- 1Beijing Key Laboratory of Farmland Soil Pollution Prevention and Remediation, Key Laboratory of Plant-Soil Interactions of Ministry of Education, College of Resources and Environmental Sciences, China Agricultural University, Beijing, China
- 2Institute of Advanced Materials, IAAM, Ulrika, Sweden
- 3School of Agriculture and Environment, The University of Western Australia, Perth, WA, Australia
- 4The UWA Institute of Agriculture, The University of Western Australia, Perth, WA, Australia
The recovery of nutrients from livestock manure has generated a lot of interest in biosolids value-adding. There is now more research on manure with high solids content but less study on manure with lower solids content. This study used swine manure slurry as the research object and comprehensively examined the characteristics of organic matter conversion, nitrogen, phosphorus, and metals release during the catalytic-thermal hydrolysis (TH) process. It was found that ammonia nitrogen showed a continuous increase with increasing temperature while inorganic phosphorus showed a rising and then decreasing trend. The addition of HCl and H2O2 (TH-HCl-H2O2) promoted the hydrolysis of organic matter, showing the best nitrogen and phosphorus release performance, releasing 62.2% of inorganic phosphorus and 50.8% of nitrogen. The release characteristics of nutrients and metals from the TH process were significantly affected by the addition of HCl and H2O2, according to structural equation modelling study. The data analysis demonstrated that the liquid fertilizer produced by TH did not surpass the environmental risk associated with nutrients or the ecological risk associated with heavy metals. This study would offer theoretical justification for biowaste conversion for agricultural applications.
1 Introduction
Resource shortage has become an inevitable common problem for human beings in the current era of rapid population, economic and technical growth (Zhang et al., 2020a). Nitrogen and phosphorus, as nutritional resources, are essential elements for the life activities of living organisms. Among them, phosphorus is a non-renewable resource, phosphate resources may be depleted in the coming decades with the increase in consumption (Karunanithi et al., 2015). With the development of scale and intensification of livestock and poultry farming, a huge number of livestock and poultry manure is produced (Bolan et al., 2010; Pan and Chen, 2021). China’s swine production accounts for 50% of the world, and China produces 3.8 billion tons of swine manure every year (Song et al., 2020), which have high nitrogen and phosphorus contents and can pose a threat to the environment and lead to eutrophication of water bodies (He et al., 2021). Therefore, the recovery of nitrogen and phosphorus from swine manure is of great significance for environmental pollution control and nutrient recycling.
The main conversion processes used today to treat livestock manure are biological and thermochemical. Biological conversion is based on aerobic composting and anaerobic digestion (Khatun et al., 2020; Zheng et al., 2022), but the composting cycle is long, occupies a large area of land and releases large amounts of greenhouse gases (Joardar et al., 2020; Li et al., 2022). There are many thermochemical conversion methods (Wu et al., 2020), such as gasification, direct combustion, thermal hydrolysis, and liquefaction. The recovery of nitrogen and phosphorus from manure resources is currently hampered by two issues. First, it is necessary to release nitrogen and phosphorus into the supernatant to facilitate recovery, such as proteins, amino acids (Bolyard and Reinhart, 2017), DNA, phospholipids, simple phosphate monoesters and phytic acid (Ekpo et al., 2016). Moreover, a recovery method with high selectivity for nitrogen and phosphorus is required because livestock manure contains a variety of complex substances. The use of thermal conversion technology allows the recovery of large amounts of nutrient resources, deodorization and elimination of pathogens. Among them, thermal hydrolysis (TH) (Zhang et al., 2019; Zhang et al., 2020b; Zhang et al., 2020c) to recover nitrogen and phosphorus from swine manure has now become a targeted area of research.
In recent years, TH has received widespread attention in livestock manure treatment because of its direct treatment of wet waste biomass (Deng et al., 2019; Shi et al., 2018). Nevertheless, energy consumption has become a major limiting factor in the promotion and application of TH technology. According to some studies, adding chemicals like acids, bases, or oxidants (H2O2) can effectively reduce energy consumption by facilitating the release and conversion of nitrogen and phosphorus (Ding et al., 2021; Xu et al., 2021; Zhang et al., 2020d). Previous studies have confirmed that HCl-assisted degradation (Zhang et al., 2020d) as well as H2O2-assisted oxidation (He et al., 2022; Zhang et al., 2020a) can catalyze the TH process to improve the release of nitrogen and phosphorus in the liquid phase and reduce energy consumption.
In addition, liquid fertilizer is an economical, environmentally-friendly and efficient fertilizer. Liquid fertilizer has the characteristics of low production cost, pollution-free production process, convenient application, high fertilizer efficiency and easy absorption and utilization by plants (Lu et al., 2021). It is well known that three main plant nutrients used for soil fertilization are nitrogen, phosphorus, and potassium. Furthermore, nitrogen and phosphorus can now be used as liquid organic fertilizer because TH treatment has been shown to increase their solubility in the liquid phase. Studies have demonstrated the fertilizer potential of hydrolysis liquor produced from thermal hydrolysis of food waste (Gao et al., 2021). Therefore, with the improvement of production technology, liquid fertilizer has broad development and application prospects.
Our hypothesis is that the addition of chemicals to the TH process for catalytic oxidation may enhance the efficiency of nitrogen and phosphorus release in manure slurries. We used a combination of geochemical, and integrated spectroscopic and microscopic approaches to verify our hypothesis and ultimately provide an understanding of the fundamental mechanisms that control the nitrogen and phosphorus recovered by TH processes. In this study, the TH processes was used to optimize the nutrient and metal release transformation processes by varying temperature and adding chemicals. The structural equation modelling was used to study the effects of temperature and chemicals on the release of nitrogen, phosphorus, and metals. Finally, environmental risk analyses were performed to evaluate the ecological risk of nutrients and metals. These investigations can provide new insights into the optimization of TH processes and the use of liquid fertilizers in crop production.
2 Materials and methods
2.1 Materials
Swine manure was collected from a swine farm in the suburb of Beijing. The samples were dried, ground, sieved through 40 mesh and sealed in a dry place for later use. The physical and chemical properties of swine manure are shown in Table 1.
2.2 Methods
12.0 g of dried swine manure was accurately weighed in a high-pressure reaction kettle, and 200 ml of deionized water was added to prepare swine manure slurry with a solids content of 6%. The transformation of swine manure in liquid phase during TH processes is studied under different HCl concentrations, H2O2 concentrations, and temperatures.
2.2.1 Thermal hydrolysis (TH) process
Prepared samples with a solids content of 6%, fixed HCl (2.5 M) addition ratio of 5%, and set corresponding seven reaction temperature gradients, i.e., 60°C, 80°C, 100°C, 120°C, 140°C, 160°C, 180°C, reaction time of 0.5 h after reaching the corresponding temperature and rotation speed of 400 rpm. Configured the sample with a solids content of 6%, fixed reaction temperature of 140°C, no H2O2, 0%–13% volume fraction of HCl (2.5 M), reaction time of 0.5 h after reaching the corresponding temperature, and rotation speed of 400 rpm. Samples with a solids content of 6% were prepared, the fixed reaction temperature was 140°C, HCl (2.5 M) addition ratio was 5%, H2O2 (30%) with a volume fraction of 0%–13% were added respectively reaction time was set to 0.5 h after reaching the corresponding temperature, and the rotation speed was set to 400 rpm. After a batch of experiments was finished, the samples were uniformly filtered and the supernatant was taken for subsequent analysis.
2.2.2 Chemical analysis
The total nitrogen and ammonia nitrogen content were determined according to the Kjeldahl method and Naxalite reagent spectrophotometry, respectively (Mahimairaja et al., 1990). The analysis for total phosphorus and inorganic phosphorus concentration was referred to the molybdenum-antimony anti-spectrophotometry (Murphy and Riley, 1962). Metal ions were analyzed by ICP-OES after digestion in Aqua Regia (3: 1 HCl/HNO3).
2.2.3 The fluorescence excitation-emission, EEM spectral analysis
Swine manure water samples were analyzed by three-dimensional fluorescence (EEM, F-7000, Hitachi, Japan) spectroscopy. All the analyzed samples were diluted 125 times. The actual measurement wavelength ranges Ex and Em were 200–700 nm and 200–600 nm, respectively, scanning interval was 10 nm, scanning speed was 12,000 nm/min, slit width was 5 nm, scanning voltage was 600 V, and blank water samples were ultrapure water. The fluorescence region integration method (FRI), proposed by (Chen et al., 2003), can be used to qualitatively analyze the fluorescence spectrum of water. The three-dimensional fluorescence spectrum data formed by emission and excitation wavelengths are divided into five regions, representing five different types of organic substances, including aromatic protein I, aromatic protein II, fulvic acid, soluble microbial metabolites, and humic acid.
2.2.4 Input electrical energy calculation
The thermal hydrolysis process of dissolving swine manure organic matter to release nutrients requires energy consumption, and the electrical energy (EE/O) consumed by each process can be used to calculate the energy consumption in the TH process. The total EE/O includes the sum of the electrical energy of the TH process (EE/OTH) and the electrical energy consumed by the catalyst (EE/OCatalyst). The equations were modified as follows (Guo et al., 2018).
where C is the specific heat capacity of the liquid (4.2 KJ kg−1°C−1), M is the mass of the liquid (kg), ΔT is the change in temperature of the TH system (°C), V(L) is the total volume of the system, (N/N0) is the percentage solubility of the nutrient P or N, CCatalyst (M) is the concentration of the catalyst, and EqCatalyst is the equivalent electrical energy consumption per mole of catalyst produced. More detailed information is provided in Supplementary Appendix Section 2.2.4.
2.2.5 Environmental risk evaluation
The environmental risk evaluation method for fertilizers was designed and optimized by the environmental risk assessment method for heavy metal pollution proposed by Hakanson (1980). The model designed by Lars Hakanson produces a composite environmental risk index that is a positive number greater than 1. The larger the value, the higher the risk level, and there is no upper limit, so it is not easy to compare the environmental risk of pollution of different types of ions. In contrast, the environmental risk index model (Cai et al., 2018) used in this work has a value between 0 and 1, with upper and lower limits of the risk level, which facilitates the understanding and comparison of the risk level. The environmental risk index values are calculated using the following calculation equations:
where Rt is the total index of environmental risk index of fertilizer; Ri represents the environmental risk index of nitrogen and phosphorus, (i = N, P); qi is the weighting coefficient of environmental pollution of nitrogen and phosphorus. Ti is the safety threshold of nitrogen and phosphorus fertilizer application. Fi is the intensity of nitrogen and phosphorus fertilizer use in a certain year, which is the actual fertilizer used in agricultural production in that year. ui represents the fertilizer utilization efficiency (50%) and m represents the replanting index (1). From Eqs 4, 5, we can see that Ri and Rt are between 1 and 0. When Ri = 0.5, Fi and Ti are equal, which is the critical point for environmental safety. In this study, the fertilizer use intensity of 250 kg ha−1 was set as the environmental safety threshold, and with reference to the current nitrogen, phosphorus and potash fertilization ratio of 1:0.5:0.5 in developed countries, the environmental safety thresholds for nitrogen and phosphorus were determined to be 125, 62.5 kg ha−1, respectively (Kulikowska and Gusiatin, 2015).
The potential ecological risk factor (Er) for metals was calculated using the Eq. 6:
where Tri is the toxic-response factor for a given metal (Zn = 1; Cr = 2; Cu = 5); Ci is the total metal concentration and Cni is the threshold limit value for a given metal (Cu = 500 mg kg−1, Zn = 1,200 mg kg−1, Cr = 150 mg kg−1) (Kulikowska and Gusiatin, 2015).
2.3 Statistical analysis
Structural equation modelling (SEM) was used to test the effects of temperature, H2O2 concentration and HCl concentration on total phosphorus (TP), total nitrogen (TN) and metals. SEM analysis was carried out the maximum likelihood evaluation method of AMOS 24.0 (AMOS, IBM, United States). All statistical analyses were performed with SPSS 25.0 (SPSS, IBM, United States).
3 Results and discussion
3.1 Influence of different temperature treatments on swine manure
3.1.1 3D-EEM analysis
The 3D-EEM of the manure slurry treated at different temperatures is shown in Figure 1. It can be seen that the position of fluorescence peak altered with the change of temperature. The fluorescence peaks were mainly located in the regions I, II, III, and IV under the treatment conditions with lower temperatures. With the increase of temperature, the fluorescence intensity of regions I, II and IV firstly increased and then decreased from 160°C, while the fluorescence intensity of regions III and V increased gradually, which indicates that the substance concentrations of aromatic protein I, aromatic protein II and soluble cell metabolic by-products increased first and then decreased during the reaction process. Starting from 160°C, aromatic protein was degraded in large quantities, and humic acid and fulvic acid-like substances increased significantly.
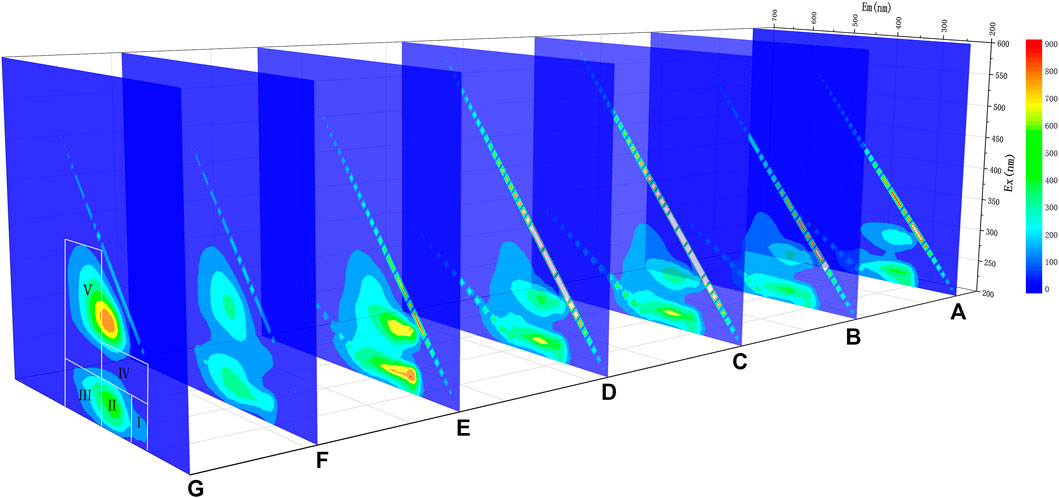
FIGURE 1. 3D-EEM analysis in TH systems at different temperatures [(A) 60°C; (B) 80°C; (C) 100°C; (D) 120°C; (E) 140°C; (F) 160°C; (G) 180°C].
The integral standard volume of fluorescence region and integral standard volume ratio of fluorescence region were estimated using FRI calculation method (Figure 2). Through the analysis, the integral standard volumes
3.1.2 Nitrogen release
The experimental results of nitrogen release with different temperatures are shown in Figure 3A, the thermodynamic model of the release process is shown in Supplementary Appendix Section 3.1. At 60°C–140°C, the change of ammonia nitrogen with temperature is not obvious. After reaching 140°C, the concentration of ammonia nitrogen increases sharply with temperature, with a release rate of 66.4%, which is mainly due to the hydrolysis of soluble proteins in the process of hydrothermal hydrolysis, which generates polypeptides, dipeptides and amino acids. The amino acids were further decarboxylated and deaminated to produce organic acids, ammonia and carbon dioxide (Zhang et al., 2020d). Therefore, the concentration of ammonia nitrogen increases with the increase of hydrothermal hydrolysis temperature and time. From the three-dimensional fluorescence related data, it can be seen that at the temperature range of 60°C–140°C, the aromatic protein in the liquid phase increases greatly with the increase of temperature, and from 140°C–160°C, the aromatic protein in the liquid phase starts to decrease sharply. This reveals that the hydrolysis process is mainly accompanied by: 1) degradation of protein substances in solid increasing soluble protein substances in liquid phase; 2) hydrolysis of soluble organic proteins in liquid phase.
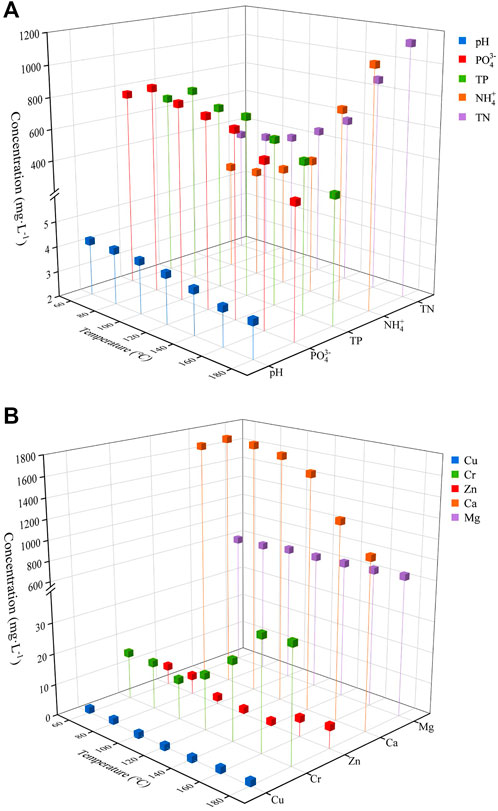
FIGURE 3. Effects of temperature on different elements [(A) nitrogen and phosphorus release, (B) metal cations].
3.1.3 Phosphorus release
As shown in Figure 3A, under the influence of the change of phosphorus release with temperature, pH of manure slurry decreased slightly. This is mainly due to the continuous degradation of organic substances and formation of low molecular acids during the reaction, resulting in the decrease of pH value (Khan et al., 2021). In addition, with the increase of temperature, phosphorus concentration in the aqueous phase tended to increase first and then decrease, similar to the results of the thermodynamic model fitting (Supplementary Appendix Section 3.1). At 80°C, the phosphorus release concentration reached a maximum, and then started to decrease, and the release rate decreased from 49.5% to 21.0%. The release of phosphorus and calcium (Ca) showed a synchronous change pattern (Figure 3B). Some studies have shown that as the temperature increases, organic phosphorus (phytate, polyphosphate, etc.) hydrolyzes to produce orthophosphate, causing the phosphate concentration in the liquid phase to increase, and as the reaction proceeds, phosphate and multivalent metal cations form precipitates, allowing phosphate to be retained in the solid phase (Deng et al., 2020). However, the pH decreased continuously during the reaction process, which inhibited the precipitation of phosphate as Ca compounds. With the increase of temperature, the proportion of humus in the solution increased continuously (Figure 2). And the abundant Ca, magnesium (Mg) and other metals in solution may react with phosphorus and facilitate the formation of humic acid (HA)- Ca-phosphorus complexes with humic acid, resulting in a simultaneous decrease of Ca and phosphorus in solution (Aragón-Briceño et al., 2021).
3.1.4 Changes in metal cations
Figure 3B shows the variation of metal cations in liquid phase with temperature change. It can be seen that with the change of temperature, the Ca concentration in solution is consistent with the changing trend of phosphorus, and Mg concentration remains constant with temperature. Heavy metals including Zn, Cu, and Cr, in the liquid phase show a regular trend of decreasing at first and then increasing, and the turning point was 100°C. The decrease of heavy metals is related to the increasing humic acid content in the solution, resulting in the complexation of metals. From the 3D fluorescence data, it can be seen that with the increase of temperature, the concentration of humic acid substances in the solution increases continuously.
Studies have shown that humic acid contains a variety of oxygen-containing functional groups, mainly in the form of carboxyl, alcoholic hydroxyl, phenolic hydroxyl, carbonyl and methoxy, which can interact with metal cations in the environment through adsorption, complexation, redox, etc. (Du et al., 2020). Studies have shown that humic acid can passivate heavy metals (Chassapis et al., 2009), and its saturated adsorption capacity for heavy metals changes with pH change. With the increase of reaction temperature, a large number of humic acids are continuously produced. Humic acid surface functional groups provide a large number of active sites, with which heavy metals are combined, thus reducing the concentration of heavy metals in solution (Bolan et al., 2011). With the further increase of temperature, the pH in the liquid phase decreases, resulting in the increased competition of H+ with heavy metals for binding sites, thus causing heavy metals in the solution to increase.
3.2 Influence of different acidity treatments on swine manure
3.2.1 Nitrogen release
Figure 4A shows the results of ammonia nitrogen concentration with HCl addition. With increasing concentration of HCl addition, it can be seen that ammonia nitrogen concentration shows a trend of first rising, then falling, and then rising. The increase in ammonia nitrogen concentration in the initial rising stage is mainly due to the addition of acid which promoted the breakdown of organic particle structure of swine manure. With the rapid decrease of pH, the concentration of ammonia nitrogen began to decrease, reaching the lowest value when HCl was added at 7%, but it was still higher than that without HCl. Then the concentration of ammonia nitrogen increased with the amount of HCl added.
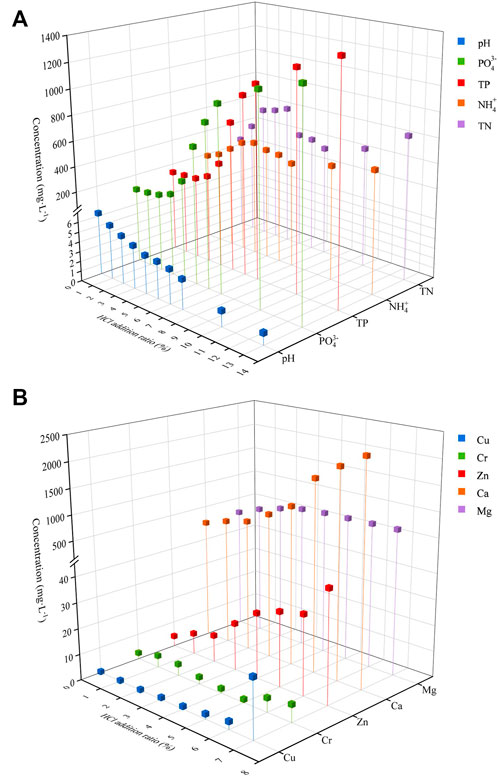
FIGURE 4. Effects of HCl addition on different elements [(A) nitrogen and phosphorus release, (B) metal cations].
Analyzing the reasons for the change in ammonia concentration with increasing HCl addition, when the HCl addition is in the range of 0%–4%, the structure of organic particles in swine manure is effectively destroyed by HCl, increasing the soluble protein in the solution and promoting the hydrolysis of ammonia nitrogen. When the HCl addition is in the range of 4%–6%, the pH reached a range of 3.03–3.75. Proteins are amphoteric electrolytes, and the dissociation state and degree of protein molecules are affected by the pH value of the solution. By changing the pH value of the solution, the charge property of the solution can be altered, the double electric layer and the hydration membrane on the surface of the protein molecule can be destroyed, this will result in an increase in the intermolecular gravitation, so that the solubility of the protein decreases, causing the precipitation of protein. Pan et al. (2022) showed that the solubility of the protein in sewage sludge is the lowest at about pH 3.3. At this point, the pH value is close to the isoelectric point of the protein, which affects the dissolution of the protein, so even the released protein is precipitated in the solid, thus affecting its hydrolysis and causing the concentration of ammonia nitrogen to drop. When the HCl content is 7%–13%, the acidity of the solution is too strong, which will further promote the destruction of organic particles, and at the same time will promote the hydrolysis process of a large number of soluble proteins to amino acids and to ammonia nitrogen (Yadav et al., 2020).
3.2.2 Phosphorus release
Figure 4A shows the liquid phase phosphate concentration at different HCl additions. The inorganic phosphate release ratio was maximized at 79.6% when the addition amount (v/v%) of HCl was 13%. The phosphate content rapidly increased as HCl addition was raised from 3% to 6%, and then the rate of increase decreased until the phosphate release amount gradually reached the maximum value under our experimental condition. The trend of total phosphorus content is consistent with inorganic phosphate. It illustrated that the HCl addition had a significant influence on the phosphate release as reported by other research work (Zhang et al., 2020d).
Phosphorus in manure is mainly present as solid-phase P, as shown by the strong linear relationships between manure P content and solids content. And various phosphorus fractions in manure have remarkably different chemical characteristics. For the first stage of the phosphate release content curve, the insoluble organic phosphorus (phospholipids and phosphate monoesters) can be decomposed to PO43−-P, which increases the concentration of PO43−-P in the liquid phase. In the second stage, it could be surmised that when the addition amount of HCl was over 4%, the structure of the phosphorus-containing solid manure particles began to be significantly broken and dispersed. The formation of protons (H+) by HCl increased as the HCl content continued to increase, resulting in an enhanced dissolution and degradation reaction of organic matter in the liquid phase (Wang et al., 2017). On the one hand, protons (H+) lead to chain reactions, resulting in continuous degradation of organic phosphate (Zhang et al., 2020a). On the other hand, these protons react with inorganic chemical components of P (Ca/P, Fe/P, Al/P) to release phosphate and metal ions. The organic substances released in the liquid with the TH-HCl process, such as humic acid-like substances could react with metal ions (Ca2+) to form complexes, and promote the dissolution reaction to continue.
3.2.3 Changes in metal cations
From the results in Figure 4B, it can be seen that the content of metal cations, Ca and Mg increased continuously with the increase of HCl addition, which on the other hand indicates that phosphorus in swine manure may form corresponding precipitates with metal cations. However, due to the increase of acidity the phosphate precipitation is likely to dissolve to release metal ions (Song et al., 2020). After the thermal reaction, it can be seen that the release of Cu, Zn, and Cr did not increase much and remained relatively constant.
3.3 Influence of H2O2 on the properties of swine manure
3.3.1 Nitrogen release
Figure 5B shows the change of ammonia nitrogen concentration with the addition of H2O2. It can be seen that the ammonia nitrogen concentration also increases first and then decreases with the concentration of hydrogen peroxide. The ammonia nitrogen concentration reaches the maximum rate of 50.8% when the H2O2 addition amount was 7%. Then ammonia nitrogen concentration began to decline, which shows that with the increase of H2O2 concentration, the organic nitrogen in the solution is continuously hydrolyzed, thus increasing the ammonia nitrogen. Compared to the TH-HCl process (Figure 4A), the addition of H2O2 resulted in a more than 3 times increase in the concentration of ammonia nitrogen, indicating that the chemical oxidant can further promote the conversion and dissolution of nitrogen. Then due to the increase of hydrogen peroxide concentration, it will undergo hydroxyl radical scavenging reaction, thus reducing the ammonia nitrogen concentration, as discussed further in Section 3.3.2.
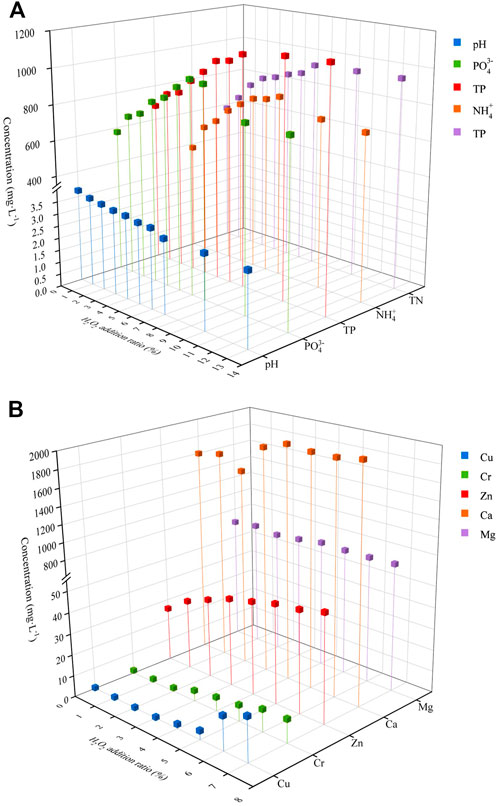
FIGURE 5. Effects of H2O2 addition on different elements [(A) nitrogen and phosphorus release, (B) metal cations].
3.3.2 Phosphorus release
The change of phosphorus concentration with the addition of hydrogen peroxide is depicted in Figure 5A. As can be seen, as the addition of hydrogen peroxide increased, so did the phosphorus release. When the addition amount reached 6%, the concentration of P release reached the maximum of 62.2%., and the concentration of phosphate then started to decline. The concentration of phosphate was still higher even with the addition of 13% hydrogen peroxide than in the absence of hydrogen peroxide addition.
In the process of hydrothermal hydrolysis, the organic matter is degraded mainly by the generation of free radicals (Liu et al., 2021). Adding an appropriate amount of hydrogen peroxide in the process of hydrothermal hydrolysis could significantly increase the oxidation of free radicals in the liquid phase and start a chain reaction (Eqs 4–6); As the amount of hydrogen peroxide increases, the oxidative radicals generated also have more decomposition effect on organophosphorus. When the amount of hydrogen peroxide is too much, the self-decomposition effect of hydrogen peroxide will take place, releasing ·OH, and when the amount of ·OH in the reaction system is too much, the hydroxyl radical scavenging reaction will take place (Han et al., 2022). Moreover, it has been shown that the coupling of HCl and H2O2 can reduce the capture of ∙OH by OH- and ensure the oxidation efficiency (Zhang et al., 2020a).
3.3.3 Changes in metal cations
The concentration of metal cations increased gradually with the increase of hydrogen peroxide addition (Figure 5B). The concentration of Mg did not change with the increase of hydrogen peroxide, while Ca increased slightly with the increase of hydrogen peroxide, and the concentration of Cu did not increase at 0%–5%. When the concentration of H2O2 is 7%, the concentrations of Cu, Zn and Cr increased by 98.1%, 49.8% and 88.0%, respectively. This is mainly because the addition of H2O2 under high temperature and pressure reaction conditions generates more free radicals, which could effectively promote the oxidative decomposition of the organic particle structure of swine manure (Zhang et al., 2020d), thus releasing the heavy metals from the organic particles. In addition, with the addition of hydrogen peroxide, the organic substances in the liquid phase are continuously degraded into low molecular organic acids (Kulikowska and Gusiatin, 2015), and the pH in the liquid phase is synchronously reduced, which also promotes the release of heavy metal cations.
3.3.4 Input electrical energy
As mentioned earlier, thermal hydrolysis of swine manure can dissolve the nutrients in the liquid phase and be used as a liquid fertilizer. To be commercialized as a fertilizer product, its economic feasibility must be considered. As measured by the consumption of electrical energy per unit of phosphorus released, it was calculated that the TH process consumed more electrical energy as the temperature increased, ranging from 102.35–1462.46 KW h m−3. At 140°C, the TH-HCl-H2O2 process consumed a total electrical energy (EE/O) of 1314.79 KW h m−3, with the energy consumption followed: EE/OH2O2 (552.60 KW h m−3)>EE/OHCl (387.57 KW h m−3)>EE/OTH (374.62 KW h m−3), with the catalyst H2O2 consuming the largest equivalent electrical energy contribution. Previous studies have shown that the addition of alkaline reagents leads to a significant increase in the input electrical energy (Zhang et al., 2020d). At a unit price of $0.07 for 1 KW h, the TH-HCl-H2O2 process costs $92.04 to treat one ton of swine manure, which is more economical than other processes, such as the extraction of phosphorus from anaerobic sludge by the HIR sequence, which costs $114.01 per ton of sludge treated (Vardanyan et al., 2018).
3.4 The relationship between nutrients, metals and reaction conditions
Structural equation modelling (SEM) can link multiple causal variables to form a composite system and test the hypothesis of relationships in the system (Yang et al., 2022). In this study, the effects of thermal hydrolysis temperature, H2O2 concentration and HCl concentration on TP, TN and metals were investigated using SEM (Figure 6). The overall goodness of fit of the model fit is indicated by the chi-square value/degree of freedom (Chi/DF = 1.501), relative fit index (NFI = 0.928), and root mean square error of approximation (RMSEA = 0.268) (Zhang et al., 2017). The continuous arrows, dashed arrows, and dot underlined arrows indicate positive significant relationship, non-significant relationship and negative significant relationship, respectively.
The results of structural equation modelling showed that H2O2 and HCl concentrations showed significant positive correlation with TP content in aqueous phase (λ = 0.93, p < 0.001; λ = 0.99, p < 0.001), which may be attributed to the decomposition of organic matter of swine manure and release phosphorus with increasing H2O2 and HCl concentrations (Chen et al., 2003); while temperature showed a significant negative correlation with TP in aqueous phase (λ = −0.84, p < 0.001), which may be attributed to the gradual transfer of phosphorus from the liquid phase to the solid phase with increasing temperature. Both the increase in temperature and HCl concentration significantly increased the release of TN (λ = 0.93, p < 0.001; λ = 0.97, p < 0.001), probably prompting the hydrolysis of soluble proteins (Zhang et al., 2020a), however, the addition of H2O2 had no significant effect on TN content. The influence of the thermal hydrolysis process on the content of the metals mainly results from three aspects: temperature, H2O2 concentration and HCl concentration, as shown in Figure 6. Temperature showed a significant negative correlation with metals (λ = −0.89, p < 0.001), which is attributed to the generation of a large amount of humic acid, which adsorbs metals and makes the content of the metals decrease in aqueous phase. H2O2 and HCl showed a significant positive correlation with metals (λ = 0.99, p < 0.001; λ = 0.88, p < 0.001), however, the processes of metals release promoted by H2O2 and HCl were different. The addition of H2O2 may promote the deconstruction of organic substances during thermal hydrolysis (Kulikowska and Gusiatin, 2015), thus releasing metals, while the addition of HCl may make the content of the metals increase by dissolving the phosphate precipitation produced during thermal hydrolysis. Therefore, to regulate the elemental release characteristics during thermal hydrolysis, multiple influencing factors need to be considered simultaneously.
3.5 Environmental risk evaluation
The ultimate purpose of thermal hydrolysis is to dissolve the nutrients in swine manure into the liquid phase and then apply it to agricultural soil as a liquid fertilizer. To avoid excessive application of fertilizer, which may cause water and air pollution by leaching, fixation and volatilization of nutrients into the environment, it is necessary to conduct environmental risk assessment of fertilizer to accurately grasp the risk level of fertilizer application and strengthen the management of fertilizer application. The nutrients environmental risk index (Ri) and the total risk index (Rt) were calculated for different reaction conditions assuming 60 kg of swine manure to be treated for the preparation of liquid fertilizer and 100 m2 of test soil (i.e., 6,000 kg swine manure per hectare). As seen in Figure 7A, phosphorus in liquid fertilizers generates greater environmental risk, with excess hydrochloric acid (HCl addition ratio >6%) causing phosphorus fertilizer intensity to exceed environmental thresholds and reach light risk (Ri = 0.5) (Hakanson, 1980).
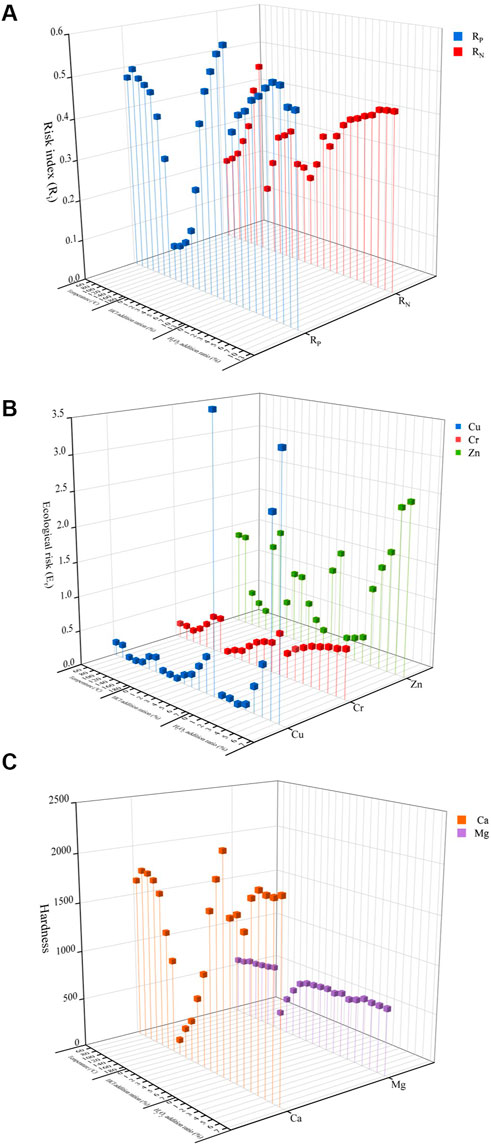
FIGURE 7. Environmental risk under different treatment conditions [(A) Risk index, (B) Ecological risk, (C) Hardness].
To ensure stable and efficient crop growth and to avoid possible environmental risks, heavy metal content in fertilizers is often regulated in agriculture. The ecological risk factor (Er) of heavy metals was calculated for different temperatures, different acidity and different catalyst concentrations (Wu et al., 2021). As seen in Figure 7B, the ecological risks associated with thermal hydrolysis were mainly due to Zn and Cu. The ecological risk factor (Er) inevitably changed with the increase of temperature due to the decrease and then increase of the heavy metal content, as explained in the previous section. At the end of thermal hydrolysis, the ecological risk factors (Er) for the three treatment groups were 2.32, 5.91, and 6.66, respectively, none of which exceeded the low ecological risk threshold (<40) (Qu et al., 2020), and these values suggest that metals in liquid fertilizers after catalytic thermal hydrolysis exhibit low ecological risk and can be used as soil conditioners.
Similarly, when liquid fertilizers are applied to the soil, Mg2+ and Ca2+ may combine with CO32− in the soil to form precipitates, thus affecting their uptake and utilization by plants and reducing their biological effectiveness (Broadley and White, 2010), therefore, attention should be paid to soil characteristics when applying liquid fertilizers. It is important to note that the risk of soil erosion may lead to an increase in water hardness due to ions entering the water body, therefore, the evaluation of the ecological risk indicator for liquid fertilizers Mg2+ and Ca2+ can be analogous to the hardness indicator for water, which in engineering practice is defined as the sum of Mg2+ and Ca2+ concentrations (Tang et al., 2021). The hardness of the liquid fertilizer was calculated for different conditions (Figure 7C), but the concentration of Mg2+ and Ca2+ decreases significantly after absorption and utilization by plants, thus reducing their environmental pollution due to the risk of loss.
4 Conclusion
Thermal hydrolysis (TH) of swine manure resulted in the hydrolysis of organic matter in swine manure and promoted the release of phosphate and ammonia nitrogen. The addition of HCl and H2O2 further accelerated the hydrolysis process of organic phosphorus and protein, compared to the TH process (140°C), the release rates of phosphate and ammonia nitrogen were increased by 17.5% (5% HCl, 6% H2O2) and 26.9% (5% HCl, 7% H2O2), respectively. According to SEM analysis, the concentrations of nutrients and metals increased significantly when the concentrations of HCl and H2O2 were increased. Moreover, calculations showed that applying the thermally hydrolysed swine manure liquid phase to the soil as a liquid fertilizer does not cause environmental risks. It should be emphasized that metal cations show an increasing trend during TH process of swine manure, but still do not exceed the minimum ecological risk threshold. In order to recover the nutrients nitrogen and phosphorus, this study demonstrates a possible route for the TH of livestock manure as a liquid fertilizer. Thus, TH process of animal manures is may be a feasible and sustainable strategy to add value to this waste resource.
Data availability statement
The original contributions presented in the study are included in the article/Supplementary Material, further inquiries can be directed to the corresponding author.
Author contributions
SX: Conceptualization, Investigation, Analysis, Data Curation, Methodology, Writing—original draft. TZ: Supervision, Scientific concept, Guiding, Technical facilities, Foundation, Writing—review and editing. AM: Writing—review and editing. AT: Writing—review and editing. NB: Writing—review and editing.
Funding
The research was sustained by the grant from Government Purchase Service Project of Ministry of Agriculture and Rural Affairs of China (Grant number 202205510310600), the National Key Technology Research and Development Program of China (Grant number 2017YFD0800202), the National Natural Science Foundation of China (Grant Number 31401944).
Acknowledgments
The authors are thankful to International Association of Advanced Materials (IAAM), Sweden for providing international network for carrying out this research.
Conflict of interest
Authors AM and AT were employed by the company Institute of Advanced Materials.
The remaining authors declare that the research was conducted in the absence of any commercial or financial relationships that could be construed as a potential conflict of interest.
Publisher’s note
All claims expressed in this article are solely those of the authors and do not necessarily represent those of their affiliated organizations, or those of the publisher, the editors and the reviewers. Any product that may be evaluated in this article, or claim that may be made by its manufacturer, is not guaranteed or endorsed by the publisher.
Supplementary material
The Supplementary Material for this article can be found online at: https://www.frontiersin.org/articles/10.3389/fenvs.2022.1005290/full#supplementary-material
References
Aragón-Briceño, C. I., Pozarlik, A. K., Bramer, E. A., Niedzwiecki, L., Pawlak-Kruczek, H., and Brem, G. (2021). Hydrothermal carbonization of wet biomass from nitrogen and phosphorus approach: A review. Renew. Energy 171, 401–415. doi:10.1016/j.renene.2021.02.109
Bolan, N. S., Adriano, D. C., Kunhikrishnan, A., James, T., McDowell, R., and Senesi, N. (2011). Dissolved organic matter. Adv. Agron. 110, 1–75. doi:10.1016/B978-0-12-385531-2.00001-3
Bolan, N. S., Szogi, A. A., Chuasavathi, T., Seshadri, B., Rothrock, M. J., and Panneerselvam, P. (2010). Uses and management of poultry litter. World's. Poult. Sci. J. 66 (4), 673–698. doi:10.1017/S0043933910000656
Bolyard, S. C., and Reinhart, D. R. (2017). Evaluation of leachate dissolved organic nitrogen discharge effect on wastewater effluent quality. Waste Manag. 65, 47–53. doi:10.1016/j.wasman.2017.03.025
Broadley, M. R., and White, P. J. (2010). Eats roots and leaves. can edible horticultural crops address dietary calcium, magnesium and potassium deficiencies? Proc. Nutr. Soc. 69, 601–612. doi:10.1017/S0029665110001588
Cai, J., Xia, X. L., Chen, H. B., Wang, T., and Zhang, H. L. (2018). Decomposition of fertilizer use intensity and its environmental risk in China's grain production process. Sustainability 10, 498–515. doi:10.3390/su10020498
Chassapis, K., Roulia, M., and Tsirigoti, D. (2009). Chemistry of metal-humic complexes contained in megalopolis lignite and potential application in modern organomineral fertilization. Int. J. Coal Geol. 78, 288–295. doi:10.1016/j.coal.2009.03.004
Chen, W., Westerhoff, P., Leenheer, J. A., and Booksh, K. (2003). Fluorescence excitation - emission matrix regional integration to quantify spectra for dissolved organic matter. Environ. Sci. Technol. 37, 5701–5710. doi:10.1021/es034354c
Deng, Y., Zhang, T., Clark, J., Aminabhavi, T., Kruse, A., Tsang, D. C. W., et al. (2020). Mechanisms and modelling of phosphorus solid-liquid transformation during the hydrothermal processing of swine manure. Green Chem. 22, 5628–5638. doi:10.1039/D0GC01281E
Deng, Y., Zhang, T., Sharma, B. K., and Nie, H. (2019). Optimization and mechanism studies on cell disruption and phosphorus recovery from microalgae with magnesium modified hydrochar in assisted hydrothermal system. Sci. Total Environ. 646, 1140–1154. doi:10.1016/j.scitotenv.2018.07.369
Ding, Y. F., Sabatini, D. A., and Butler, E. C. (2021). Phosphorus recovery and recycling from model animal wastewaters using materials prepared from rice straw and corn cobs. Water Sci. Technol. 83, 1893–1906. doi:10.2166/wst.2021.094
Du, Q., Zhang, S. S., Song, J. P., Zhao, Y., and Yang, F. (2020). Activation of porous magnetized biochar by artificial humic acid for effective removal of lead ions. J. Hazard. Mat. 389, 122115. doi:10.1016/j.jhazmat.2020.122115
Ekpo, U., Ross, A. B., Camargo-Valero, M. A., and Fletcher, L. A. (2016). Influence of pH on hydrothermal treatment of swine manure: Impact on extraction of nitrogen and phosphorus in process water. Bioresour. Technol. 214, 637–644. doi:10.1016/j.biortech.2016.05.012
Gao, S. M., Lu, D., Qian, T. T., and Zhou, Y. (2021). Thermal hydrolyzed food waste liquor as liquid organic fertilizer. Sci. Total Environ. 775, 145786. doi:10.1016/j.scitotenv.2021.145786
Guo, K. H., Wu, Z. H., Yan, S. W., Yao, B., Song, W. H., Hua, Z. C., et al. (2018). Comparison of the UV/chlorine and UV/H2O2 processes in the degradation of PPCPs in simulated drinking water and wastewater: Kinetics, radical mechanism and energy requirements. Water Res. 147 (15), 184–194. doi:10.1016/j.watres.2018.08.048
Hakanson, L. (1980). An ecological risk index for aquatic pollution control.a sedimentological approach. A sedimentol. approach. Water Res. 14, 975–1001. doi:10.1016/0043-1354(80)90143-8
Han, Z. P., Li, Z., Li, Y., Shang, D. H., Xie, L. B., Lv, Y., et al. (2022). Enhanced electron transfer and hydrogen peroxide activation capacity with N, P-codoped carbon encapsulated CeO2 in heterogeneous electro-fenton process. Chemosphere 287, 132154. doi:10.1016/j.chemosphere.2021.132154
He, G., Liu, X. S., and Cui, Z. L. (2021). Achieving global food security by focusing on nitrogen efficiency potentials and local production. Glob. Food Sec. 29, 100536. doi:10.1016/j.gfs.2021.100536
He, X., Zhang, T., Niu, Y., Xue, Q., Ali, E. F., Shaheen, S. M., et al. (2022). Impact of catalytic hydrothermal treatment and Ca/Al-modified hydrochar on lability, sorption, and speciation of phosphorus in swine manure: Microscopic and spectroscopic investigations. Environ. Pollut. 299, 118877. doi:10.1016/j.envpol.2022.118877
Joardar, J. C., Abdus Salam, A. B., Ashrafuzzaman, M., Sikder, S., and Mahmud, A. (2020). Influence of municipal solid waste compost on yield of tomato applied solely and in combination with inorganic fertilizer where nitrogen is the only variable factor. Malays. J. Sustain. Agric. 5 (1), 29–33. doi:10.26480/mjsa.01.2021.29.33
Karunanithi, R., Szogi, A. A., Bolan, N., Naidu, R., Loganathan, P., Hunt, P. G., et al. (2015). Phosphorus recovery and reuse from waste streams. Adv. Agron. 131, 173–250. doi:10.1016/bs.agron.2014.12.005
Khan, N., Mohan, S., and Dinesha, P. (2021). Regimes of hydrochar yield from hydrothermal degradation of various lignocellulosic biomass: A review. J. Clean. Prod. 288, 125629. doi:10.1016/j.jclepro.2020.125629
Khatun, A., Sikder, S., and Joardar, J. C. (2020). Effect of co-compost made from cattle manure and sawdust on the growth and yield of okra (Abelmoschus Esculentus L.) Malays. J. Sustain. Agric. 4 (1), 36–39. doi:10.26480/mjsa.01.2020.36.39
Kulikowska, D., and Gusiatin, Z. M. (2015). Sewage sludge composting in a two-stage system: Carbon and nitrogen transformations and potential ecological risk assessment. Waste Manag. 38, 312–320. doi:10.1016/j.wasman.2014.12.019
Li, H., Zhang, T., Shaheen, S. M., Abdelrahman, H., Ali, E. F., Bolan, N. S., et al. (2022). Microbial inoculants and struvite improved organic matter humification and stabilized phosphorus during swine manure composting: Multivariate and multiscale investigations. Bioresour. Technol. 351, 126976. doi:10.1016/j.biortech.2022.126976
Liu, Y. Y., Sun, Y. Q., Wan, Z. H., Jing, F. Q., Li, Z. X., Chen, J. W., et al. (2021). Tailored design of food waste hydrochar for efficient adsorption and catalytic degradation of refractory organic contaminant. J. Clean. Prod. 310, 127482. doi:10.1016/j.jclepro.2021.127482
Lu, D., Qian, T. T., Le, C. C., Pan, C. Z., Cao, S. B., Ng, W. J., et al. (2021). Insights into thermal hydrolyzed sludge liquor-identification of plant-growth-promoting compounds. J. Hazard. Mat. 403, 123650. doi:10.1016/j.jhazmat.2020.123650
Mahimairaja, S., Bolan, N. S., Hedley, M. J., and Macgregor, A. N. (1990). Evaluation of methods of measurement of nitrogen in poultry and animal manures. Fertilizer Res. 24 (3), 141–148. doi:10.1007/BF01073582
Murphy, J., and Riley, J. P. (1962). A modified single solution method for the determination of phosphate in natural waters. Anal. Chim. Acta X. 27, 31–36. doi:10.1016/S0003-2670(00)88444-5
Pan, D., and Chen, H. (2021). Border pollution reduction in China: The role of livestock environmental regulations. China Econ. Rev. 69, 101681. doi:10.1016/j.chieco.2021.101681
Pan, W. K., Tang, S., Zhou, J. J., Liu, M. J., Xu, M., Kuzyakov, Y., et al. (2022). Plant-microbial competition for amino acids depends on soil acidity and the microbial community. Plant Soil 475, 457–471. doi:10.1007/s11104-022-05381-w
Qu, J., Zhang, L., Zhang, X., Gao, L., and Tian, Y. (2020). Biochar combined with gypsum reduces both nitrogen and carbon losses during agricultural waste composting and enhances overall compost quality by regulating microbial activities and functions. Bioresour. Technol. 314, 123781. doi:10.1016/j.biortech.2020.123781
Shi, Y., Zhang, T., Ren, H., Kruse, A., and Cui, R. (2018). Polyethylene imine modified hydrochar adsorption for chromium (VI) and nickel (II) removal from aqueous solution. Bioresour. Technol. 247, 370–379. doi:10.1016/j.biortech.2017.09.107
Song, C., Yuan, W., Shan, S., Ma, Q., Zhang, H., Wang, X., et al. (2020). Changes of nutrients and potentially toxic elements during hydrothermal carbonization of pig manure. Chemosphere 243, 125331. doi:10.1016/j.chemosphere.2019.125331
Tang, C., Rygaard, M., Rosshaug, P. S., Kristensen, J. B., and Albrechtsen, H. (2021). Evaluation and comparison of centralized drinking water softening technologies: Effects on water quality indicators. Water Res. 203, 117439. doi:10.1016/j.watres.2021.117439
Vardanyan, A., Kafa, N., Konstantinidis, V., Shin, S. G., and Vyrides, I. (2018). Phosphorus dissolution from dewatered anaerobic sludge: Effect of pHs, microorganisms, and sequential extraction. Bioresour. Technol. 249, 464–472. doi:10.1016/j.biortech.2017.09.188
Wang, T., Zhai, Y., Zhu, Y., Peng, C., Wang, T., Xu, B., et al. (2017). Feedwater pH affects phosphorus transformation during hydrothermal carbonization of sewage sludge. Bioresour. Technol. 245, 182–187. doi:10.1016/j.biortech.2017.08.114
Wu, J., Chen, W., Zhao, Z., Zhang, K., Zhan, Y., Wu, J., et al. (2021). Give priority to abiotic factor of phosphate additives for pig manure composting to reduce heavy metal risk rather than bacterial contribution. Bioresour. Technol. 341, 125894. doi:10.1016/j.biortech.2021.125894
Wu, Q., Wang, H., Zheng, X., Liu, F., Wang, A., Zou, D., et al. (2020). Thermochemical liquefaction of pig manure: Factors influencing on oil. Fuel 264, 116884. doi:10.1016/j.fuel.2019.116884
Xu, H. Q., Guo, L., Zhao, Y. G., Gao, M. C., Jin, C. J., Ji, J. Y., et al. (2021). Accelerating phosphorus release from waste activated sludge by nitrilotriacetic acid addition during anaerobic fermentation process and struvite recovery. Process Saf. Environ. Prot. 147, 1066–1076. doi:10.1016/j.psep.2021.01.033
Yadav, B., Chavan, S., Atmakuri, A., Tyagi, R. D., and Drogui, P. (2020). A review on recovery of proteins from industrial wastewaters with special emphasis on pha production process: Sustainable circular bioeconomy process development. Bioresour. Technol. 317, 124006. doi:10.1016/j.biortech.2020.124006
Yang, X. C., Liu, D. P., Fu, Q., Li, T. X., Hou, R. J., Li, Q. L., et al. (2022). Characteristics of greenhouse gas emissions from farmland soils based on a structural equation model: Regulation mechanism of biochar. Environ. Res. 206, 112303. doi:10.1016/j.envres.2021.112303
Zhang, J. Y., Wang, Z. Y., Wang, Y. W., Zhong, H., Sui, Q. W., Zhang, C. P., et al. (2017). Effects of graphene oxide on the performance, microbial community dynamics and antibiotic resistance genes reduction during anaerobic digestion of swine manure. Bioresour. Technol. 245, 850–859. doi:10.1016/j.biortech.2017.08.217
Zhang, T., He, X., Deng, Y., Tsang, D. C. W., Jiang, R., Becker, G. C., et al. (2020). Phosphorus recovered from digestate by hydrothermal processes with struvite crystallization and its potential as a fertilizer. Sci. Total Environ. 698, 134240. doi:10.1016/j.scitotenv.2019.134240
Zhang, T., He, X., Deng, Y., Tsang, D. C. W., Yuan, H., Shen, J., et al. (2020). Swine manure valorization for phosphorus and nitrogen recovery by catalytic–thermal hydrolysis and struvite crystallization. Sci. Total Environ. 729, 138999. doi:10.1016/j.scitotenv.2020.138999
Zhang, T., Wu, X., Fan, X., Tsang, D. C. W., Li, G., and Shen, Y. (2019). Corn waste valorization to generate activated hydrochar to recover ammonium nitrogen from compost leachate by hydrothermal assisted pretreatment. J. Environ. Manage. 236, 108–117. doi:10.1016/j.jenvman.2019.01.018
Zhang, T., Wu, X., Li, H., Tsang, D. C. W., Li, G., and Ren, H. (2020). Struvite pyrolysate cycling technology assisted by thermal hydrolysis pretreatment to recover ammonium nitrogen from composting leachate. J. Clean. Prod. 242, 118442. doi:10.1016/j.jclepro.2019.118442
Zhang, T., Wu, X., Shaheen, S. M., Zhao, Q., Liu, X., Rinklebe, J., et al. (2020). Ammonium nitrogen recovery from digestate by hydrothermal pretreatment followed by activated hydrochar sorption. Chem. Eng. J. 379, 122254. doi:10.1016/j.cej.2019.122254
Keywords: swine manure slurry, thermal hydrolysis, catalytic, nutrients, metals
Citation: Xie S, Zhang T, Mishra A, Tiwari A and Bolan NS (2022) Assessment of catalytic thermal hydrolysis of swine manure slurry as liquid fertilizer: Insights into nutrients and metals. Front. Environ. Sci. 10:1005290. doi: 10.3389/fenvs.2022.1005290
Received: 28 July 2022; Accepted: 16 September 2022;
Published: 03 October 2022.
Edited by:
Zifu Li, University of Science and Technology Beijing, ChinaReviewed by:
Wenbing Tan, Chinese Research Academy of Environmental Sciences, ChinaZhihua Xiao, Hunan Agricultural University, China
Copyright © 2022 Xie, Zhang, Mishra, Tiwari and Bolan. This is an open-access article distributed under the terms of the Creative Commons Attribution License (CC BY). The use, distribution or reproduction in other forums is permitted, provided the original author(s) and the copyright owner(s) are credited and that the original publication in this journal is cited, in accordance with accepted academic practice. No use, distribution or reproduction is permitted which does not comply with these terms.
*Correspondence: Tao Zhang, dGFvemhhbmdAY2F1LmVkdS5jbg==