- 1Department of Zoology and Entomology, Rhodes University, Makhanda, South Africa
- 2Centre for Invasion Biology (CIB), South African Institute for Aquatic Biodiversity (SAIAB), Makhanda, South Africa
- 3Department of Entomology and Arachnology, Albany Museum, Makhanda, South Africa
- 4DST/NRF Research Chair in Inland Fisheries and Freshwater Ecology, South African Institute for Aquatic Biodiversity (SAIAB), Makhanda, South Africa
- 5Department of Biological Sciences, Faculty of Science, Engineering and Agriculture, University of Venda, Thohoyandou, South Africa
Introduced organisms are seen as one of the greatest threats to resource sustainability worldwide, and aquatic macroinvertebrates are regarded as good indicators of the health of water resources. To explore these two perspectives, the responses of macroinvertebrate faunas to native and introduced fishes in three headwater tributaries of the Keiskamma River system, South Africa, were examined by comparing potential indicator communities in reaches considered to be fishless, reaches invaded by introduced salmonid species, and reaches containing native fishes. Patterns in the macroinvertebrate faunal assemblage data were driven strongly by season and flow rate, and less strongly by the presence of insectivorous fishes and biotope availability, a finding in parallel with several similar studies from the region. This affirms that aquatic macroinvertebrate faunas are responsive indicators of both environmental and biotic factors and leaves room for further studies to resolve the effects of non-native fish in the Keiskamma River system and other similar systems from South Africa.
Introduction
Invasive introduced fishes have been observed globally to be the most pressing threat to native freshwater faunas (Clavero & Garcia-Berthou 2005). Their introduction can precipitate knock-on effects through trophic cascades (Brett & Goldman 1996; Tronstad et al., 2010; Ripple et al., 2016; Lane 2017) that ultimately compromise the sustainability of aquatic resources, from food and water supply to sport and tourism (Ellender & Weyl 2014). South Africa offers opportunities to study this situation because non-native fish species have been introduced into its impoundments and rivers since the late 1800s for the creation of recreational fisheries (de Moor & Bruton 1988; van Rensburg et al., 2011; McCafferty et al., 2012; Ellender & Weyl 2014), and as a result, the country is regarded as one of six global aquatic invasion hotspots where introduced fish account for more than a quarter of freshwater fish species diversity (Leprieur et al., 2008).
In particular, two introduced salmonid sport-fishes, rainbow trout (Oncorhynchus mykiss Walbaum 1792) and brown trout (Salmo trutta L. 1758), are listed in the top 100 of the world’s worst invasive species (Lowe et al., 2000) due to their damaging effects on recipient ecosystems (Cambray 2003). Habitat preferences and physiological tolerances limit their South African distributions to cool, clear, perennial streams and higher-altitude impoundments (Bjornn & Reiser 1991; Armstrong et al., 2003; Ellender et al., 2016), where their effects are generally deemed to exceed anthropogenic effects of pollution and habitat degradation in these rural, montane habitats (Ellender & Weyl 2014; Weyl et al., 2015). Worldwide, introduced trout are reported to affect native faunas negatively through predation and competition, which have been shown to extirpate native vertebrates and invertebrates in New Zealand (Townsend & Crowl 1991; McIntosh et al., 1992; Flecker & Townsend 1994; McIntosh & Townsend 1995; Townsend 1996) and Australia (Lintermans 2000); alter invertebrate community structure and function in Patagonia (Buria et al., 2007; Albariño & Buria 2011); alter invertebrate communities (Herbst et al., 2009; Alexiades & Kraft 2017) and disrupt reciprocal subsidies in North America (Matthews et al., 2002; Epanchin et al., 2010); and exclude native fish (Inoue et al., 2009) and disrupt trophic subsidies in Japan (Baxter et al., 2004). This makes rainbow and brown trout good candidates for studying environmental effects on indicator communities.
Some of these effects have been explored in South Africa (Rivers-Moore et al., 2013; Shelton et al., 2015a, 2015b, 2016). Most work on the ecological effects of trout in southern Africa have focused on native fish and amphibians (e.g. Woodford & Impson 2004; Karssing et al., 2012; Kadye et al., 2013; Avidon et al., 2018), but recent studies have examined the effects of trout on invertebrates. Large-scale field experiments (Shelton et al., 2015a; 2015b) and an in situ mesocosm experiment (Shelton et al., 2016) examined the effects of O. mykiss on the fish and invertebrate faunas of the Breede River catchment in the Cape Fold Ecoregion (sensu Abell et al., 2008). Native fish were found to be stronger regulators of the aquatic invertebrate community than O. mykiss, which preyed more heavily on terrestrial invertebrate subsidies. It was also demonstrated that O. mykiss depleted populations of endemic native barb species (Cyprinidae) through size-selective predation (sensu Rincón & Lobón-Cerviá, 1999), while alleviating predation pressure on grazing invertebrates by usurping the native fish (Shelton et al., 2015a; 2015b).
Macroinvertebrate assemblages are reliable indicators of local ecological processes because of their members’ ubiquity, abundance, rapid life-cycle turnover and biological importance in the food chain (Palmer 1996; Dickens and Graham. 2002). They therefore provide a tool for examining conservation questions. Aquatic macroinvertebrates respond predictably to water temperature, season and water quality (King, 1981; 1983; Rivers-Moore 2012; Eady et al., 2013) and have been used to monitor collateral effects of eradicating introduced fishes (Bellingan et al., 2015; 2019). Trends in invertebrate assemblage composition in response to gradients along the river, including anthropogenic influences, have been described in South Africa (King 1981; Palmer et al., 1991; 1993a; 1993b; 1994) and elsewhere (Wiberg-Larsen et al., 2000), reflecting the expectations of the river continuum concept (RCC: Vannote et al., 1980). The RCC anticipates that autochthonous and allochthonous production in canopy-covered headwaters will be physically broken up by guilds of scraping and shredding invertebrates, and that the resulting particles are washed downstream and further processed by guilds of collector invertebrates (Figure 1). This type of bottom-up trophic chain has been termed a trophic escalade (Lane 2017). The influence of upstream-downstream gradient (i.e.,: the underpinning mechanism of the RCC) must be considered when using macroinvertebrates as monitoring tools. In a study of the macroinvertebrate community under the presence or absence of O. mykiss and S. trutta upstream and downstream of waterfalls along the Sterkspruit, Lotheni and Mooi Rivers in the Drakensberg-Maloti Highlands Ecoregion, South Africa, Rivers-Moore et al. (2013) could not demonstrate conclusively that introduced predatory fish negatively affected their associated macroinvertebrate assemblages, and suggested that their upstream-downstream comparisons may have been confounded by overriding river continuum variables, even though their study was conducted in high altitude, low order streams.
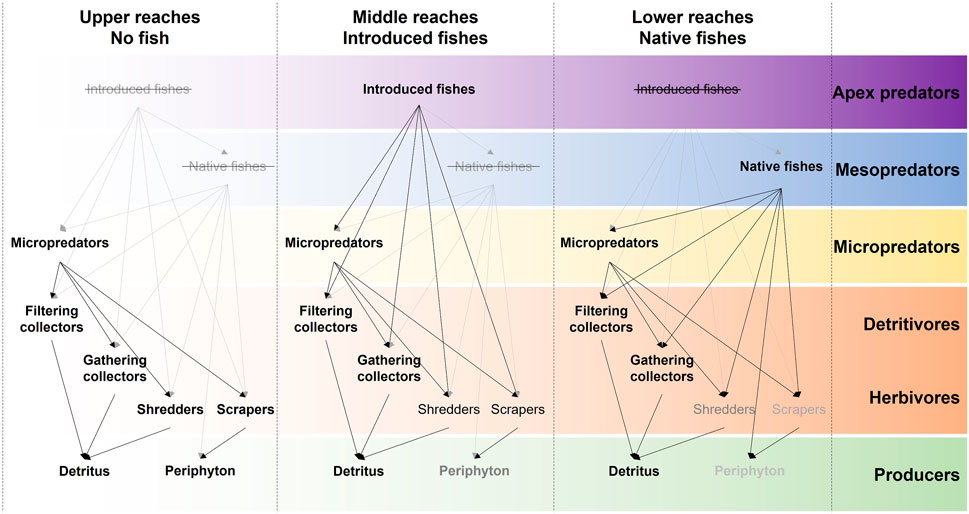
FIGURE 1. Postulated trophic web of the upper Keiskamma river system. The top-down trophic cascade of predators is based on observations by Ellender (2014) and Ellender & Weyl (2014). The distribution of fishes between middle and lower reaches follows Ellender et al. (2016). The bottom-up trophic escalade (Lane 2017) of organic particle processors is a feature of the River Continuum Concept (Vannote et al., 1980). Text and line shade represent differences in particular organismal and resource abundances. For instance, moving downstream, periphyton decreases (due to increased turbidity and decreased light penetration). In the case of insectivorous fishes, strikethrough represents absences in keeping with particular river reach for this study.
The Amatolo-Winterberg Highlands Ecoregion (Abell et al., 2008), where trout have been established for over a century (O. mykiss and S. trutta introduced into the Keiskamma headwaters from 1903—see de Moor & Bruton 1988), provides a unique opportunity to study the effect of native and invasive fish on macroinvertebrates. The distributions of native and introduced fish species in the upper catchment were mapped in detail (Ellender 2014; Ellender et al., 2016), and the uppermost stream reaches of the Cata, Mnyameni and Gwiligwili Rivers are naturally fishless. Brown trout have invaded sections of the Cata (0.01 fish/m−3) and Rainbow trout sections of the Mnyameni (0.1 fish/m−3) rivers, which are separated from the fishless upper reaches by waterfalls and from the lower reaches by impoundments. Below the impoundments on the Cata and Mnyameni, and lower reaches of the Gwiligwili, the rivers are populated by the insectivorous native fish species, Amatolacypris trevelyani (Günther, 1877) (0.2 fish/m³) and Enteromius anoplus (Weber, 1897) (0.004 fish/m³) (see Gaiger 1975; Cambray 1983; Ellender 2014). Macroinvertebrate communities therefore probably experience different predatory regimes and an abiotic environmental gradient, which in turn may result in trophic cascades (Brett & Goldman 1996). This system forms a “natural experiment” sensu DiNardo (2008), where the effects of fish predator regime on macroinvertebrate assemblages can be tested (Figures 1, 2). Indeed, river continuum effects have been reported from the Amatolo-Winterberg Ecoregion (Palmer et al., 1994).
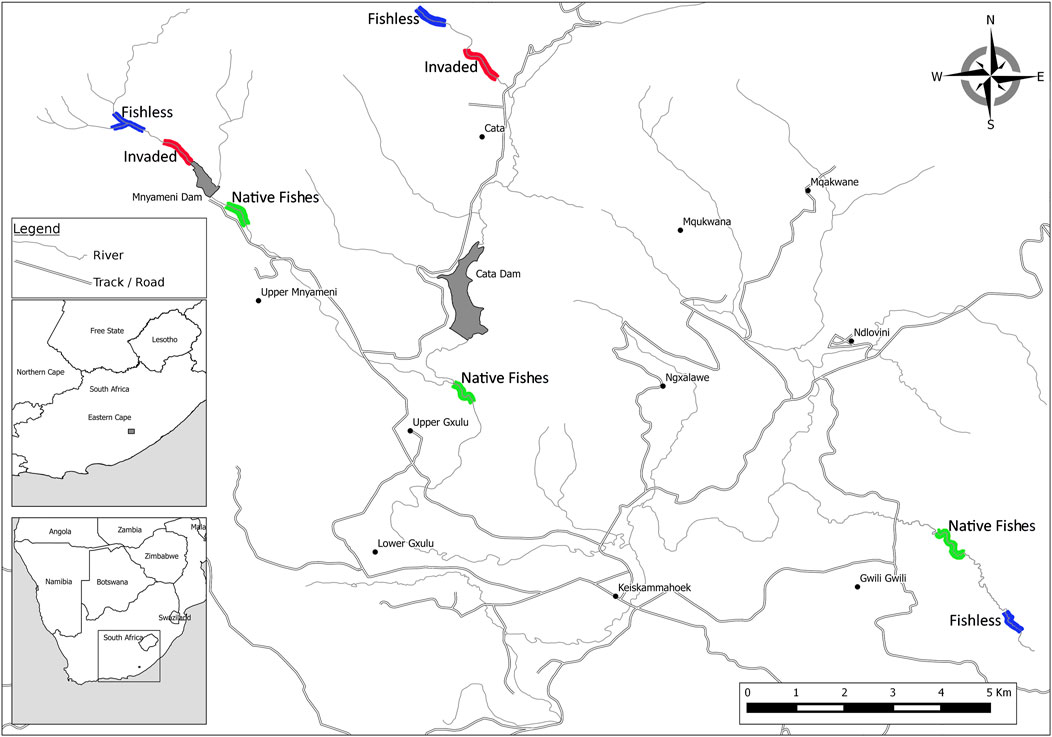
FIGURE 2. Map of the upper Keiskamma river system, Eastern Cape, South Africa, showing the distribution of sampling locations within reaches of stream that were fishless (blue), invaded by non-native trout (red) and reaches containing native fish species (green) described by Ellender’s (2014) study of the system (modified from Ellender (2014).
Both bottom-up environmental gradients and top-down fish predation are likely to drive aquatic macroinvertebrate community structure. The RCC predicts that the dominant functional feeding groups (FFGs) will change from scrapers, shredders and gathering collectors in the upper reaches to filter feeders in the lower reaches as light penetration and the particle sizes of particulate organic matter decrease (cf. Vannote et al., 1980). Macroinvertebrate species richness and diversity should increase toward the lower reaches as food resources diversify (Clarke et al., 2008). Conversely, the effects of predatory fish may decrease macroinvertebrate diversity through a trophic cascade (cf. Ripple et al., 2016). Certain functional feeding groups like gathering collectors and micropredators (i.e. larger macroinvertebrates) will be disproportionally affected by predation by fish (Flecker & Allan 1984; Meissner & Muotka 2006). Moreover, the invasive trout and the native fish communities may have different effects on macroinvertebrates. On the one hand, trout may have a greater impact on native macroinvertebrates if they have a higher functional response compared to native fish species (Alexander et al., 2014). On the other hand, as found previously, trout may be more dependent on terrestrial food sources (Li et al., 2016) and native fish may have a greater impact on aquatic macroinvertebrates (Ripple et al., 2016).
We examined the response of native aquatic macroinvertebrates to predation pressure by native and invasive insectivorous fish species in three headwater tributaries of the Keiskamma River system in the Eastern Cape, by examining variation in macroinvertebrate species richness, diversity, and community composition. We hypothesise that the macroinvertebrate community is predominantly structured by a longitudinal environmental gradient along the river (bottom-up hypothesis), taking seasonal effects into account. Specifically, the following patterns were expected from bottom-up drivers, following predictions from the RCC:
1) Species from the functional feeding groups scrapers, shredders and gathering collectors should be more abundant where forest canopy covers the stream and input of leaflitter is greater (Vannote et al., 1980).
2) Filtering collectors should increase in abundance toward the lower reaches as food particle size decreases (Vannote et al., 1980).
3) Species richness and diversity should increase towards the lower reaches, due to greater quantities and variety of food resources (Clarke et al., 2008; Ellender et al., 2016).
Conversely, we hypothesise the macroinvertebrate community is structured by insectivorous fishes (top-down hypothesis). The following patterns were expected from top-down drivers:
4) Invertebrate taxa, especially large-bodied species from the predator and gathering collector groups, should be less abundant in the presence of fish (Flecker & Allan 1984; Meissner & Muotka 2006).
5) Species richness and diversity should decrease in river reaches containing fish (e.g., Flecker & Townsend 1994).
6) The degree of predation impact differs between introduced and native fish species (Alexander et al., 2014; Ripple et al., 2016).
Methods
Study area
The riverine habitat consisted of three tributaries of the Keiskamma River, in the Eastern Cape, South Africa: the Cata, Gwiligwili and Mnyameni Rivers (Figure 2), which are swift-flowing streams of 3rd-, 2nd- and 2nd-order, respectively. Reaches selected for sampling macroinvertebrate assemblages were based on the distribution of fishes along these streams, following Ellender (2014). The riverbanks of the fishless reaches of all three rivers, and the invaded reaches of the Mnyameni and Cata Rivers, were completely shaded by a canopy of indigenous mist-belt forest (Mucina et al., 2006), a source of allochthonous primary production in the rivers. The riparian vegetation along the native fish reaches of the Gwiligwili, Cata and Mnyameni rivers was Savannah Thornveld with sparsely distributed indigenous Podocarpus (Podocarpaceae) trees.
The fishless reach of the Mnyameni River was characterized by large, fairly deep pools broken up by short riffles over cobbled beds; this reach thus has the greatest average depth of 0.35 ± 0.16 m, and the highest pool-to-riffle ratio (Table 1). The Gwiligwili River was shallowest, ranging from 0.06 ± 0.03 to 0.07 ± 0.04 m deep along the fishless and native fish reaches, respectively. The fishless and invaded reaches of the Cata River were similar in substrate proportions, while the native fish reach contained the highest proportion of riffle compared to the remaining sites (Table 1). The mean stream width ranged from 7.80 ± 3.30 m at the fishless Mnyameni reach to 1.2 ± 0.4 m at the fishless Gwiligwili reach. The Gwiligwili River, being a narrower stream with a smaller catchment, flowed less strongly than the Cata and Mnyameni Rivers, with flow increasing downstream as the stream profile widened.
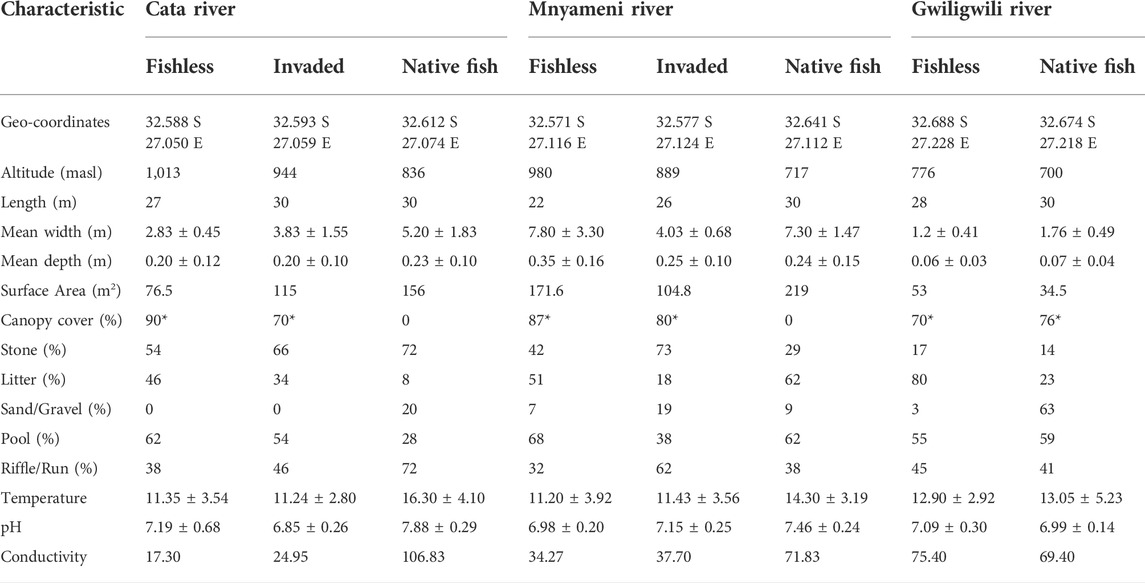
TABLE 1. Summary of the location, habitat and physicochemical characteristics (mean ± SD) for eight reaches sampled quarterly on three headwater tributaries of the Keiskamma River from July 2012 - April 2013. Values marked with an asterisk (*) indicate qualitative estimations.
Sampling, field measurements and taxon classification
Sampling was carried out quarterly, in July and October of 2012, and January and April of 2013, corresponding to winter, spring, summer and autumn seasons. For each season, we collected ten samples in each reach in each river from sites roughly 30 m long (Table 1; Figure 2). Samples were collected with a modified Surber sampling technique (Hauer & Resh 1996). Two frames of 300 mm × 300 mm were constructed from polyvinyl chloride (PVC) tubing and supported 120 mm apart by clear Perspex panes, to allow a channel of water to flow from upstream to downstream through the Surber box and into the net. A long-handled water net with frame dimensions of 300 mm × 300 mm and mesh size 500 μm, known commonly as a standard ‘SASS’ net (Dickens & Graham 2002), was held flush behind the box and the substrate within the box area was disturbed vigorously by hand for 1 minute to dislodge invertebrates. The water flowing through the box carried the invertebrates into the net. Where stream flow was low or negligible, water was flushed manually through the box sampler. At each sample, the flow from the area of stream was recorded using a Marsh McBirney, Inc. Flo-Mate (Model 2000) portable electromagnetic flow meter and flow was categorised, based on current speed, as no flow (0 m/s), slow flow (0.05–0.3 m/s), medium flow (0.3–0.5 m/s) and fast flow (>0.5 m/s), as flow is a key differentiator between biotope type; visually estimated the percentage canopy cover; and categorised biotope from which the sample was collected as stones, litter or gravel, broadly following Wadeson & Rowntree (1998) and Dickens & Graham (2002) (Table 1). Conductivity, pH and temperature were recorded at each site and each sampling event (Hanna HI98219 Combo pH and electrical conductivity meter, HANNA Instruments Inc., Woonsocket, United States) (Table 1).
Macroinvertebrates were identified to species or morphospecies level in the laboratory using published keys for the southern African region (for example, see Barber-James & Lugo-Ortiz 2003; de Moor & Scott 2003), except for earthworms (Oligochaeta) and roundworms (Nematoda) that were identified to subclass level. Further, we allocated the insect species to functional feeding groups (filtering collectors, gathering collectors, predators, scrapers and shredders) following Cummins & Klug (1979) and Merritt & Cummins (1996), with additional input from, e.g., Agnew (1962) and Palmer et al. (1993a).
Analysis of species composition
We used generalised linear models for multivariate abundance data (‘manyglm’ from R package ‘mvabund’, Wang et al., 2020), with a negative binomial distribution family, to study the relationship between macroinvertebrate community composition and river reaches with different fish predation regimes (hereafter referred to as “river reach”), flow rate, canopy cover, temperature, season, biotope, pH, depth and electroconductivity. Preliminary analyses using manyglm and Bayesian ordination (see below) indicated negligible differences in species composition between rivers, and we therefore focused on the longitudinal gradient, disregarding river identity. Compared to distance-based methods, model-based multivariate methods do not require data transformation, are not affected by differences in β-diversity among groups, and are not disproportionately influenced by a few relatively highly abundant species (Warton et al., 2012). Nevertheless, only species present in 5% or more of all samples were included in the species matrix.
Collinearity between predictor variables was examined to identify redundant variables before constructing the model (function ‘vif’, R package ‘car’, Fox & Weisberg 2019), and the model was further simplified using a backwards stepwise model selection procedure and Akaike’s information criterion values to identify the combination of predictor variables that best explained variation in species composition. A Dunn-Smyth residuals-fitted values plot and a quantile-quantile plot was used to evaluate whether the model was appropriate for the data (Dunn & Smyth 1996; Wang et al., 2012). Results were expressed as a marginal analysis of variance (each predictor is tested after all other predictors have been taken into account), with Rao’s score test statistics and p-values that have been adjusted to account for correlation among species.
Manyglm also supplied univariate regression output for each species with p-values adjusted for multiple testing, which we used for a more detailed analysis of those species most affected by the presence of fish or the environmental gradient, noting each species’ functional group. This allowed us to probe the potential mechanisms underlying any significant community ensemble response (see predictions 1, 2 and 4).
To illustrate the results, we used a Bayesian ordination technique (function ‘boral’ from R package ‘boral’, Hui 2020) that complements manyglm, where no predictor variables are specified except for a fixed row-effect and two latent variables that represent the scores for an ordination plot with two axes (Hui 2016). The predictor variables found to best explain variation in species composition were then superimposed on the ordination plot with functions ‘ordispider’ to indicate groups, and ‘envfit’ to indicate vectors for continuous variables (R package ‘vegan’, Oksanen et al., 2019).
Analysis of species richness and diversity
We examined variation in macroinvertebrate species richness and diversity among the three predation regimes by constructing species accumulation curves (Mao Tau estimate: Colwell et al., 2012) and Tsallis’s diversity accumulation curves (Tsallis 1988) equivalent to the Shannon diversity index (R vegan’s ‘specaccum’ and ‘tsallisaccum’ respectively) based on all recorded species (see predictions 3 and 5). We were primarily interested in the effect of invasive or native insectivorous fish presence (i.e. differences between river reaches), but we used the findings from the species composition analyses to verify whether it is reasonable to pool samples from different seasons. Accumulation curves were more useful than single index values because Gwiligwili lacks trout and consequently the trout-invaded reaches are represented by fewer samples than the fishless or native fish reaches. Accumulation curves were fitted to the asymptotic Lomolino model (Dengler 2009) to compare estimated richness and diversity values at equal sample sizes, and to estimate total species richness or diversity (curve asymptotes) under the hypothetical condition of complete sampling.
Finally, the presence or absence of rare species and singletons (i.e., those species that were not included in the species matrix for manyglm) may contribute to the differences in species richness among river reaches (Spiller & Schoener 1998; Thompson & Withers 2003; Lopez et al., 2012). Such species on their own do not supply enough data to study with regression or multivariate analyses (e.g., manyglm) and their absence may be falsely recorded due to sampling error or low detectability. However, following from predictions 1, 2 and 4, if multiple species from certain functional groups are consistently excluded from certain river reaches, this may be reflected in different patterns of species richness among functional groups. Therefore, we used species accumulation curves to determine the species richness of each separate functional group of each river reach at 20 samples (to equalize sampling effort in the different river reaches).
Results
Species composition
Temperature was collinear with other predictors (variance inflation factor >20.03); without temperature, all remaining variables’ variance inflation factors were <5.4. Forty-five of the 104 taxa (or morphospecies) of macroinvertebrate identified from the samples taken were included in the species matrix for species composition analyses (Supplementary Table S1). Based on Akaike’s information criterion values (lower indicating better model fit), the combination of predictor variables that best explain variation in macroinvertebrate composition was season, flow rate, river reach, biotope, canopy cover, conductivity and pH (Table 2). The Dunn-Smyth residuals plot and the quantile-quantile plot indicated that the model was appropriate for the data (Supplementary Figure S1). River reach was the third most important variable after season and flow rate (Table 2). Consequently, we created separate Bayesian ordinations for each season with river reach indicated, and we added flow rate as a vector (numerically ordered from no flow to fast flow), along with vectors for conductivity, canopy cover and pH (Figure 3). Biotope was not illustrated to prevent overcomplicating Figure 3. The bottom-up hypothesis was supported in all seasons, with regard to the influence of the upstream-downstream environmental changes in canopy cover, conductivity and pH on macroinvertebrate composition. River reach remained a significant predictor of macroinvertebrate composition after all environmental variables have been taken into account, suggesting support for the top-down hypothesis where different river reaches largely represents different predation regimes. However, the strength of this effect varied among seasons, e.g., with macroinvertebrate composition overlapping more in autumn and being more differentiated in spring (Figure 3). Further, macroinvertebrate composition may vary among reaches due to environmental variables that have not been included in this study, necessitating the further supporting univariate analyses.
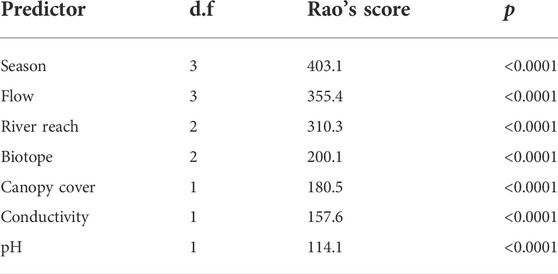
TABLE 2. General results from a multivariate abundance generalised linear model with the combination of predictor variables that best explain variation in macroinvertebrate species composition. Results are expressed as a marginal analysis of variance, with model degrees of freedom (d.f.), Rao’s score test statistic, and p-value for each predictor. Residual d.f. = 302.
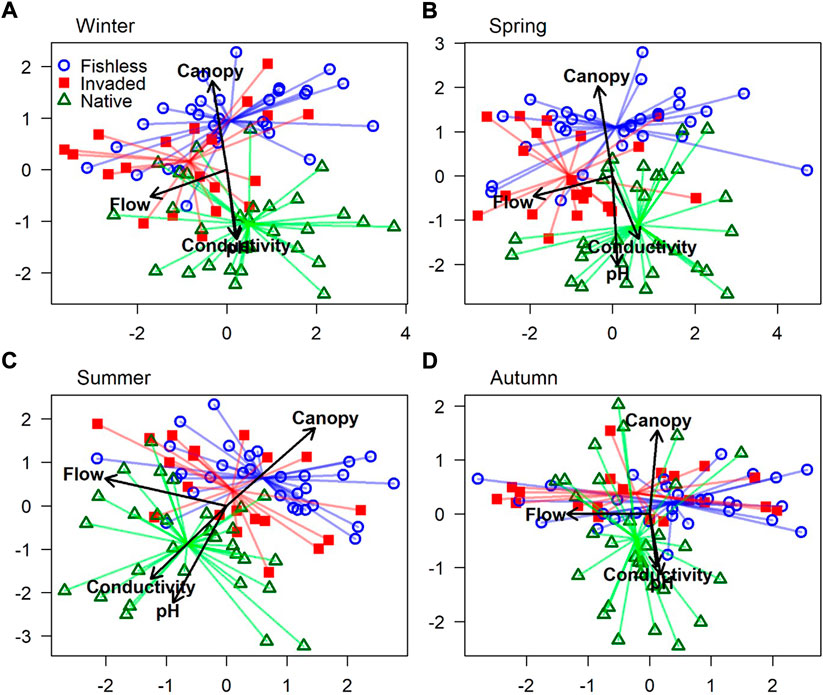
FIGURE 3. Bayesian ordination plots, for (A). Winter, (B). Spring, (C). Summer and (D). Autumn, based on latent variable 1 (x-axis) and 2 (y-axis) showing macroinvertebrate composition in river reaches without fish, invaded by trout, and inhabited by native fish. Ordered flow rate class, electroconductivity, percentage canopy cover and pH are illustrated with vectors.
A closer look at the individual species with significant relationships with one or more predictor variables indicated four gathering collectors, two predators, and seven scrapers that varied significantly with river reach after other predictors have been taken into account (Table 3, see complete univariate results in Supplementary Table S1). Supplementary Figure S2 illustrates how each of these 13 macroinvertebrates varied among river reaches in each season—predicted abundance values were extracted from the multivariate generalised linear model where all predictors except river reach (i.e.,: insectivorous fish regime) and season were kept constant. Cheleocloeon excisum and Baetis harrisoni (Ephemeroptera: Baetidae), Castanophlebia sp. 1 (Ephemeroptera: Leptophlebiidae), Chironominae sp. 1 (Diptera: Chironomidae), Aeshna sp. 1 (Odonata: Aeshnidae), and Dugesia sp. 1 (Platyhelminthes: Dugesiidae) appeared to be more abundant in the native fish reach. Afroptilum sudafricanum (Ephemeroptera: Baetidae), Adenophlebia sp. 1 (Ephemeroptera: Leptophlebiidae), Afronurus harrisoni (Ephemeroptera: Heptageniidae), and Lestagella penicillata (Ephemeroptera: Teloganodidae) were less abundant in the reaches with invasive and native fish. Adenophlebia sp. 1 and Cloeodes sp. 1 (Ephemeroptera: Baetidae) were less abundant in the invaded reach than in the native fish reach. Conversely, Burnupia sp. 1 (Mollusca: Ancylidae) and Euthraulus sp. 1 (Ephemeroptera: Leptophlebiidae) were more abundant in the invaded fish reach.
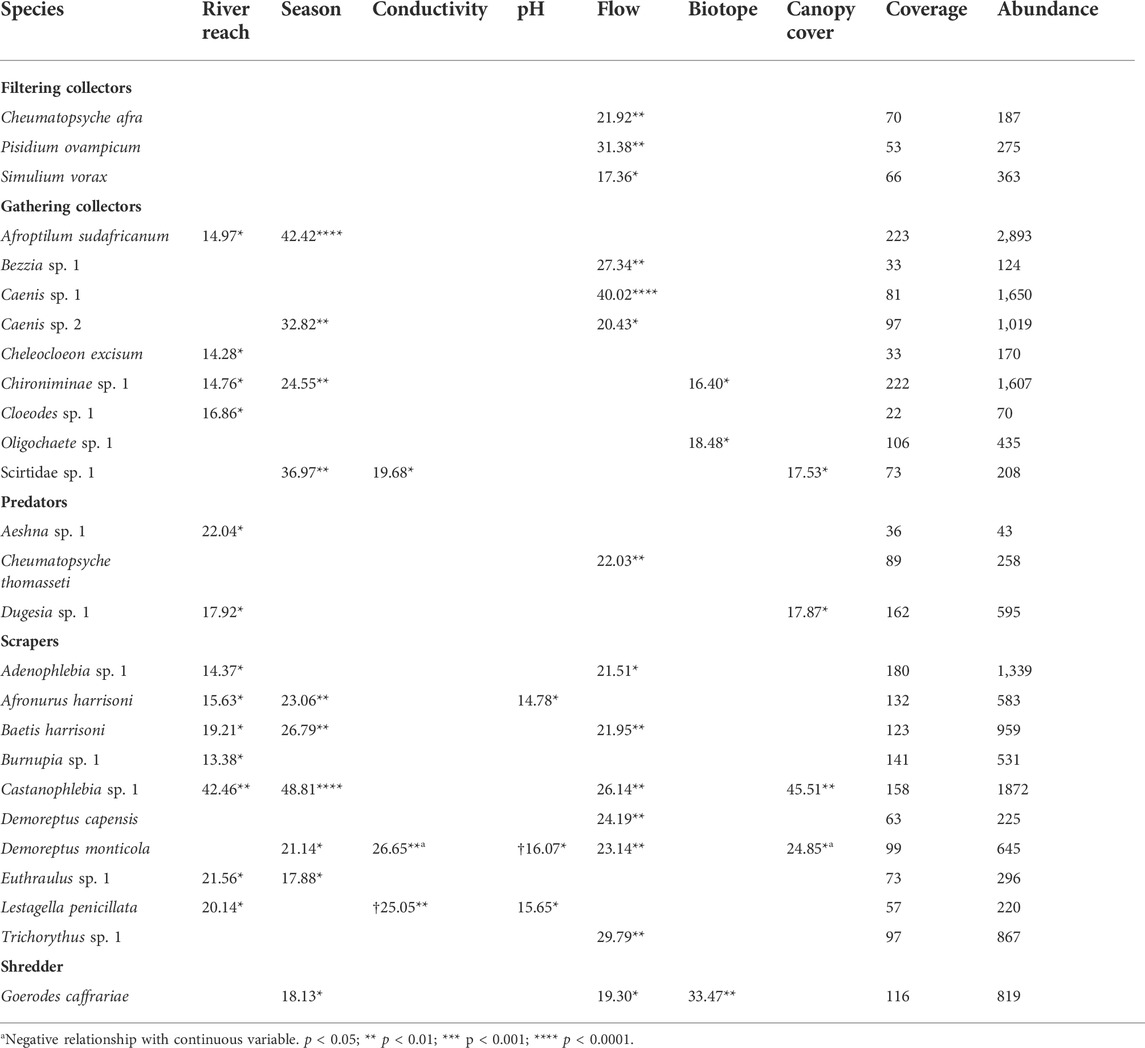
TABLE 3. Univariate results (marginal analysis of variance) for those species that had a significant relationship with one or more of the predictor variables. Rao’s score test statistics are given, and p-value, adjusted for multiple testing, is symbolised using asterisks. Coverage indicates the number of samples (out of 320) each species was present in, while abundance reflects the total count of individuals across all samples.
Species richness and diversity
Following the strong seasonal influence found for species composition and abundance, species richness and Shannon diversity in river reaches with different predation regimes were examined separately for different seasons (Table 4; Figures 4, 5 and Supplementary Figure S3). Support for the bottom-up and top-down hypotheses were found; however strength of support varied among season as both species richness and diversity fluctuated over seasons, with differences among river reaches especially prominent in summer (Table 4; Figures 4, 5). Species richness and diversity in the native fish reach were their lowest out of the three reaches in winter, intermediate between the other reaches in spring, and highest of the three reaches in summer (Table 4; Figures 4, 5). Species richness in the invaded reach was lowest of the three reaches in all seasons except winter (Table 4; Figure 4). Species diversity in the invaded reach was highest in winter and spring, intermediate between the other reaches in summer, and lowest in autumn (Table 4; Figure 5).
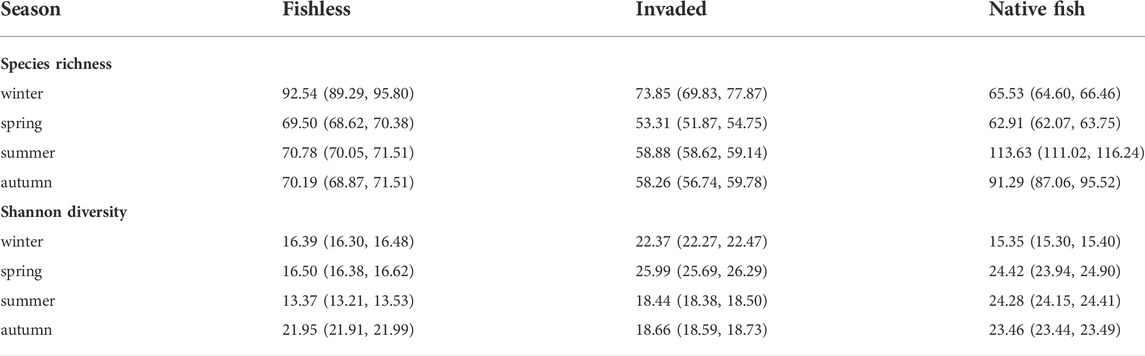
TABLE 4. Asymptotes of Lomolino models fitted to species accumulation and Tsallis’s diversity accumulation curves, represent estimated species richness and Shannon diversity, respectively, under condition of complete sampling. Values in brackets indicate the 95% confidence interval.
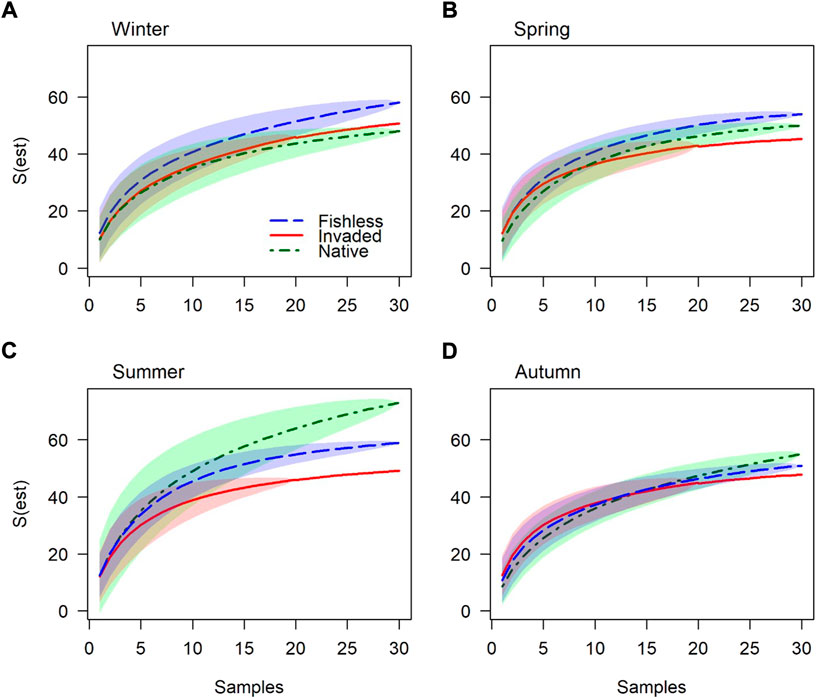
FIGURE 4. Macroinvertebrate species richness accumulation curves for river reaches without fish, invaded by trout, and inhabited by native fish, for (A). Winter, (B). Spring, (C). Summer and (D). Autumn. Standard deviation is indicated by shaded areas. Species richness between 20 and 30 samples in the invaded reach were extrapolated from the Lomolino model.
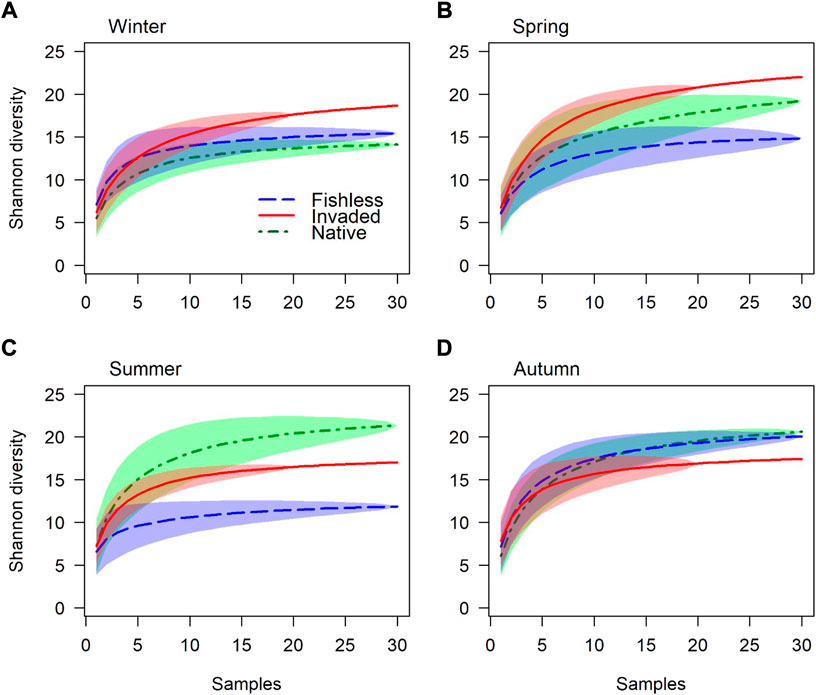
FIGURE 5. Macroinvertebrate Tsallis’s accumulation curves equivalent to Shannon diversity, for river reaches without fish, invaded by trout, and inhabited by native fish, for (A). Winter, (B). Spring, (C). Summer and (D). Autumn. Standard deviation is indicated by shaded areas. Species richness between 20 and 30 samples in the invaded reach were extrapolated from the Lomolino model.
A strong seasonal influence on patterns of variation in species richness was also observed within functional groups (Figure 6). In the fishless reach, species richness was greatest for filtering collectors in autumn, gathering collectors in winter and spring, predators in winter, scrapers in spring and summer and shredders in winter and spring (Figure 6). In the native fish reach, species richness was greatest for filtering collectors in summer, gathering collectors in summer and autumn, predators in spring and summer, and scrapers in autumn (Figure 6). In the invaded reach, species richness was lowest for filtering collectors in summer, gathering collectors in spring and predators in winter, summer and autumn (Figure 6).
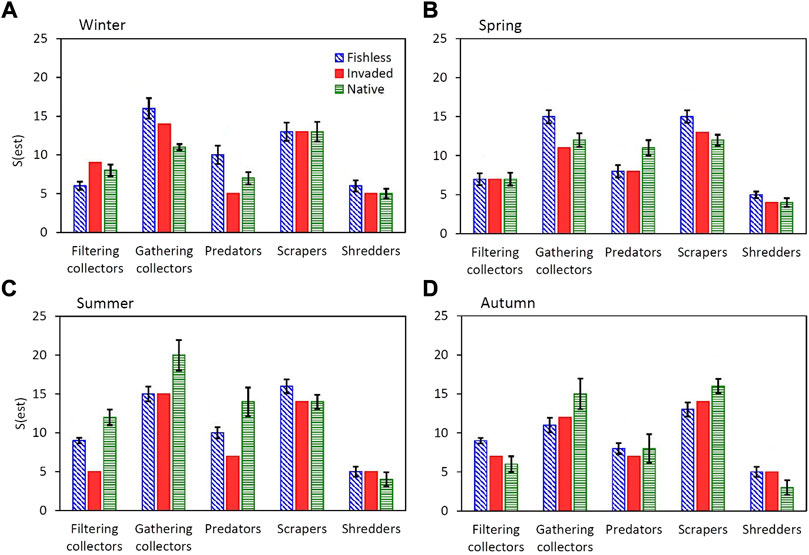
FIGURE 6. Species richness from accumulation curves at 20 samples for (A). Winter, (B). Spring, (C). Summer and (D). Autumn, for separate functional groups per fishless, invaded and native fish river reaches. Error bars indicate standard deviations of S(est). See Supplementary Table S2 for further detail.
Discussion
The RCC predicts that the structure of macroinvertebrate assemblages changes along the gradient of streams (cf. Figure 1), particularly when longitudinal linkages are strong, as is the case for pristine forested headwater streams moving towards foothill reaches (Vannote et al., 1980; Poole 2002; Arthington 2012). Based on variation in species composition, abundance of certain common species, species richness and diversity, and contributions from different functional groups, our findings generally indicate that environmental and seasonal variation are dominant drivers of macroinvertebrate community structure in these headwater tributaries of the Keiskamma River—the differences observed between upper fishless, middle trout-invaded and lower reaches with native fish can largely be attributed to the upper-to-lower environmental gradient (favouring predictions 1, 2, and 3).
Nevertheless, although it was difficult to disentangle the effects of the cohabiting fish fauna from the environmental gradient, we found some compelling patterns of variation in abundance and presence of specific species and functional groups that suggest both detractive and augmentative effects of fish presence. We found some evidence in support of both the bottom-up and top-down hypotheses, depending on the species or functional group considered, and strongly modulated by season.
Season and, secondarily, flow rate were the most important predictors of variation in species composition. Seasonal changes can be expected in the macroinvertebrates comprising the species matrix, which includes many univoltine species for which abundance and biomass may vary over orders of magnitude during the year. The degree of overlap between macroinvertebrate composition of the different reaches varied among seasons, e.g. with a greater overlap between reaches observed in autumn and clearer differences between reaches observed in spring (Figure 3). Flow rate interacts with river morphology and substrate and affects the distribution of food particles and dissolved oxygen, so its influence on species composition is expected (Brittain & Eikeland, 1988). Flow rate is expected to decrease along the river continuum, but in the Keiskamma tributaries flow rate varied within river reaches instead of across them (Figure 3).
After season and flow rate, river reach was the third most important variable, followed by biotope, canopy cover, pH and conductivity. The effect of biotope may be due to the different degrees of protection that are offered to inhabitants by different biotopes over and above spatial, phylogenetic and predation effects (Clarke et al., 2008). Canopy cover, pH and conductivity all changed along the upper-lower environmental gradient (Table 1; Figure 3); canopy cover also represents where shredders’ predominant food sources enters the river. River reach, a proxy for fish presence, remains an important predictor after bottom-up environmental variables have been taken into account. However, river reach may still cover other environmental gradient variables that were not explicitly measured in the current study, like availability and variety of particulate food and interactions with other invertebrates (Richardson 1991). Therefore, interpreting the possible effect of fish presence requires a closer look at the responses of specific species to identify mechanisms of action.
Thirteen macroinvertebrate taxa were found to vary significantly among river reaches, with an apparent seasonal influence on degree of variation, after all other environmental predictors have been taken into account (Supplementary Figure S2). Nine of these species were mayflies, which are known to be influenced by predatory fish. For example, mayflies may show avoidance behaviour in response to predatory fish (Culp et al., 1991; Cowan & Peckarsky 1994; McIntosh & Townsend 1994; Huhta et al., 2000), abundance of Baetis mayflies was reduced in streams occupied by S. trutta (Meissner & Muotka 2006), and rainbow trout have altered mayfly distributions (Albariño & Buria 2011). Nevertheless, even for these thirteen species, effects from fish predation were difficult to confirm. For example, the mayfly A. sudafricanum, a gathering collector, decreased in abundance from upper to lower reaches—such a pattern is not necessarily due to suppression by predatory fish, as it could be expected from the river continuum concept (cf. Figure 1). Further, some species, like C. excisum (gathering collector), Chironominae sp. 1 (gathering collector), Aeshna sp. 1 (predator) and Baetis harrisoni (scraper), were more abundant in the native fish reaches, possibly due to a stronger predation effect by trout compared to native fish, but more likely because of a greater variety and abundance of food in the native fish reaches (Ellender et al., 2016). The possible influence of insectivorous fish presence was clearer in Cloeodes sp. 1 (gathering collector), and Adenophlebia sp. 1 (scraper), which were least abundant in the invaded reach, whereas the limpet mollusc Burnupia sp. 1 (scraper) and the mayfly Euthraulus sp. 1 (scraper) seem to thrive in the presence of trout, perhaps because of a trophic cascade involving the absence of native fish species and certain other competing invertebrates (cf. Figure 1).
Patterns of variation in species richness and diversity among river reaches also generally varied with season (Table 4); in some cases, patterns even reversed in different seasons, e.g., for gathering collectors in spring and autumn (Figure 6). Some observed patterns suggest bottom-up environmental drivers of aquatic macroinvertebrate faunas; for example, higher species richness and diversity in the native reach in summer and autumn (Table 4; Figures 4–6), perhaps due to greater quantities and variety of food resources and greater primary productivity at higher temperatures (Ellender et al., 2016). In contrast, lower species richness and diversity in the invaded and native reaches may, in some cases, suggest a suppressing impact from fish predation. Species richness and diversity of all species together in winter, and filtering collector species richness in autumn, was lowest in the native reach, suggesting predation by native fish (Figures 4–6). Further, species richness in spring, summer and autumn, species diversity in autumn, and species richness of predators (winter, summer and autumn), gathering collectors (spring) and filtering collectors (summer), was lowest in the invaded reach, suggesting a greater negative impact on macroinvertebrates by trout compared to native fish (Figures 4–6). However, a decrease in species richness from the upper to lower reaches for gathering collectors (winter), scrapers (spring) and shredders (all seasons) is expected from the RCC and the detractive effect of fish predation could not be established or ruled out where such patterns were found (Figure 6).
Conclusion
Although multiple bottom-up and top-down drivers and interactions structure the macroinvertebrate assemblages in the Keiskamma River headwaters (Figure 1), a few consistent effects could be identified when synthesising the outcomes of the species composition, abundance, richness and diversity analyses.
First, environmental variables appear the strongest drivers of the aquatic macroinvertebrate assemblages of the Keiskamma River headwaters—favouring predictions 1–3; however, patterns of abundance and presence of certain species and functional groups suggest that predation by introduced trout and native fishes could significantly influence the structure and dynamics of the macroinvertebrate community—favouring predictions 4–6. Evidence of an effect of fish predation on macroinvertebrate communities were similarly subtle in other studies. For example, Herbst et al. (2009) found no difference in invertebrate densities in fishless streams compared to paired trout streams, but they reported lower invertebrate richness and diversity from invaded streams. Flecker & Allan (1984) found that substrate type determined the abundance, richness or density of stream macroinvertebrates, while only the largest macroinvertebrates were affected by predation. Locally, Rivers-Moore et al. (2013) examined macroinvertebrate assemblages for the effects of trout specifically and concluded that overriding environmental factors related to upstream/downstream effects made detecting the effects of introduced salmonids on macroinvertebrates difficult.
Second, although introduced predators have been shown to have a greater impact on prey species than natives (e.g., Alexander et al., 2014), we could not confirm in this study that trout had a greater detractive influence (through predation) on macroinvertebrates, as it may simply be easier to distinguish unexpectedly higher or lower values in the middle reach from the background environmental gradient.
Third, patterns of species composition, richness and diversity varied strongly with season, probably due to large variations in abundance of univoltine macroinvertebrates. However, there may be other possible explanations, including competition among macroinvertebrates due to seasonal variation in food resources (e.g., Richardson 1991), and seasonal changes in predation intensity, particularly by trout, which may switch seasonally between terrestrial and aquatic food sources (Li et al., 2016; Milardi et al., 2016).
The experimental design employed during this study examined predation by salmonids under “natural” circumstances (a natural experiment sensu DiNardo 2008), where the densities of neither prey nor predator were experimentally manipulated, in systems where trout have formed self-sustaining, wild, populations. Further scope exists for exploring the relative strengths of top-down predation and bottom-up environmental effects in these streams, particularly investigating intra-annual variation in food resources and competitive interactions for both macroinvertebrates and fish. This may only be achievable through manipulative experiments, e.g., by exclusion of trout within invaded reaches (e.g., Allan 1982), or through the use of stable isotopes. Moreover, as shown in this study, more insight can be provided by studies recording intra- and inter-annual variation, compared to single-season studies.
Data availability statement
The original contributions presented in the study are included in the Supplementary Material, further inquiries can be directed to the corresponding author.
Ethics statement
The animal study was reviewed and approved by South African Institute for Aquatic Biodiversity (SAIAB) Animal Ethics Committee.
Author contributions
TB conceptualized the study, collected the field and laboratory data, and drafted and finalized the documentation. SH conducted the analyses and drafted and finalized the documentation. MV supervised, drafted and finalized the documentation. OW conceptualized and funded the study and drafted the documentation.
Funding
This work was financially and logistically supported by the National Research Foundation (NRF) of South Africa, DST-NRF—South African Research Chairs Initiative of the Department of Science and Technology, Grant Nos. 110507: Professional Development Programme Grant Nos. 104911; the DST-NRF Centre of Excellence in Invasion Biology.
Acknowledgments
We warmly thank the Amatola Wild Trout fishing guides at Cata: Luyolo Tete, Luthando Mboso and also Siyathemba Nkume, Jimba Ham, Brendon Dredge, Gareth Snyman for assistance in the field; Boniswa Tontsi and sis’ uNomvume and her family for welcoming researchers to stay in her home; Bosupeng Motshegoa, Ekhona Ntloko, Mizu and Sirius for assistance in the laboratory; Helen Barber-James, John Midgley (Albany Museum) and Tony Dold (Schonland Herbarium) for taxonomic assistance; Cassandra Barker for academic guidance; We acknowledge use of infrastructure and equipment provided by the South African institute for Aquatic Biodiversity (SAIAB). Two reviewers are also acknowledged and thanked for valuable comments made towards the improvement of the manuscript. Any opinion, findings and conclusions or recommendations expressed in this material are those of the author and do not necessarily reflect the views of the NRF.
Conflict of interest
The authors declare that the research was conducted in the absence of any commercial or financial relationships that could be construed as a potential conflict of interest.
Publisher’s note
All claims expressed in this article are solely those of the authors and do not necessarily represent those of their affiliated organizations, or those of the publisher, the editors and the reviewers. Any product that may be evaluated in this article, or claim that may be made by its manufacturer, is not guaranteed or endorsed by the publisher.
Supplementary material
The Supplementary Material for this article can be found online at: https://www.frontiersin.org/articles/10.3389/fenvs.2022.1004939/full#supplementary-material
References
Abell, R., Thieme, M. L., Revenga, C., Bryer, M. T., Kottelat, M., Bogutskaya, N. G., et al. (2008). Freshwater ecoregions of the world: A new map of biogeographic units for freshwater biodiversity conservation. BioScience 58, 403–414. doi:10.1641/b580507
Agnew, J. D. (1962). The distribution of Centroptiloides bifasciata (E. P.) (Baetidae: Ephemeroptera) in southern Africa, with ecological observations on the nymphs. Hydrobiologia 20, 367–372. doi:10.1007/bf00033357
Albariño, R. J., and Buria, L. M. (2011). Altered mayfly distribution due to strong interactions with alien rainbow trout in Andean streams of Patagonia. Limnologica 41, 220–227. doi:10.1016/j.limno.2010.07.004
Alexander, M. E., Dick, J. T., Weyl, O. L., Robinson, T. B., and Richardson, D. M. (2014). Existing and emerging high impact invasive species are characterized by higher functional responses than natives. Biol. Lett. 10, 20130946. doi:10.1098/rsbl.2013.0946
Alexiades, A. V., and Kraft, C. E. (2017). Effects of stocked trout on stream invertebrate communities. J. Freshw. Ecol. 32, 95–102. doi:10.1080/02705060.2016.1248502
Allan, J. D. (1982). The effects of reduction in trout density on the invertebrate community of a mountain stream. Ecology 63, 1444–1455. doi:10.2307/1938871
Armstrong, J. D., Kemp, P. S., Kennedy, G. J. A., Ladle, M., and Milner, N. J. (2003). Habitat requirements of Atlantic salmon and Brown trout in rivers and streams. Fish. Res. 62, 143–170. doi:10.1016/s0165-7836(02)00160-1
Arthington, A. H. (2012). “Environmental flows: Saving rivers in the third millennium,” in Freshwater ecology series (Berkley, California, USA: University of California Press), 406.
Avidon, S., Shelton, J., Marr, S., Bellingan, T., Esler, K., and Weyl, O. L. F. (2018). Preliminary evaluation of non-native rainbow trout (Oncorhynchus mykiss) impact on the Cederberg ghost frog (Heleophryne depressa) in South Africa’s Cape Fold Ecoregion. Afr. J. Aquatic Sci. 43, 313–318. doi:10.2989/16085914.2018.1507898
Barber-James, H. M., and Lugo-Ortiz, C. R. (2003). “Ephemeroptera,” in Guides to the freshwater invertebrates of southern Africa. Volume 7: Insecta I. Ephemeroptera, Odonata & Plecoptera. Editors I. J. de Moor, J. A. Day, and F. C. de Moor (Pretoria, South Africa: Water Research Commission), 288.
Baxter, C. V., Fausch, K. D., Murakami, M., and Chapman, P. L. (2004). Fish invasion restructures stream and forest food webs by interrupting reciprocal prey subsidies. Ecology 85, 2656–2663. doi:10.1890/04-138
Bellingan, T. A., Hugo, S., Woodford, D. J., Gouws, J., Villet, M. H., and Weyl, O. L. F. (2019). Rapid recovery of macroinvertebrates in a South African stream treated with rotenone. Hydrobiologia 834, 1–11. doi:10.1007/s10750-019-3885-z
Bellingan, T. A., Woodford, D., Gouws, J., Villet, M. H., and Weyl, O. L. F. (2015). Rapid bioassessment of the effects of repeated rotenone treatments on invertebrate assemblages in the Rondegat River, South Africa. Afr. J. Aquatic Sci. 40, 89–94. doi:10.2989/16085914.2014.984651
Bjornn, T. C., and Reiser, D. W. (1991). “Chapter 4: Habitat requirements of salmonids in streams,” in Influences of forest and rangeland Management on salmonid fishes and their habitats. Editor W. R. Meehan (Bathesda, Maryland, U.S.A: American Fisheries Society), 83–138.
Brett, M. T., and Goldman, C. R. (1996). A meta-analysis of the freshwater trophic cascade. Proc. Natl. Acad. Sci. U. S. A. 93, 7723–7726. doi:10.1073/pnas.93.15.7723
Brittain, J. E., and Eikeland, T. J. (1988). Invertebrate drift: A review. Hydrobiologia 166, 77–93. doi:10.1007/bf00017485
Buria, L., Albariño, R., Villanueva, V. D., Modenutti, B., and Balseiro, E. (2007). Impact of exotic rainbow trout on the benthic macroinvertebrate community from Andean-Patagonian headwater streams. Fundam. Appl. Limnol. 168, 145–154. doi:10.1127/1863-9135/2007/0168-0145
Cambray, J. A. (1983). The feeding habits of minnows of the genus Barbus (Pisces, Cyprinidae) in Africa, with special reference to Barbus anoplus Weber. J. Limnological Soc. South. Afr. 9, 12–22. doi:10.1080/03779688.1983.9639406
Cambray, J. A. (2003). The global impact of alien trout species – A review; with reference to their impact in south Africa. Afr. J. Aquatic Sci. 28, 61–67. doi:10.2989/16085914.2003.9626601
Clarke, A., Mac Nally, R., Bond, N., and Lake, P. S. (2008). Macroinvertebrate diversity in headwater streams: A review. Freshw. Biol. 53, 1707–1721. doi:10.1111/j.1365-2427.2008.02041.x
Clavero, M., and Garcia-Berthou, E. (2005). Invasive species are a leading cause of animal extinctions. Trends Ecol. Evol. 20, 110. doi:10.1016/j.tree.2005.01.003
Colwell, R. K., Chao, A., Gotelli, N. J., Lin, S. Y., Mao, C. X., Chazdon, R. L., et al. (2012). Models and estimators linking individual-based and sample-based rarefaction, extrapolation and comparison of assemblages. J. Plant Ecol. 5, 3–21. doi:10.1093/jpe/rtr044
Cowan, C. A., and Peckarsky, B. L. (1994). Diel feeding and positioning periodicity of a grazing mayfly in a trout stream and a fishless stream. Can. J. Fish. Aquat. Sci. 51, 450–459. doi:10.1139/f94-047
Culp, J. M., Glozier, N. E., and Scrimgeour, G. J. (1991). Reduction of predation risk under the cover of darkness: Avoidance responses of mayfly larvae to a benthic fish. Oecologia 86, 163–169. doi:10.1007/bf00317527
Cummins, K. W., and Klug, M. J. (1979). Feeding ecology of stream invertebrates. Annu. Rev. Ecol. Syst. 10, 147–172. doi:10.1146/annurev.es.10.110179.001051
de Moor, F. C., and Scott, M. (2003). “Trichoptera,” in Guides to the freshwater invertebrates of southern Africa. Editors I. J. de Moor, J. H. Day, and F. C. de Moor (Pretoria, South Africa: Water Research Commission), 8, 209. Insecta II: Hemiptera, Megaloptera, Neuroptera, Trichoptera & Lepidoptera.
de Moor, I. J., and Bruton, M. N. (1988). Atlas of alien and translocated indigenous aquatic animals of southern Africa. South African National Scientific Program Report No. 144. Pretoria, South Africa: Council for Scientific and Industrial Research, 310.
Dengler, J. (2009). Which function describes the species-area relationship best? A review and empirical evaluation. J. Biogeogr. 36, 728–744. doi:10.1111/j.1365-2699.2008.02038.x
Dickens, C. W., and Graham, P. M. (2002). The South African Scoring System (SASS) Version 5 rapid bioassessment method for rivers. Afr. J. Aquatic Sci. 27, 1–10. doi:10.2989/16085914.2002.9626569
DiNardo, J. (2008). “Natural experiments and quasi-natural experiments,” in The New Palgrave Dictionary of Economics. Editors S. N. Durlauf, and L. E. Blume. 2nd ed. (London, United Kingdom: Palgrave Macmillan), 856–864.
Dunn, P. K., and Smyth, G. K. (1996). Randomized quantile residuals. J. Comput. Graph. Statistics 5, 236–244. doi:10.2307/1390802
Eady, B., Rivers-Moore, N. A., and Hill, T. (2013). Relationship between water temperature predictability and aquatic macroinvertebrate assemblages in two South African streams. Afr. J. Aquatic Sci. 38, 163–174. doi:10.2989/16085914.2012.763110
Ellender, B. R. (2014). “Ecological consequences of non-native fish invasion in Eastern Cape headwater streams,” in Doctor of Philosophy Thesis (Grahamstown, South Africa: Rhodes University), 224.
Ellender, B. R., Rivers-Moore, N. A., Coppinger, C. R., Bellingan, T. A., and Weyl, O. L. F. (2016). Towards using thermal stress thresholds to predict salmonid invasion potential. Biol. Invasions 18, 3513–3525. doi:10.1007/s10530-016-1244-9
Ellender, B. R., and Weyl, O. L. F. (2014). A review of current knowledge, risk and ecological impacts associated with non-native freshwater fish introductions in South Africa. Aquat. Invasions 9, 117–132. doi:10.3391/ai.2014.9.2.01
Epanchin, P. N., Knapp, R. A., and Lawler, S. P. (2010). Non-native trout impact an alpine-nesting bird by altering aquatic-insect subsidies. Ecology 91, 2406–2415. doi:10.1890/09-1974.1
Flecker, A. S., and Allan, J. D. (1984). The importance of predation, substrate and spatial refugia in determining lotic insect distributions. Oecologia 64, 306–313. doi:10.1007/bf00379126
Flecker, A. S., and Townsend, C. R. (1994). Community-wide consequences of trout introduction in New Zealand streams. Ecol. Appl. 4, 798–807. doi:10.2307/1942009
Fox, J., and Weisberg, S. (2019). An {R} companion to applied regression. Third Edition. Thousand Oaks, California, USA: Sage.
Gaigher, I. G. (1975). The ecology of a minnow, Barbus trevelyani (pisces: Cyprinidae) in the tyume river, eastern Cape. Ann. Cape Prov. Mus. Nat. Hist. 11, 1–19.
Hauer, F. R., and Resh, V. H. (1996). “Chapter 16: Benthic macroinvertebrates,” in Methods in stream ecology. Editors F. R. Hauer, and G. A. Lamberti (San Diego, California, U.S.A: Academic Press), 339–370.
Herbst, D. B., Silldorff, E. L., and Cooper, S. D. (2009). The influence of introduced trout on the benthic communities of paired headwater streams in the Sierra Nevada of California. Freshw. Biol. 54, 1324–1342. doi:10.1111/j.1365-2427.2009.02187.x
Huhta, A., Muotka, T., and Tikkanen, P. (2000). Nocturnal drift of mayfly nymphs as a post-contact antipredator mechanism. Freshw. Biol. 45, 33–42. doi:10.1046/j.1365-2427.2000.00615.x
Hui, F. K. C. (2016). boral: Bayesian ordination and regression analysis of multivariate abundance data in R. Methods Ecol. Evol. 7, 744–750. doi:10.1111/2041-210x.12514
Hui, F. K. C. (2020). boral: Bayesian ordination and regression AnaLysis. Methods Ecol. Evol. 7, 744–750. R package version 1.9. doi:10.1111/2041-210X.12514
Inoue, M., Miyata, H., Tange, Y., and Taniguchi, Y. (2009). Rainbow trout (Oncorhynchus mykiss) invasion in Hokkaido streams, northern Japan, in relation to flow variability and biotic interactions. Can. J. Fish. Aquat. Sci. 66, 1423–1434. doi:10.1139/f09-088
Kadye, W. T., Chakona, A., Marufu, L. T., and Samukange, T. (2013). The impact of non-native rainbow trout within Afro-montane streams in eastern Zimbabwe. Hydrobiologia 720, 75–88. doi:10.1007/s10750-013-1624-4
Karssing, R. J., Rivers-Moore, N. A., and Slater, K. (2012). Influence of waterfalls on patterns of association between trout and Natal cascade frog Hadromophryne natalensis tadpoles in two headwater streams in the uKhahlamba Drakensberg Park World Heritage Site, South Africa. Afr. J. Aquatic Sci. 37, 107–112. doi:10.2989/16085914.2012.666381
King, J. M. (1983). Abundance, biomass and diversity of benthic macro-invertebrates in a Western Cape river, South Africa. Trans. R. Soc. S. Afr. 45, 11–34. doi:10.1080/00359198309520092
King, J. M. (1981). The distribution of invertebrate communities in a small South African river. Hydrobiologia 83, 43–65. doi:10.1007/bf02187150
Lane, P. A. (2017). A review of the trophic cascade concept using the lens of loop analysis: “The truth is the whole”. Food Webs 13, 1–11. doi:10.1016/j.fooweb.2017.10.002
Leprieur, F., Beauchard, O., Blanchet, S., Oberdorff, T., and Brosse, S. (2008). Fish invasions in the world’s river systems: when natural processes are blurred by human activities. PLoS Biol. 6, e28. doi:10.1371/journal.pbio.0060028
Li, J. L., Gerth, W. J., Van Driesche, R. P., Bateman, D. S., and Herlihy, A. T. (2016). Seasonal and spatial fluctuations in Oncorhynchus trout diet in a temperate mixed-forest watershed. Can. J. Fish. Aquat. Sci. 73, 1642–1649. doi:10.1139/cjfas-2015-0520
Lintermans, M. (2000). Recolonization by the mountain galaxias Galaxias olidus of a montane stream after the eradication of rainbow trout Oncorhynchus mykiss. Mar. Freshw. Res. 51, 799–804. doi:10.1071/mf00019
Lopez, L. C., de Aguiar Fracasso, M. P., Mesquita, D. O., Palma, A. R. T., and Riul, P. (2012). The relationship between percentage of singletons and sampling effort: A new approach to reduce the bias of richness estimates. Ecol. Indic. 14, 164–169. doi:10.1016/j.ecolind.2011.07.012
Lowe, S., Browne, M., Boudjelas, S., and De Poorter, M. (2000). “100 of the world’s worst invasive alien species: A selection from the global invasive species database,” in Invasive species specialist group (ISSG), a specialist group of the species survival commission (New Zealand: SSC of the World Conservation Union IUCN, University of Auckland), 12.
Matthews, K. R., Knapp, R. A., and Pope, K. L. (2002). Garter snake distributions in high-elevation aquatic ecosystems: Is there a link with declining amphibian populations and non-native trout introductions? J. Herpetology 36, 16–22. doi:10.1670/0022-1511(2002)036[0016:gsdihe]2.0.co;2
McCafferty, J. R., Ellender, B. R., Weyl, O. L. F., and Britz, P. (2012). The use of water resources for inland fisheries in South Africa. Water sa. 38, 327–344. doi:10.4314/wsa.v38i2.18
McIntosh, A. R., Townsend, C. R., and Crowl, T. A. (1992). Competition for space between introduced Brown trout (Salmo trutta L.) and native galaxiid (Galaxias vulgaris Stokel) in a New Zealand stream. J. Fish. Biol. 41, 63–81. doi:10.1111/j.1095-8649.1992.tb03170.x
McIntosh, A. R., and Townsend, C. R. (1995). Impacts of an introduced predatory fish on mayfly grazing in New Zealand streams. Limnol. Oceanogr. 40, 1508–1512. doi:10.4319/lo.1995.40.8.1508
McIntosh, A. R., and Townsend, C. R. (1994). Interpopulation variation in mayfly antipredator tactics: Differential effects of contrasting predatory fish. Ecology 75, 2078–2090. doi:10.2307/1941612
Meissner, K., and Muotka, T. (2006). The role of trout in stream food webs: Integrating evidence from field surveys and experiments. J. Animal Ecol. 75, 421–433. doi:10.1111/j.1365-2656.2006.01063.x
Merrit, R. W., and Cummins, K. W. (1996). “Trophic relations of macroinvertebrates,” in Methods in stream ecology. Editors F. R. Hauer, and G. A. Lamberti (San Diego, California, U.S.A: Academic Press), 435–474.
Milardi, M., Thomas, S. M., and Kahilainen, K. K. (2016). Reliance of brown trout on terrestrial prey varies with season but not fish density. Freshwater Biology 61 (7), 1143–1156. doi:10.1111/fwb.12775
Mucina, L., and Rutherford, M. C. (2006). The vegetation of South Africa, Lesotho and Swaziland. Pretoria: South African National Biodiversity Institute, 807.
Oksanen, J., Blanchet, F. G., Friendly, M., Kindt, R., Legendre, P., McGlinn, D., et al. (2019). vegan: Community ecology package. R package version 2.5-6, Available at: https://CRAN.R-project.org/package=vegan.
Palmer, C. G., O’Keeffe, J. H., and Palmer, A. R. (1991). Are macroinvertebrate assemblages in the Buffalo River, southern Africa, associated with particular biotopes? J. North Am. Benthol. Soc. 10, 349–357. doi:10.2307/1467662
Palmer, C. G., O’Keeffe, J. H., Palmer, A. R., Dunne, T., and Radloff, S. E. (1993b). Macroinvertebrate functional feeding groups in the middle and lower reaches of the Buffalo River, Eastern Cape, South Africa. I. Dietary variability. Freshw. Biol. 29, 441–453. doi:10.1111/j.1365-2427.1993.tb00778.x
Palmer, C. G., O’Keeffe, J. H., and Palmer, A. R. (1993a). Macroinvertebrate functional feeding groups in the middle and lower reaches of the Buffalo River, eastern Cape, South Africa. II. Functional morphology and behaviour. Freshw. Biol. 29, 455–462. doi:10.1111/j.1365-2427.1993.tb00779.x
Palmer, C. G., Palmer, A. R., O’Keeffe, J. H., and Palmer, R. W. (1994). Macroinvertebrate community structure and altitudinal changes in the upper reaches of a warm temperate southern African river. Freshw. Biol. 32, 337–347. doi:10.1111/j.1365-2427.1994.tb01130.x
Palmer, R. W. (1996). Invertebrates in the Orange River, with emphasis on conservation and management. South. Afr. J. Aquatic Sci. 22, 3–51. doi:10.1080/10183469.1996.9631371
Poole, G. C. (2002). Fluvial landscape ecology: Addressing uniqueness within the River discontinuum. Freshw. Biol. 47, 641–660. doi:10.1046/j.1365-2427.2002.00922.x
Richardson, J. S. (1991). Seasonal food limitation of detritivores in a montane stream: an experimental test. Ecology 72 (3), 873–887. doi:10.2307/1940589
Rincón, P. A., and Lobón-Cerviá, J. (1999). Prey-size selection by Brown trout (Salmo trutta L.) in a stream in northern Spain. Can. J. Zool. 77, 755–765. doi:10.1139/z99-031
Ripple, W. J., Estes, J. A., Schmitz, O. J., Constant, V., Kaylor, M. J., Lenz, A., et al. (2016). What is a trophic cascade? Trends Ecol. Evol. 31, 842–849. doi:10.1016/j.tree.2016.08.010
Rivers-Moore, N. A., Fowles, B., and Karssing, R. J. (2013). Impacts of trout on aquatic macroinvertebrates in three Drakensberg rivers in KwaZulu-Natal, South Africa. Afr. J. Aquatic Sci. 38, 93–99. doi:10.2989/16085914.2012.750592
Rivers-Moore, N. A. (2012). Turnover patterns in fish versus macroinvertebrates - implications for conservation planning. Afr. J. Aquatic Sci. 37, 301–309. doi:10.2989/16085914.2012.708857
Shelton, J. M., Samways, M. J., and Day, J. A. (2015b). Non-native rainbow trout change the structure of benthic communities in headwater streams of the Cape Floristic Region, South Africa. Hydrobiologia 745, 1–15. doi:10.1007/s10750-014-2067-2
Shelton, J. M., Samways, M. J., and Day, J. A. (2015a). Predatory impact of non-native rainbow trout on endemic fish populations in headwater streams in the Cape Floristic Region of South Africa. Biol. Invasions 17, 365–379. doi:10.1007/s10530-014-0735-9
Shelton, J. M., Samways, M. J., Day, J. A., and Woodford, D. J. (2016). Are native cyprinids or introduced salmonids stronger regulators of benthic invertebrates in South African headwater streams? Austral Ecol. 41, 633–643. doi:10.1111/aec.12352
Spiller, D. A., and Schoener, T. W. (1998). Lizards reduce spider species richness by excluding rare species. Ecology 79, 503–516. doi:10.1890/0012-9658(1998)079[0503:lrssrb]2.0.co;2
Thompson, G. G., and Withers, P. C. (2003). Effect of species richness and relative abundance on the shape of the species accumulation curve. Austral Ecol. 28, 355–360. doi:10.1046/j.1442-9993.2003.01294.x
Townsend, C. R., and Crowl, T. A. (1991). Fragmented population structure in a native New Zealand fish: An effect of introduced Brown trout? Oikos 61, 347. doi:10.2307/3545242
Townsend, C. R. (1996). Invasion biology and ecological impacts of Brown trout Salmo trutta in New Zealand. Biol. Conserv. 78, 13–22. doi:10.1016/0006-3207(96)00014-6
Tronstad, L. M., Hall, R. O., Koel, T. M., and Gerow, K. G. (2010). Introduced lake trout produced a four-level trophic cascade in Yellowstone Lake. Trans. Am. Fish. Soc. 139, 1536–1550. doi:10.1577/t09-151.1
Tsallis, C. (1988). Possible generalization of Boltzmann-Gibbs statistics. J. Stat. Phys. 52, 479–487. doi:10.1007/bf01016429
Van Rensburg, B. J., Weyl, O. L. F., Davies, S. J., van Wilgen, N. J., Spear, D., Chimimba, C. T., et al. (2011). “Invasive vertebrates of South Africa,” in Biological invasions. Editor D. Pimentel. 2nd edn. (Boca Raton, Florida, U.S.A: CRC Press), 325–378.
Vannote, R. L., Minshall, G. W., Cummins, K. W., Sedell, J. R., and Cushing, C. E. (1980). The river continuum concept. Can. J. Fish. Aquat. Sci. 37, 130–137. doi:10.1139/f80-017
Wadeson, R. A., and Rowntree, K. M. (1998). Application of the hydraulic biotope concept to the classification of instream habitats. Aquat. Ecosyst. Health Manag. 1, 143–157. doi:10.1080/14634989808656911
Wang, Y. I., Naumann, U., Dirk, E., Wilshire, J., and Warton, D. I. (2020). mvabund: Statistical methods for analysing multivariate abundance data. R package version 4.1.3 Available at: https://CRAN.R-project.org/package=mvabund (Accessed 10 02, 2022).
Wang, Y. I., Naumann, U., Wright, S. T., and Warton, D. I. (2012). mvabund–an R package for model‐based analysis of multivariate abundance data. Methods Ecol. Evol. 3, 471–474. doi:10.1111/j.2041-210x.2012.00190.x
Warton, D. I., Wright, T. W., and Wang, Y. (2012). Distance-based multivariate analyses confound location and dispersion effects. Methods Ecol. Evol. 3, 89–101. doi:10.1111/j.2041-210x.2011.00127.x
Weyl, O. L. F., Ellender, B. R., Wasserman, R. J., and Woodford, D. J. (2015). Unintended consequences of using alien fish for human benefit in protected areas. Koedoe 57, 1–5. doi:10.4102/koedoe.v57i1.1264
Wiberg-Larsen, P., Brodersen, K. P., Birkholm, S., Grøn, P. N., and Skriver, J. (2000). Species richness and assemblage structure of Trichoptera in Danish streams. Freshw. Biol. 43, 633–647. doi:10.1046/j.1365-2427.2000.00546.x
Keywords: aquatic macroinvertebrates, cyprinidae, salmonidae, river continuum hypothesis, functional feeding groups, freshwater conservation, biological monitoring
Citation: Bellingan TA, Hugo S, Villet MH and Weyl OLF (2022) Season and environment modulate aquatic invertebrates’ responses to trout and indigenous fishes in three South African mountain streams. Front. Environ. Sci. 10:1004939. doi: 10.3389/fenvs.2022.1004939
Received: 27 July 2022; Accepted: 28 September 2022;
Published: 31 October 2022.
Edited by:
Eugenia López-López, Instituto Politécnico Nacional de México, MexicoReviewed by:
Mauricio Cetra, Federal University of São Carlos, BrazilFernando Mayer Pelicice, Federal University of Tocantins, Brazil
Copyright © 2022 Bellingan, Hugo, Villet and Weyl. This is an open-access article distributed under the terms of the Creative Commons Attribution License (CC BY). The use, distribution or reproduction in other forums is permitted, provided the original author(s) and the copyright owner(s) are credited and that the original publication in this journal is cited, in accordance with accepted academic practice. No use, distribution or reproduction is permitted which does not comply with these terms.
*Correspondence: Terence A. Bellingan, dC5iZWxsaW5nYW5AcnUuYWMuemE=
†Deceased