- 1Institute of Hydrogeology and Environmental Geology, Chinese Academy of Geological Sciences, Shijiazhuang, China
- 2Key Laboratory of Groundwater Science and Engineering, Ministry of Natural Resource, Shijiazhuang, China
- 3Shanxi Geological Engineering Exploration Institute CO., Ltd. Shanxi, Taiyuan, China
Rivers are the main supply sources in inland areas for human activities, but they are also regarded as the most susceptible water bodies to pollutants. Understanding the key factors influencing the chemical characteristic is the basis for water supply and public health concern. And it is helpful for the protection of surface water under the influence of human activities. To reveal the hydrochemical process of river water and the key factors affecting the chemical compositions, a total of 33 samples from rivers in Muling-Xingkai Plain are collected for principal component analysis and hydrochemical analysis. Results indicate that river water is characterized by the type of HCO3-Ca and mixed HCO3-Ca·Na. But some samples with relative high nitrate content have Cl− as the dominant anion. The natural sources of chemical ions in river water are silicate and carbonate minerals. The chemical fertilizers only slightly influence the chemical compositions of river water due to the retardation of black soil with weak permeability. The chemical compositions of river water in Muling river are significantly influenced by domestic sewage compared with that in Abuqin river and Qihulin river. The widespread thick black soils play a key roles in protecting the river quality and groundwater quality, and human activities only play a limited roles in determining the river quality in the Muling-Xingkai Plain. At present, the contents of major chemical ions in river water meet the irrigation standard. Although the irrigation with river water do not lead to the food safety issue, the government agencies should adopt adequate measures to control the indiscriminate discharge of domestic sewage and application of fertilizers for preventing the accumulation of pollutants in rivers. This study is beneficial to the efficient management of surface water resources in agricultural areas with similar geological conditions and hydrogeological conditions.
1 Introduction
Due to the widespread availability and accessibility, river water plays a vital roles in social and economic development. Rivers usually are the important water resources for domestic, industrial and agricultural purposes (Rehman Qaisar et al., 2018; Xiao et al., 2019). However, rivers also play a crucial role in accepting industrial, agricultural and domestic wastewater, and they are regarded as the most susceptible water bodies to pollutants (Marzieh et al., 2020; Liu et al., 2021). River water with high level of chemical components exceeding the relevant standard is indirectly or directly influencing the human health (Huang et al., 2018; Kaline et al., 2020). Understanding the chemical characteristic of river water is a prerequisite for effective and efficient water management (Tiyasha et al., 2020; He et al., 2022).
The chemical characteristic of river water has attracted great attention all over the world (Peter et al., 2020; Feng et al., 2022). Many studies were concentrated on the famous rivers, such as the Amazon river (Ríos-Villamizar et al., 2017), the Nile (Wael et al., 2021), the Yangtze river (Zhang et al., 2022) and the Yellow river (Lv et al., 2022), etc. Their results were contributed to the protection and conservation of water resources and other natural resources. Generally, the chemical compositions of river water depend on natural factors such as recharge water quality and soil-water-rock interaction. Moreover, human activities can also impact surface water chemistry and profoundly alter the chemical compositions of river water to such an extent that surface water suitability becomes limited (Tripathee et al., 2016; Singh et al., 2017; Daou et al., 2018; Soares et al., 2020). The influences of human activities on the chemical compositions of river water are not only by inputting pollutants, but also by influencing the quality of groundwater recharging rivers (Yemeli et al., 2021). So the chemistry of river water is regulated by complex interactions under various physical, chemical, and biological environments (Barzegar et al., 2019; Hakimi et al., 2021). Understanding the sources of chemical ions in river water is the basis of the prediction and prevention of river pollution (Pandey et al., 2018; Richard and Irfan, 2020). Thus, it is of great significance to distinguish the effects of geogenic and anthropogenic factors on chemical compositions of river water for the sustainable development of water resource and society.
Many useful approaches are used to distinguish the sources of chemical components in groundwater and river water (Xiao et al., 2021). Hydrochemical analyse is an effective method which is performed based on the correlation among different chemical variables (Qaisar et al., 2020; Xiao et al., 2022a). Principal component analysis (PCA) is an unbiased method which can indicate associations between variables. These methods have been widely used to investigate chemical patterns, to determine various factors controlling the chemical evolution processes, and to show the origin and mobility of both geogenic and anthropogenic pollutants (Liu and Han, 2020; Sunkari et al., 2021; Laouni et al., 2022). These studies have provided a solid foundation for the protection of natural resources.
Muling-Xingkai Plain (MXP), locating in the northeast part of China, is one of the largest and most important grain production bases in China. River water is the important supply source for agricultural production. Previous study showed the chemical compositions of river water were influenced by agricultural activities (Cao et al., 2012). But a hydrogeochemical investigation conducted by author found that, a certain amount of domestic sewage was directly discharged into river channels. This process could contribute pollutant to river water. At present, knowledge about natural factors and human influences on water quality of river water in the MXP is still unclear, which has important constrains on agricultural irrigation management and food security. Additionally, the hydrochemical process of river water with the influence of agricultural activities in northeast China are not identified. Thus, a typical agricultural zone of northeast China was selected to deal with the hydrogeochemical characteristics, governing mechanisms, and chemical evolution processes of river water. Specifically, the main objectives of this study are to 1) show the sources of chemical ions in river water, 2) identify the factors influencing the chemical compositions of river water, and 3) provide some implications for the management on water resource in the MXP. The results will be beneficial to the protection of water resources and the sustainable development of agricultural production in the MXP and the areas with similar geological conditions and hydrogeological conditions.
2 Study area
MXP locates between longitudes of 131°30′–133°40′ E and latitudes of 45°05′–46°17′ N, with an area of about 10,000 km2. MXP is one of the most important grain production bases in China, and the farmlands are widely distributed. Rice is one of the important crops in the MXP, and the rice plant area is more than 85% in the whole crop areas. The climate is a temperate monsoon climate, characterized by hot summer and cold winter. The average annual precipitation is 570 mm, ranging from 360 mm to 850 mm. The precipitation is mainly concentrated in the summer (June to August), accounting for 53% of the total precipitation. The annual mean temperature is approximately 3.5°C.
Abuqin River (AR), Qihulin (QR) and Muling River (MR) are three major rivers in the MXP, and the flow direction of rivers is from west to east (Figure 1). AR and QR originate from northwestern mountains in the MXP, with the lengths of 145 km and 260 km, respectively. MR originates from the Laoye mountains far away from the MXP, and passes through several cities and towns along its course. The length of downstream part of MR in the MXP is only 200 km. The annual runoffs of AR, QR and MR are 20 m3 s−1, 30 m3 s−1 and 70 m3 s−1, respectively.
River water is mainly recharged by the meteoric water and the groundwater in the riverine areas. In addition, a certain mount of rural wastewater is directly discharged into river channels in the local zones. Thus, wastewater from human activities can be regarded as one of the recharge sources. River water is principally discharged by evaporation, artificial extraction, and flowing into the Wusuli River. River water is the important supply source for agricultural irrigation.
Aquifers principally consist of Quaternary alluvial deposits, and the depth to groundwater is about 2–20 m. Phreatic aquifer is widely overlain by the black clay layer with small infiltration coefficient. Coarse-textured soils with high infiltration capacity only distribute in the riverine areas (Figure 2). Rock debris and soils are consisted of quartz (52%), plagioclase (21%), clay mineral (16%), potassium feldspar (10%) and carbonates (1%) (Su et al., 2022).
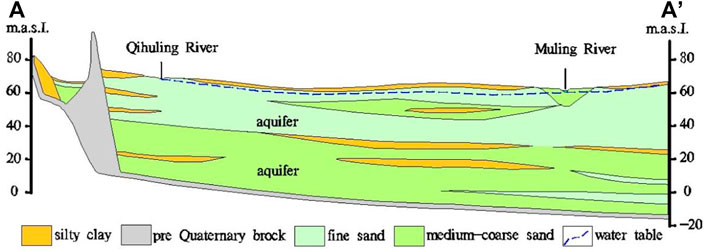
FIGURE 2. Hydrogeologic cross section A-A′ shown in Figure 1.
3 Materials and methods
3.1 Sampling and laboratory analysis
A total of 33 river water samples were collected in July 2018, and 7, 12, and 14 river water samples were collected from AR, QR and MR, respectively (Figure 1). Water samples were collected from each site at depth of 30 cm beneath the surface. Water temperature and pH were performed in site using a portable pH instrument. River water samples were collected in 1500-ml high density polyethylene sampling bottles. Prior to sample collection, sampling bottles were rinsed third times with the water to be sampled. Samples were stored under a temperature of 4°C, and were filtrated through 0.45-μm filter membranes once sent to the laboratory. Major cations (K+, Na+, Ca2+, and Mg2+) were determined by inductively coupled plasma-mass spectrometry. Anions (NO3−, Cl−, and SO42−) were measured by anion chromatography. The detection limits of ion analyses were 0.05ppm. HCO3− was measured by HCl titration on the spot. TDS values were obtained based on the above analysis data. The accuracy and precision of the analytical technique were evaluated by analyzing a certified standard reference material, certified waste water trace metals solution. Uncertainties were less than ± 5% for all analyzed elements. All the analyses were carried out at the Groundwater Mineral Water and Environmental Monitoring Center of the Institute of Hydrogeology and Environmental Geology, Chinese Academy of Geological Sciences.
3.2 Methodology
In this study, PCA and hydrochemical analysis were used to determine the hydrochemical process and controls on the hydrochemistry of river water in the MXP. PCA can be used to identify the principal components and their significance, and to further show various environmental issues (Khound and Bhattacharyya, 2018). The rotation of principal components (PCs) was carried out using varimax method. The terms “strong,” “moderate,” and “weak” classified by Liu et al. (2003) were applied to PC loading, corresponding to the absolute loading values of >0.75, 0.75–0.5, and 0.5–0.3, respectively.
Hydrochemical analysis was carried out based on the Gibbs diagram, piper trilinear diagram, correlation analysis of chemical variables. Gibbs diagram was mapped to identify the natural source of chemical ions in river water. Piper trilinear diagram was drawn to understand the dominant water types. Correlation analysis of chemical variables was mainly used to evaluate various hydrochemical evolution process in river water. These methods were jointly used to determine the chemical characteristics and dominate factors controlling the chemical compositions of river water.
4 Results and Discussion
4.1 General chemical characterization
The descriptive statistics of major chemical variables in river water are summarized in Table 1, including minimum, maximum, mean value, the standard deviation, and coefficient of variation (CV). Ca2+ (20.44 mg/L) is the most abundant major cation followed by Na+ (13.46 mg/L), Mg2+ (5.92 mg/L), K+ (2.69 mg/L), and NH4+ (0.12 mg/L). Variable coefficient of NH4+ and Na+ is highest. HCO3− (74.11 mg/L) is the most dominant major anion followed by SO42− (19.38 mg/L), Cl− (13.70 mg/L), and NO3− (8.12 mg/L). But NO3− has the most significant variation. The high variation of chemical variables in river water can be attributed to the multiple geogenic and anthropogenic sources.
Concentrations of the main chemical ions in MR are highest, while the values of chemical variables in AR are lowest. The mean concentration of chemical ions in QR is slightly higher than that in AR. Samples from MR are characterized by high NO3− content with low coefficient of variation (CV), which is related to the human activities. The CVs of Ca2+, HCO3− and NO3− in AR are lowest compared with other two rivers. The chemical compositions of river water in AR are stable, reflecting the slight influence by external factors.
Piper diagram was introduced to identify the hydrochemical types and chemical characteristics of river water samples in the MXP (Figure 3). It can be seen that most samples have Ca2+ as the dominant cation, and only one sample collected from QR has Na+ as the dominant cation. Most samples have HCO3− as the dominant anion, and two samples collected from QR and MR have Cl− as the dominant anion.
Two hydrochemical facies HCO3-Ca and mixed HCO3-Ca·Na were determined from the Piper plot, indicating river water undergone an evolution from HCO3-Ca type to mixed type. Specifically, samples from AR are characterized by the water type of Ca·Mg-HCO3. The water types of samples from QR are Ca·Mg-HCO3 and Ca·Mg-HCO3·Cl. The water types of samples from MR are predominantly Ca·Na-HCO3, but some samples are characterized by the water type of Ca·Mg·Na-HCO3 and Ca·Na·Mg-HCO3·Cl. The difference of water types among rivers indicates that the chemical compositions of river water are affected by multiple factors. The samples with low nitrate content are dominated by HCO3-Ca type, and those with relative high nitrate content are mainly mixed HCO3-Ca·Na type and HCO3·Cl type. The dominant anions show a significant gradual evolving trend from HCO3− to Cl− with the increase of nitrate content. Thus, anthropogenic factor is influencing the chemical composition of river water in the MXP.
4.2 Geogenic source of chemical ion in river water
Chemical ions in river water have multiple sources from physical, chemical, and biological processes, including atmospheric precipitation, mineral weathering (e.g., carbonates, silicates, and evaporites), and human activities (Rehman Qaisar et al., 2018; Barzegar et al., 2019). Gibbs plot, mixing diagram and ion ratios of major constitutes in water can be used to identify the source of chemical ions.
Gibbs plot can detect three natural factors dominating the chemical compositions of river water: precipitation, rock dominance, and evaporation. As shown in Figure 4, the weight ratios of Na+/(Na++Ca2+) and Cl−/(Cl− + HCO3−) of most samples are less than 0.5, with mean TDS of 100 mg/L. The chemical compositions of river water are strongly influenced by the rock weathering in the MXP (Marandi and Shand, 2018). Rivers pass through various rocks and soils, which are the main contributors to the high levels of minerals in river water. The dissolution of various minerals leads to an abundance of chemical ions in river water. Precipitation is only the original source of chemical ions in river water. Evaporation is not the key factors dominating the concentration of chemical ions due to the humid climate and abundant rainfall in the study area.
Weathering of carbonate rocks may contribute Ca2+, Mg2+, and HCO3−, and weathering of silicate rocks can produces Na+, K+, Ca2+, Mg2+, and HCO3−. Dissolution of evaporites mainly produces SO42-, Ca2+,Cl−, and Na+ (Paudyal et al., 2016). Dissolution of different minerals can lead to different combinations of major ions in water. The mixing diagram can be used to infer dissolution of minerals with respect to three representative lithology. The mixing diagram is depicted based on Na+-normalized molar ratios (Figure 5). Most of samples lie close to the end-member of silicate, indicating that the chemical compositions of river water are dominated by the silicate dissolution.
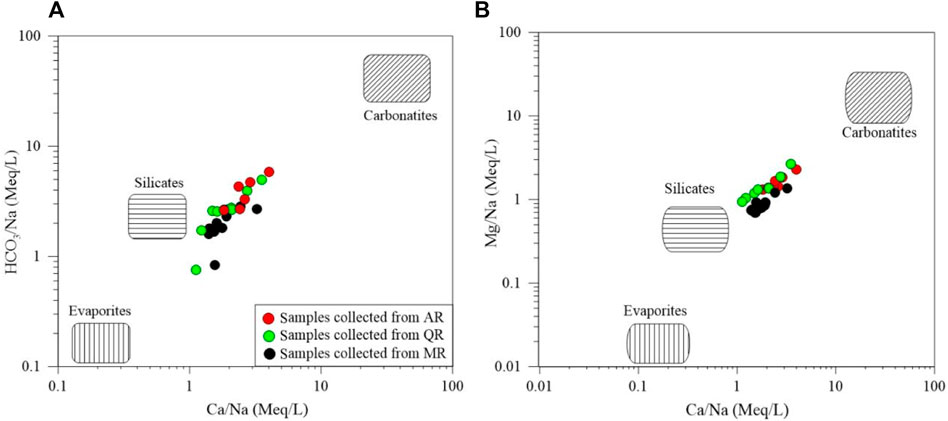
FIGURE 5. Bivariate diagrams of (A) (HCO3/Na) vs. (Ca/Na), and (B) (Mg/Na) vs. (Ca/Na) for surface water samples in the study area.
The mineralogical analysis has shown the compositions of rocks and soils are silicate minerals. That is why the chemical weathering of silicate rocks is the main contributor to the chemistry of river water in the MXP.
The concentration ratios of chemical ions can indicate various hydrochemical processes. The sources of Ca2+, Mg2+ and HCO3− can be deduced from the milliequivalent ratio of c (Ca2++Mg2+)/cHCO3-. As shown in Figure 6A, most of samples from these three rivers are close to the 1:1 equivalent line, showing that the Ca2+, Mg2+ and HCO3− in river water are derived from dissolution of silicate and carbonate minerals. However, some samples from MR fall below the 1:1 equivalent line, suggesting the silicate dissolution is responsible for the relevant chemical compositions of river water. There is a significant relationship between the c (Cl− + SO42-) and c (Na++K+) (r2 = 0.81) in the rives (Figure 6B), which suggests their similar source. When the halite is dominant in river water, cNa+/cCl− would be approximately 1.0. And if it is >1.0, the source of Na+ is other Na-bearing salt. As shown in Figure 6D, averaged cNa+/cCl− of samples from AR and MR is much higher than 1.0 (AR = 1.79, and MR = 1.55). Then, dissolution of other Na-bearing salts or ion exchange are influencing the Na+ contents of river water. Figure 6C shows that Sr2+ concentrations are linearly correlated with the concentration of (Na++K+) (r2 = 0.81). This further identifies the chemical compositions of river water in the MXP are dominated by the silicate minerals dissolution.
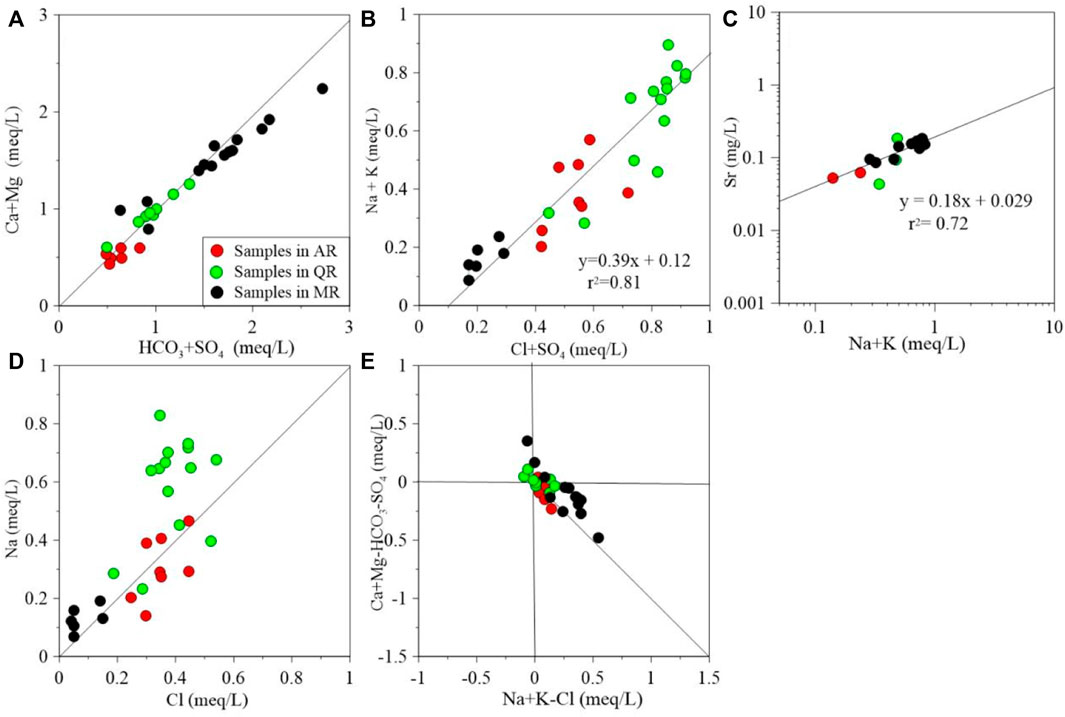
FIGURE 6. Bivariate diagrams of (A) (Ca + Mg) vs. (HCO3 + SO4), (B) (Na + K) vs. (Cl + SO4), (C) Sr vs. (Na + K), (D) Na vs. Cl, (E) (Ca + Mg-HCO3-SO4) vs. (Na + K-Cl).
4.3 Anthropogenic source of chemical ion in river water
As shown in Figure 6D, the averaged cNa+/cCl− of samples from QR is slightly less than 1.0 (QR = 0.89). The plot of (Ca + Mg)—(HCO3 + SO4) against (Na + K—Cl) could be employed to evaluate the role of water-rock interaction on hydrochemistry. If the relation between these two parameters is linear with a slope of −1.0, ion exchange is significant in controlling geochemical compositions. Figure 6E shows that water samples do not fall along the line with a slope of −1.0. Therefore, chemical compositions of river water in the MXP are not significantly dominated by ion exchange.
The major anthropogenic components in the groundwater and surface water include Cl− and NO3−, as well as SO42-. Groundwater and surface water has a small ratio of NO3−/Cl− under natural condition, otherwise it is assumed to have been influenced by anthropogenic activities (Xiao et al., 2022b). The relation between NO3−/Cl− ratio and Cl− content can provide important information to distinguish the input sources of NO3− and Cl− in water.
As shown in Figure 7, some samples collected from MR and QR are mainly plotted in the dominance of sewage input. This suggests the source of nitrate is the domestic effluents. Most samples collected from AR and QR are mainly plotted in the dominance of fertilizer and manure inputs, but the ratio of NO3−/Cl− is less than 0.22. Generally, natural water sampled outside of human influence usually has a ratio of NO3−/Cl− ranging from 0.05 to 0.22. Thus, the nitrate contribution of agricultural practices would be considerable negligible. Although the nitrogenous fertilizers and ammonium chloride fertilizers are widely used in the MXP, agricultural activities only play a slight role in determining the chemical compositions.
A certain amount of rural wastewater is directly discharged into the river channels or the seepage pits as the lack of sewage treatment plant in the rural areas. Thus, discharge of domestic sewage can directly and indirectly influence the chemical compositions of river water, especially for the water from MR. Therefore, the hydrochemistry of nitrate enriched river water are governed by domestic sewage from the residential areas.
4.4 Factors in dominating the chemical compositions of river water
PCA can be used to discuss the key factors dominating the chemical compositions. 11 physico-chemical variables have been analyzed in water samples based on data integrity. The results are shown in Table 2. The chemical compositions of river water are controlled by a two-factor model, and the cumulative variance of two PCs is 79.15%. PC1, which comprises strong loading of TDS, HCO3−, Ca2+, Mg2+, Na+, SO42-, pH and Cl−, accounts for 68.19% of the total variance. TDS values increase with the concentrations of HCO3−, Ca2+, Mg2+ and Na+. Water-rock interactions are mainly responsible for their existence in river water (Huang et al., 2018). PC2 with high positive loading of Cl− and NO3−, accounts for 16.23% of the total variance. Domestic sewage and agricultural effluent contain abundant ammonia nitrogen and chloride in the MXP. Thus, the discharge of wastewater from human activities is responsible for the high level of Cl− and NO3− in river water.
For AR, PC1 with high positive loading of TDS, HCO3−, K+, Na+ and Mg2+, indicating the contribution of minerals to the chemical compositions. PC2 has high positive loading of Cl− and NO3−, accounting the impact of human activities. For QR, PC1 has high positive loading of pH, Ca2+, Na+ and HCO3−, indicating the chemical compositions of river water are controlled by water-rock interaction. PC2 has high positive loading of K+, Na+, Cl−, and NO3−, suggesting the human activities are influencing the chemical compositions of river water. For MR, PC1 with high positive loading of HCO3−, pH, Ca2+, Mg2+, and TDS, indicates the water-rock interaction is influencing the chemical compositions of river water. PC2 with high positive loading of NO3− and Cl−, showing that the chemical compositions of river water have been influenced by human activities.
Based on the discussion above mentioned, it is can be concluded that the natural sources of chemical ions in river water are the silicate minerals and the carbonate minerals, and the anthropogenic sources are the agricultural fertilizers and domestic sewage. The contribution of each sources can be quantified based on PCA (Figure 8).
The chemical compositions of river water in the MXP are mainly dominated by the dissolution of the silicate and carbonate minerals. Fertilizer and sewage only play a limited role in determine the qualities of river water. However, the density of population in the riverine areas of MR is largest compared with other two rivers. A large quantity of wastewater is discharged into MR or the seepage pits in the riverine areas. So the influence of sewage on the chemical compositions of river water in MR is significant. Thus, the impact of external factors on chemical compositions is closely related to the density of population in the riverine areas.
The anthropogenic factors have influenced the chemical compositions of river water in the MXP. But the concentrations of major chemical ions in river water do not exceed the irrigation standard. Agricultural production and discharge of domestic sewage do not lead to the food safety issue.
The lateral discharge of groundwater into rivers is an important way of anthropogenic influence on the chemical compositions of river water. Most of crops grow in rich, black soil in the MXP. Black soils with weak permeability can deter or retard the downward infiltration of irrigation water with abundant chemical fertilizers. At present, most of groundwater samples in the MXP is characterized by low level of chemical ions (Su et al., 2020). Thus, agricultural activities do not influence the chemical composition of river water significantly due to the weak permeability of black soil. The saturation index of calcite, dolomite and gypsum is below zero, suggesting the river water is in an unsaturated state. The dissolution of silicate minerals and carbonate minerals still will be the main source of chemical ions in river water.
4.5 Suggestions for protection of river water
Due to the discharge of domestic sewage in the riverine zones with coarse sediments, some groundwater samples along the rivers are characterized by high level of NO3−, Cl−, and SO42- (Su et al., 2020). Although the chemical compositions of river water are slightly influenced by groundwater, the continuous inflow of contaminants by groundwater discharge is a potential threat to river quality. Therefore, some measures should be adopted to further protect the groundwater and surface water resources for maintaining the food security. It is helpful for the management of surface water resource in agricultural areas.
Anthropogenic factors can be effectively controlled (Huang et al., 2013). The specific strategies are: 1) to keeping the use of chemicals to an absolute minimum; 2) to monitor and control the discharge of the wastewater in the riverine areas; 3) to construct the sewage disposal system in the rural zone near rivers; 4) to set the key protection region in the riverine areas; and 5) to regularly monitor the quality of groundwater in the riverine zones and river water.
5 Conclusion
The present study was conducted to elucidate the hydrochemical process and controls on the hydrochemistry of river water in an agricultural region of northeast Chain. The main findings are as follows:
(1) Most samples in the MXP have Ca2+ and HCO3− as the dominant ions, and only few samples have Na+ and Cl− as the dominant ions. River water is characterized by the type of HCO3-Ca and mixed HCO3-Ca·Na. But few samples with relative high nitrate content have Cl− as the dominant anion. Natural and anthropogenic factors are jointly influencing the chemical compositions of river water.
(2) The chemical compositions of river water in the MXP are mainly dominated by the natural dissolution of silicate minerals and carbonate minerals. Agricultural activities do not lead to the deterioration of river water quality. Domestic sewage only slightly influence the chemical compositions of river water in MR. The widespreadly thick black soils play a key roles in protecting the river quality and groundwater quality. So the human activities only play a limited roles in determine the river quality. At present, the surface-water irrigation do not lead to food security issues, and the concentrations of major chemical ions in river water meet the standard for irrigation.
(3) Water protection, that based on chemical characteristic of river water and human activities intensity, is recommended to guarantee food safe and residents’ health in the study area. Due to the coarse sediments overlying the aquifers in the riverine zones, the government agencies should adopt some strategies to control the discharge of various wastewater for preventing the accumulation of pollutants in surface water and groundwater.
Data availability statement
The raw data supporting the conclusion of this article will be made available by the authors, without undue reservation.
Author contributions
This article is the combined effort of all the author. CS and YL formulated and wrote the article, with contributions from all coauthors ZC wrote a detailed the introduction CS and WW contributed to the analysis. ZZ collected the samples in study area.
Funding
This study was financially supported by the National Natural Science Foundation of China (No. 41602268) and China Geological Survey’s project (No. DD20160311).
Conflict of interest
Author YL was employed by the Shanxi Geological Engineering Exploration Institute CO., Ltd. Shanxi
The remaining author declares that the research was conducted in the absence of any commercial or financial relationships that could be construed as a potential conflict of interest.
Publisher’s note
All claims expressed in this article are solely those of the authors and do not necessarily represent those of their affiliated organizations, or those of the publisher, the editors and the reviewers. Any product that may be evaluated in this article, or claim that may be made by its manufacturer, is not guaranteed or endorsed by the publisher.
References
Barzegar, R., Moghaddam, A. A., Soltani, S., Baomid, N., Tziritis, E., Adamowski, J., et al. (2019). Natural and anthropogenic origins of selected trace elements in the surface waters of Tabriz area, Iran. Environ. Earth Sci. 78 (8), 254. doi:10.1007/s12665-019-8250-z
Cao, Y. J., Tang, C. Y., Song, X. F., Liu, C. M., and Zhang, Y. H. (2012). Characteristics of nitrate in major rivers and aquifers of the Sanjiang Plain, China. J. Environ. Monit. 14 (10), 2624. doi:10.1039/C2EM30032J
Daou, C., Salloum, M., Legube, B., Kassouf, A., and Ouaini, N. (2018). Characterization of spatial and temporal patterns in surface water quality: A case study of four major Lebanese rivers. Environ. Monit. Assess. 190 (8), 485. doi:10.1007/s10661-018-6843-8
Feng, W., Lu, H. W., Yao, T. C., Guan, Y. L., Xue, Y. X., and Yu, Q. (2022). Water environmental pressure assessment in agricultural systems in central asia based on an integrated excess nitrogen load model. Sci. Total Environ. 803, 149912. doi:10.1016/j.scitotenv.2021.149912
Hakimi, Y., Orban, P., Deschamps, P., and Brouyere, S. (2021). Hydrochemical and isotopic characteristics of groundwater in the continental intercalaire aquifer system: Insights from mzab ridge and surrounding regions, north of the Algerian sahara. J. Hydrology Regional Stud. 34, 100791. doi:10.1016/j.ejrh.2021.100791
He, J., Tao, L., Peng, Y. E., Tong, L., Zhao, X. W., Shao, X., et al. (2022). Distribution and impacts on the geological environment of antiviral drugs in major waters of Wuhan, China. zgdzyw. 5 (3), 402–410. doi:10.31035/cg2022047
Huang, G. X., Liu, C. Y., Sun, J. C., Zhang, M., Jing, J. H., and Li, L. P. (2018). A regional scale investigation on factors controlling the groundwater chemistry of various aquifers in a rapidly urbanized area: A case study of the pearl river delta. Sci. Total Environ. 625, 510–518. doi:10.1016/j.scitotenv.2017.12.322
Huang, G. X., Sun, J. C., Zhang, Y., Chen, Z. Y., and Liu, F. (2013). Impact of anthropogenic and natural processes on the evolution of groundwater chemistry in a rapidly urbanized coastal area, South China. Sci. Total Environ. 463-464 (5), 209–221. doi:10.1016/j.scitotenv.2013.05.078
Kaline, M., Ricardo, H. T., Felipe, R. P., Roberta, A. V., Timothy, O. R., Diego, R. M., et al. (2020). Multiscale land use impacts on water quality: Assessment, planning, and future perspectives in Brazil. J. Environ. Manag. 270, 110879. doi:10.1016/j.jenvman.2020.110879
Khound, N. J., and Bhattacharyya, K. G. (2018). Assessment of water quality in and around Jia-Bharali river basin, North Brahmaputra Plain, India, using multivariate statistical technique. Appl. Water Sci. 8 (8), 221. doi:10.1007/s13201-018-0870-z
Laouni, B., Belkacem, B., and Laouni, G. (2022). Multivariate analysis and geochemical investigations of groundwater in a semi-arid region, case of Ghriss Basin superficial aquifer North-West Algeria. J. Groundw. Sci. Eng. 10 (3), 233–249. doi:10.19637/j.cnki.2305-7068.2022.03.003
Liu, C. W., Lin, K. H., and Kuo, Y. M. (2003). Application of factor analysis in the assessment of groundwater quality in a Blackfoot disease area in Taiwan. Sci. Total Environ. 313 (1-3), 77–89. doi:10.1016/S0048-9697(02)00683-6
Liu, J., and Han, G. (2020). Major ions and δ34S-SO4 in Jiulongjiang River water: Investigating the relationships between natural chemical weathering and human perturbations. Sci. Total Environ. 724, 138208. doi:10.1016/j.scitotenv.2020.138208
Liu, R. P., Li, Z. Z., Liu, F., Dong, Y., Jiao, J. P., Sun, P. P., et al. (2021). Microplastic pollution in Yellow River: Current status and research progress of biotoxicological effects. China Geol. 4, 1–8. doi:10.31035/cg2021081
Lv, D. Y., Yu, C., Zhuo, Z. J., Meng, S. R., and Liu, S. B. (2022). The distribution and ecological risks of antibiotics in surface water in key cities along the lower reaches of the Yellow River:A case study of Kaifeng City, China. China Geol. 5, 411–420. doi:10.31035/cg2022032
Marandi, A., and Shand, P. (2018). Groundwater chemistry and the Gibbs diagram. Appl. Geochem. 97, 209–212. doi:10.1016/j.apgeochem.2018.07.009
Marzieh, M., Ali, S., and Vahideh, S. (2020). Effects of heavy metal contamination on river water quality due to release of industrial effluents. J. Clean. Prod. 277, 123380. doi:10.1016/j.jclepro.2020.123380
Pandey, L. K., Lavoie, I., Morin, S., Park, J., Lyu, J., Choi, S., et al. (2018). River water quality assessment based on a multi-descriptor approach including chemistry, diatom assemblage structure, and non-taxonomical diatom metrics. Ecol. Indic. 84, 140–151. doi:10.1016/j.ecolind.2017.07.043
Paudyal, R., Kang, S., Sharma, C. M., Tripathee, L., Huang, J., Rupakheti, D., et al. (2016). Major ions and trace elements of two selected rivers near Everest region, southern Himalayas, Nepal. Environ. Earth Sci. 75, 46. doi:10.1007/s12665-015-4811-y
Peter, J. R., Ricardo, G. P., David, J. V., Justin, K. R., Justin, N., and Aashish, K. (2020). Water quality impacts of urban and non-urban arid-land runoff on the Rio Grande. Sci. Total Environ. 729, 138443. doi:10.1016/j.scitotenv.2020.138443
Qaisar, M., Muhammad, A., and Muhammad, R. (2020). Integration of geoelectric and hydrochemical approaches for delineation of groundwater potential zones in alluvial aquifer. J. Groundw. Sci. Eng. 8 (4), 366–380. doi:10.19637/j.cnki.2305-7068.2020.04.007
Rehman Qaisar, F. U., Zhang, F., Pant, R. R., Wang, G. X., Khan, S., and Zeng, C. (2018). Spatial variation, source identification, and quality assessment of surface water geochemical composition in the Indus River Basin, Pakistan. Environ. Sci. Pollut. Res. 25 (13), 12749–12763. doi:10.1007/s11356-018-1519-z
Richard, J. C., and Irfan, A. B. (2020). Impacts of irrigation water used allocations on water quality and crop productivity: The lee irrigation system in Pakistan. Irrig. Drain. 69, 38–51. doi:10.1002/ird.2402
Ríos-Villamizar, E. A., Piedade, M. T. F., Junk, W. J., and Waichman, A. V. (2017). Surface water quality and deforestation of the Purus river basin, Brazilian Amazon. Int. Aquat. Res. 9, 81–88. doi:10.1007/s40071-016-0150-1
Singh, H., Singh, D., Singh, S. K., and Shukla, D. N. (2017). Assessment of river water quality and ecological diversity through multivariate statistical techniques, and Earth observation dataset of rivers Ghaghara and Gandak. India. Int. J. River Basin Manag. 1-14, 347–360. doi:10.1080/15715124.2017.1300159
Soares, A. L. C., Pinto, C. C., and Oliveira, S. C. (2020). Impacts of anthropogenic activities and calculation of the relative risk of violating surface water quality standards established by environmental legislation: A case study from the piracicaba and paraopeba river basins, Brazil. Environ. Sci. Pollut. Res. 27, 14085–14099. doi:10.1007/s11356-020-07647-1
Su, C., Li, Z., Wang, W., Cheng, Z., Zheng, Z., and Chen, Z. (2022). Key factors dominating the groundwater chemical composition in a grain production base: A case study of muling–Xingkai Plain, northeast China. Water 14 (14), 2222. doi:10.3390/w14142222
Su, C., Zhang, F. E., Cui, X. S., Cheng, Z. Y., and Zheng, Z. X. (2020). Source characterization of nitrate in groundwater using hydrogeochemical and multivariate statistical analysis in the muling-xingkai plain, northeast China. Environ. Monit. Assess. 192, 456. doi:10.1007/s10661-020-08347-6
Sunkari, E. D., Abu, M., and Zango, M. S. (2021). Geochemical evolution and tracing of groundwater salinization using different ionic ratios, multivariate statistical and geochemical modeling approaches in a typical semi-arid basin. J. Contam. Hydrology 236, 103742. doi:10.1016/j.jconhyd.2020.103742
Tiyasha, T., Tung, T. M., and Yaseen, Z. M. (2020). A survey on river water quality modelling using artificial intelligence models: 2000–2020. J. Hydrology 585, 124670. doi:10.1016/j.jhydrol.2020.124670
Tripathee, L., Kang, S., Sharma, C. M., Rupakheti, D., Paudyal, R., Huang, J., et al. (2016). Preliminary health risk assessment of potentially toxic metals in surface water of the Himalayan rivers. Nepal. Bull. Environ. Contam. Toxicol. 97 (6), 855–862. doi:10.1007/s00128-016-1945-x
Wael, M. B., Octavian, G. D., Hussein, E. I. S., Atef, E., and Marina, V. F. (2021). A review of major and trace elements in Nile River and Western Red Sea sediments: An approach of geochemistry, pollution, and associated hazards. Appl. Radiat. Isotopes 170, 109595. doi:10.1016/j.apradiso.2021.109595
Xiao, J., Wang, L. Q., Deng, L., and Jin, Z. D. (2019). Characteristics, sources, water quality and health risk assessment of trace elements in river water and well water in the Chinese Loess Plateau. Sci. Total Environ. 650, 2004–2012. doi:10.1016/j.scitotenv.2018.09.322
Xiao, Y., Hao, Q. C., Zhang, Y., Zhu, Y. C., Yin, S. Y., Qin, L. M., et al. (2021). Investigating sources, driving forces and potential health risks of nitrate and fluoride in groundwater of a typical alluvial fan plain. Sci. Total Environ. 802, 149909. doi:10.1016/j.scitotenv.2021.149909
Xiao, Y., Liu, K., Hao, Q. C., Li, Y. S., Xiao, D., and Zhang, Y. J. (2022a). Occurrence, controlling factors and health hazards of fluoride-enriched groundwater in the lower flood plain of Yellow river, northern China. Expo. Health 14, 345–358. doi:10.1007/s12403-021-00452-2
Xiao, Y., Liu, K., Hao, Q. C., Xiao, D., Zhu, Y. C., Yin, S. Y., et al. (2022b). Hydrogeochemical insights into the signatures, Genesis and sustainable perspective of nitrate enriched groundwater in the piedmont of Hutuo watershed, China. Catena 212, 106020. doi:10.1016/j.catena.2022.106020
Yemeli, E. J., Temgoua, E., and Kengni, L. (2021). Hydro-geochemistry of groundwater and surface water in Dschang town (West Cameroon): Alkali and alkaline-Earth elements ascertain lithological and anthropogenic constraints. J. Groundw. Sci. Eng. 9 (3), 212–224. doi:10.19637/j.cnki.2305-7068.2021.03.004
Keywords: hydrochemistry, human activities, surface water, Muling-Xingkai Plain, groundwater
Citation: Su C, Liu Y, Cheng Z, Wang W and Zheng Z (2022) Hydrochemical process and controls on the hydrochemistry of river water in the Muling-Xingkai Plain, Northeast China. Front. Environ. Sci. 10:1010367. doi: 10.3389/fenvs.2022.1010367
Received: 03 August 2022; Accepted: 12 October 2022;
Published: 20 October 2022.
Edited by:
Yong Xiao, Southwest Jiaotong University, ChinaReviewed by:
Linxian Huang, University of Jinan, ChinaFatemeh Barzegari Banadkooki, Payame Noor University, Iran
Copyright © 2022 Su, Liu, Cheng, Wang and Zheng. This is an open-access article distributed under the terms of the Creative Commons Attribution License (CC BY). The use, distribution or reproduction in other forums is permitted, provided the original author(s) and the copyright owner(s) are credited and that the original publication in this journal is cited, in accordance with accepted academic practice. No use, distribution or reproduction is permitted which does not comply with these terms.
*Correspondence: Yuan Liu, 2930648938@qq.com