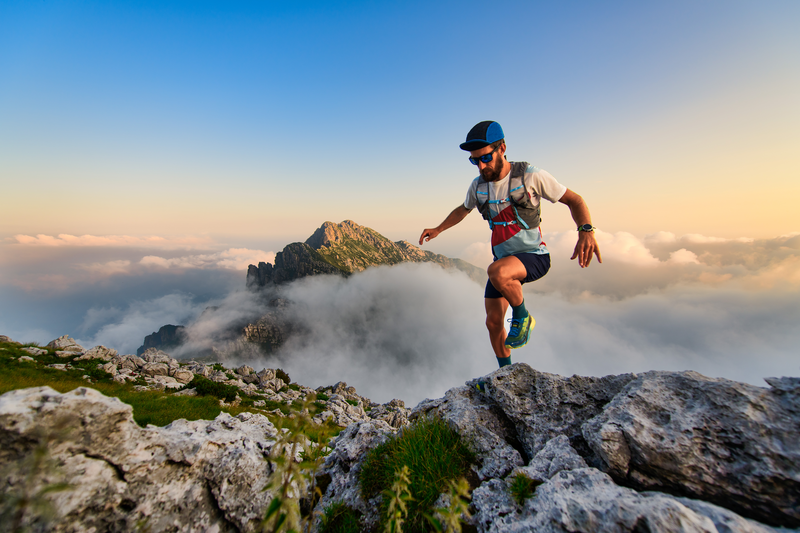
94% of researchers rate our articles as excellent or good
Learn more about the work of our research integrity team to safeguard the quality of each article we publish.
Find out more
ORIGINAL RESEARCH article
Front. Environ. Sci. , 23 November 2022
Sec. Toxicology, Pollution and the Environment
Volume 10 - 2022 | https://doi.org/10.3389/fenvs.2022.1001504
Water pollution and control are important issues of our lasting concern. Environmental media often contains a variety of compounds. Tritium is widely present in nature due to human activities. As an endocrine disruptor, genistein is widely found in water body. Will it cause damage when combined with tritiated water and genistein? In this study, Zebrafish embryos were randomly divided into 4 groups: blank control group (simple E3 medium), tritiated water exposure group (tritiated water with a final concentration of 3.7*102Bq/mL in the medium), and genistein exposure group (the final concentration of 1.4 mg/L genistein in the medium) and the combined exposure group of tritiated water and genistein (3.7*102 Bq/mL tritium water +1.4 mg/L genistein). The results show that tritiated water with 3.7*102 Bq/ml exposure alone did not affect the development of zebrafish embryos. However, the survival rate, hatching rate and heart rate of zebrafish larvae decreased combined exposure with genistein, and the abnormality rate and apoptotic cells in the embryos and the level of oxidative stress increased. The results of RNA sequencing showed that the combined exposure of tritiated water and genistein affected the gene expression of zebrafish embryos. Differential genes were mainly enriched in many pathways, such as p53 signaling pathway, steroid hormone biosynthesis, PPAR signaling pathway, metabolism of xenobiotics by cytochrome P450. The results of qRT-PCR and gene knockout experiment showed that cyp19a1b gene may plays an important role in the toxic effects of combined exposure.
Tritium is a natural radionuclide, and its main natural source is the interaction of cosmic rays and gas in the upper atmosphere (Canadian nuclear safety commission, 2009). Since the second half of the 20th century, tritium has been mainly produced by human activities, especially during the period from 1945 to 1980, when atmospheric nuclear tests caused about 1.7*1020 Bq of tritium to be emitted into the atmosphere (Schell and Sauzay, 1973). The development of nuclear industry has also led to an increase in the amount of tritium released into the environment. For example, in 2020, the British nuclear industry discharged about 1.4*1015 Bq of tritium into the environment (Scottish environment protection agency, 2020). The average activity detected by French nuclear power plants during normal operation is 2*106 Bq/L, although the tritium activity detected downstream is only 1–65 Bq/L, due to the dilution of seawater after discharge (Autorité de sûreté nucléaire, 2013). In addition, nuclear accident will also cause a large amount of tritium emissions. For example, the accident treatment of the Fukushima nuclear power plant will emit 22*1012 Bq of tritium into the environment every year (Ministry of economy ,trade and industry, 2021). In the future, new nuclear facilities such as the International Thermonuclear Reactor (ITER) will increase the emissions of tritium into the environment (Arcanjo et al., 2020). Tritium discharged into the environment mainly exists in the form of HTO, and is enriched in organisms through the food chain (Jha et al., 2005; Jaeschke and Bradshaw, 2013; Kim et al., 2015). After tritium decays, it releases low-energy (average value of 5.7 keV) less penetrating (range of 0.56 um in water) beta particles. Because of these characteristics, the risk of external exposure caused by tritium is negligible. But once it enters living body, it will deposit a considerable amount of energy in cells or tissues, causing internal radiation damage (HPA, 2007; Guéguen et al., 2018; Higley et al., 2021). The biological effects of HTO have been studied in many species, such as causing DNA damage in marine invertebrates and vertebrate cells (Hagger et al., 2005; Jha et al., 2005), on the development of the nervous system in mice and rats (Gao et al., 2002; Wang et al., 2010), on immune effect in human cells s (Gonen et al., 2014; Quan et al., 2019).
As is known to all, natural environmental media usually contain multiple compounds (Sun et al., 2020), for example permethrin (Chen et al., 2016), imidacloprid (Ge et al., 2015), abamectin (Sanches et al., 2017). Thus considering only the biological effects induced by one substance may underestimate the environmental toxicity of environmental exposures (Ribeiro et al., 2012; Shen et al., 2020). There is an urgent need to carry out related research on how the joint exposure of tritium and other environmental pollutants affects sensitive organisms.
Due to human activities, many natural plant compounds with phytoestrogen properties, such as genistein, exist in agricultural, household and industrial wastewater and may be discharged into the environment (Sarasquete et al., 2017). Genistein was found to have a concentration of 10 ug/L in wastewater from a pulp mill in Ontario, Canada (Kiparissis et al., 2001). Spengler et al. (Spengler et al., 2001) found that genistein was present in the effluent of 60% of wastewater treatment plants in Germany, with a concentration of 3–38 ng/L. On the other hand, soybean meal is often used to partially replace fish meal due to its high protein content, good amino acid composition, good palatability to fish, easy availability, and low price. But in fact, most of the by-products of legumes contain different concentrations of estrogen (Patisaul and Jefferson, 2010; Wang et al., 2013). Genistein (4,5,7-trihydroxy-isoflavone) is one of the isoflavones in flavonoids. It is widely found in soy foods and can bind to estrogen receptors (Sassi-Messai et al., 2009; Ganai and Farooqi, 2015). But unlike typical estrogen agonists, it will stimulate or inhibit estrogen according to the co-activator and co-inhibitor proteins in the cell (Ganai and Farooqi, 2015; Sarasquete et al., 2018a). In vitro cell experiments have shown that high concentrations of genistein can cause a variety of cell apoptosis and death (Das et al., 2006; Touny and Banerjee, 2006), At the same time, studies have found that genistein can also inhibit the activity of a variety of cellular enzymes, including prostaglandin H synthase, protein tyrosine kinase and protein kinase (Thors et al., 2007; Ganai and Farooqi, 2015). In addition, in vivo studies have shown that high concentrations of genistein can cause developmental and growth toxicity, genetic toxicity and mouse brain cell apoptosis (Choi and Lee, 2004; National Toxicology Program, 2008).
It can be seen that, as two common environmental exposures, tritium and genistein have different toxicity to organisms. So far, no relevant research found on the impact of both together on sensitive organisms when they are exposed in the environment. To this end, we selected the classic water model organism zebrafish, explored the effect of combined exposure of tritiated water and genistein on the early growth and development of zebrafish, and searched for the key target genes of the combined exposure of tritiated water and genistein through transcriptome sequencing, to clarify the toxic effects of their joint exposure to zebrafish and its specific molecular mechanism.
Genistein (purity>99%) was purchased from Hebei Bailingwei Superfine Co. Ltd. (Hebei, China). Tritiated water (97% purity, 185 MBq) was purchased from PerkinElmer. E3 solution (5 mM NaCl, 0.17 mM KCl, 0.33 mM CaCl2, 0.33 mM MgSO4, 0.01% methylene blue) diluted tritiated water to a concentration of 3.7*105 Bq/ml stock solution for use. Wild-type zebrafish (AB) and transgenic zebrafish (cmlc-gfp) were purchased at China Zebrafish Resource Center and kept in the laboratory. HiScript III All-in-one RT SuperMix Perfect for qPCR and ChamQ Universal SYBR qPCR Master Mix were purchased from Vazyme (Nanjing, China), and TRIzol reagent was purchased from Life Technologies (United States).
Adult male and female wild-type zebrafish (AB) and transgenic zebrafish (cmlc-gfp) which heart gene cmlc is labeled with the fluorescent protein gene GFP were reared separately in the circulatory system, water temperature was maintained at 28 ± 1°C, and the light-dark cycle was 14:10. The transgenic zebrafish (cmlc-gfp) was used for the observation of cardiac malformations. Zebrafish were fed three times a day, with hatched brine shrimp in the morning and evening, and fodder at noon. Healthy female and male fish were put in a spawning tank separated by a baffle for the first night according to the ratio of 1:1 or 1:2. The next morning, the plastic baffle was pulled out and let the fish mate freely induced with light. The eggs were collected within 30 min and incubated with E3 solution at 28°C for 2 h.
The eggs at 2 h post fertilization (2 hpf) were randomly selected into petri dishes, and 50 eggs in each dish were exposed to concentrations of 1 mg/L, 1.25 mg/L, 1.5 mg/L, 1.75 mg/L, and 2 mg/L of genistein solution respectively, (the concentration of 2 g/L genistein stock solution was diluted with E3 culture solution), the mortality was recorded up to 120 hpf, and then SPSS25 software was used to perform probabilistic regression analysis to determine the sub-lethal concentration of genistein. Zebrafish eggs were randomly divided into 4 groups, and they were exposed to E3 solution, 3.7*102 Bq/ml tritiated water, 1.4 mg/L genistein, tritiated water and genistein solution respectively. All exposure groups were set with three replicate groups, and the experiment was repeated three times.
In order to observe the toxicity of combined exposure of genistein and tritiated water to zebrafish, the mortality, hatching rate and deformity rate of zebrafish were recorded under the MoticSMA-168 stereo microscope every 24 h until 120hpf. During the exposure process, all dead embryos and larvae were taken out in time, and the criteria for judging death were as described in the article (OECD 2013) (Organisation for Economic Co-operation and Development OECD, 2013). At the same time, the spontaneous movement of the embryo’s tail within 1 min at 24 hpf, the heart rate of 48 hpf, 72 hpf, and 96 hpf zebrafish larvae for 20 s, and the body length of 96hpf zebrafish larvae were measured. The eggs of transgenic zebrafish (cmlc-gfp) which heart structure can been seen during the development were used to observe the morphological changes of the heart during poisoning process.
The apoptosis of zebrafish larvae during development was determined by acridine orange (AO) staining. Zebrafish larvae exposed for 96 h were taken out from each petri dish, 10 in each group. After being washed twice with E3 medium, they were stained with AO solution at a concentration of 5 mg/L in a dark environment for 1 h, and then washed with E3 medium three times to remove excess AO reagent. After being anesthetized with tricaine at a concentration of 0.0016 M, they were observed and photographed under a fluorescence microscope.
DCFH-DA was used to evaluate the production of reactive oxygen species (ROS) in zebrafish larvae by measuring the converted fluorescent DCF (2,7, dichlorofluorescein). In a dark environment, the 96hpf zebrafish larvae of each group were exposed to DCFH-DA solution at a concentration of 20ug/ml for 1 h, then washed twice with E3 medium to remove excess DCFH-DA, and observed under a fluorescence microscope after being anesthetized. The image was processed with ImageJ software.
After 96 h of exposure, 40 zebrafish larvae were randomly selected from each petri dish and placed in a 1.5 ml EP tube, and kept at −80°C after removing the culture solution. Total RNA was extracted with TRIzol reagent, NanoDrop2000 spectrophotometer was used to determine the purity and concentration of RNA, and cDNA was synthesized using HiScript III All-in-one RT SuperMix Perfect for qPCR. ChamQ Universal SYBR qPCR Master Mix was used for quantitative real-time polymerase chain reacton (qRT-PCR) and that was completed in the PRISM 7300 qPCR system. The reaction system is 20ul under the following conditions: denaturation at 95°C for 30 s, followed by 40 cycles of amplification at 95°C for 10 s and 60°C for 30 s. The primers of the target gene were designed by the NCBI website, and gapdh was the internal reference gene. The primer sequence is shown in Supplementary Table S1, and the relative quantification was carried out by the 2−ΔΔCT method.
In order to verify the role of cyp19a1b in the development process, cyp19a1b-transgenic zebrafish was constructed using CRISPR/Cas9 technology. The sgRNA target sequences 5′-GCAGGGGGAGAGTATATATT-3′, T7 started TAATA CGACTCACTATA, and 20 bases gttttagagctagaaatagc constituted forward primers, which were then amplified by PCR to obtain sgRNA. Then sgRNA (final concentration of 200 ng/ul) and Cas9 mRNA (final concentration of 400 ng/ul) were mixed and injected into wild-type zebrafish embryos at the single-cell stage. Subsequently, the injected eggs were exposed in the above-mentioned poisoned solution to observe the growth and development.
Data were presented as the mean ± SD. Statistical differences between two or more groups were determined using the Student’s t-test and one-way analysis of variance (ANOVA) followed by the Student-Newman-Keuls test. p-value of <0.05 indicated statistical significance.
In order to determine the exposure concentration of genistein, zebrafish embryo mortality was observed after exposure to different concentrations of genistein, and analyzed with SPSS software to find the sub-lethal concentration (LC50) of genistein to zebrafish. As shown in Supplementary Table S2, the LC50 of genistein is 1.4 mg/L.
Previous experiments by our group have shown that tritiated water exposure at concentrations of 3.7*102 Bq/ml and below does not affect the early growth and development of zebrafish. Therefore, we chose 3.7*102 Bq/ml tritiated water and 1.4 mg/L genistein as the combined exposure dose. The results of the survival rate, hatching rate, heart rate, spontaneous tail-coiling and deformity rate of zebrafish embryos are shown in Figure 1. In terms of survival rate, the survival rate of zebrafish in each treatment group at 0–96 hpf was not significantly different from that of the control group, but by 120 hpf, the survival rate of the genistein-exposed group and the combined exposure group showed a significant decrease (p < 0.01) (Figure 1A). The hatching rate of 120 hpf is shown in Figure 1B. Compared with the control group, the hatching rate of the genistein-exposed group and the combined exposure group both decreased significantly (p < 0.01). The length of the combined exposure group was significantly lower than that of the control group, while the length of the other groups did not change significantly (p < 0.0001) (Figure 1C). The results of spontaneous tail movement are shown in Figure 1D. Compared with the control group, the combined exposure group showed a significant increase (p < 0.05) (Figure 1D). The morphological observation results of zebrafish embryos showed that the rate of embryo malformation in the combined exposure group (p < 0.001) and the genistein alone exposure group (p < 0.01) increased. The results are shown in Figure 2. The main manifestations were yolk sac edema, spinal curvature, and pericardial edema.
FIGURE 1. The growth and development of zebrafish during the exposure. (A) survival rate of zebrafish in each group at 120 hpf; (B) hatching rate of zebrafish embryos at 96 hpf; (C) the length of zebrafish larvae at 96 hpf; (D) spontaneous movement of the zebrafish embryo tail at 24 hpf. 10 samples were taken from each parallel samples of each experimental group. *p < 0.05, **p < 0.01, ****p < 0.0001.
FIGURE 2. The morphology of zebrafish larvae. (A) control group; (B) 3.7*102 Bq/ml HTO group; (C) 1.4 mg/L genistein group; (D) 3.7*102 Bq/ml HTO+1.4 mg/L genistein group; (E) the malformation rate of 96 hpf zebrafish larvae. 10 samples were taken from each parallel samples of each experimental group. SC: spinal curvature; YSE: yolk sac edema; PE: pericardial edema. **p < 0.01, ***p < 0.001.
Heart morphology and heart rate of 96 hpf zebrafish embryos were also observed, and the results are shown in Figure 3. Compared with the control group, the heart rate of the combined exposure group showed a significant decrease (p < 0.0001), while there was no significant change in the heart rate of the genistein and tritium exposure group. Heart morphology of transgenic zebrafish (cmlc-gfp) in combined exposure group and zebrafish in genistein group changed, mainly manifested as the enlarged distance between the atrium and the ventricle and the changed shape of heart from the original spherical to elongated, as shown in Figure 3A–D.
FIGURE 3. The morphology of the heart of zebrafish larvae. (A) The morphology of the heart in every group; (B) the heart rate of zebrafish at 96 hpf; (C) The distance between the atria and ventricles of 96 hpf zebrafish larvae. 10 samples were taken from each parallel samples of each experimental group. ****p < 0.0001.
These results showed that 3.7*102 Bq/ml tritiated water alone will not affect the development of zebrafish embryos, while the toxicity to zebrafish embryos was greatly increased after the addition of genistein, and both have a synergistic effect.
The results of ROS production of zebrafish larvae at 96 hpf are shown in Figure 4. ROS production was mainly in the heart and brain regions of zebrafish larvae. Compared with the control group, the oxidative stress level of zebrafish larvae did not change significantly after tritiated water exposure, but the oxidative stress level of the genistein and combined exposure groups increased significantly (Figure 4F).
FIGURE 4. Reactive oxygen species (ROS) in 96hpf zebrafish larvae. (A) control group; (B) 3.7*102 Bq/ml HTO group; (C) 1.4 mg/L genistein group; (D) 3.7*102 Bq/ml HTO+1.4 mg/L genistein group; (E) positive control group; (F) the quantity analysis of fluorescence intensity. 10 samples were taken from each parallel samples of each experimental group. *p < 0.05, **p < 0.01, ***p < 0.001.
Figure 5 shows the apoptosis results of zebrafish larvae. The apoptosis induced by genistein and tritiated water was mainly concentrated in the heart and yolk sac, as shown in Figure 5A–D. Analyzing the fluorescence intensity with ImageJ software showed that compared with the control group, the fluorescence intensity of the genistein group and the combined exposure group was significantly increased, while the tritiated water alone exposure group did not change, indicating that both genistein and combined exposure induced the increase of cell apoptosis in zebrafish embryos.
FIGURE 5. Apoptosis in 96 hpf zebrafish larvae. (A) control group; (B) 3.7*102 Bq/ml HTO group; (C) 1.4 mg/L genistein group; (D) 3.7*102 Bq/ml HTO+1.4 mg/L genistein group; (E) Fluorescence intensity analysis. 10 samples were taken from each parallel samples of each experimental group. *p < 0.05, **p < 0.01.
96 hpf larvae from the tritiated water and genistein combined exposure group and the control group were collected for transcriptome sequencing. Sequencing results showed that compared with the control group, there were 1,061 differentially expressed genes in the combined exposure group, of which 745 genes were up-regulated and 316 were down-regulated. The volcano map and cluster analysis map of the differential genes are shown in Figures 6A,B.
FIGURE 6. Analysis of transcriptome sequencing results. (A) Differential gene compare control with 3.7*102 Bq/ml HTO+1.4 mg/L genistein at 96hpf, (B) Volcano map, (C) Cluster map, (D) GO enrichment analysis, (E) KEGG enrichment analysis.
Next, the differential genes were annotated with GO enrichment analyses. The results showed that the biological processes that the differential genes of 96 hpf mainly enriched included visual perception, lipid transport, neuron projection regeneration, etc.; molecular functions mainly included lipid transport, steroid hydroxyl activity, peptidase activity, etc.; cell components were mainly related to the extracellular space and extracellular matrix (Figure 6D).
Analysis of the differential gene KEGG showed that there were a total of 146 genes enriched to the top 20 signaling pathways of KEGG, the main pathways were “Phototransduction”, “ECM-receptor interaction”, “p53 signaling pathway”, “Steroid hormone biosynthesis”, “PPAR signaling pathway”, “Metabolism of xenobiotics by cytochrome P450″, etc. (Figure 6E).
Among the differential genes screened above, several genes as cyp19a1b, cyp3a65, f13a1a.1, fam20cl, ghrh, prss59.1, vtg1 with obvious changes were selected for RT-qPCR verification. The results are shown in Supplementary Figure S1. cyp19a1b was significantly up-regulated by tritiated water and genistein poison, and f13a1a.1, fam20cl, and ghrh were also up-regulated (p < 0.05). At the same time, the main genes p53, bax, caspase-8 involved in cell apoptosis, were tested, and the results showed that the p53, bax, and caspase-8 genes in the combined exposure group were up-regulated.
Zebrafish embryo cyp19a1b was significantly up-regulated after combined exposure to tritiated water and genistein, which could play an important regulatory role in the toxic effects of combined exposure. To this end, a cyp19a1b gene knockout zebrafish model was constructed to study the regulatory effects of cyp19a1b by using the deformity rate and heart rate that were significantly changed after joint exposure as observation indicators. The results in Figures 7A,B showed that after cyp19a1b gene knockout, compared with zebrafish embryos without gene interference, the abnormalities of the combined-exposure group and genistein-exposed zebrafish larvae were alleviated, and the abnormality rate was significantly reduced. After the cyp19a1b gene was knocked out, compared with zebrafish embryos without gene interference, the heart rate of the combined exposure group and the genistein group showed a certain degree of recovery, as shown in Figure 7C.
FIGURE 7. The development of zebrafish during the exposure after cyp19a1b gene knockout. (A) The morphology of zebrafish larvae, (B) the malformation rate of 96 hpf zebrafish larvae, (C) the heart rate of zebrafish at 96 hpf, (D) spontaneous movement of the zebrafish embryo tail at 24 hpf. 10 samples were taken from each parallel samples of each experimental group. “-” mean cyp19a1b is knockdown. **p < 0.01, ***p < 0.001, ****p < 0.0001.
Aquatic systems are inhabited by a large variety of species, and are also the final receptors of most pollutants. Therefore, it is necessary and urgent to evaluate the ecological risks of environmental exposures (Adam-Guillermin et al., 2012). This study showed that LC50 of genistein was 1.4 mg/L, which was consistent with the results of Sarasquete (Sarasquete et al., 2018b) and others. It can be determined that a certain concentration of genistein will affect the growth and development of zebrafish, mainly including survival rate, decline of hatching rate and increase of body deformity rate. Tritiated water of 3.7*102 Bq/ml will not cause changes in survival rate, deformity rate and hatching rate of zebrafish. Strand (Strand et al., 1977) et al. exposed rainbow trout to 3.7*102 Bq/ml tritiated water for 20 days, and found no increase in mortality. However Hagger et al. (Hagger et al., 2005) observed that the mortality and deformity rate of mytilus edulis embryos in tritiated water with a concentration of 0.37 kBq/ml increased, and increased with the increase of tritiated water concentration. The difference in these results may be due to the different sensitivity of different species to radiation. After the combined exposure of tritiated water and genistein, the survival rate of zebrafish embryos decreased obviously, and the rate of abnormalities increased significantly. The change of their heart rate showed that when tritiated water and genistein were exposed seperately, there was no obvious effect on the heart rate, but when both were exposed together, the heart rate drops significantly. These results all indicated that the combined exposure of both presented a synergistic effect on the growth and development of zebrafish and increased toxicity. In this study, the hatching rate of zebrafish embryos was significantly reduced after combined exposure, and the delay or inhibition of hatching would hinder the absorption of nutrients and eventually lead to the death of embryos in the chorion (Lee et al., 2019), which may be one of the reason for the reduced survival rate of embryos in the co-treatment group.
The hatching process is achieved by a combination of biochemical and behavioral phenomena, starting with enzymes synthesized by hatching glands to digest the chorion, followed by tearing of the chorion by twisting the embryo (de Gaspar et al., 1999). Disturbing any point will hinder the hatching process, resulting in reduced hatchability (Westernhagen, 1988). In the observation of the abnormality of embryonic development in the genistein group and the combined exposure group, there was obvious yolk sac edema in both groups, and the abnormality of the yolk sac was an important cause of the hypoplasia of the hatching gland (Tian et al., 2019). There will be a reduction in the enzymes synthesized by the hatching glands, thus hindering the hatching process.
The results of transcriptomics screened many differential genes, such as P53 signaling pathway genes, including casp3b, casp8, and tp53, etc., in which the activation of tp53 protein can cause hindered DNA replication or cell apoptosis (Henson and Taylor, 2020). The results of qRT-PCR verification proved that bax and caspase-8 in the combined exposure group were up-regulated. Apoptosis is a process of programmed cell death induced during embryonic and immune development and has clear morphological characteristics (Obeng, 2021). Genistein can cause early apoptosis of zebrafish embryos, and early cell apoptosis can induce embryonic death (Sassi-Messai et al., 2009), which may explain the embryonic mortality is significantly increased at 96hpf. The results of AO staining proved that the number of apoptotic cells in the combined exposure group increased markedly, and they were mainly concentrated in the back of the brain and the heart.
The destruction of basic components of cells by reactive oxygen species (ROS) is generally considered to be a serious mechanism for the pathogenesis of many diseases (Banaee, 2013). When present at lower levels, ROS plays a key role in cellular homeostasis, however excess ROS can lead to cellular dysfunction, protein and lipid peroxidation, and DNA damage, ultimately leading to irreversible cell death. Many studies have confirmed that some poisons can cause cell damage by inducing cells to produce excessive ROS (Banaee, 2013). In this study, it was found that ROS levels in zebrafish larvae increased with exposure to tritiated water and genistein. The increase of reactive oxygen species may disrupt the originally balanced oxidation and antioxidant systems in the zebrafish body, leading to oxidative stress (Sepici-Dinçel et al., 2009). The up-regulation of a large number of genes in the two biological processes of redox and lipid transport in GO analysis also indicates that the antioxidant system in zebrafish has been disorder. It has been reported that exposure to tritiated water will also increase the level of ROS in zebrafish larvae (Gagnaire et al., 2020). We speculate that in terms of oxidative stress, the combined effects of tritiated water and genistein on zebrafish embryos may be superimposed, which leads to excessively high active oxygen of zebrafish larvae, and the original balanced redox system in the body is disrupted, thus affecting the normal function of cells.
Heart is the first functional organ for zebrafish development, and heart rate is an important indicator in fish embryo toxicity experiments (Glickman and Yelon, 2002). The heart rate suppression of zebrafish embryos is often observed in other toxicity experiments (Yang et al., 2016; Sun and Liu, 2017). Previous studies have found that ROS has the function of promoting fibrosis, leading to extracellular matrix remodeling by inducing cardiac fibroblasts and matrix proteases, and also directly impairing the contraction of cardiomyocytes by modifying excitation-contraction coupled central proteins. The heart rate of zebrafish was significantly decreased after co-exposure to tritiated water and genistein, and the abnormal pericardial and cardiac structures observed in transgenic zebrafish (cmlc-gfp) induced by co-exposure to tritiated water and genistein may be caused by excessive ROS production. It can be seen that the combined exposure of tritiated water and genistein injures the structure and function of the zebrafish embryo’s heart, and the heart is the main target organ of combined poisoning.
Spontaneous movements and activities have been shown to begin before responses to sensory stimuli (Drapeau et al., 2002), and the first spontaneous movements can be observed in zebrafish embryos at 17 hpf, before they can receive sensory stimuli. Some studies suggest that behavioral maturation may be based on spontaneous neural activity during development and can be used as an important toxicological endpoint for evaluating the toxicity of chemicals to fish (Wang et al., 2019; Fraysse et al., 2006). Fraysse (Fraysse et al., 2006) and others found that 108 mM propranolol could reduce the spontaneous movement of 24 hpf zebrafish embryos by reducing the activity of Na ion channels. Wang (Wang et al., 2019) and others found that Clethodim at a concentration of more than 10 mg/L could reduce the spontaneous movement of the zebrafish tail by reducing the activity of acetylcholinease. After the combined exposure of tritiated water and genistein, the spontaneous movement of the zebrafish embryo’s tail at 24 hpf increased, which could mean that the synapse was affected during the development process, because excessive neuromuscular activity would disrupt the development of the synapse (Lefebvre et al., 2004).
Aromatase is an enzyme complex that exists in all spinal animals and controls the dynamic equilibrium of estrogen, which plays an important role in many biological functions including reproduction, behavior and memory, bone density and vascular homeostasis (Blakemore and Naftolin, 2016). Unlike most mammals, there are two different aromatase genes in bony fish, one is cyp19a1b, which is expressed in brain; the other is cyp19a1a, which is expressed in gonads (Bondesson et al., 2015). cyp19a1b gene was obviously up-regulated after the combined exposure of tritiated water and genistein, which may be related to zebrafish growth and nervous system injury. Studies have shown that aromatase could promote the repair of brain damage (Peterson et al., 2004). CRISPR/Cas9 technology interfered with the cyp19a1b gene of wild-type zebrafish embryos, which reduced the malformation rate of zebrafish larvae after joint exposure, while the heart rate increased accordingly, indicating that the toxic effect of joint poisoning in zebrafish after inhibiting cyp19a1b appeared to relieve. It shows that the combined exposure of tritiated water and genistein induces the up-regulation of cyp19a1b gene, which interferes with the growth and development of zebrafish embryos, causing abnormalities in multiple indicators such as morphology, heart rate and tail movement.
In short, tritiated water has a toxic and sensitizing effect on genistein, and the combined exposure of both induces obvious abnormal growth and development of zebrafish. cyp19a1b gene plays an important role in the toxic effects of combined exposure to tritiated water and genistein, and inhibiting its expression can reduce the toxic effects of combined exposure (Supplementary Figure S2). These research results confirm the ecological risks of the combined exposure of environmental pollutants such as tritiated water, and provide a new theoretical basis for environmental pollution control.
The raw data supporting the conclusion of this article will be made available by the authors, without undue reservation.
The animal study was reviewed and approved by the Animal Care/User Ethics Committee of Soochow University, Suzhou City, P. R. China.
Conception and design: FC, QZ, JW and YT; development of methodology and acquisition of data: LS, NC, HX, and TW; analysis and interpretation of data: FL, QC; writing and revision of the manuscript: FC, QZ, and YT.
This work was supported by the National Natural Science Foundation of China (grant numbers U186720) and the Priority Academic Program Development of Jiangsu Higher Education Institutions (PAPD).
The authors declare that the research was conducted in the absence of any commercial or financial relationships that could be construed as a potential conflict of interest.
All claims expressed in this article are solely those of the authors and do not necessarily represent those of their affiliated organizations, or those of the publisher, the editors and the reviewers. Any product that may be evaluated in this article, or claim that may be made by its manufacturer, is not guaranteed or endorsed by the publisher.
The Supplementary Material for this article can be found online at: https://www.frontiersin.org/articles/10.3389/fenvs.2022.1001504/full#supplementary-material.
Adam-Guillermin, C., Pereira, S., Della-Vedova, C., Hinton, T., and Garnier-Laplace, J. (2012). Genotoxic and reprotoxic effects of tritium and external gamma irradiation on aquatic animals. Rev. Environ. Contam. Toxicol. 220, 67–103. doi:10.1007/978-1-4614-3414-6_3
Arcanjo, C., Adam-Guillermin, C., Murat El Houdigui, S., Loro, G., Della-Vedova, C., Cavalie, I., et al. (2020). Effects of tritiated water on locomotion of zebrafish larvae: A new insight in tritium toxic effects on a vertebrate model species. Aquat. Toxicol. 219, 105384. doi:10.1016/j.aquatox.2019.105384
Banaee, M. (2013). Physiological Dysfunction in Fish after Insecticides Exposure, Insecticides - Development of Safer and More Effective Technologies. Editor Stanislav Trdan (IntechOpen). doi:10.5772/54742
Blakemore, J., and Naftolin, F. (2016). Aromatase: Contributions to physiology and disease in women and men. Physiol. (Bethesda) 31, 258–269. doi:10.1152/physiol.00054.2015
Bondesson, M., Hao, R., Lin, C.-Y., Williams, C., and Gustafsson, J.-Å. (2015). Estrogen receptor signaling during vertebrate development. Biochimica Biophysica Acta - Gene Regul. Mech. 1849, 142–151. doi:10.1016/j.bbagrm.2014.06.005
Chen, X., Li, H., Zhang, J., Ding, Y., and You, J. (2016). Does cadmium affect the toxicokinetics of permethrin in Chironomus dilutus at sublethal level? Evidence of enzymatic activity and gene expression. Environ. Pollut. 218, 1005–1013. doi:10.1016/j.envpol.2016.08.051
Choi, E. J., and Lee, B. H. (2004). Evidence for genistein mediated cytotoxicity and apoptosis in rat brain. Life Sci. 75, 499–509. doi:10.1016/j.lfs.2004.01.010
Canadian nuclear safety commission (2009). Investigation of the environmental fate of tritium in the atmosphere Canada.
Das, A., Banik, N. L., and Ray, S. K. (2006). Mechanism of apoptosis with the involvement of calpain and caspase cascades in human malignant neuroblastoma SH-SY5Y cells exposed to flavonoids. Int. J. Cancer 119, 2575–2585. doi:10.1002/ijc.22228
de Gaspar, I., Blanquez, M. J., Fraile, B., Paniagua, R., and Arenas, M. I. (1999). The hatching gland cells of trout embryos: Characterisation of N- and O-linked oligosaccharides. J. Anat. 194 (1), 109–118. doi:10.1046/j.1469-7580.1999.19410109.x
Drapeau, P., Saint-Amant, L., Buss, R. R., Chong, M., McDearmid, J. R., and Brustein, E. (2002). Development of the locomotor network in zebrafish. Prog. Neurobiol. 68, 85–111. doi:10.1016/S0301-0082(02)00075-8
Fraysse, B., Mons, R., and Garric, J. (2006). Development of a zebrafish 4-day embryo-larval bioassay to assess toxicity of chemicals. Ecotoxicol. Environ. Saf. 63, 253–267. doi:10.1016/j.ecoenv.2004.10.015
Gagnaire, B., Arcanjo, C., Cavalié, I., Camilleri, V., Simon, O., Floriani, M., et al. (2020). Tritiated water exposure in zebrafish (Danio rerio): Effects on the early-life stages. Environ. Toxicol. Chem. 39, 648–658. doi:10.1002/etc.4650
Ganai, A. A., and Farooqi, H. (2015). Bioactivity of genistein: A review of in vitro and in vivo studies. Biomed. Pharmacother. 76, 30–38. doi:10.1016/j.biopha.2015.10.026
Gao, W.-M., Lu, H.-M., Dong, J.-C., Zhang, W., Zhou, X.-Y., Jenkins, L. W., et al. (2002). Postnatal growth, neurobehavioral and neurophysiologic changes of prenatal low-dose β-radiation from tritiated water in mice. Neurotoxicol. Teratol. 24, 247–254. doi:10.1016/S0892-0362(02)00202-7
Ge, W., Yan, S., Wang, J., Zhu, L., Chen, A., and Wang, J. (2015). Oxidative stress and DNA damage induced by imidacloprid in zebrafish (Danio rerio). J. Agric. Food Chem. 63, 1856–1862. doi:10.1021/jf504895h
Glickman, N. S., and Yelon, D. (2002). Cardiac development in zebrafish: Coordination of form and function. Semin. Cell Dev. Biol. 13, 507–513. doi:10.1016/s1084952102001040
Gonen, R., German, U., Alfassi, Z. B., and Priel, E. (2014). Production of DNA double strand breaks in human cells due to acute exposure to tritiated water (HTO), Herzliya , Israel. NSIIsrael, 367.
Guéguen, Y., Priest, N. D., Dublineau, I., Bannister, L., Benderitter, M., Durand, C., et al. (2018). In vivo animal studies help achieve international consensus on standards and guidelines for health risk estimates for chronic exposure to low levels of tritium in drinking water. Environ. Mol. Mutagen. 59, 586–594. doi:10.1002/em.22200
Hagger, J. A., Atienzar, F. A., and Jha, A. N. (2005). Genotoxic, cytotoxic, developmental and survival effects of tritiated water in the early life stages of the marine mollusc, Mytilus edulis. Aquat. Toxicol. 74, 205–217. doi:10.1016/j.aquatox.2005.05.013
Henson, H. E., and Taylor, M. R. (2020). A sart1 zebrafish mutant results in developmental defects in the central nervous system. Cells 9, 2340. doi:10.3390/cells9112340
Higley, K., Real, A., and Chambers, D. (2021). ICRP publication 148: Radiation weighting for reference animals and plants. Ann. ICRP 50, 9–133. doi:10.1177/0146645319896548
Hpa, (2007). Review of risks from tritium: Report of the independent advisory group on ionising radiation/[prepared by the subgroup on tritium internal dosimetry of the advisory group on ionising radiation]. Chilton: Health Protection Agency.
Jaeschke, B. C., and Bradshaw, C. (2013). Bioaccumulation of tritiated water in phytoplankton and trophic transfer of organically bound tritium to the blue mussel, Mytilus edulis. J. Environ. Radioact. 115, 28–33. doi:10.1016/j.jenvrad.2012.07.008
Jha, A. N., Dogra, Y., Turner, A., and Millward, G. E. (2005). Impact of low doses of tritium on the marine mussel, Mytilus edulis: Genotoxic effects and tissue-specific bioconcentration. Mutat. Research/Genetic Toxicol. Environ. Mutagen. 586, 47–57. doi:10.1016/j.mrgentox.2005.05.008
Kim, S. B., Shultz, C., Stuart, M., and Festarini, A. (2015). Tritium uptake in rainbow trout (Oncorhynchus mykiss): HTO and OBT-spiked feed exposures simultaneously. Appl. Radiat. Isot. 98, 96–102. doi:10.1016/j.apradiso.2015.01.020
Kiparissis, Y., Hughes, R., Metcalfe, C., and Ternes, T. (2001). Identification of the isoflavonoid genistein in bleached kraft mill effluent. Environ. Sci. Technol. 35, 2423–2427. doi:10.1021/es001679+
Lee, W. S., Cho, H.-J., Kim, E., Huh, Y. H., Kim, H.-J., Kim, B., et al. (2019). Bioaccumulation of polystyrene nanoplastics and their effect on the toxicity of Au ions in zebrafish embryos. Nanoscale 11, 3173–3185. doi:10.1039/c8nr09321k
Lefebvre, J. L., Ono, F., Puglielli, C., Seidner, G., Franzini-Armstrong, C., Brehm, P., et al. (2004). Increased neuromuscular activity causes axonal defects and muscular degeneration. Development 131, 2605–2618. doi:10.1242/dev.01123
Ministry of economy ,trade and industry (2021). Basic policy on handling of ALPS treated water at the tokyo electric power company holdings’ Fukushima daiichi nuclear power station.
National Toxicology Program (2008). Multigenerational reproductive study of genistein (Cas No. 446-72-0) in Sprague-Dawley rats (feed study). Natl. Toxicol. Program Tech. Rep. Ser. 539, 1–266.
Organisation for Economic Co-operation and Development OECD (2013). Test No. 236: Fish Embryo Acute Toxicity (FET) Test, OECD Guidelines for the Testing of Chemicals, Section 2. Paris: OECD Publishing. doi:10.1787/9789264203709-en
Obeng, E. (2021). Apoptosis (programmed cell death) and its signals - a review. Braz. J. Biol. 81, 1133–1143. doi:10.1590/1519-6984.228437
Patisaul, H. B., and Jefferson, W. (2010). The pros and cons of phytoestrogens. Front. Neuroendocrinol. 31, 400–419. doi:10.1016/j.yfrne.2010.03.003
Peterson, R. S., Lee, D. W., Fernando, G., and Schlinger, B. A. (2004). Radial glia express aromatase in the injured zebra finch brain. J. Comp. Neurol. 475, 261–269. doi:10.1002/cne.20157
Quan, Y., Zhou, C., Deng, B., and Lin, J. (2019). The low dose effects of human mammary epithelial cells induced by internal exposure to low radioactive tritiated water. Toxicol. Vitro 61, 104608. doi:10.1016/j.tiv.2019.104608
Ribeiro, C., Urbatzka, R., Castro, L. F. C., Carrola, J., Fontainhas-Fernandes, A., Monteiro, R. A. F., et al. (2012). In vitro exposure of nile tilapia (Oreochromis niloticus) testis to estrogenic endocrine disrupting chemicals: mRNA expression of genes encoding steroidogenic enzymes. Toxicol. Mech. Methods 22, 47–53. doi:10.3109/15376516.2011.593053
Sanches, A. L. M., Vieira, B. H., Reghini, M. V., Moreira, R. A., Freitas, E. C., Espíndola, E. L. G., et al. (2017). Single and mixture toxicity of abamectin and difenoconazole to adult zebrafish (Danio rerio). Chemosphere 188, 582–587. doi:10.1016/j.chemosphere.2017.09.027
Sarasquete, C., Úbeda-Manzanaro, M., and Ortiz-Delgado, J. B. (2018). Effects of the isoflavone genistein in early life stages of the Senegalese sole, Solea senegalensis: Role of the survivin and proliferation versus apoptosis pathways. BMC Vet. Res. 14, 16. doi:10.1186/s12917-018-1333-3
Sarasquete, C., Úbeda-Manzanaro, M., and Ortiz-Delgado, J. B. (2017). Effects of the soya isoflavone genistein in early life stages of the Senegalese sole, Solea senegalensis: Thyroid, estrogenic and metabolic biomarkers. Gen. Comp. Endocrinol. 250, 136–151. doi:10.1016/j.ygcen.2017.06.008
Sarasquete, C., Úbeda-Manzanaro, M., and Ortiz-Delgado, J. B. (2018). Toxicity and non-harmful effects of the soya isoflavones, genistein and daidzein, in embryos of the zebrafish, Danio rerio. Comp. Biochem. Physiology Part C Toxicol. Pharmacol. 211, 57–67. doi:10.1016/j.cbpc.2018.05.012
Sassi-Messai, S., Gibert, Y., Bernard, L., Nishio, S.-I., Ferri Lagneau, K. F., Molina, J., et al. (2009). The phytoestrogen genistein affects zebrafish development through two different pathways. PLoS One 4, e4935. doi:10.1371/journal.pone.0004935
Schell, W. R., and Sauzay, G. (1973). World distribution of environmental tritium, Environment Radioactivity Washington DC USA.
Scottish environment protection agency (2020). Radioactivity in food and the environment (RIFE) report 2020. New Delhi, India: Food Safety Briefing.
Sepici-Dinçel, A., Cağlan Karasu Benli, A., Selvi, M., Sarikaya, R., Sahin, D., Ayhan Ozkul, I., et al. (2009). Sublethal cyfluthrin toxicity to carp (Cyprinus carpio L.) fingerlings: Biochemical, hematological, histopathological alterations. Ecotoxicol. Environ. Saf. 72, 1433–1439. doi:10.1016/j.ecoenv.2009.01.008
Shen, W., Lou, B., Xu, C., Yang, G., Yu, R., Wang, X., et al. (2020). Lethal toxicity and gene expression changes in embryonic zebrafish upon exposure to individual and mixture of malathion, chlorpyrifos and lambda-cyhalothrin. Chemosphere 239, 124802. doi:10.1016/j.chemosphere.2019.124802
Spengler, P., Körner, W., and Metzger, J. W. (2001). Substances with estrogenic activity in effluents of sewage treatment plants in southwestern Germany. 1. Chemical analysis. Environ. Toxicol. Chem. 20, 2133–2141. doi:10.1002/etc.5620201001
Strand, J. A., Fujihara, M. P., Burdett, R. D., and Poston, T. M. (1977). Suppression of the primary immune response in rainbow trout, Salmo gairdneri sublethally exposed to tritiated water during embryogenesis. J. Fish. Res. Bd. Can. 34, 1293–1304. doi:10.1139/f77-191
Sun, G., and Liu, K. (2017). Developmental toxicity and cardiac effects of butyl benzyl phthalate in zebrafish embryos. Aquat. Toxicol. 192, 165–170. doi:10.1016/j.aquatox.2017.09.020
Sun, S., Jiang, T., Lin, Y., Song, J., Zheng, Y., and An, D. (2020). Characteristics of organic pollutants in source water and purification evaluations in drinking water treatment plants. Sci. Total Environ. 733, 139277. doi:10.1016/j.scitotenv.2020.139277
Thors, L., Alajakku, K., and Fowler, C. J. (2007). The 'specific' tyrosine kinase inhibitor genistein inhibits the enzymic hydrolysis of anandamide: Implications for anandamide uptake. Br. J. Pharmacol. 150, 951–960. doi:10.1038/sj.bjp.0707172
Tian, S., Teng, M., Meng, Z., Yan, S., Jia, M., Li, R., et al. (2019). Toxicity effects in zebrafish embryos (Danio rerio) induced by prothioconazole. Environ. Pollut. 255, 113269. doi:10.1016/j.envpol.2019.113269
Touny, L. H. E., and Banerjee, P. P. (2006). Identification of both Myt-1 and Wee-1 as necessary mediators of the p21-independent inactivation of the cdc-2/cyclin B1 complex and growth inhibition of TRAMP cancer cells by genistein. Prostate 66, 1542–1555. doi:10.1002/pros.20495
Wang, H., Zhou, L., Meng, Z., Su, M., Zhang, S., Huang, P., et al. (2019). Clethodim exposure induced development toxicity and behaviour alteration in early stages of zebrafish life. Environ. Pollut. 255, 113218. doi:10.1016/j.envpol.2019.113218
Wang, M., Yuan, S., Xiao, L., Chen, G., and Zhang, W. (2010). Cell proliferation of neurons in fetal brain in rat exposed in vitro to β radiation from HTO, Taiyuan: Radiation Protection 30 (5), 259–264.
Wang, Q., Ge, X., Tian, X., Zhang, Y., Zhang, J., and Zhang, P. (2013). Soy isoflavone: The multipurpose phytochemical (Review). Biomed. Rep. 1, 697–701. doi:10.3892/br.2013.129
Westernhagen, H. V. (1988). “4 sublethal effects of pollutants on fish eggs and larvae,” in The physiology of developing fish - eggs and larvae (Elsevier),11 253–346. doi:10.1016/S1546-5098(08)60201-0
Keywords: zebrafish, tritium, genistein, combined exposure, cyp19a1b
Citation: Cui F, Zhang Q, Wan J, Sun L, Chen N, Xue H, Wang T, Luo F, Chen Q and Tu Y (2022) Toxic effects of combined exposure of tritiated water and genistein on the growth and development of zebrafish and its mechanism. Front. Environ. Sci. 10:1001504. doi: 10.3389/fenvs.2022.1001504
Received: 07 August 2022; Accepted: 03 November 2022;
Published: 23 November 2022.
Edited by:
Carlito Baltazar Tabelin, University of New South Wales, AustraliaReviewed by:
Ajay Pradhan, Örebro University, SwedenCopyright © 2022 Cui, Zhang, Wan, Sun, Chen, Xue, Wang, Luo, Chen and Tu. This is an open-access article distributed under the terms of the Creative Commons Attribution License (CC BY). The use, distribution or reproduction in other forums is permitted, provided the original author(s) and the copyright owner(s) are credited and that the original publication in this journal is cited, in accordance with accepted academic practice. No use, distribution or reproduction is permitted which does not comply with these terms.
*Correspondence: Yu Tu, dHV5dUBzdWRhLmVkdS5jbg==
†These authors have contributed equally to this work
Disclaimer: All claims expressed in this article are solely those of the authors and do not necessarily represent those of their affiliated organizations, or those of the publisher, the editors and the reviewers. Any product that may be evaluated in this article or claim that may be made by its manufacturer is not guaranteed or endorsed by the publisher.
Research integrity at Frontiers
Learn more about the work of our research integrity team to safeguard the quality of each article we publish.