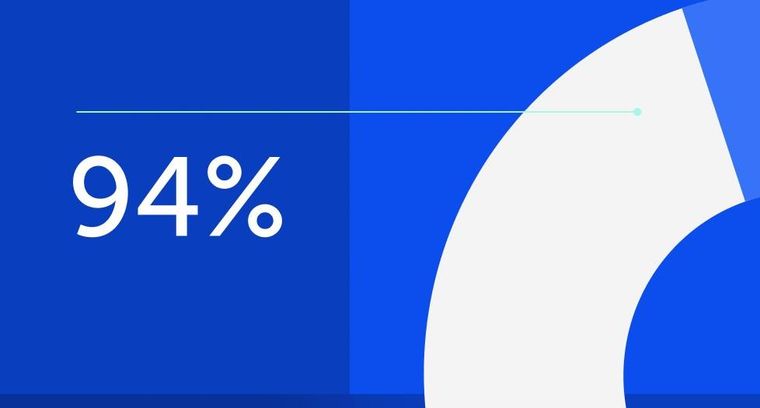
94% of researchers rate our articles as excellent or good
Learn more about the work of our research integrity team to safeguard the quality of each article we publish.
Find out more
ORIGINAL RESEARCH article
Front. Environ. Sci., 10 November 2022
Sec. Toxicology, Pollution and the Environment
Volume 10 - 2022 | https://doi.org/10.3389/fenvs.2022.1009802
This article is part of the Research TopicEnvironmental Impacts and Risks of Car Tire and Styrene-Butadiene Rubber: Microplastic Pollution and Contaminant TransportView all 7 articles
Materials made of rubber are a source of polymers released into the environment, where tire abrasions are a major contributor. In many hot, arid environs, whole tire losses are more common than in moderate climates, and lead to the accumulation of additional tire material on road sides, which over time can be the source of secondary micro-tires. Other rubber containing material from cars such as mud-flaps and floor mats has been seen as an appreciable additional source of micro-rubber. Due to the lack of precipitation, it is expected that modes of micro-tire relocation are more limited in arid regions than in more moderate climates. This 2-year study examines the rubber/tire content from 34 sampling sites within the region of Al Ain, Eastern region of Abu Dhabi Emirate, United Arab Emirates. The samplings include road dust, soil along roadsides, storm water run-off samples and samples collected in Wadis (creek beds that seasonally carry water) and one artificial lake. The mean average number of micro-tires in road dust was found to be 44.4 ± 40.6 micro-tires/g. In soil samples alongside the roads, it was 22.8 ± 42.1 micro-tires/g. The mean average size of micro-tires for all 34 sampling sites was found to be 106.9 ± 98.5 µm. It could be seen that rubber/tire materials desiccate over time during the high temperatures of the Emirati summer, become hard, brittle and break into smaller pieces, resulting in secondary micro-tire particles. The study of run-offs showed that tire particles are swept into storm drains during larger, very infrequently occurring rain events. However, the predominant mode of micro-tire transport was found to be on road by physical contact with cars, off-road by a combination of physical contact with moving objects and air movement. Samples taken from an artificial lake and three Wadis within the confines of Al Ain have shown only few micro-tire particles, so that the dispersion of micro-tires is not as pervasive as had been expected prior to this study.
Abraded tire particles contribute significantly to the overall release of synthetic polymers into our environment (Sundt et al., 2014; Lassen et al., 2015; Magnusson et al., 2016; Sherrington et al., 2016). As oftentimes the size of tire abrasions is less than 5 mm, they fall into the category of microplastics (MP). Indeed, together with textile fibers they are deemed to be the most important source of MP released into nature, where a recent assessment has revealed that over a third of MP reaching the aquatic environment is tire and road wear particle (TRWP) derived (Siegfried et al., 2017).
Annual tire wear emission estimates have been published a number of countries (Table 1). Also, tire emissions have been estimated for different types of vehicles. For instance, on urban roads, an average passenger car is estimated to emit between 40 and 360 mg MP/km (Luhana et al., 2004; Hillenbrand et al., 2005) and an average truck 1,000–1,500 mg MP/km (Hillenbrand et al., 2005). However, emissions depend not only on the type of tire/tread, the type of road, speed, and loading of the vehicle, but also on climatic factors (Alexandrova et al., 2007; Goßmann et al., 2021). In addition, in hot climates whole tire failures are more frequent. In areas where macro-tires resulting from whole tire failures are not collected completely, these macro-tires will give rise to secondary micro-tires over time. Baensch-Baltruschat et al. (2021), Kole et al. (2017) give two very good recent overviews on the multifaceted topic of TWRPs. Tire materials can be diverse and inherently complex. Microplastic analysis of TRWP in road dust has been conducted in such diverse locations as Melbourne, Australia (Roychand and Pramanik, 2020), Gothenburg, Sweden (Järlskog et al., 2020), Oldenburg, Germany (Goßmann et al., 2021) Bushehr City (Abbasi et al., 2017) and Asaluyeh County (Abbasi et al., 2019), both in Iran. The studies from Australia and Iran notwithstanding, little is known about tire wear emissions in hot climates.
TWRP emissions include both fine airborne particulates (such as PM10 and PM2.5) and coarse particulates. Here, we only look at the coarse particulates, with diameters above 5 μm. The tire wear particles (TWPs) contain synthetic rubbers made from petrochemical products, leading to a certain hydrophobicity of the materials (National Institute of Environmental Research, 2011). Thus, micro-tires with their large specific surface areas can readily adsorb persistent organic pollutants (POPs) (Bakir et al., 2014). The accumulation of TWPs in the environment may result in the intake of TWPs by organisms, with the possibility of an eventual bioaccumulation and biomagnification of POPs (Bakir et al., 2014). In temperate zones, TWPs often find their way into the water system by being swept up in run-offs, some of which would be handled by wastewater treatment plants. There are several cases of TWPs having been found in sewage treatment facilities (e.g., Kole et al., 2017; Lee et al., 2018).
In this contribution, the accumulation and distribution of tire-wear in the environment of a landlocked city in an arid region was investigated. For this purpose, the region in and around Al Ain City in the United Arab Emirates (UAE) was chosen as the location. The UAE is located between 22°29′ and 26°4′ north latitude and between 51°5′ and 56°23 east longitude in the Eastern part of the Arabian Peninsula, covering an area of 77,700 km2. The UAE climate, especially in the interior, can be classified as a hot (arid) desert climate. July and August are the hottest months, with a maximum temperature of over 49°C having been reported on the coastal plain. January and February are the coldest months of the year, with an average minimum temperature of 10°C to 14°C. Al Ain is the third largest city of the UAE and the second largest within the Emirate of Abu Dhabi with a population of about 767.000. It lies inland, near the Al-Hajar mountains and the Omani border, 120 km from Dubai and 160 km from Abu Dhabi. Although Al Ain lies within an arid region, rains can occur, especially in the winter months, where they can last for a day or two. In the summer, rains are much shorter (10 min.−1 h), usually accompanying a thunder or dust-storm. There were four such rain events in the main study period of July-August 2020.
While UAE coastal run-offs into the Arabic Gulf and the Gulf of Oman have been estimated for recurring (e.g., every 5 years) heavy rainstorm events (Abdouli et al., 2019), run-offs from such an event in land-locked Al Ain will not reach the sea. Some of the rainwater runs into Wadis (creek beds that seasonally carry water) that transverse the city of Al Ain. The Wadis end outside of Al Ain in the desert, where in the case of heavy rainstorm events the water will fan out over a larger area.
The total length of roads in Abu Dhabi’s Al Ain region was over 5.7 thousand kilometers, as of 2019. The total length of all roads in the Abu Dhabi Emirate in that year was around 27 thousand kilometers (Saleh, 2022). The roads near all sampling sites are paved roads and kept in very good condition. As of 2009, the total number of vehicles in Al Ain was 176,584 of which 1142 were motorcycles, 158,970 light vehicles, 2,537 buses (light and heavy), 9,926 light and heavy trucks and 4,008 heavy mechanical equipment (Statistics Centre - Abu Dhabi, 2010).
For this study, in the summer 2020, road dust and soil samples were taken from 34 locations and screened for micro-tires. The locations included soil and road dust samples from a wide variety of locations with different traffic volumes in- and outside the confines of Al Ain. Specifically, soil samples were taken from next to a highway, a city thoroughfare, a major truck road, a farm access road and streets in residential areas. Road dust samples were taken from residential streets, from a major truck road, a 2-lane road on the outskirts of the city and from one parking lot in a residential area. Also, soil samples were taken from three Wadis and one artificial lake as the examples of locations in Al Ain that have surface water, at least intermittently. In addition, meso-tire particles and fragmented mud-flap rubber were investigated in view of changes in their physical properties, especially their mechanical properties, as a function of their exposure to the aridity and the outdoor temperatures of an Emirati summer. Meso-tire particles and rubber from mud-flaps were part of this study as in Al Ain these frequently accumulate on the road and at the road side. In separate experiments, the potential of meso-, and micro-tires, collected as TWP from a road, to fragment into smaller pieces was studied when rolled over by a vehicle. Also, the fate of two TWP, a meso-tire particle and a fragmented mud-flap rubber, was studied on a private road over a duration of 8 weeks. These fragmentation experiments were deemed important as it was thought that TWP might well fragment at a different rate in hot arid conditions than in more temperate zones.
Thespecific points to be addressed were: What is the micro-tire load in soils and road dust in a typical mid-sized land-locked city on the Arabian Peninsula and do micro-tires penetrate the environment, especially open water sources? What role does temperature and aridity play in the fragmentation of tire particles to micro-tires? Does water in arid regions with infrequent rainstorms water still play a role in the dissemination of microtire particles or are other modes of microparticle transport more important?
The main sampling was carried out in June 2020—August 2020. August 2020. The sampling pool includes eight road dust samples 11 samples of soils along roadsides 13 samples from Wadis and a lake and 2 storm water samples (Map, Figures 1, 2). The sampling sites includes the following locations: (Al Murahibeen St–Al Muwaij’i (S1, S4), Al Ghawwas St (S2) 1st St–‘Asharij (S3), Mohammed Bin Zayed The First St–zAl Towayyah–Abu Dhabi (S5), E40–Industrial City–Abu Dhabi (S6-S8), Al Markhaniyyah–Abu Dhabi Wadi (S9), Zakher Lake (S10, S19), Asharij-UAEU, (S11), University street-'Asharij-UAEU, (S12, S23), Zakher–Abu Dhabi (S13), E95-Nahil Road (S14), E66-Al Ain Dubai Road (S15), Dubai Al Ain Road (S16), Green Mubazzrah (S17), E30- al Rawdha Road (S18, S20), Al Sad–Abu Dhabi (S21), Abu Dhabi–Al Ain Rd–Al Salamat (S22), Al Jahili–Abu Dhabi Wadi (S24), Al Towayyah–Abu Dhabi (Wadi) (S25-S34).
FIGURE 1. Study area and sampling locations in Al Ain, Abu Dhabi Emirate (Eastern region), United Arab Emirates.
FIGURE 2. A view of the different sampling sites (A) an artificial Zakhar Lake (B) a wadi passing through the city area (C) speed bump (D) road shoulder of a highway located outside the city skirts (E) road shoulder of 2-lane road located inside the city (F) pristine desert (G) shoulders of a truck road optically divided into inner and outer zones (H) a sand dune with embedded gravels, microplastics and tires pieces (I) evidence of microplastics and tires pieces embedded in the sand dune.
A short description of the sites is as follows:
S1-S4: quiet residential areas within Al Ain City–the road dust was collected near speed bumps (S1-S3) and from a side parking on a residential road (S4)
S5: Soil collected next to a main through way.
S6-S8: Road dust/debris collected from the verge of the old truck road from Al Ain to Abu Dhabi, which has a high density of mostly heavy trucks.
S9: Soil sample taken from a dry Wadi within the City confines. Two small service roads run parallel to the Wadi. Near the sampling site, a small road passes over the Wadi via a small box girder bridge.
S10, S19: Zakher lake is a man-made lake within the city confines of Al Ain, about 17 km from the city center. The old truck road, which connects Al Ain with Abu Dhabi, runs by the lake at a distance of 300 m. Except for a farm, no housing or economic activity exist within 500 m of the lake. The lake has been created by the municipality through the discharge of treated industrial sewage water and road surface runoff stormwater. In the last 5 years, no water has been added artificially, and in recent years the lake has decreased in size significantly. Sand dune driving and horse-riding activities have been noticed around the lake.
S11: Stormwater sample taken from a run off from a medium travelled road.
S12: Stormwater sample taken from a run off from one of the UAEU parking lots.
S13: 3 soil samples from a desert location 50, 100, and 150 m away from a dead-end road.
S14: Soil sample taken from the side of a well-travelled road which connects Al Ain to Sweihan.
S15: Soil sample taken from the side of a less travelled road connecting Al Ain City with Al Foah.
S16: Soil sample taken from the side of a 6 lane* way highway with heavy traffic.
S17: Road dust sample taken from the roadside of a 2-lane road.
S18, S20: Soil sample taken from the side of a medium-travelled 2 lane road.
S21: Soil sample taken from the side of a 2-lane farm access road.
S22: Soil sample taken from the side of a 6-lane highway (Al Ain-Abu Dhabi)
S23: Soil sample taken from a medium frequented 4 lane road.
S24: Soil sample taken from a Wadi bed. The Wadi is within the city and has two service roads running parallel to it. It is near a commercial area.
S25-34: Al Towayyah Wadi is a large creek bed that originates in Oman and transverses Al Ain (east to west). A 6-lane highway runs parallel to the Wadi for 25 km from the Omani border westward. However, at the collection points S22-S24 the nearest parallel running highly frequented road is a 4-lane road 500 m away from the Wadi. Midway is a highly frequented 4-lane road crossing the Wadi via a high box girder bridge.
*here, number of lanes is counted as the overall number. A 4-lane road has two lanes going in each direction.
Soil (approx. 30 g) was collected from the top soil up to a depth of 2 cm with a Lotus Star trowel, model 26375. The collected road dust and soil samples were carefully placed into air-tight, low density polythene ziplock bags and sealed. Before transferring the samples, the ziplock bags were analyzed to make sure that they were free of any contamination. All samples in the ziplock bags were carefully transported to the laboratory. A GPS device Garmin (Manotana 680) was used to record the coordinate points of the sampling sites.
Attention was paid during the samplings to cover different road surface sites including side parking, areas near speed bumps, footpaths and road side areas (see above). The road dust samples (S1, S2, and S3) were collected from three locations on three different roads, (S4) from a road side parking, (S6, S7, and S8) from road kerbs and (S17) from a road verge. The soil sample (S5) was collected from a road kerb-side, (S14-S16 and S18 -S23) from the soil along road sides, (S10, S19) from the shore of Zakher lake, (S9, S24-34) from the sediments of the dry beds of 3 Wadis, (S11-12) from road surface rain water runoff. For (S11-S12) the rain water was collected during a rain storm event just before a storm drain in a steel bucket (25 L capacity).
A 1 m2 quadrant frame was used for macroscopic tire sampling (S5). At sampling sites S6, S7, and S8, the road shoulder was optically separated into two sections (Figure 3) one to and the other away from the road lanes, the first covered partially with coarser gravel, the other connecting with the soil of the neighboring countryside. The sampling areas were 8.50 m × 3.40 m [inner shoulder, site S6], 8.50 m × 1.20 m [outer shoulder, (S7)].
FIGURE 3. (A) Tire particles segregated from road dust sample (B) tire particles processed through Fiji ImageJ.
Triplicates of soil and road dust samples were weighed (each 1 g, electronic balance ABT 220-5DM) and the complete sample, very thinly spread, was screened under a stereomicroscope (Model SZ2-ILST) for micro-tire content. Microphotos were taken of the complete sample and processed with Fiji ImageJ for size distribution analysis (Figure 3). The Ferret’s diameter was used to represent the size of the micro-tires. The Ferret’s diameter corresponds to the longest distance between any two points along the particle’s boundary (Schneider et al., 2012). The storm water (S11/S12) was filtered through a Whatman filter paper (ashless, grade 42, 2.5 μm pore size). The infiltrate was air dried and weighed. The dried filter cake was gently mixed to obtain a cake in granular form and screened under the stereomicroscope. Triplicate samples of dried filter cake (1 g each) were used to screen for micro-tire content.
The microstructural features of a mud-flap and a tire sample were obtained using a JEOL Analytical Scanning Electron Microscope (Model: JSM-6010PLUS/LA, Tokyo, Japan) equipped with a secondary electron imaging mode. The samples were mounted on brass stubs using double-sided adhesive carbon tape as a conductor path and were sputter-coated with gold up to 15 nm thickness using a Cressington 108 auto sputter coater and thickness controller MTM-20. Fields of the sample were inspected under a high-vacuum (ULVAC KIKO lnc, Model: G-100DB, Miyazaki, Japan) and micrographs of the sample were recorded using InTouch Scope JSM software using a power of 20 kV. The elemental composition was examined by JEOL-SEM equipped with an energy dispersive X-ray detector (EDS). FT-IR spectroscopy of micro-tire particles and micro-rubber materials was carried out on material that was finely abraded with a file. The IR spectra of the materials were taken as KBr pellets of the samples with Perkin Elmer Spectrum Two and Thermo Nicolet Nexus 670 FT-IR spectrometers. Attenuated total reflection (ATR) spectra of the rubber samples were performed with a Shimadzu IRPrestige-21 attached with Pike Miracle Single Reflection Horizontal ATR Accessory (Madison WI).
Over 100 days, the fate of a number of rubber (mud-flap)/tire particles sequestered from the road was investigated, both in their natural locations on the road and along the roadside as well as embedded in soil under controlled outdoor laboratory conditions. Temperatures of the surfaces the meso-tires and meso-rubbers were resting on or embedded in were measured with an ikon IK-T2020 non-contact infrared thermometer. The temperature readings were compared with those of an Easy-Read® general purpose liquid-in-glass laboratory thermometer.
Measurements showed that in the time July-August temperatures of rubber pieces lying on the ground exceeded 70°C for about 3 h on a daily basis. The measured record surface temperature was 78.9°C. The rubber pieces cooled down to just above 36°C at night, allowing for a 4°C difference between highest and lowest temperature after sunset, with an overall maximum temperature difference of 42.8°C (Supplementary Figures S4). Mean temperatures in UAE are expected to rise over the next decades (AlSarmi and Washington, 2014; Elhakeem and Elshorbagy, 2015). How this will affect the overall precipitation is not clear, however, a trend towards a more frequent occurrence of extreme rainfall events is noted (Chowdhury et al., 2016). High surface temperatures in the summer could result in the dissipation of small organic molecules from tire and rubber material such as oils and plasticizers, making them more brittle, leading to a more facile mechanical fragmentation into smaller particles.
The tire dispersal through air movement was investigated by using 24 meso-tire and 16 micro-tire particles of different sizes and mass. The tire particles were placed in an open desert location near Towayyah Wadi and monitored on a daily basis for 21 days (for meso-tire particles)/7 days (for micro-tires) to measure the displacement of each particle with time. The wind speed was measured using an anemometer (SKEIDO GM816 Digital Anemometer). A position was marked as point zero as a reference used to measure the relative distance travelled by each tire particle.
Hardness tests were performed on old and fresh rubber (mud-flap)/tire pieces. The hardness tests were conducted according to the ASTM D2240 standard using a Shore-D durometer. The tests were used to determine the material’s resistance to surface indentation (Mourad, 2010). The tensile tests were conducted to determine the highest load value that the material can bear before failure (Djakhdane et al., 2016; Babaghayou et al., 2020). The tensile tests were conducted at 5 mm/min crosshead speed using an Instron Universal Testing Machine with 50 kN maximum load capacity.
Road dust samples were taken from residential roads near speed bumps, from a parking lot, from a two-lane road and from route E30, a heavily travelled road outside of Al Ain. Table 2 illustrates the micro-tire abundance/g and size distribution in road dust samples. The highest abundance of micro-tires in road dust samples was found in samples collected near speed bumps (S1-S3) with an average of 73.5 ± 42.6 micro-tires/g. The variation in the number of micro-tires for speed bump sampling sites (S1-S3) was related to road sweeping activity. A bi-weekly road sweeping activity was recorded for the sampling sites S1 and S2, but there was no road sweeping at sampling site S3 during the whole study period. Speed bumps are built to serve to slow down cars in residential areas, before or at crossings without traffic lights and in front of the entries/exits to/from buildings of importance. Both the deceleration before and the acceleration of vehicles after the speed bumps lead to more wear and tear of tires as compared to areas on roads where vehicles travel at more or less constant speed. Therefore, it was not surprising that these sites had the highest count of micro-tires among collected the road dust samples. With 54.3 particles/g, slightly fewer micro-tires were found at a parking lot within a residential area. Also, road dust from the roadside of a two-lane road leading to Green Mubazzarah, a recreational area, showed with 22 particles/g a lower micro-tire concentration.
E30 is one of the main highways in the United Arab Emirates and connects the two big cities of Al Ain and Abu Dhabi. It mostly serves trucks and therefore is locally known as Abu Dhabi-Al Ain Truck Road. A lot of tire debris, including macro- and meso-tires were found on the shoulders of the truck road. There is no road sweeping activity on this road. The road shoulders were optically separated into an inner shoulder and an outer shoulder (Figure 3). The size range of tire pieces found on the inner shoulder was 10.54–544.4 mm (Table 3) with a mean average size of 96.1 ± 85 mm, whereas the size range of all tire particles on the outer shoulder was 0.68–549.1 mm with a mean average size of 51.62 ± 57.82 mm. Thus, the inner shoulder had heavier and larger sized tire particles as compared to the outer shoulder (for S8: [F1 (1, 258) = 8.87, p = 0.0032]). The size and abundance of specifically micro-tires was also assessed by collecting samples (S6-S8) from the road. The inner shoulder was found to have fewer micro-tire particles than the outer shoulder. The size range was also significantly different between inner and outer shoulder. The samples showed fewer micro-tires (with an average of 10.7 micro-tires/g over all samples) than the other road dust sampling sites. The smaller size and higher abundance of tire particles on the outer shoulder when compared to the inner shoulder indicates that small sized tires can be carried away from the road with the air pressure generated by the vehicles. The truck road is found outside the city confines and is affected regularly by winds. This raises the possibility that winds may indeed carry away the rubber/tire particles and disperse them within the surrounding area (see also 3.3). A photo (Figure 2J) taken at a site located 50 m away from sampling site S7 off the truck road provides evidence of tire particles embedded in a sand dune and seems to indicate that such a dispersal of micro-tires takes place. An additional factor in regard to the tire dispersal could be that the road is slightly elevated over the surroundings.
TABLE 3. Micro-tire size range, mean size and number of tire particles/per soil location along road side.
Overall, the mean average size of micro-tires found from all collected road dust samples was 108.5 μm ± 108.3 µm with a size range of 19.9 to 2109.9 µm. Only 16% of micro-tires in all 9 road dust samples had a size less than 50 μm, while 45.7% had a size between 50 and 100 µm. Only, 3.4% micro-tires were found to have a size more than 300 µm. The average abundance of micro-tires from all road dust samples was found 41.5 ± 37.7 particles/g sample. A high variation in number of micro-tires/g samples was found among the road dust sampling sites. Our results showed a higher abundance of micro-tires in road dust as compared to samples taken by Abbasi et al. (2019) in Asalyyeh, Iran, (16.7 micro-tires/g sample) but a lower abundance than in samples taken in Bushehr City, Iran (78.2 micro-tires/g sample, Abbasi et al., 2017).
Soil samples alongside roads were taken from a number of locations that included sites next to highways (S16, S22), next to heavily (S14), medium (S23) and lightly travelled (S15) two and four lane roads as well as next to a farm access road (S21). Here, it was interesting to see that a higher traffic density of a road did not translate directly to a higher density of micro-tires in the soil alongside the road. This can be seen when comparing the micro-tire density in the soil next to a farm-access road (19 particles/g soil) with that alongside a highway (13 particles/g). Variations in the micro-tire density cannot only be attributed to the location of the sampling site and the utilization of the road, but also to the road structure, and whether sweeping activities are performed on a regular basis. The overall size distribution graphs for all 34 sampling sites are given in Supplementary Figures S1.
The micro-tire count varied from 2 particles/g to 148 particles/g soil. The maximum abundance (148 particles/g) of micro-tires was found in soil along a heavily traveled road (S5) located inside the city area. The location was located next to a sidewalk, 3 m away from the road. There is also a service road running parallel to the road in 7 m distance to the sampling site. There is no regular road sweeping activity near this site. Overall, the mean average size of micro-tires from all soil samples along the roads was 106.5 ± 79.7 μm with a size range of 18.8–742.9 μm. 16% of the micro-tires found in the sampled soils along the roads had a size less than 50 μm, whereas the majority of the micro-tires (41.6%) were found to have a size range of 50 to 100 μm. Only 3.1% of the micro-tires from all 11 soil samples were found to have a size above 300 μm.
Overall, the micro-tire concentrations in soils alongside roads are also high, albeit with variations from location to location. The worry is that micro-tires deposit on crops that are grown near to the roads. In the residential areas of Al Ain, oftentimes vegetables are planted under palm trees alongside the roads. Also, farms are directly located alongside highways outside of the city confines. The soil collected from a farm access road (S21) was notably contaminated with micro-tires, also.
In principle, micro-tires can move with water (Unice et al., 2013) and with air (Evangeliou et al., 2020). Even in semi-arid regions, water has been found to be a mode of transport (Piñon-Colin et al., 2019) for micro-tires. The role of rain water runoff for micro-tire dispersal was assessed by collecting rain water run-off samples from two different sampling sites (S11-S12). The samples were filtered to obtain the micro-tire particles. The analysis revealed an average of 0.31 micro-tires/L of storm water for samples (S11-S12) (Table 4). The fate of the micro-tires in rain water runoff depends upon whether the storm water is being treated and is run through a wastewater treatment plant before its disposal (Kole et al., 2017; Lee et al., 2018). In Al Ain, the storm water drainage is entirely separated from the wastewater and storm water is directly discharged into Wadis that are specifically used to collect the storm water in case of heavy rainstorms, where lastly the water seeps into the ground outside of the city in an open desert area.
The role of storm water in micro-tire dispersal was also investigated by collecting sedimentary soil samples (S9, S10, S19, S24-S34) from the bed of three different wadis and two samples (S10-S13) from the shore of Zakher lake, an artificial lake that has been created from treated wastewater. The analysis results of the collected samples (S9, S10, S19, S24-S34) and (S10-S13) indicate very few tire particles (Tables 4, 5). This seems to demonstrate that rain water only plays a limited role in the relocation of micro-tires in the environment.
For the few micro-tires found in the Wadis and Zakher lake, the mean average size was found to be 77.8 ± 80.3 µm with a size range of 12.5–377.8 µm. The majority of the micro-tires (53.7%) had a size of less than 50 µm. Only 2.4% of the micro-tires had a size above 300 µm.
To better understand the movement of tire particles with the air, the Aeolian dispersal of both meso-tire and micro-tire particles was studied. For this, 24 meso-tire particles with an average weight of 314 mg and an average length of 1.92 cm were placed in an open desert area near Al Ain and their displacement was studied over a period of 21 days. No severe weather event occurred during this time, where the average wind speed during the study period (21 days) was found to be 16.7 km/h. Photos of the tires were taken on a daily basis. The photos were used to measure the displacement of each tire particle. The results indicate that overall, the lighter weight tire particles dispersed farthest (Table 6). The mean average distance travelled by all tires was 11.1 cm. The maximum distance travelled by a tire particle was 41.1 cm and the minimum distance was 3 cm. Overall, all tires showed a displacement within 21 days (Supplementary Figures S5, S6). A similar experiment of 7-day duration was carried out with 16 micro-tires with an average weight of 150 mg and an average length of 4.2 mm. Here, the maximum distance travelled by a tire piece was 57 cm in 7 days, whereas the average distance travelled was 17 cm.
Soil sampling sites (S13), located in an open desert area, off a dead-end road, were selected to be able to see whether there would be appreciable dispersal of micro-tires away from roads. Three soil samples were collected 50 m apart from each other (Sample 13a, 50 m away from the road, Sample 13b, 100 m and sample 13c, 150 m away from the road). The analysis revealed 3 micro-tires/g 50 m away from the road, 1 micro-tire/g 100 m away, but no micro-tires were found in the samples collected 150 m away from the roadside (Table 3). The size distribution of the identified micro-tires varied with the distance from the road, i.e., the particles found at 100 m from the road were on the average smaller than those found 50 m away. This indicates that the smaller the size of the particles the more the chances are to be moved by the wind.
In regard to the micro-tire distribution along roads on the outskirts of Al Ain, the role of possible Aeolian dispersal of micro-tires was already discussed in Section 3.1 for sampling locations (S6-S8). Overall, there is some dispersion of micro-tires into the “hinterland” that brings the danger of deposition of micro-tires on arable soil.
Apart from tire fragments a main source of rubber particles on the roads in the United Arab Emirates comes from slipped mud-flaps of trucks. It was noted that in the summer, soil and road surface temperatures can reach more than 70°C (see 2.5). This leads to the desiccation of tire/rubber wear found on and alongside the roads. In order to understand this process better, a macro-tire particle and a macro-particle stemming from a mud-flap, both found newly shed on the road, were placed on a soil surface in an open space within the university campus, and their weight was monitored over the time of 10 days for the tire particle and of 98 days for the mud-flap piece. Prior to this experiment, the tire and the mud-flap particle were subjected to XRD and SEM-EDS analysis (Supplementary Figures S8, S9) to have a better understanding of their composition. Both tire and mud-flap are composite materials, combining organic and inorganic compounds. In the EDS, the mud-flap exhibited significant calcium content due to the presence of calcium carbonate (CaCO3) in its composition as evidenced by the XRD spectrum. A FT-IR spectrum of the mud-flap rubber sample also showed the presence of CaCO3 (i.e., with absorption bands at 1436, 874, and 719 cm−1). In addition, the silicon content with 6.99 mass% was found to be relatively high, most likely due to the presence of silicone in the mud-flap. Silicon and calcium contents were much lower in the tire particle. In the tire particle, zinc (2.0 w%) was found. Zinc in form of zinc oxide is used as a catalyst in the vulcanization process of tires and zinc has already been suggested to be used as a marker for the detection of micro-tires (Klöckner et al., 2021; Müller et al., 2022). Typically, tires as well as mud-flaps also possess small organic molecules in form of oil constituents and plasticizers. It is the loss of these small molecules apart from the loss of moisture that would lead to weight reduction of the particles in the high temperatures of the UAE summer. In the experiment, it was found that the mud-flap particle lost 6.12% of its weight after 98 days and was continuing to lose weight even after this time, albeit at a much-reduced rate. On the other hand, the tire particle lost much of its weight during the first day, and after 10 days weight loss was incremental. With 0.378 w%, the tire particle lost much less weight than the mud-flap particle. The loss of small organic molecules turn rubber and micro-tire particles into porous and brittle structures that can easily fragment into many smaller particles.
To better understand the mechanical fragmentation of tire particles, old tire particles were collected from shoulders of truck road E30 (S6). The weight and size of the tire particles were measured and the particles were distributed into three ziplock bags, with the bags at the end containing 60, 50, and 40 pieces, respectively. The initial size distribution of the tire pieces in the bags is given in Supplementary Figure S2. A car having a weight of 1670 kg was driven over each bag 10 times at a speed of 40 km/h. The bags were brought into the laboratory, and the tire contents were placed into a Petri dish for quantification and characterization. The fragmented tires from each bag were photographed by using a stereomicroscope (Model SZ2-ILST) (Figure 4). For counting and determining the size distribution of the resulting tire particles, the photos were analyzed utilizing ImageJ software. A comparison of the size distribution of the micro-tires, before and after, clearly shows the fragmentation of the micro-tires into particles of much smaller size (Supplementary Figure S2A,B).
The mean average size of the hundred micro-tires that were collected from the road originally and used for the experiment was 638.1 um, while the mean average size of the particles after being run over ten times was 44.6 um. Size distribution graphs of the micro-tires, before and after, are given in Supplementary Figure S2G.
Also, the fate of a meso-rubber from a mud-flap and a meso-tire piece newly shed on a private road with an average car count of 2500 cars/day was followed for 8 weeks. The results revealed fractures and fragmentation in the rubber piece, however, the tire piece did not undergo a significant change (Figures 5A,B). Microscopic photos of newly abraded mud-flap rubber revealed a cracked surface with a shiny appearance that might reflect leakage from additives, such as oils, incorporated in the tire manufacturing process. The shiny appearance only lasted for a short time, after which it started fading. Overall, the results revealed that meso-tire particles take time to fragment into smaller pieces (see above, and Figure 6). Should tire pieces remain on the road for a long period of time in the arid conditions of the Arabian Peninsula, they become brittle and it is then that they fragment into smaller pieces relatively easily.
FIGURE 5. (A) Micro-photo of a rubber pieces before the tire abrasion experiment (B) micro-photo of the same rubber piece after the end of 8 weeks of experiment.
FIGURE 6. The display of a rubber particle from a mud flap (top row) and a tire particle (bottom row) from day 1 to week 8.
Although the composition of the volatiles exuded by the tires under the high temperatures of the Emirati environment has not been studied, there is a worry that they can impact the environment. This may necessitate additional research.
Three groups of rubber samples stemming from a truck mud-flap were subjected to hardness and tensile tests. Group 1 samples were not exposed to the Sun, while group 2 and 3 samples were exposed to the Sun in the open environment for 1.5 and 15 months, respectively. The hardness tests for all the three sample groups provided consistent results, with average values of 37, 42, and 55 with a maximum deviation of ±2 for groups 1, 2, and 3, respectively. This clearly indicates that group 1 has the lowest hardness, group 2 has an intermediate value, and group 3 has the highest value. This shows that, exposure to Sun light, increases the materials’ hardness and, with that, brittleness. The relationship between hardness and wear resistance can be divided into three categories for elastomers, depending on the hardness ranges: soft, intermediate, and hard elastomers. Hill et al. (1997) have investigated the wear resistance for polyurethane elastomers. They have suggested that for soft polyurethane elastomers (60 A–75 A) increasing hardness decreases the wear rate, for intermediate hardness polyurethane elastomers (75 A–95 A), increasing hardness has no effect on the wear rate, and for hard polyurethane elastomers (95 A–65 D), increasing hardness increases the wear rate. For the purpose of comparison, the hardness conversion relationship for polyurethane materials is 85 A = 36 D and 93 A = 44 D (Hill et al., 1997).
Three tensile tests were conducted with the samples at 5 mm/min crosshead speed using an Instron Universal Testing Machine with 50 kN maximum load capacity. The tensile tests were conducted to determine the highest load value that the material can bear before failure (Djakhdane et al., 2016; Babaghayou et al., 2020). All the tests have provided consistent results, with an average yield strength of 1.70 MPa and an average tensile strength (fracture strength) of 2.62 MPa.
As stated above, rubber products are usually made up of a mixture of natural and synthetic rubbers as well as a combination of additives, which makes it difficult to know the exact composition of a commercial rubber product. It is difficult to know the exact service conditions of the used rubber material under consideration, and the degradations it has faced. Nevertheless, for the purpose of comparison, the tensile and hardness values of the samples tested are compared with the elastomer database from the ANSYS Granta EduPack, and are summarized in Tables 1, 2 (Supplementary Material, p. 16), respectively.
It can be said that the hardness and brittleness of the rubber increases significantly with the time of exposure to the temperatures and solar irradiation typical for the Emirati summer.
Road dust and soil samples alongside roads were collected from 34 sampling sites within the region of Al Ain, Eastern region of Abu Dhabi Emirate, United Arab Emirates. The size range of the tire particles collected from the sampling sites was found to be between 12.52 and 2109.8 µm. The majority (44.2%) of micro-tires have a size range between 50 and 100 µm. 17% had a size less than 50 µm and only 3.2% micro-tires had a size larger than 300 µm. The mean average size of the collected micro-tires (S1-S34) was found to be 103.5 ± 73.8 µm.
Rare storm events transport tire/rubber particles into storm drains, however, the major mode of transport for the particles is through air movement. Appreciable micro-tire concentrations can be found up to 50 m off-road, however, the study has shown that water bodies (one artificial lake and three Wadis) that are more than 200 m away from roads are relatively free of micro-tires.
High surface temperatures of the Emirati summer result in the dissipation of small organic molecules from tire and other rubber materials on roads such as oils and plasticizers, making the particles brittle, leading to their facile mechanical fragmentation into smaller particles. The composition of the volatiles under the ambient conditions in the UAE and similar regions and their effect on the environment should be studied further.
The original contributions presented in the study are included in the article/Supplementary Material, further inquiries can be directed to the corresponding author.
RH: Sampling, investigation, writing original draft, validation, visualization, RA: Writing, editing. FG: Formal data analysis, data curation. SI: Investigation of mechanical properties, writing. VP: Thermal analysis. TR: XRD and SEM/EDS measurements A-HM: Investigation of mechanical properties, editing. ME: Formal data analysis, data curation. FH: Writing, editing TT: Writing, review and editing, supervision, project administration.
The authors thank Mohnnad Kordi and Hazem Ali for the collection of samples S14–S23.
The authors declare that the research was conducted in the absence of any commercial or financial relationships that could be construed as a potential conflict of interest.
All claims expressed in this article are solely those of the authors and do not necessarily represent those of their affiliated organizations, or those of the publisher, the editors and the reviewers. Any product that may be evaluated in this article, or claim that may be made by its manufacturer, is not guaranteed or endorsed by the publisher.
The Supplementary Material for this article can be found online at: https://www.frontiersin.org/articles/10.3389/fenvs.2022.1009802/full#supplementary-material
Abbasi, S., Keshavarzi, B., Moore, F., Delshab, H., Soltani, N., and Sorooshian, A. (2017). Investigation of microrubbers, microplastics and heavy metals in street dust: A study in Bushehr city, Iran. Environ. Earth Sci. 76, 798. doi:10.1007/s12665-017-7137-0
Abbasi, S., Keshavarzi, B., Moore, F., Turner, A., Kelly, F. J., Dominguez, A. O., et al. (2019). Distribution and potential health impacts of microplastics and microrubbers in air and street dusts from Asaluyeh County, Iran. Environ. Pollut. 244, 153–164. doi:10.1016/j.envpol.2018.10.039
Abdouli, K., Hussein, K., Ghebreyesus, D., and Sharif, H. O. (2019). Coastal runoff in the United Arab Emirates. The hazard and opportunity. Sustainability 11, 5406. doi:10.3390/su11195406
Alexandrova, O., Kaloush, K. E., and Allen, J. O. (2007). Impact of asphalt rubber friction course overlays on tire wear emissions and air quality models for Phoenix, Arizona, airshed. Transp. Res. Rec. 2011, 98–106. doi:10.3141/2011-11
AlSarmi, S. H., and Washington, R. (2014). Changes in climate extremes in the arabian Peninsula: Analysis of daily data. Int. J. Climatol. 34, 1329–1345. doi:10.1002/joc.3772
Babaghayou, M. I., Mourad, A.-H. I., and Cherunurakal, N. (2020). “Anisotropy evaluation of LDPE/LLDPE/PIB trilayer films,” in 2020 advances in science and engineering technology international conferences (ASET) (IEEE), 1–3. doi:10.1109/ASET48392.2020.9118263
Baensch-Baltruschat, B., Kocher, B., Kochleus, C., Stock, F., and Reifferscheid, G. (2021). Tyre and road wear particles - a calculation of generation, transport and release to water and soil with special regard to German roads. Sci. Total Environ. 752, 141939. doi:10.1016/j.scitotenv.2020.141939
Bakir, A., Rowland, S. J., and Thompson, R. C. (2014). Transport of persistent organic pollutants by microplastics in estuarine conditions. Estuar. Coast. Shelf Sci. 140, 14–21. doi:10.1016/j.ecss.2014.01.004
Chowdhury, C. R., Mohamed, M. M. A., and Murad, A. (2016). “Variability of extreme hydro-climate parameters in the NorthEastern region of united Arab Emirates,” in 12th international conference on hydroinformatics (South Korea: HIC 2016), 639–634.
Councell, T. B., Duckenfield, K. U., Landa, E. R., and Callender, E. (2004). Tire-wear particles as a source of zinc to the environment. Environ. Sci. Technol. 38, 4206–4214. doi:10.1021/es034631f
Djakhdane, K., Dehbi, A., Mourad, A.-H. I., Zaoui, A., and Picuno, P. (2016). The effect of sand wind, temperature and exposure time on tri-layer polyethylene film used as greenhouse roof. Plastics Rubber Compos. 45, 346–351. doi:10.1080/14658011.2016.1198860
Elhakeem, A., and Elshorbagy, W. (2015). Hydrodynamic evaluation of long-term impacts of climate change and coastal effluents in the Arabian Gulf. Mar. Pollut. Bull. 101, 667–685. doi:10.1016/j.marpolbul.2015.10.032
Evangeliou, N., Grythe, H., Klimont, Z., Heyes, C., Eckhardt, S., Lopez-Aparicio, S., et al. (2020). Atmospheric transport is a major pathway of microplastics to remote regions. Nat. Commun. 11, 3381. doi:10.1038/s41467-020-17201-9
Goßmann, I., Halbach, M., and Scholz-Böttcher, B. M. (2021). Car and truck tire wear particles in complex environmental samples – a quantitative comparison with “traditional” microplastic polymer mass loads. Sci. Total Environ. 773, 145667. doi:10.1016/j.scitotenv.2021.145667
Hill, D. J., Killeen, M., O’Donnell, J., Pomery, P., St John, D., and Whittaker, A. (1997). Laboratory wear testing of polyurethane elastomers. Wear 208, 155–160. doi:10.1016/S0043-1648(96)07514-X
Hillenbrand, T., Toussaint, D., Böhm, E., Fuchs, S., Scherer, U., Rudolphi, A., et al. (2005). Einträge von Kupfer, Zink und Blei in Gewässer und Böden—analyse der Emissionspfade und möglicher Emissionsminderungsmaßnahmen. Germany: Umweltbundesamt: Dessau.
Järlskog, I., Strömvall, A.-M., Magnusson, K., Gustafsson, M., Polukarova, M., Galfi, H., et al. (2020). Occurrence of tire and bitumen wear microplastics on urban streets and in sweepsand and washwater. Sci. Total Environ. 729, 138950. doi:10.1016/j.scitotenv.2020.138950
Klöckner, P., Seiwert, B., Weyrauch, S., Escher, B. I., Reemtsma, T., and Wagner, S. (2021). Comprehensive characterization of tire and road wear particles in highway tunnel road dust by use of size and density fractionation. Chemosphere 279, 130530. doi:10.1016/j.chemosphere.2021.130530
Kole, P. J., Löhr, A. J., Van Belleghem, F., and Ragas, A. (2017). Wear and tear of tyres: A stealthy source of microplastics in the environment. Int. J. Environ. Res. Public Health 14, 1265. doi:10.3390/ijerph14101265
Lassen, C., Hansen, S. F., Magnusson, K., Hartmann, N. B., Jensen, R., Nielsen, P., et al. (2015). Occurrence, effects and sources of releases to the environment in Denmark. Copenhagen: Environmental Protection Agency. DanishAvailable at: http://mst.dk/service/publikationer/publikationsarkiv/2015/nov/rapport-ommikroplast.
Lee, J., Kim, E., Han, G., Tongco, J. V., Shin, S. G., and Hwang, S. (2018). Microbial communities underpinning mesophilic anaerobic digesters treating food wastewater or sewage sludge: A full-scale study. Bioresour. Technol. 259, 388–397. doi:10.1016/j.biortech.2018.03.052
Luhana, L., Sokhi, R., Warner, L., Mao, H., Boulter, P., McCrae, I., et al. (2004). Characterization of exhaust particulate emissions from road vehicles (particulates); FP5 Particulates Project. Available at: http://ec.europa.eu/transport/%0Aroadsafety_library/publications/particulates_d2.pdf.
Magnusson, K., Eliasson, K., Fråne, A., Haikonen, K., Johan, H., Olshammar, M., et al. (2016). Swedish sources and pathways for microplastics to the marine environment. IVL Swed. Environ. Res. Inst. C 183 (C), 8.
Milani, M., Pucillo, F. P., Ballerini, M., Camatini, M., Gualtieri, M., and Martino, S. (2004). First evidence of tyre debris characterization at the nanoscale by focused ion beam. Mat. Charact. 52, 283–288. doi:10.1016/j.matchar.2004.06.001
Mourad, A.-H. I. (2010). Thermo-mechanical characteristics of thermally aged polyethylene/polypropylene blends. Mat. Des. 31, 918–929. doi:10.1016/j.matdes.2009.07.031
Müller, A., Kocher, B., Altmann, K., and Bruan, U. (2022). Determination of tire wear markers in soil samples and their distribution in a roadside soil. Chemosphere 294, 133653. doi:10.1016/j.chemosphere.2022.133653
Piñon-Colin, T., Rodriguez-Jimenez, R., Rogel-Hernández, E., Alvarez, A., and Wakida, F. T. (2019). Microplastics in stormwater runoff in a semiarid region, Tijuana, Mexico. Sci. Total Environ. 704, 135411. doi:10.1016/j.scitotenv.2019.135411
Roychand, R., and Pramanik, B. K. (2020). Identification of micro-plastics in Australian road dust. J. Environ. Chem. Eng. 8, 103647. doi:10.1016/j.jece.2019.103647
Saleh, S. (2022). Length of roads in the Abu Dhabi greater region from 2015 to 2019. Abu Dhabi. Available at: https://www.statista.com/statistics/1067783/uae-length-of-roads-in-abu-dhabi-greater-region/.
Schneider, C. A., Rasband, W. S., and Eliceiri, K. W. (2012). NIH image to ImageJ: 25 years of image analysis. Nat. Methods 9, 671–675. doi:10.1038/nmeth.2089
Sherrington, C., Darrah, C., Hann, S., Cole, G., and Corbin, M. (2016). Study to support the development of measures to combat a range of marine litter sources.
Siegfried, M., Koelmans, A. A., Besseling, E., and Kroeze, C. (2017). Export of microplastics from land to sea. A modelling approach. Water Res. 127, 249–257. doi:10.1016/j.watres.2017.10.011
Statistics Centre - Abu Dhabi, (2010). Statistical yearbook of Abu Dhabi 2010. Abu Dhabi. Available at: https://www.scad.ae/Release Documents/Statistical Yearbook 2010_1.pdf.
Sundt, P., Schulze, P.-E., and Syversen, F. (2014). Sources of microplasticpollution to the marine environment. Nor. Environ. Agency Miljødirektoaret 86.
Unice, K. M., Kreider, M. L., and Panko, J. (2013). Comparison of tire and road wear particle concentrations in sediment for watersheds in France, Japan, and the United States by quantitative pyrolysis GC/MS analysis. Environ. Sci. Technol. 47, 8138–8147. doi:10.1021/es400871j
Verschoor, A., Poorter, L. de, Dröge, R., Kuenen, J., and Valk, E. de (2016). Emission of microplastics and potential mitigation measures Abrasive cleaning agents, paints and tyre wear. Netherlands.
Keywords: tires, roadwear, microplastic, tire fragmentation, soil, storm water
Citation: Habib RZ, Al Kendi R, Ghebremedhin F, Elkashlan M, Iftikhar SH, Poulose V, Ramachandran T, Mourad A-HI, Hamed F and Thiemann T (2022) Tire and rubber particles in the environment—A case study from a hot arid region. Front. Environ. Sci. 10:1009802. doi: 10.3389/fenvs.2022.1009802
Received: 02 August 2022; Accepted: 26 October 2022;
Published: 10 November 2022.
Edited by:
Stephan Wagner, Hochschule Fresenius, GermanyReviewed by:
Diego Nogueira, Federal University of Santa Catarina, BrazilCopyright © 2022 Habib, Al Kendi, Ghebremedhin, Elkashlan, Iftikhar, Poulose, Ramachandran, Mourad, Hamed and Thiemann. This is an open-access article distributed under the terms of the Creative Commons Attribution License (CC BY). The use, distribution or reproduction in other forums is permitted, provided the original author(s) and the copyright owner(s) are credited and that the original publication in this journal is cited, in accordance with accepted academic practice. No use, distribution or reproduction is permitted which does not comply with these terms.
*Correspondence: Thies Thiemann, dGhpZXNAdWFldS5hYy5hZQ==
Disclaimer: All claims expressed in this article are solely those of the authors and do not necessarily represent those of their affiliated organizations, or those of the publisher, the editors and the reviewers. Any product that may be evaluated in this article or claim that may be made by its manufacturer is not guaranteed or endorsed by the publisher.
Research integrity at Frontiers
Learn more about the work of our research integrity team to safeguard the quality of each article we publish.