- 1Shenzhen Academy of Environmental Sciences, Shenzhen, China
- 2School of Civil and Environmental Engineering, Harbin Institute of Technology (Shenzhen), Shenzhen, China
- 3College of Civil and Transportation Engineering, the Underground Polis Academy, Shenzhen University, Shenzhen, Guangdong, China
- 4Shenzhen Environmental Science and New Energy Laboratory, Tsinghua-Berkeley Shenzhen Institute, Tsinghua University, Shenzhen, China
In this study, the degradation of bisphenol A (BPA) by ozonation was studied systematically by investigating the effects of different factors, including ozone dosages (0.25–1.50 mg/L), temperatures (10–50°C), initial solution pH (3.0–11.0) and interfering ions. The reaction kinetics were analyzed at the same time. In addition, the generation of intermediates was analyzed and the possible mechanism was proposed by combining with the density functional theory (DFT) calculation. At last, the variation and controlling effect of toxicity was also evaluated. The results showed that ozonation had a stronger degrading ability of BPA (1.0 mg/L). A complete removal efficiency was obtained within 10 min when dosing only 1.0 mg/L ozone. The BPA degradation reactions were well fitted with pseudo-second-order kinetics and could well adapt with the wide range of pH (3.0–9.0), during which over 91% removal of BPA was achieved. The indirect pathway by •OH oxidation was proved mainly responsible for BPA degradation by the scavenging and electron paramagnetic resonance experiments. HCO3−, NH4+ and humic acid showed a certain inhibiting effect. Fe3+ and Cu2+ played a catalytic role on BPA degradation. The DFT calculation has identified that the active regions of BPA was focused at C6, C4, C5 and C1 in terms of radical and electrophilic attack. Thus, combining the results of DFT with GC/MS-MS detection, two degradation pathways of BPA were proposed. Toxicity evaluation showed that, due to the generation and accumulation of more toxic intermediates, values of T% had a sharp increase at initial stage. However, with ozone dosage increasing to 1.50 mg/L, the toxicity could be effectively controlled much lower (5%) than BPA itself (49%).
Highlights
• Ozonation could effectively degrade BPA and control the toxicity of water samples.
• Only 1.0 mg/L of ozone was needed for completely removing of BPA (1.0 mg/L).
• Over 91% removal of BPA was achieved at a wide pH range of 3.0–9.0.
• BPA degradation by ozonation was promoted at the presence of PO43-, Cu2+ and Fe3+.
• The value of T% could increased significantly up to 68% at initial reaction stage.
• The increase of toxicity was due to the formation of more toxic intermediates.”
1 Introduction
Bisphenol A (2, 2-bis (4-hydroxyphenyl) propane) (BPA) is one of the most important raw material, which has been widely used in plastics and eroxy resins industry (Sharma and MishraDionysioukumar, 2015; Yang, 2015). BPA is also a typical endocrine disrupting chemical (EDC). Due to the strong chemical resistance and stable characteristics of BPA, its estrogenic activity can be exhibited at low concentration levels (<1 μg/m3) (Wu, 2008). Toxicological data shows that BPA also has adverse effects on aquatic life, animals, and even human health (Ahmadi et al., 2016; Teppala et al., 2012; Bu and WuHeHuang, 2016; Han and Hong 2016). It can affect the brain development and behavioral differentiation of infants, children and adolescents by long-term exposure. Due to its widespread use and moderate water solubility (Hu et al., 2019), BPA could been easily discharged into industrial wastewater, river, lake, groundwater and other natural water bodies (Trojanowicz et al., 2020; Huang et al., 2012). Therefore, it is necessary to apply effective technologies to degrade BPA and control its biotoxicity.
At present, technologies for BPA degradation have included biological degradation, physical adsorption and advanced oxidation processes (AOPs), etc. Conventional biological (Zielin’ska et al., 2016; Ferro Orozco et al., 2013; Schröder, 2006) as well as physical-chemical treatment techniques (Bhatnagar and Anastopoulos, 2016) cannot satisfactorily remove BPA from water as well as reduce the biological toxicity. In order to conquer the limitations of conventional processes, a lot of AOPs have been developed, such as ozonation (Ahmad et al., 2015), photocatalysis (Reddy et al., 2018; Gao et al., 2020; Li et al., 2022; Luo et al., 2022), photoelectrocatalysis (He et al., 2017), Fenton/photo-Fenton (Xiao et al., 2019) and persulfates or sulfite based oxidation (Wu et al., 2018, 2021, 2022). However, due to the stability and catalytic efficiency of catalysts, photocatalytic oxidation was often restricted in application. In Fenton based oxidation process, the yield of sludge was high. In addition, severe reaction conditions (such as solution pH) were required to achieve stable degradation efficiency (Yang et al., 2016). In contrast, owing to the high oxidative potential of ozone molecule itself (2.08 V) (Kusvuran and Yildirim, 2013) and produced hydroxyl radical (•OH, 2.7 V) (Masten et al., 1994; Garoma et al., 2010), ozonation has been proved as an effective and widely applied method for treating refractory organic pollutants (Quan et al., 2020; Neyens and Baeyens, 2003). It also has the advantages of obvious treatment effect, easy engineering implementation, and easy popularization and application. However, the systematic studies of BPA degradation by ozonation were still limited. It is necessary to apply ozonation for degrading BPA from water.
In the current researches on BPA degradation, most of them have focused on the common influencing factors such as oxidant dosage, initial substrate concentration, pH, temperature, etc (Oguzie et al., 2020; Van Aken et al., 2019; Tay et al., 2012). Since BPA has been widely used in the production of electronic products, the wastewater often contained various complex components such as metal ions and buffer solvents, which were important parameters affecting degradation process. Therefore, it is necessary to investigate the influences of interfering ions in water on BPA degradation. At the same time, most studies have focused on the removal of BPA itself, researches involving the change and control effect of biological toxicity were still relatively insufficient. However, researches have found that more toxic intermediates might be generated during BPA degradation process (Reddy et al., 2018; He et al., 2017; Xiao et al., 2019), which further increased the biological toxicity of the treated wastewater. On the one hand, if the biological toxicity index was ignored, it would be unfavorable for the effective operations of the subsequent biological treatment process. On the other hand, it would pose a threat to the receiving water body and aquatic organisms if the wastewater was directly discharged. Thus, it is of essential to analysis the controlling effect of the toxicity during BPA degradation process. In addition, some possible pathways have been presumed only by identification of the products which was detected through liquid chromatography-mass spectrometry (LC-MS) or gas chromatography-mass spectrometry (GC-MS) [Kusvuran, 2013; Farre et al., 2005], lacking the verification by theoretical analysis and calculations. In recent years, quantum chemical calculation has been verified can explain the reaction from the point of thermodynamic energy. Thus, combining the theory with experiment could comprehensive predict and mutual verify the mechanisms of BPA degrading. In addition, the luminescent bacteria have the advantage of high sensitivity and simple operation during the process of toxicity detecting, which have been applied for testing the integrated and acute toxicity of the reaction samples (Dai et al., 2014; Grimme et al., 2010).
In this study, the performance of ozonation on BPA degradation was systematically investigated under different influencing factors, including ozone dosage, temperature, initial solution pH and interfering ions. The organic intermediates were detected and identified by GC/MS-MS. The possible degradation pathways were proposed based on the products. At the same time, density functional theory (DFT) calculation was employed to analyze the intermediate products and BPA degradation pathways by ozonation. In addition, the variation and control effect of toxicity during BPA degradation was also analyzed.
2 Material and methods
2.1 Materials
All the chemicals were used as purchased without any further purification. BPA with a purity >99% was purchased from Sigma Aldrich and used as received. Humic acid, sodium phosphate, potassium nitrate, hydroxylammonium chloride, 5,5-dimethyl-1-pyrroline N-oxide (DMPO), methanol (HPLC grade) and dichloromethane (HPLC grade) were obtained from Aladdin Industrial Corporation (China). The freeze-dried bacteria Vibro fischeri (V. fischeri, CS234) used for toxicity testing was obtained from SDIX (United States). All solutions were prepared with deionized and ultra pure water (Milli-Q Direct 8, United States). Ozone was produced from purified oxygen by an ozone generator (CF-G-3-10, QingDao GuoLin Co. Ltd., China).
2.2 Degradation experiments
Experiments were performed at room temperature (25 ± 1°C) in 1000 ml conical flasks with BPA solution of certain concentration. The desired solution pH was adjusted by hydrochloric acid or sodium hydroxide solution. The reaction temperature was controlled with ice bath or water bath. The saturated ozone solution was prepared by putting the ozone generated by the ozone generator into pure water for 2 h. According to the required ozone concentration (0.25, 0.50, 1.00, 1.50 mg/L), the calculated volume of ozone solution was added into the conical flask. Then, the conical flask was stirred slightly to ensure the uniform distribution of ozone in the system. The reaction was sampled periodically (0, 1, 2, 3, 5, 10 min) for 25 ml and quenched immediately by adding 30 μL 0.18 M hydroxylamine hydrochloride solution. The samples were then centrifuged (12,000 r/min) by a centrifugal machine (Centrifuge 5418, Eppendorf, Germany) for 5 min prior to subsequent analysis. All the experiments were carried out in duplicate.
2.3 Analytical methods
The concentration of BPA in the reaction solution was determined using a Ultra Performance Liquid Chromatography (UPLC, Waters Acquity H-Class, United States) equipped with a TUV detector at the wavelength of 280 nm. The column was BEHC18 (1.7 × 100 mm, 3.5 µm) with the temperature set at 30 °C. The mobile phase, methanol/water (70/30, v/v), was run in an isocratic mode. The flow rate was maintained at 0.2 ml/min for all runs and the injection volume for UPLC analysis was 5 μL. A detection limit of quantitation was 1 μg/L of BPA and a recovery rate of added standard was 91.5% obtained in this study.
The intermediates of BPA degradation were quantified using gas chromatography with mass spectrometry (GC/MS-MS, 7890A-5975C, Agilent, United States). A HP-5 column (5% phenylmethylpolysiloxane) with the dimension of 30 m × 0.25 mm and 0.25 μm of film thickness was used to separate the organic species with the carrier gas of helium at a flow rate of 1 ml/min. The data for quantitative analysis was acquired in the electron impact mode (70 eV) with scanning in the range of 45–280 m/z. Concentration of dissolved ozone was determined by the indigo colorimetric method (APHA, 2005) using a spectrophotometer (Shimadzu 2450). The solution pH was measured by a portable digital pH meter throughout experiments (pHS-3B, Shanghai, China).
The toxicity of the sample was detected by a DeltaTox II Toxicity Detection System (DeltaTox® II, SDIX, United States), which method was according to the ISO standard based on the inhibition of bioluminescence emitted by the luminescent bacteria V. fischeri (Laws et al., 2000). In addition, the results of this method have been proved that could comprehensively reflect the toxicity of the water samples, which was caused by the target pollutants and their degradation intermediates (Ding et al., 2015). The luminescence intensities (X) of the water samples to the luminescent bacteria during the reaction were detected after contacting for 15 min. Then, the relative inhibitory rate (T%), which could reflect the integrated toxicity of the water samples, was calculated for each sample relative to the control by Eqs. T%=(X0-Xt)/X0*100% (where X0 and Xt represents the luminescence intensity of the water sample to the luminescent bacteria at 0 and t reaction time, respectively).
2.4 Quantum chemical computation
The quantum chemical computation was carried out by using the density functional theory (DFT) with the Hirshfeld population analysis (Delley, 2000; Kusvuran et al., 2010). The molecule of BPA was initially subjected to geometry optimization relying on the B3LYP level. Then, exchange terms of Hartree, Fock and Becke were combined with the Lee-Yang-Parr correlation function. The standard 6-31G * basis was used at the same time. At last, in order to establish the active sites as well as the local reactivity of the BPA molecular, the distribution of Frontier molecular orbitals and Fukui indices was determined.
3 Results and discussion
3.1 Performance of ozonation on BPA degradation
This study evaluated the performance of ozonation process for BPA degradation under different ozone dosages (0.25, 0.50, 1.00 and 1.50 mg/L) and the results were shown in Figure 1A. Other experimental parameters, including BPA concentration, initial solution pH, temperature and oxidation reaction time, were respectively 1.00 mg/L, 7.0, 25 ± 1°C and 10 min. In general, as depicted in Figure 1A, BPA degradation by ozonation revealed a similar two-stage trend. A rapid degradation was obtained at the initial stage, which was followed by a relatively slow and gradually steady stage. When the dosage of ozone increased from 0.25 to 1.00 mg/L, the degradation efficiency of BPA increased significantly from 81.92% to 100%. As the ozone dosage continued to increase to 1.5 mg/L, the time required to completely degrade BPA was shortened from 10 to 5 min, showing ozonation a rapid and highly efficient method. It has been generally proved that there exist two pathways in the ozonation process for pollutants removal, i.e. the direct oxidation pathway by molecular ozone (O3 with a redox potential of 2.08 V) and the indirect one by newly generated radicals (•OH with a redox potential of 2.80 V) (Kusvuran et al., 2010; Wang Y. et al., 2022). Thus, with the increase of ozone dosage, the concentrations of O3 and •OH in the reaction system increased, increasing the effective collision frequencies with BPA (in particular C=C bond connected by two benzene rings and C-O bond connected by benzene rings (Tay et al., 2012; Pan et al., 1984)) and further promoting the degradation efficiency of BPA.
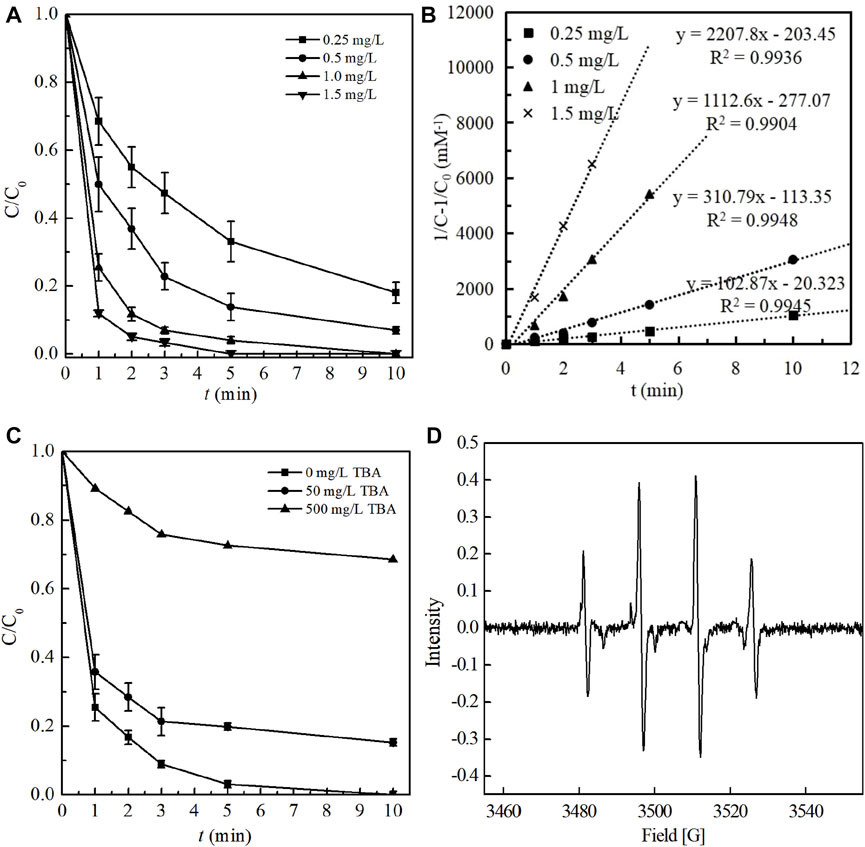
FIGURE 1. (A) Effect of ozone dosage on BPA degradation and (B) Kinetic fitting under different ozone dosage (Experimental conditions: temperature = 25 ± 1°C; initial solution pH = 7.0 [BPA]0 = 1.00 mg/L; ozone dosage of 0.25, 0.5, 1.0, 1.5 mg/L); (C) The free radical scavenging experiments by adding TBA and (D) EPR spectra during ozonation system (Experimental conditions: temperature = 25 ± 1°C; initial solution pH = 7.0 [BPA]0 = 1.00 mg/L; ozone dosage 1.0 mg/L; TBA concentration 0, 50, 500 mg/L).
At the same time, the reactions of BPA with O3 and •OH during ozoantion process could be expressed by the well established pseudo-second-order kinetics under different ozone dosages as follows (as depicted in Figure 3B):
Where kobs, which equals to kOH [·OH] + k O 3 [O3], is the pseudo-second-order rate constant for BPA degradation during ozonation; C0 and C is respectively the initial concentration and the residual concentration of BPA at reaction time t. The results exhibited that kobs increased linearly with ozone dosage (R2 > 0.98) (Supplementary Figure S1). With the increase of ozone dosage from 0.25 to 1.50 mg/L, the value of kobs was obviously promoted from 103.9 to 2207.8 mM−1min−1, revealing that ozone dosage played a critical role in the BPA degradation process by ozonation.
In order to verify the dominant active species responsible for BPA degradation, the free radical scavenging experiments were conducted by respectively adding 50 and 500 mg/L tert-Butanol (TBA), which is well known as the typical scavenger for •OH. The molarity of TBA was 100 and 1000 folds more than BPA and the results were shown in Figure 1C. As the concentration of TBA was 0, 50 and 500 mg/L, the removal efficiencies of BPA after 10 min contacting reaction were respectively 100%, 84.75% and 31.45%, indicating that TBA could significantly inhibit the degradation of BPA in ozonation process. Thus, it could be concluded from the result that •OH was the predominant oxidation species and the indirect pathway was mainly responsible for BPA degradation in ozonation process in this study. The reason might be that in this study, ozone was added in the form of dissolved ozone water, which decomposed more violently and fully, and induced to more free radicals produced.
In addition, to further determine the free radical species produced in the ozonation system, a direct identification analysis was performed using electron paramagnetic resonance (EPR) spectroscopy, which method has been widely used in other similar researches (Wu et al., 2020; Wu et al., 2019). As the existence time of •OH in aqueous solution was very short, DMPO was used to capture •OH so as to form a stable product DMPO-OH with a specific signal peak (Wang et al., 2022b). As seen from Figure 1D, four characteristic peaks with an intensity ratio of 1:2:2:1 was clearly achieved, corresponding to the characteristic peak of •OH. The results further directly proved the existence of •OH.
3.2 Effect of temperature on BPA degradation
The effect of reaction temperature on BPA degradation in ozonation process was investigated by varying the temperature in the range of 10–50°C, other operating conditions including BPA, ozone dosage and initial solution pH were respectively as 1 mg/L, 1 mg/L and 7.0. The degradation efficiencies of BPA and the reaction kinetics were illustrated in Figure 2.
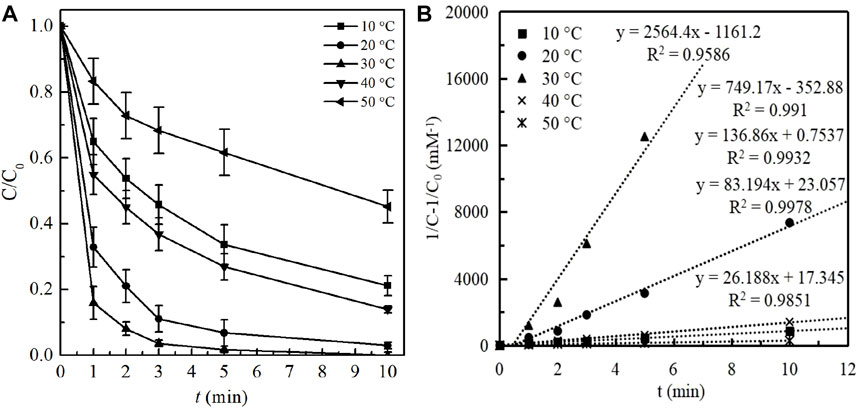
FIGURE 2. (A) Effect of reaction temperature on BPA degradation and (B) Kinetic fitting under different temperature. (Experimental conditions: [BPA]0 = 1.00 mg/L; ozone dosage 1.0 mg/L; initial solution pH = 7.0; temperature = 10, 20, 30, 40, 50°C).
As shown in Figure 2A, the removal efficiency of BPA increased sharply from 78.81% to 100% within 10 min as the temperature increased from 10 to 30°C, which reflected that elevating temperature in a certain range could bring an effective improvement of BPA degradation. This phenomenon has also be explained by other reported researches that the effective collision frequencies of oxidants (ozone molecular and newly formed •OH) with BPA could be improved with the temperature rise, which further contributed to BPA degradation (Pan et al., 1984). This conclusion was also certificated by the values of kobs, which significantly increased from 83.19 to 2564.4 mM−1min−1 as the temperature rose from 10 to 30°C. However, it was worth noting that excessive increase of the temperature would accelerate the decomposition rate of ozone and reduce the amount of oxidants in the solution (Qi et al., 2015), which was unfavorable to BPA degradation. Therefore, as the temperature was 40 and 50°C, the degradation efficiency of BPA was notably decreased to 86.02 and 55.81%. In addition, the corresponding reaction constant kobs was respectively decreased to 136.86 and 26.19 mM−1min−1.
In general, the effect of temperature on BPA degradation by ozonation was primarily attributed to its impact on ozone stability and the effective collision frequencies between oxidants and pollutants. Moreover, it could be seen from the results that the optimal reaction temperatures of this system were 25 and 30°C, under which conditions a complete removal of BPA being both obtained. Considering that additional cost and instability generated from the process of temperature control, the room temperature (25 ± 1°C) was adopted in the subsequent experiments.
3.3 Effect of initial solution pH on BPA degradation
Figure 3 presented the effect of initial solution pH on BPA degradation and the reaction constant kobs in the ozonation process. The experiments were carried out by changing the initial solution pH in the range of 3.0–11.0, with other conditions of [BPA]0 = 1.00 mg/L; ozone dosage 1.0 mg/L; temperature = 25 ± 1°C.
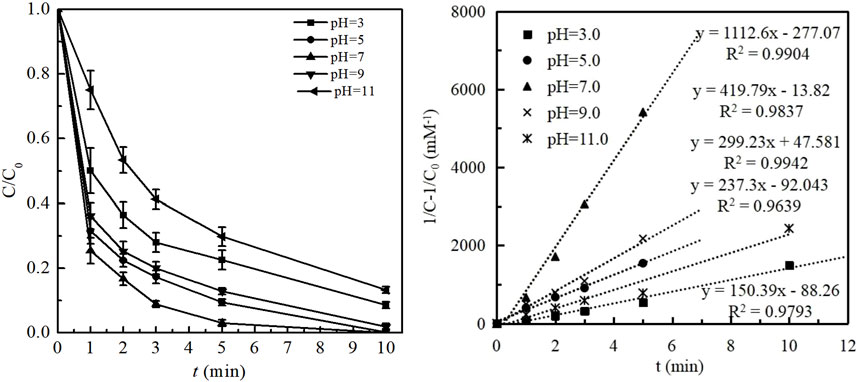
FIGURE 3. (A) Effect of initial solution pH on BPA degradation and (B) Kinetic fitting under different initial solution pH. (Experimental conditions: [BPA]0 = 1.00 mg/L; ozone dosage 1.0 mg/L; initial solution pH = 3.0, 5.0, 7.0, 9.0, 11.0; temperature = 25 ± 1°C).
Generally, as shown in Figure 3A, over 91% removal of BPA was obtained within 10 min in the ozonation process at a wide pH range of 3.0–9.0, indicating the strong adaptability of ozonation technology to initial solution pH. As the pH value increased from 3.0 to 5.0, the degradation efficiency of BPA significantly promoted from 91.44% to 99.75%. Correspondingly, as shown in Figure 3B, the reaction constant kobs increased from 150.39 to 299.23 mM−1min−1. The complete removal of BPA was obtained as the initial pH of 7.0 and the kobs value of 1112.6 mM−1min−1. As the initial pH further increased to 9.0 and 11.0, the removal efficiency of BPA gradually decreased to 98.16% and 86.74%, respectively, with the value of kobs decreasing to 419.79 and 237.30 mM−1min−1. In general, the degradation efficiency of BPA by ozonation under acidic condition was much better than that under alkaline one.
Such pH dependence of degradation performance could be attributed to two reasons, (i) the pattern variation of BPA under different pH based on its pKa; (ii) the self-decomposition of ozone and the generation of •OH was dependent of solution pH (Zhang et al., 2020). BPA has two dissociation constants, i.e., pKa1 = 9.6 and pKa2 = 10.2. Thus, the predominant species is the undissociated BPA when pH at the range of 3.0–9.0. With the increase of solution pH (pH > 9.6), more and more BPA molecules existed in the species of BPA− and BPA2−, which would increase the electron density on the benzene ring and not be beneficial to BPA degradation via direct oxidation pathway. Additionally, it is known that the self-decomposition of ozone would be promoted with the increase of pH, resulting in the more generation of •OH (Gardoni et al., 2012; Sánchez-Polo et al., 2013) and accelerating BPA degradation by indirect oxidation pathway. As •OH has been proved as the dominant active species, it played a vital role in BPA degradation in this study. Thus, the removal efficiency of BPA increased with the increase of initial solution pH from 3.0 to 7.0. However, with the initial pH further increasing from 9.0 to 11.0, excessive •OH would collide with each other and be quenched, resulting in the decrease of available oxidants and the weaken of BPA degradation. In summary, the combined impacts of initial solution pH on BPA degradation by ozonation process resulted in the effective removal of BPA at a wide pH range of 3.0–9.0. However, compared with the alkaline condition, the removal efficiency of BPA by ozonation was slightly higher under acidic condition, which indicated that as well as the indirect pathway by •OH oxidation, the direct one by ozone mulecular oxidation also played an important role on BPA degradation. In this study, a complete removal of BPA was obtained when the initial pH was 7.0. In addition, increasing solution pH also improved the cost of operation. Therefore, the optimal initial pH of 7.0 without any adjustment was selected in this study from the economic strategy and practical point of view.
3.4 Effects of interfering ions on BPA degradation
Most wastewater normally contains metal ions, inorganic ions and organic matters which coexisting with organic pollutants. Thus, the effects of typical ions, including Fe3+, Cu2+, NO3−, PO43-, NH4+, HCO3−, humic acid (HA) (Tay et al., 2012), on the removal efficiencies of BPA by ozonation were carried out at the concentrations of 0–10 mg/L. Other experimental conditions were as follows [BPA]0 = 1.00 mg/L; ozone dosage 0.25 mg/L; initial solution pH = 7.0; temperature = 25 ± 1°C. The experimental results were presented in Figure 4. In general, with the increase dosing of interfering ions, the degradation efficiency of BPA by ozonation was significantly promoted by the metal ions (Fe3+ and Cu2+), slightly enhanced by PO43-, obviously weakened by NH4+, HCO3− and HA, respectively.
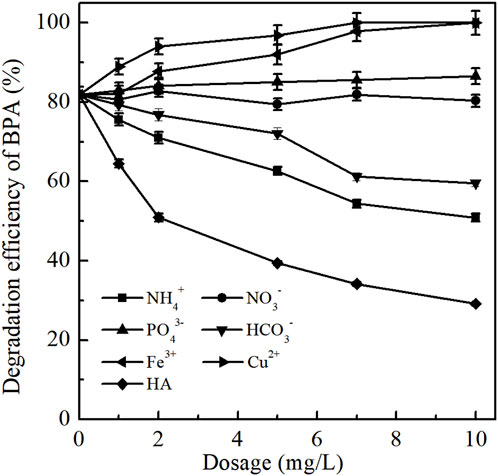
FIGURE 4. Effects of co-existing interfering ions on BPA degradation. (Experimental conditions: [BPA]0 = 1.00 mg/L; ozone dosage 0.25 mg/L; initial solution pH = 7.0; temperature = 25 ± 1°C; the dosage of Fe3+, Cu2+, NO3−, PO43-, NH4+, HCO3− and HA was 0, 1, 2, 5, 7, 10 mg/L).
3.4.1 Metal ions
The addition of Fe3+ and Cu2+ both promoted BPA degradation by ozonation, and the promoting effect was more obvious with the increase of their dosages. Taking Fe3+ as an example, when the dosage of Fe3+ increased from 0 to 10 mg/L, the degradation efficiency of BPA increased from 81.92% to 100% within 10 min. The reasons mainly include two aspects: on the one hand, the addition of Fe3+ might cause the formation of Fe(OH)3 colloid by hydrolysis of Fe3+ in water (Zeng et al., 2012), which has the role of coagulation and flocculation, so that part of BPA can be removed by adsorption. On the other hand, Fe3+ has a catalytic effect on ozone, which was conducive to the generation of •OH, thereby strengthening the degradation of BPA by indirect oxidation pathway. The main reactions were shown in Eqs. 3–6 (Kang et al., 2018)
The effect mechanism of Cu2+ on BPA degradation in the ozonation process was similar with that of Fe3+. Under neutral conditions, Cu2+ mainly existed in the form of Cu(OH)2 in aqueous solution. Cu(OH)2 also had coagulation function, and could catalyze ozone-induced chain reactions. In addition, the complete removal of BPA could be achieved within 5 min when Cu2+ was dosed, which indicated that the catalytic effect of Cu2+ on ozone was more obvious than that of Fe3+.
3.4.2 Inorganic ions
As seen from Figure 4, the presence of nitrate ion (NO3−) at concentration levels of 0–10 mg/L almost had no influence on BPA degradation in ozonation process. The average removal efficiency of BPA kept fluctuating in the ranges of 79.43%–82.84% before and after the dosing of NO3−. This might be due to that NO3− does not compete oxidants with BPA.
With the dosage of PO43- increasing from 0 to 10 mg/L, the removal efficiency of BPA gradually increased from 81.92% to 86.56%. The addition of PO43- could enhance the ionic strength of the reaction system, resulting in a positive ortho-salt effect and being beneficial to BPA degradation by ozonation. In addition, PO43- might have a small promoting effect on the ozone decomposition, which facilitated the removal of BPA by generating superoxide radicals and promoting the generation of •OH (Graham et al., 2004).
The inhibition role of NH4+ on BPA degradation in ozonation process was mainly reflected in its reducibility and competition for ozone molecular and hydroxyl radical. Therefore, when the concentration of NH4+ increased from 0 to 10 mg/L, the degradation efficiency of BPA significantly decreased from 81.92% to 50.85%.
Meanwhile, the presence of HCO3− also inhibited the degradation of BPA by ozonation and this influence was enhanced with the increase of HCO3− concentration. The dosing of HCO3− could increase the alkalinity of the reaction system, which might increase the solution pH at a slight degree and further promote BPA degradation by indirect oxidation pathway. However, HCO3− was also a strong •OH inhibitor [40], which can capture •OH to form CO3•-, thus adversely affecting the degradation efficiency of BPA [41]. The reactions were shown in Eqs. 7, 8.
3.4.3 Organic matter
There are a lot of complex organic compounds in water, many of which have unsaturated structures. Thus, the existence of these organic compounds might have obvious influence on the oxidation reactions. As shown in Figure 4, humic acid (HA) played a great inhibition role on BPA degradation in ozonation process. With the increase of HA concentration from 0 to 10 mg/L, the removal efficiency of BPA significantly decreased from 81.92% to 29.17%. This was because HA is a natural macromolecular aromatic carboxylic acid with a large number of hydroxyl, carboxyl, and carbonyl functional groups attached to the ring, which was easier to react with the oxidants than BPA (Oguzie et al., 2020; Deborde et al., 2008).
3.5 Identification of products and proposition of mechanism
The identification of products during BPA degradation by ozonation was evaluated by GC/MS-MS. The main fragment ions (m/z) and abundance (%) of reaction products in comparison to the NIST data library are summarized in Table 1. From the GC/MS-MS analysis, nine identified intermediate compounds were 3-methyl-1,4-pentadiene, 1,4-pentadien-3-one, phenol, 1,4-dihydroxy benzene, 4-benzoquinone, cis-butenedioic acid, 4-isopropenylphenol, 4-isopropylphenol and (2E,4Z)-3-(2-(4-Hydroxyphenyl) propan-2-yl)he xa-2,4-dienedioic acid. Four of them have been identified in the earlierly reported ozonation process [Kusvuran et al., 2013; Deborde et al., 2008], such as (2E,4Z)-3-(2- (4-Hydroxyphenyl) propan-2-yl) hexa-2,4-dienedioic acid, 4-isopropenylphenol, 4-benzoquinone and 1,4-dihydroxy benzene. The product of 4-isopropenylphenol has also been detected during photocatalytic (Kan et al., 2022; Li et al., 2022) and Fenton-like catalytic (Chu et al., 2021; Xu et al., 2021) degradation of BPA. In addition, phenol, 4-isopropylphenol and even short-chain acids have been found in the electrocatalytic degradation of BPA (Mohammad et al., 2021), which was also similar with this study.
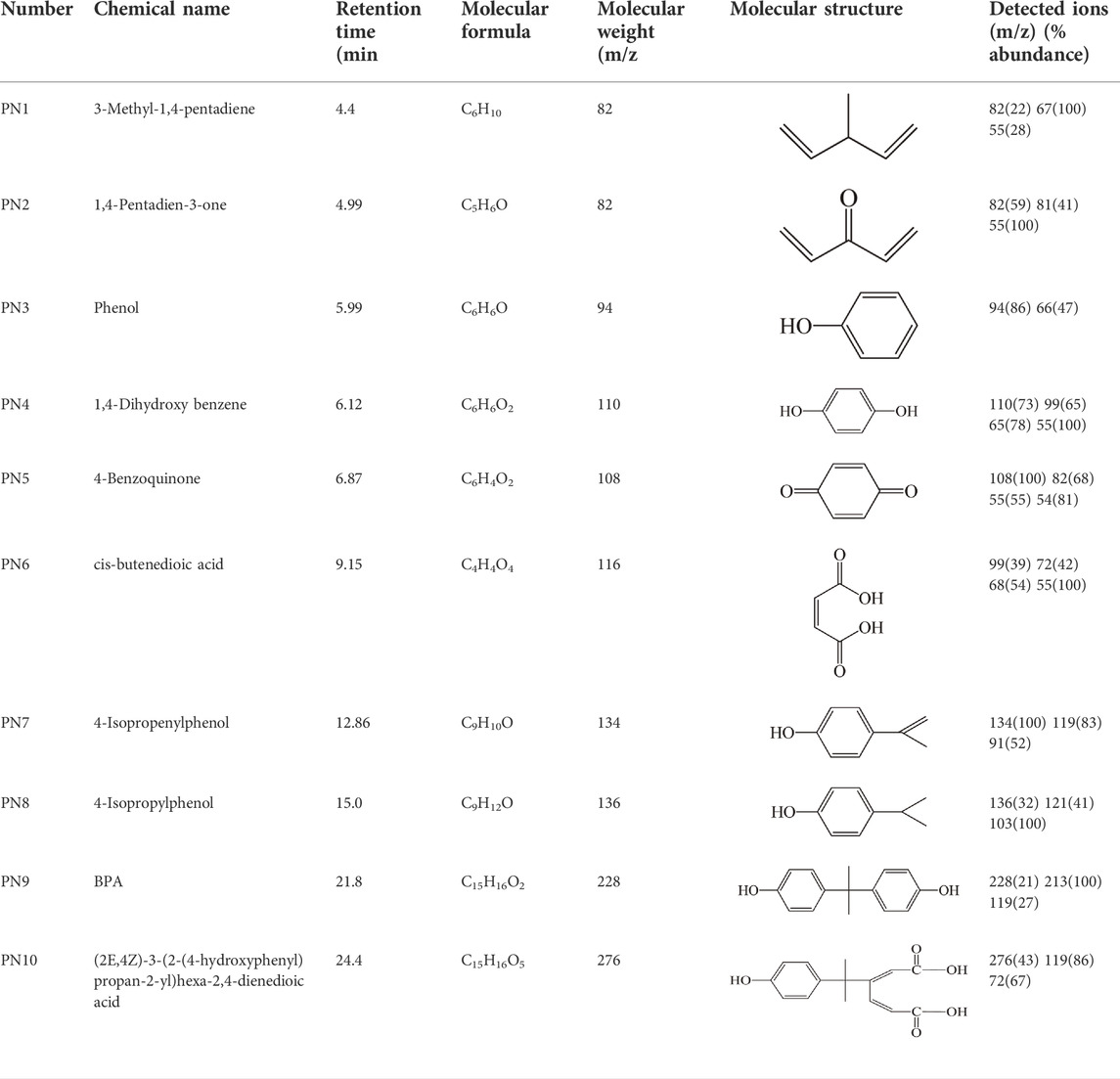
TABLE 1. Main fragment ions (m/z) and abundance (%) of reaction intermediates identified by GC/MS-MS.
In order to elucidate the degradation pathways of BPA by ozonation, theoretical DFT method was used, during which the regioselectivity for radicals attacking being focused. Supplementary Figure S2 shows the structure of BPA. From the calculations of the Hirshfeld charge distribution and Fukui function of carbon atoms (Table 2), it could be seen that the most positive parts of f-function are localized on C1 (C1′), C4 (C4′) and C6 (C6′), which means para and o-rtho positions of hydroxyl are favourable reactive sites for electrophilic attack. While most positive parts of f + function are localized on C2 (C2′), C3 (C3′), C5 (C5′) and C6 (C6’), which demonstrated that the C2, C3, C5 and C6 in BPA with high values are the most reactive sites for radical attacks. Then, values of the enthalpy (ΔH, kcal/mol) and the Gibbs free energy (ΔG, kcal/mol) for the reactions between BPA and radicals at SMD/B3LYP/6-311G** level of DFT calculation were calculated, as shown in Figure 5. The values of ΔG at C1, C4, C5, C6 were respectively −7.26, −8.24, −4.17 and −9.78 kcal/mol, which were all negative and showed all reactions being thermodynamically exothermic and feasible. In addition, as the ΔH between •OH and C6 (5.35 kcal/mol) was the lowest, indicating that reactions on the C6 site is easier than on other positions. In general, the reactions between BPA and •OH were sorted from easy to difficult as C6 > C4 > C5 > C1.
By combining the results of GC/MS-MS detection and DFT evaluation, the degradation mechanism was proposed and presented in Figure 6. Due to the electrophilic character of BPA, ozone commonly reacts with aromatic rings by electrophilic substitution or 1,3-dipolar cycloaddition (Mvula and Sonntag, 2003; Peternel et al., 2012). However, due to the non-selectively and high-reactivity (108–1010 L/(mol∙s)), the indirect •OH oxidation was chosen as the main pathway for BPA degradation by ozonation system. BPA could be easily attacked by •OH at the sites of C6 and C4 through addition, oxidation and cleavaged of the C-C bonds to produce various intermediates then also began to react with these degradation intermediates containing double bonds in their chemical structures [Kusvuran et al., 2013; Wang et al., 2022].
Accordingly, the degradation of BPA by ozonation can be divided into two possible pathways related to the bonds broken. The first pathway was attacking the C-O bond of the benzene ring (C6) by the generated •OH, through which the benzene ring was cracked and BPA was oxidized to form PN10. Then, PN10 was transformed into phenol (PN3) and cis-butenedioic acid (PN6) through the cleavage of C-C bond. PN3 was oxidized to PN5 (4-benzoquinone), which was further cracked and oxidized to short-chain products (PN1 and PN2) and even minerized to CO2 and H2O. The second pathway of •OH oxidation started at the C4 site. The C-C bond connecting two benzene rings was attacked, by which the monobenzene ring products was formed (PN4, PN7 and PN8). These three intermediates could be mutual converted through the channels of the addition reactions or hydrogen extraction reactions. Then, they could also be gradually degraded to PN3, PN5, PN1 and PN2 by a series reactions of dehydroxylation and oxidation. Finally, the products with small molucules were further oxidized and even mineralized into CO2 and H2O.
3.6 Variation and controlling effect of the toxicity
As one of the most important ecological parameters, the evolution of the toxicity during BPA degradation by ozonation was estimated on the basis of toxicity tests performed on the luminous bacteria, with which specific method has been explained in the Experimental Section (Freitas et al., 2017; Li et al., 2008). In the experiments, ozone dosages were varied between 0.25 and 1.50 mg/L. The other conditions of initial BPA concentration, temperature and the pH value were 1 mg/L, 25 ± 1°C and 7.0, respectively. During ozone contacting (0–60 min), the solution samples at different reaction times were orderly inoculated with the bacteria V. fischeri. The luminescence relative inhibitory rate (T%) was calculated and evaluated after 15 min. The results obtained were shown in Figure 7.
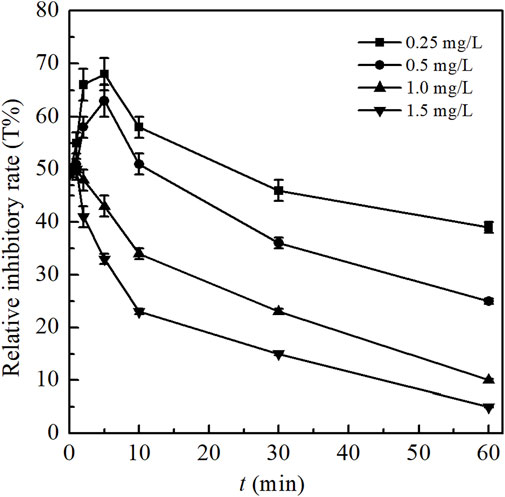
FIGURE 7. Toxicity evolution during BPA degradation by ozonation at different ozone dosages. (Experimental conditions: [BPA]0 = 1.00 mg/L; temperature = 25 ± 1°C; initial solution pH = 7.0; O3 dosage = 0.25, 0.50, 1.00, 1.50 mg/L).
As can be observed in Figure 7, all the values of the relative inhibitory rate under different ozone dosages increased sharply at the initial stage of reactions, then gradually decreased and finally reached a steady state with the further degradation of BPA and its intermediates, which indicated the toxicity changes of the water samples. BPA is a toxic compound. Its initial bioluminescence inhibition without the presence of ozone was around 49%. Take ozone dosage of 0.25 mg/L as an example, the value of T% rapidly increased to the maximum 68% within 5 min reaction in ozonation process, indicating the significant increase of biotoxicity. This might be attributed to the generation and accumulation of more toxic intermediates of BPA, such as 4-isopropylphenol, cis-butenedioic acid, 4-benzoquinone, 1,4-dihydroxy benzene and phenol (as exhibited in Table 1). Toxicological data have shown that the values of LD50 for these above products were respectively 880, 400, 103, 320, and 530 mg/kg (oral dose, mouse), which were all much lower than that of BPA (4200 mg/kg, oral dose, mouse) and indicated the higher toxicity than BPA itself (Freitas et al., 2017). In addition, as shown in Supplementary Figure S2, the peak area of some intermediates (such as 4-isopropylphenol, 1,4-dihydroxy benzene and phenol) increased sharply to the maximum at the initial stage of the reactions and then gradually decreased as the degradation prolonged, which was consistent with the variation of the toxicity. Due to a series of effects such as ring opening, chain breaking and mineralization in the degradation process, intermediates were continuously oxidized or even mineralized into CO2 and H2O, resulting in the gradual reduction of the water samples’ biotoxicity (Malik et al., 2020). Thus, the value of T% gradually decreased to 39% after 60 min reaction when dosing 0.25 mg/L of ozone. Similar findings have been obtained by other researches (Alum et al., 2004; Bertanza et al., 2011; Kim et al., 2008), where more estrogenic intermediates were generated at the initial stage of BPA degradation. However, the level of estrogenicity decreased to below the initial level and subsequently became stable after long contact time and high inlet ozone concentration. It has been reported that more than 98% of the estrogenicity of BPA (initial concentration of 0.10 mg/L) was removed by 1.4 mg/L ozone.
It can also been concluded from Figure 7 that the toxicity of the treated water samples could be effectively controlled with the increase of the ozone dosage. When the dosing of ozone increased from 0.25 to 1.50 mg/L, the maximum values of the relative inhibitory rate obviously decreased from 68% (at 5 min) to 50% (at 1 min), indicating the less formations of more toxic intermediates and less required time for their accumulation. In addition, after reaction for 60 min, the values of T% were significantly decreased from 39% to 5%, which were all below the initial T% of BPA (49%). The complete removal of BPA was achieved when the ozone dosage increased to 1.0 and 1.5 mg/L respectively within 10 and 5 min, which means that the toxic products could be further effectively degraded. From the results obtained, it could be deduced that ozonation is a promising technology for effectively controlling the toxicity of the water samples as well as significantly degrading BPA.
4 Conclusion
The degradation of BPA by ozoantion was systematically investigated in this study, and the major conclusions are summarized as follows:
1) Ozonation was an effective method for BPA degradation. By dosing 1.0 mg/L of ozone, BPA (1.0 mg/L) was completely removed within 10 min under pH of 7.0 and temperature at 25 ± 1°C. The degradation reactions were well fitted with the pseudo-second-order kinetics and •OH was proved as the predominant oxidation species.
2) Ozonation could effectively adapt the temperature and pH of the reaction solution. A complete removal of BPA was achieved at the optimal reaction temperatures range of 25–30°C. In addition, over 91% of BPA could be removed at a wide pH range of 3.0–9.0.
3) The presence of PO43-, Fe3+ and Cu2+ were beneficial to the degradation of BPA, which played the role of ionic enhancer, catalyst, flocculant, etc. However, by competing the reaction active species, BPA degradation was inhibited at the presence of •OH inhibitors (HCO3−), organic compounds (HA) and reducing substance (NH4+).
4) Results of the DFT calculation revealed that the reaction sites between BPA and •OH were sorted from easy to difficult as C6 > C4 > C5 > C1.
5) The biotoxicity of water samples increased first and then decreased with the reaction time, which was caused by the generation and accumulation of more toxic intermediates. However, as dosing 1.50 mg/L ozone, the values of T% could be effectively controlled at 5% after 60 min reactions.
Data availability statement
The original contributions presented in the study are included in the article/Supplementary Material, further inquiries can be directed to the corresponding author.
Author contributions
QH performed the data analyses, wrote and revised the manuscript; WD contributed to the conception of the study; HW performed the experiments; BY helped perform the analysis with constructive discussions; PL contributed to the conception of the study and contributed significantly to analysis and manuscript preparation; LX helped perform the revised the manuscript; ZD helped perform the experiments and analysis. All authors have read and agreed to the published version of the manuscript.
Funding
This work was supported by the Project of Shenzhen Science and Technology Innovation Committee (No. KCXFZ202002011006362) and the National Natural Science Foundation of China (Grant No.4217070174).
Acknowledgments
We acknowledge all the authors for their contributions. We sincerely thank the reviewers and the editor for their effort to review this manuscript.
Conflict of interest
The authors declare that the research was conducted in the absence of any commercial or financial relationships that could be construed as a potential conflict of interest.
Publisher’s note
All claims expressed in this article are solely those of the authors and do not necessarily represent those of their affiliated organizations, or those of the publisher, the editors and the reviewers. Any product that may be evaluated in this article, or claim that may be made by its manufacturer, is not guaranteed or endorsed by the publisher.
Supplementary material
The Supplementary Material for this article can be found online at: https://www.frontiersin.org/articles/10.3389/fenvs.2022.1009499/full#supplementary-material
References
Ahmad, N. A., Yuzir, M. A., Yong, E. L., Abdullah, N., and Salim, M. R. (2015). Removal of bisphenol A (BPA) in surface water by ozone oxidation process. Appl. Mech. Mater. 735, 210–214. doi:10.4028/www.scientific.net/AMM.735.210
Ahmadi, M., Rahmani, H., Takdastan, A., Jaafarzadeh, N., and Mostoufi, A. (2016). A novel catalytic process for degradation of bisphenol A from aqueous solutions: A synergistic effect of nano-Fe3O4 @alg-Fe on O3/H2O2. Process Saf. Environ. Prot. 104, 413–421. doi:10.1016/j.psep.2016.09.008
Alum, A., Yoon, Y., Westerhoff, P., and Abbaszadegan, M. (2004). Oxidation of bisphenol A, 17-estradiol, and 17-ethynyl estradiol and byproduct estrogenicity. Environ. Toxicol. 19, 257–264. doi:10.1002/tox.20018
APHA (2005). Standard methods for the examination of water and wastewater. 21 th Ed. Washington D.C.
Bertanza, G., Pedrazzani, R., Dal Grande, M., Papa, M., Zambarda, V., Montani, C., et al. (2011). Effect of biological and chemical oxidation on the removal of estrogenic compounds (NP and BPA) from wastewater: An integrated assessment procedure. Water Res. 45, 2473–2484. doi:10.1016/j.watres.2011.01.026
Bhatnagar, A., and Anastopoulos, I. J. C. (2016). Adsorptive removal of bisphenol A (BPA) from aqueous solution: A review. Chemosphere 168, 885–902. doi:10.1016/j.chemosphere.2016.10.121
Bu, X., and WuHeHuang, X. G. J. (2016). Novel adaptive neural control design for a constrained flexible air-breathing hypersonic vehicle based on actuator compensation. Acta Astronaut. 120, 75–86. doi:10.1016/j.actaastro.2015.12.004
Chu, J., Kang, J., Seong, J., and Chang, G. (2021). Bisphenol A degradation using waste antivirus copper film with enhanced sono-Fenton-like catalytic oxidation. Chemosphere 276, 130218. doi:10.1016/j.chemosphere.2021.130218
Dai, Q., Wang, J., Yu, J., Chen, J., and Chen, J. (2014). Catalytic ozonation for the degradation of acetylsalicylic acid in aqueous solution by magnetic CeO2 nanometer catalyst particles. Appl. Catal. B Environ. 144, 686–693. doi:10.1016/j.apcatb.2013.05.072
Deborde, M., Rabouan, S., Mazellier, P., Duguet, J. P., and Legube, B. (2008). Oxidation of bisphenol A by ozone in aqueous solution. Water Res. 42, 4299–4308. doi:10.1016/j.watres.2008.07.015
Delley, B. (2000). From molecules to solids with the DMol3 approach. J. Chem. Phys. 113, 7756–7764. doi:10.1063/1.1316015
Ding, S., Wu, J., Zhang, M., Lu, H., Mahmood, Q., and Zheng, P. (2015). Acute toxicity assessment of ANAMMOX substrates and antibiotics by luminescent bacteria test. Chemosphere 140, 174–183. doi:10.1016/j.chemosphere.2015.03.057
Farre, M. J., Franch, M. I., Malato, S., Ayllon, J. A., Peral, J., and Domenech, X. (2005). Degradation of some biorecalcitrant pesticides by homogeneous and heterogeneous photocatalytic ozonation. Chemosphere 58, 1127–1133. doi:10.1016/j.chemosphere.2004.09.064
Ferro Orozco, A. M., Lobo, C. C., Contreras, E. M., and Zaritzky, N. E. (2013). Biodegradation of bisphenol-A (BPA) in activated sludge batch reactors: Analysis of the acclimation process. Int. Biodeterior. Biodegrad. 85, 392–399. doi:10.1016/j.ibiod.2013.09.005
Freitas, E. D., Bubna, G., Brugnari, T., Kato, C., Nolli, M., Rauen, T., et al. (2017). Removal of bisphenol A by laccases from Pleurotus ostreatus and Pleurotus pulmonarius and evaluation of ecotoxicity of degradation products by laccases from Pleurotus ostreatus and Pleurotus pulmonarius. Chem. Eng. J. 330, 1361–1369. doi:10.1016/j.cej.2017.08.051
Gao, J., Luo, C., GanGan, L., Wu, D., Tan, F., Cheng, X., et al. (2020). A comparative study of UV/H2O2 and UV/PDS for the degradation of micro-pollutants: Kinetics and effect of water matrix. Environ. Sci. Pollut. Res. 27, 24531–24541. doi:10.1007/s11356-020-08794-1
Gardoni, D., Vailati, A., and Canziani, R. (2012). Decay of ozone in water: A review. Ozone Sci. Eng. 34 (4), 233–242. doi:10.1080/01919512.2012.686354
Garoma, T., Matsumoto, S. A., Wu, Y., and Klinger, R. (2010). Removal of bisphenol A and its reaction-intermediates from aqueous solution by ozonation. Ozone Sci. Eng. 32, 338–343. doi:10.1080/01919512.2010.508484
Graham, N., Jiang, C. C., Li, X. Z., Jiang, J. Q., and Ma, J. (2004). The influence of pH on the degradation of phenol and chlorophenols by potassium ferrate. Chemosphere 56, 949–956. doi:10.1016/j.chemosphere.2004.04.060
Grimme, S., Antony, J., Ehrlich, S., and Krieg, H. (2010). A consistent and accurate ab initio parametrization of density functional dispersion correction (DFT-D) for the 94 elements H-Pu. J. Chem. Phys. 132, 154104. doi:10.1063/1.3382344
Han, C., and Hong, Y. C. (2016). Bisphenol A, hypertension, and cardiovascular diseases: Epidemiological, laboratory, and clinical trial evidence. Curr. Hypertens. Rep. 18, 11. doi:10.1007/s11906-015-0617-2
He, L., Liu, H., Lv, B., Liu, P., and Lin, B. (2017). Visible-light-driven photocatalytic performance of nanohybrid incorporating nickel ions into the tetratitanate interlayer. Vacuum 138, 64–69. doi:10.1016/j.vacuum.2017.01.024
Hu, Y., Zhu, Q., Yan, X., Liao, C., and Jiang, G. (2019). Occurrence, fate and risk assessment of BPA and its substituents in wastewater treatment plant: A review. Environ. Res. 178, 108732. doi:10.1016/j.envres.2019.108732
Huang, Y. Q., Wong, C. K., Zheng, J. S., Bouwman, H., Barra, R., Wahlstrom, B., et al. (2012). Bisphenol A (BPA) in China: A review of sources, environmental levels, and potential human health impacts. Environ. Int. 42, 91–99. doi:10.1016/j.envint.2011.04.010
Huang, Y., Yang, T., Liang, M., Wang, Y., Xu, Z., Zhang, D., et al. (2019). Ni-Fe layered double hydroxides catalized ozonation of synthetic wastewater containing Bisphenol A and municipal secondary effluent. Chemosphere 235, 143–152. doi:10.1016/j.chemosphere.2019.06.162
Kan, L., Chang, C., Wang, Q., and Wang, X. (2022). Glycol assisted splitting BiOIO3 into plasmonic bismuth coupled with BiOI co-modified Bi2WO6 (BiOI/Bi/Bi2WO6) to form indirect Z-scheme heterojunction for efficient photocatalytic degradation of BPA. Sep. Purif. Technol. 15, 121537. doi:10.1016/j.seppur.2022.121537
Kang, Y. M., Kim, M. K., and Zoh, K. D. (2018). Effect of nitrate, carbonate/bicarbonate, humic acid, and H2O2 on the kinetics and degradation mechanism of Bisphenol-A during UV photolysis. Chemosphere 204, 148–155. doi:10.1016/j.chemosphere.2018.04.015
Kim, S.-E., Park, N.-S., Yamada, H., and Tsuno, H. (2008). Modeling of decomposition characteristics of estrogenic chemicals during ozonation. Environ. Technol. 29, 287–296. doi:10.1080/09593330802099643
Kusvuran, E., Gulnaz, O., Samil, A., and Erbil, M. (2010). Detection of double bond-ozone stoichiometry by an iodimetric method during ozonation processes. J. Hazard. Mat. 175, 410–416. doi:10.1016/j.jhazmat.2009.10.021
Kusvuran, E., and Yildirim, D. (2013). Degradation of bisphenol A by ozonation and determination of degradation intermediates by gas chromatography-mass spectrometry and liquid chromatography-mass spectrometry. Chem. Eng. J. 220, 6–14. doi:10.1016/j.cej.2013.01.064
Laws, S. C., Carey, S. A., Ferrell, J. M., Bodman, G. J., and Cooper, R. L. (2000). Estrogenic activity of octylphenol, nonylphenol, bisphenol A and methoxychlor in rats. Toxicol. Sci. 54, 154–167. doi:10.1093/toxsci/54.1.154
Li, C., Li, X., Graham, N., and Gao, N. (2008). The aqueous degradation of bisphenol A and steroid estrogens by ferrate. Water Res. 42, 109–120. doi:10.1016/j.watres.2007.07.023
Li, Z., Luo, C., Tan, F., Wu, D., Zhai, X., Wang, S., et al. (2022). UV light irradiation combined with nitrate for degradation of bisphenol A: Kinetics, transformation pathways, and acute toxicity assessment. Environ. Sci. Water Res. Technol. 8, 586–596. doi:10.1039/d1ew00796c
Luo, C., Wang, S., Wu, D., Cheng, X., and Ren, H. (2022). UV/Nitrate photocatalysis for degradation of Methylene blue in wastewater: Kinetics, transformation products, and toxicity assessment. Environ. Technol. Innovation 25, 102198. doi:10.1016/j.eti.2021.102198
Malik, S. N., Ghosh, P. C., Vaidya, A. N., and Mudliar, S. N. (2020). Ozone pretreatment of biomethanated distillery wastewater in a semi batch reactor: Mapping pretreatment efficiency in terms of COD, color, toxicity and biohydrogen generation. Biofuels 11 (7), 801–809. doi:10.1080/17597269.2017.1416521
Masten, S. J., Davies, S. H. R., Luo, C., Tan, F., Wu, D., Zhai, X., et al. (1994). The use oLiUV light irradiation combined with nitrate for degradation of bisphenol A: Kinetics, transformation pathways, and acute toxicity assessment. Environ. Sci. Water Res. Technol. 8, 586–596. doi:10.1039/d1ew00796c
Mohammad, R., Amin, A., Abdollah, D., Amir, S., Davood, N., Mohammad, K., et al. (2021). Enhanced electrocatalytic degradation of bisphenol A by graphite/β-PbO2 anode in a three-dimensional electrochemical reactor. J. Environ. Chem. Eng. 9 (5), 106072. doi:10.1016/j.jece.2021.106072
Mvula, E., and Von Sonntag, C. (2003). Ozonolysis of phenols in aqueous solution. Org. Biomol. Chem. 1, 1749–1756. doi:10.1039/B301824P
Neyens, E., and Baeyens, J. (2003). A review of classic Fenton’s peroxidation as an advanced oxidation technique. J. Hazard. Mat. 98, 33–50. doi:10.1016/S0304-3894(02)00282-0
Oguzie, K. L., Qiao, M., Zhao, X., Oguzie, E. E., Njoku, V. O., and Obodo, G. A. (2020). Oxidative degradation of Bisphenol A in aqueous solution using cobalt ion-activated peroxymonosulfate. J. Mol. Liq. 313, 113569. doi:10.1016/j.molliq.2020.113569
Pan, G. Y., Chen, C. L., Chang, H. M., and Gratzl, J. S. (1984). Studies on ozone bleaching. I. The effect of PH, temperature, buffer systems and heavy metal-ions on stability of ozone in aqueous solution. J. Wood Chem. Technol. 4, 367–387. doi:10.1080/02773818408070655
Peternel, I., Koprivanac, N., and Grcic, I. (2012). Mineralization of p-chlorophenol in water solution by AOPs based on UV irradiation. Environ. Technol. 33, 27–36. doi:10.1080/09593330.2010.504233
Qi, F., Chu, W., and Xu, B. (2015). Ozonation of phenacetin in associated with a magnetic catalyst CuFe2O4: The reaction and transformation. Chem. Eng. J. 262, 552–562. doi:10.1016/j.cej.2014.09.068
Quan, X., Hu, R., Chang, H., Tang, X., Huang, X., Cheng, C., et al. (2020). Enhancing microalgae growth and landfill leachate treatment through ozonization. J. Clean. Prod. 248, 119182. doi:10.1016/j.jclepro.2019.119182
Reddy, P. V. L., Kim, K. H., Kavitha, B., Kumar, V., Raza, N., and Kalagara, S. J. J. o. E. M. (2018). Photocatalytic degradation of bisphenol A in aqueous media: A review. J. Environ. Manag. 213, 189–205. doi:10.1016/j.jenvman.2018.02.059
Sahoo, N., Pakshirajan, K., and Ghosh, P. (2010). Enhancing the biodegradation of 4-chlorophenol by Arthrobacter chlorophenolicus A6 via medium development. Int. Biodeterior. Biodegrad. 64, 474–480. doi:10.1016/j.ibiod.2010.05.008
Sánchez-Polo, M., Ocampo-Pérez, R., Rivera-Utrilla, J., and Mota, A. J. (2013). Comparative study of the photodegradation of bisphenol A by HO, SO4− and CO3−/HCO3 radicals in aqueous phase. Sci. Total Environ. 463, 423–431. doi:10.1016/j.scitotenv.2013.06.012
Schröder, H. F. (2006). The elimination of the endocrine disrupters 4-nonylphenol and bisphenol A during wastewater treatment - comparison of conventional and membrane assisted biological wastewater treatment followed by an ozone treatment. Water Pract. Technol. 1. doi:10.2166/wpt.2006.060
Sharma, J., and MishraDionysiouKumar, I. M. D. D. V. (2015). Oxidative removal of Bisphenol A by UV-C/peroxymonosulfate (PMS): Kinetics, influence of co-existing chemicals and degradation pathway. Chem. Eng. J. 276, 193–204. doi:10.1016/j.cej.2015.04.021
Takdastan, A., Kakavandi, B., Azizi, M., and Golshan, M. J. C. E. J. (2018). Efficient activation of peroxymonosulfate by using ferroferric oxide supported on carbon/UV/us system: A new approach into catalytic degradation of bisphenol A. Chem. Eng. J. 331, 729–743. doi:10.1016/j.cej.2017.09.021
Tay, K. S., Abd Rahman, N., and Abas, M. R. B. (2012). Degradation of bisphenol A by ozonation: Rate constants, influence of inorganic anions, and by-products. Maejo Int. J. Sci. Technol. 6 (01), 77–94. doi:10.14456/mijst.2012.7
Teppala, S., Madhavan, S., and Shankar, A. (2012). Bisphenol A and metabolic syndrome: Results from nhanes. Int. J. Endocrinol., 1–5. doi:10.1155/2012/598180
Trojanowicz, M., Bojanowska-Czajka, A., Szreder, T., Mczyńska-Wielgosz, S., Nichipor, H. J. C. E. J., Fornal, E., et al. (2020). Application of ionizing radiation for removal of endocrine disruptor bisphenol A from waters and wastewaters. Chem. Eng. J. 403, 126169. doi:10.1016/j.cej.2020.126169
Van Aken, P., Lambert, N., Van den Broeck, R., Degrève, J., and Dewil, R. (2019). Advances in ozonation and biodegradation processes to enhance chlorophenol abatement in multisubstrate wastewaters: A review. Environ. Sci. Water Res. Technol. 5, 444–481. doi:10.1039/C8EW00562A
Wang, L., Qi, C., Lu, Y., Arowo, M., and Shao, L. (2022b). Degradation of Bisphenol A by ozonation in a rotating packed bed: Modeling by response surface methodology and artificial neural network. Chemosphere 286, 131702. doi:10.1016/j.chemosphere.2021.131702
Wang, Y., Lin, Y., Yang, C., Wu, S., Fu, X., and Li, X. (2022a). Calcination temperature regulates non-radical pathways of peroxymonosulfate activation via carbon catalysts doped by iron and nitrogen. Chem. Eng. J. 451, 138468. doi:10.1016/j.cej.2022.138468
Wu, C. (2008). Decolorization of C.I. Reactive red 2 in O3, fenton-like and O3/Fenton-like hybrid systems. Dyes Pigments 77, 24–30. doi:10.1016/j.dyepig.2007.03.002
Wu, S., Li, H., Li, X., He, H., and Yang, C. (2018). Performances and mechanisms of efficient degradation of atrazine using peroxymonosulfate and ferrate as oxidants. Chem. Eng. J. 353, 533–541. doi:10.1016/j.cej.2018.06.133
Wu, S., Lin, Y., Yang, C., Du, C., Teng, Q., Ma, Y., et al. (2019). Enhanced activation of peroxymonosulfte by LaFeO3 perovskite supported on Al2O3 for degradation of organic pollutants. Chemosphere 237, 124478. doi:10.1016/j.chemosphere.2019.124478
Wu, S., Liu, H., Yang, C., Li, X., Lin, Y., Yin, K., et al. (2020). High-performance porous carbon catalysts doped by iron and nitrogen for degradation of bisphenol F via peroxymonosulfate activation. Chem. Eng. J. 392, 123683. doi:10.1016/j.cej.2019.123683
Wu, S., Shen, L., Lin, Y., Yin, K., and Yang, C. (2021). Sulfite-based advanced oxidation and reduction processes for water treatment. Chem. Eng. J. 414 (15), 128872. doi:10.1016/j.cej.2021.128872
Wu, S., Yang, C., Lin, Y., and Cheng, J. (2022). Efficient degradation of tetracycline by singlet oxygen-dominated peroxymonosulfate activation with magnetic nitrogen-doped porous carbon. J. Environ. Sci. 115, 330–340. doi:10.1016/j.jes.2021.08.002
Xiao, K., Liang, H., Chen, S., Yang, B., Zhang, J., and Li, J. J. C. (2019). Enhanced photoelectrocatalytic degradation of bisphenol A and simultaneous production of hydrogen peroxide in saline wastewater treatment. Chemosphere 222, 141–148. doi:10.1016/j.chemosphere.2019.01.109
Xu, S., Zhang, Y., Tang, R., Zhang, X., Zhen, H., and Han, Q. (2021). Enhancing Fenton-like catalytic efficiency of Bi2WO6 by iodine doping for pollutant degradation. Sep. Purif. Technol. 277 (15), 119447. doi:10.1016/j.seppur.2021.119447
Yang, C. W. (2015). Degradation of bisphenol A using electrochemical assistant Fe(II)-activated peroxydisulfate process. Water Sci. Eng. 8, 139–144. doi:10.1016/j.wse.2015.04.002
Yang, Y., Guo, H., Zhang, Y., Deng, Q., and Zhang, J. (2016). Degradation of bisphenol A using ozone/persulfate process: Kinetics and mechanism. Water Air Soil Pollut. 227, 53. doi:10.1007/s11270-016-2746-x
Zeng, Z., Zou, H., Li, X., Sun, B., Chen, J., and Shao, L. (2012). Ozonation of phenol with O3/Fe (II) in acidic environment in a rotating packed bed. Ind. Eng. Chem. Res. 51, 10509–10516. doi:10.1021/ie300476d
Zhang, H., He, Y., Lai, L., Yao, G., and Lai, B. (2020). Catalytic ozonation of Bisphenol A in aqueous solution by Fe3O4–MnO2 magnetic composites: Performance, transformation pathways and mechanism. Sep. Purif. Technol. 245, 116449. doi:10.1016/j.seppur.2019.116449
Keywords: bisphenol A (BPA), ozonation, interfering ions, pathways, toxicity
Citation: Han Q, Dong W, Wang H, Yu B, Dong Z, Li M, Xie L and Dai Z (2022) Performance of ozonation on bisphenol a degradation: Efficiency, mechanism and toxicity control. Front. Environ. Sci. 10:1009499. doi: 10.3389/fenvs.2022.1009499
Received: 02 August 2022; Accepted: 25 August 2022;
Published: 15 September 2022.
Edited by:
Shihai Deng, Xi’an Jiaotong University, ChinaReviewed by:
Zacharias Frontistis, University of Western Macedonia, GreeceShaohua Wu, Guangdong University of Petrochemical Technology, China
Congwei Luo, Shandong Jianzhu University, China
Copyright © 2022 Han, Dong, Wang, Yu, Dong, Li, Xie and Dai. This is an open-access article distributed under the terms of the Creative Commons Attribution License (CC BY). The use, distribution or reproduction in other forums is permitted, provided the original author(s) and the copyright owner(s) are credited and that the original publication in this journal is cited, in accordance with accepted academic practice. No use, distribution or reproduction is permitted which does not comply with these terms.
*Correspondence: Zijun Dong, ZG9uZ3ppanVuQHN6dS5lZHUuY24=