. 31, 9–15. doi:10.1016/0165-1161(75)90058-8
Serencsits, C. M., Faul, H., Foland, K., Hussein, A., and Lutz, T. (1981). Alkaline ring complexes in Egypt: Their ages and relationship in time. J. Geophys. Res. 86 (B4), 3009–3013. doi:10.1029/jb086ib04p03009
Soliman, H. A. M., Hamed, M., Lee, J-S., and Sayed, A. E-D. H. (2019). Protective effects of a novel pyrazolecarboxamide derivative against lead nitrate induced oxidative stress and DNA damage in Clarias gariepinus. Environ. Pollut. 247, 678–684. doi:10.1016/j.envpol.2019.01.074
Soliman, H. A. M., Hamed, M., and Sayed, A. E-D. H. (2021). Investigating the effects of copper sulfate and copper oxide nanoparticles in nile tilapia (Oreochromis niloticus) using multiple biomarkers: The prophylactic role of spirulina. Environ. Sci. Pollut. Res. 28 (23), 30046–30057. doi:10.1007/s11356-021-12859-0
Stevens, M. L. (1997). Fundamentals of Clinical Hematology. Saunders, Philadelphia, PA: Talapatra SN, Banerjee SKDetection of micronucleus and abnormal nucleus in erythrocytes from the gill and kidney of Labeo bata cultivated in sewage fed fish farms. Food Chem. Toxicol. 45, 210–215. doi:10.1016/j.fct.2006.07.022
Tadic, M., Panjan, M., Tadic, B. V., Lazovic, J., Damnjanovic, V., Kopani, M., et al. (2019). Magnetic properties of hematite (– FeO) nanoparticles synthesized by sol-gel synthesis method: The influence of particle size and particle size distribution. J. Electr. Eng. 70, 71–76. doi:10.2478/jee-2019-0044
Talapatra, S. N., and Banerjee, S. K. (2007). Detection of micronucleus and abnormal nucleus in erythrocytes from the gill and kidney of Labeo bata cultivated in sewage fed fish farms. Food Chem. Toxicol. 45, 210–215.
Tang, Q., Feng, L., Jiang, W., Liu, Y., Jiang, J., Li, S., et al. (2013). Effects of dietary copper on growth, digestive, and brush border enzyme activities and antioxidant defense of hepatopancreas and intestine for young grass carp (Ctenopharyngodon idella). Biol. Trace Elem. Res. 155 (3), 370–380. doi:10.1007/s12011-013-9785-6
Trifuoggi, M., Pagano, G., Guida, M., Palumbo, A., Siciliano, A., Gravina, M., et al. (2017). Comparative toxicity of seven rare Earth elements in sea urchin early life stages. Environ. Sci. Pollut. Res. 24 (25), 20803–20810. doi:10.1007/s11356-017-9658-1
Vieira, L. R., Gravato, C., Soares, A., Morgado, F., and Guilhermino, L. (2009). Acute effects of copper and mercury on the estuarine fish pomatoschistus microps: Linking biomarkers to behaviour. Chemosphere 76 (10), 1416–1427. doi:10.1016/j.chemosphere.2009.06.005
Wang, W., Mai, K., Zhang, W., Ai, Q., Yao, C., Li, H., et al. (2009). Effects of dietary copper on survival, growth and immune response of juvenile abalone, Haliotis discus hannai Ino. Aquaculture 297 (1-4), 122–127. doi:10.1016/j.aquaculture.2009.09.006
Waseem, M., and Parvez, S. (2013). Mitochondrial dysfunction mediated cisplatin induced toxicity: Modulatory role of curcumin. Food Chem. Toxicol. 53, 334–342. doi:10.1016/j.fct.2012.11.055
Wilson, J. M., Bunte, R. M., and Carty, A. J. (2009). Evaluation of rapid cooling and tricaine methanesulfonate (Ms222) as methods of euthanasia in zebrafish (Danio rerio). J. Am. Assoc. Lab. Anim. Sci. 48, 785–789.
Woryi, J., Ugbomeh, A., Gabriel, U., and Daka, E. (2020). Chronic effects of paraquat on haematology of african catfish (Clarias gariepinus). IJISET-Int J. Innov. Sci. Eng. Technol. 7, 73–83.
Keywords: Alkaline rocks, SOD, DNA, antioxidant, blood profile
Citation: Saad E, Hamed M, Elshahawy AM, Abd El-Aal M and Sayed AE-DH (2023) Effects of major and trace elements from the El Kahfa ring complex on fish: Geological, physicochemical, and biological approaches. Front. Environ. Sci. 10:1013878. doi: 10.3389/fenvs.2022.1013878
Received: 07 August 2022; Accepted: 18 November 2022;
Published: 16 January 2023.
Edited by:
Chuan Wu, Central South University, ChinaReviewed by:
Hamdy Soliman, Sohag University, EgyptCopyright © 2023 Saad, Hamed, Elshahawy, Abd El-Aal and Sayed. This is an open-access article distributed under the terms of the Creative Commons Attribution License (CC BY). The use, distribution or reproduction in other forums is permitted, provided the original author(s) and the copyright owner(s) are credited and that the original publication in this journal is cited, in accordance with accepted academic practice. No use, distribution or reproduction is permitted which does not comply with these terms.
*Correspondence: Alaa El-Din H. Sayed, YWxhYXNheWVkQGF1bi5lZHUuZWc=; Eman Saad, RW1hbi5zYWFkQGF1bi5lZHUuZWc=
Disclaimer: All claims expressed in this article are solely those of the authors and do not necessarily represent those of their affiliated organizations, or those of the publisher, the editors and the reviewers. Any product that may be evaluated in this article or claim that may be made by its manufacturer is not guaranteed or endorsed by the publisher.
Research integrity at Frontiers
Learn more about the work of our research integrity team to safeguard the quality of each article we publish.