- Key Laboratory of Plant Resource Conservation and Germplasm Innovation in Mountainous Region (Ministry of Education), Collaborative Innovation Center for Mountain Ecology Agro-Bioengineering (CICMEAB), College of Life Sciences/Institute of Agro-Bioengineering, Guizhou University, Guiyang, China
Removal of nitrogen from hydroxylamine could effectively improve the wastewater treatment efficiency. In this work, Bacillus thuringiensis EM-A1 was obtained from a biogas digester with hydroxylamine as the only nitrogen source. Hydroxylamine (100%) and total nitrogen (71.86%) were efficiently removed under the following conditions: 30°C, sucrose as carbon source, carbon to nitrogen ratio 40, rotation speed 150 rpm, pH 9.58, and inoculant concentration of 0.58×108 colony-forming units. Ammonium was completely consumed by strain EM-A1, and 8.32 ± 0.08 mg/L of nitrate was produced during the ammonium removal process. During aerobic denitrification, the removal efficiencies of NO2−-N and NO3−-N by strain EM-A1 were 100 and 76.67%, respectively. There were about 29.34 ± 0.18%, 26.71 ± 0.36%, and 23.72 ± 0.88% initial total nitrogen lost as nitrogenous gas when NH4+, NO3−, and NO2− were separately used as the sole nitrogen source. The specific activities of ammonia monooxygenase, hydroxylamine oxidoreductase, nitrate reductase, and nitrite oxidoreductase were successfully detected as 0.37, 0.88, 0.45, and 0.70 U/mg protein, respectively. These results indicated that B. thuringiensis EM-A1 is a promising candidate for bioremediation of inorganic nitrogen from wastewater.
Introduction
In recent years, human activities have resulted in surface water and groundwater pollution by nitrogen (N) compounds in many areas of China. The death of aquatic animals caused by the eutrophication of water bodies is a typical example of the destruction of the nitrogen cycle through human activities (Heil et al., 2016; Soler-Jofra et al., 2021). The increasing emission of N compounds into the environment has become a serious threat to human health. Accumulation of large amounts of inorganic N could affect the reproduction and metabolic activities of humans and animals (Jiang, 2000). High N pollution has become an urgent problem for humans, animals, and nature (Jiang, 2000; Zhang et al., 2008; Wu, 2018). The efficient and effective removal of inorganic N from wastewater has become an important research field. Recently, N removal by classical biological nitrification–denitrification has been widely used as one of the most effective and economical processes for wastewater treatment.
Forty percent of surface water does not meet international drinking water standards, and the ammonium nitrogen (NH4+-N) is the main N-containing pollutant (Feng et al., 2012). Additionally, the nitrite (NO2−-N) could be generated from NH4+-N oxidation process. When NO2−-N is accumulated in the body, it may affect the delivery of oxygen through the blood and cause death by hypoxia (Zhang et al., 2013). Compared with NH4+-N and NO2−-N, the nitrate (NO3−-N), as another pollutant in wastewater, is more stable and difficult to biodegrade at normal temperature (Su et al., 2016). Therefore, the isolation of bacteria that can efficiently remove NH4+-N, NO2−N, and NO3−-N is very important to purify the N pollution wastewater.
Previous studies showed that hydroxylamine (NH2OH) is an inevitable intermediate in the process of heterotrophic nitrification (Chen and Ni, 2011; Chen and Ni, 2012; Huang et al., 2013). Excessive NH2OH accumulation would lower the total nitrogen (TN) removal efficiency and might also result in bacterial death in wastewater (Ouyang et al., 2020; Xing et al., 2020). For instance, 0.2, 1.0, and 5.0 mg/L of NH2OH strongly inhibited the activities of nitrite-oxidizing bacteria, Nitrobacter, and Nitrobacter agilis, respectively (Soler-Jofra et al., 2021). Unfortunately, the NH2OH could accumulate to 4.3 mg/L from ammonium oxidation in partial nitritation granular airlift, which could result in an increase of ammonium accumulation in a continuous partial nitrification airlift reactor (Poot et al., 2016; Soler-Jofra et al., 2021). Therefore, exploiting the bacteria that can effectively remove NH2OH is important to improve the wastewater treatment efficiency. However, the studies on nitrifying or denitrifying bacteria that can effectively remove NH2OH are rarely reported (Zhao et al., 2010b; Chen et al., 2014; Liu et al., 2019; Wang and He, 2020), because it is difficult to provide electrons to denitrifying bacteria through NH2OH nitrification (Jetten et al., 1997). In addition, the majority of heterotrophic nitrification and aerobic denitrification bacteria are unsuitable to purify the alkaline wastewaters, such as papermaking, chemical, textile, food, petroleum, chloro-alkali, and beverage industries (Jain et al., 2014; Xia et al., 2020).
In this study, the strain of EM-A1 was isolated with the sole NH2OH source, which was identified as Bacillus thuringiensis. The inorganic nitrogen removal efficiencies and intermediate products accumulation were conducted by a series of shake flask tests. The cell growth and NH2OH removal characteristics with the critical influence factors, such as inoculum size, carbon (C) source, C to N ratio (C/N), pH, rotation speeds, and temperature, were carefully investigated. The pathways of heterotrophic nitrification and aerobic denitrification were analyzed by nitrogen balance. Furthermore, the specific activities of ammonia monooxygenase, hydroxylamine oxidoreductase, nitrate reductase, and nitrite oxidoreductase involved in the removal of NH4+-N, NH2OH, NO3−-N, and NO2−-N were detected. The results observed from the above experiments may explain why strain EM-A1 could be used as an alternative microbial resource for the removal of multiple inorganic nitrogen forms, especially for NH2OH, from the wastewater treatment system.
Materials and Methods
Materials
The biogas residue sample was collected from biogas digester, Guiyang country, Guizhou province, China.
Media
The enrichment medium (g/L) consisted of 0.124 NH2OH.HCl, 3.6763 sodium citrate, 0.04 MgSO4, 1.5 KH2PO4, 0.009 Fe2(SO4)3, 0.014 CaCl2, and 3.5 K2HPO4. The pH was adjusted to 7.2.
The screening medium for NH2OH (g/L) consisted of 0.0992 NH2OH.HCl, 3.6763 sodium citrate, 3.5 K2HPO4, 1.5 KH2PO4, 0.04 MgSO4, 0.009 Fe2(SO4)3, and 0.014 CaCl2. The pH was adjusted to 7.2.
The bromothymol blue (BTB) solid medium (pH 7.0) contained (g/L): 8.5 sodium succinate, 1 NaNO2, 0.2 CaCl2·7H2O, 1 MgSO4·7H2O, 1 KH2PO4, 0.5 FeCl2·6H2O, BTB reagent 1 ml [1.5% in ethanol], and 20 agar (He et al., 2018).
LB medium (pH 7.2) was prepared according to standard procedures (Chen et al., 2011).
The basic medium (BM) (g/L) used to evaluate heterotrophic nitrification and aerobic denitrification consisted of 3.6763 sodium citrate, 1.5 KH2PO4, 0.144 KNO3, 0.0944 (NH4)2SO4 or 0.0986 NaNO2, 0.04 MgSO4, 0.009 Fe2(SO4)3, and 0.014 CaCl2. The pH was adjusted to 7.2. All media were autoclaved at 121°C for 30 min.
Bacterial Isolation and Identification
The sample from biogas digester (1 ml) was inoculated into enrichment medium and cultured for 4 days (20°C, 150 rpm), and the operation was repeated three times. The enriched culture (25, 35, and 45 µl) was spread on BTB agar plates with a glass rod. After inoculation (20°C, 48 h), bacteria with blue circular colonies were selected and cultured on fresh BTB plates. After 3–5 rounds of purification, the purified nitrifying bacteria were inoculated on LB solid medium (20°C, 48 h) and stored at 4°C for later experiments. All operations were performed under aseptic conditions.
The isolated single colonies were inoculated on BTB medium and the colony morphology was observed on the LB medium. The scanning electron microscopy (SU8100; Hitachi, Japan) is used for strain morphology, and gram staining was examined under optical microscopy (Olympus BX53-DIC; Olympus, Japan). The 16S rRNA gene was amplified by polymerase chain reaction (PCR) using universal primers 27F (5′-AGAGTTTGACCTGGCTAG-3′) and 1492R (5′-GGTTACCTTTTTTGTTACGACTT-3′) (Guo et al., 2013). The 16S rRNA gene sequencing was performed by General Biol (Anhui, China). Obtained alignment was performed by the database of BLAST. The phylogenetic tree was constructed by MEGA 6.0.
Nitrification and Denitrification Ability of Strain EM-A1
To evaluate the nitrification and denitrification capacity of strain EM-A1, three N compounds (NH4+, NO2−, and NO3−) were used as sole N source in BM instead of NH2OH. For each N compound, five species of sodium citrate were selected as the C sources. The OD600 of the inoculant was adjusted to 0.25 × 108 CFU in 250 ml Erlenmeyer flasks, and then incubated aerobically at 20°C, 150 rpm for 48 h.
Evaluation of NH2OH and Total N Removal Capacity
The effects of different culture conditions (inoculant concentration, dissolved oxygen (DO), temperature, C/N ratio, C source, and pH) on NH2OH and TN removal by strain EM-A1 were evaluated. The inoculant concentration was evaluated by the optical density at 600 nm (OD600). Controls were prepared containing screening medium without bacteria inoculation. After 91 h inoculation, cultures were taken to measure the cell density and various inorganic N contents (including NH4+-N, NO2−-N, NO3−-N, NH2OH, and TN). Single-factor-effect tests on the N removal capacity of strain EM-A1 were tested as follows: temperature (5, 10, 15, 20, 25, and 30°C), pH (6.52, 6.92, 7.45, 7.98, 8.56, and 9.58), rotation speed (0, 50, 100, 150, and 200 rpm), C source (sodium citrate, sodium potassium tartrate, sodium carbonate, sucrose, and glucose), C/N ratio (5, 10, 15, 20, 25, 30, 35, 40, and 45), and inoculum concentration (0.35 × 108, 0.42 × 108, 0.5 × 108, 0.58 × 108, and 0.68 × 108 colony-forming units (CFUs). Strain EM-A1 was inoculated at 20°C with rotation speed (150 rpm) for 91 h. All experiments were carried out at 20°C except for the temperature treatments. The removal efficiencies of NH2OH and TN were calculated to determine the optimal conditions for nitrification by strain EM-A1.
The formula of nitrogen removal efficiency was (L1−L2)/L1 × 100%, where L1 and L2 represent the initial and final concentrations of NH2OH (or TN) in the medium at 91 h, respectively.
Nitrogen Balance Analysis
To explore the nitrogen balance during inorganic nitrogen removal process, the strain EM-A1 was harvested after cultivation and centrifuged at 8,000 × g for 5 min. To ensure the inclusion of intracellular total nitrogen in the final total nitrogen, the Scientz-IID ultrasonic cell disruption system was used to break bacterial cells. The calculations of lost N as nitrogen gas were determined as described previously (Chen et al., 2021). And all the nitrogen balances of inorganic nitrogen were performed under the condition of C/N ratio of 15.
Enzyme Activities
After cultivation, the cells of strain EM-A1 were harvested with sole NH4+-N, NH2OH, NO2−-N, or NO3−-N and centrifuged at 6,000 × g for 5 min. The total protein was extracted using a protein extraction kit (CWBIO), and the protein concentration was determined using the BCA protein assay kit (Beijing, China). The ammonia monooxygenase (AMO) was detected by Welai Company (Guiyang, China). The HAO activity trial included 20 ml reaction solution contained potassium ferricyanide, Tris–HCl buffer (pH 7.5), NH2OH, and enzyme extract. The disappearance of hydroxylamine from the reaction mixture was used to demonstrate the HAO (Chen et al., 2021). For evaluation of the activity of nitrate reductase (NR), the reaction mixture (20 ml) contained Tris-HCl buffer (pH = 7.4), 0.02 mmol/l NADH, enzyme extract and nitrate. The disappearance of nitrate from the reaction mixture was used to estimate the activity of NR. The nitrite oxidoreductase (NIR) activity was measured by nitrite reductase kit (COMIN).
The specific activity (U/mg) was defined as the amount of enzyme that catalyzed the transformation of 1 μmol of the substrate per minute by the amount of protein in mg. The nonenzyme extract addition treatment was used as the blank controls. All assays were displayed in triplicate.
Analytical Methods
Bacterial growth was determined by OD600. The concentrations of TN, NO3−-N, NO2—N, and NH4+-N were measured by alkaline potassium persulfate digestion-ultraviolet spectrophotometry, ultraviolet spectrophotometry, N-(1-naphthalene)-diaminoethane photometry, and indophenol blue method, respectively (Guo et al., 2013). NH2OH was analyzed by indirect spectrophotometry (Frear and Burrell, 1955). The pH meter (DDS-307A, China) was used to detect pH. All experiments were tested in triplicate.
Results and Discussion
Identification of Strain EM-A1
Strain EM-A1 showed white circular, opaque, and smooth with dry surfaces on the LB plates (Figure 1A), gram positive (Figure 1B), and had rod-shaped cells (Figure 1C). BLAST alignment analysis exhibited that strain EM-A1 had the highest similarity (99%) with the B. thuringiensis. A phylogenetic tree was constructed and revealed that strain EM-A1 belongs to B. thuringiensis based on its phylogenetic relationship (Figure 2). Based on morphological analysis, strain EM-A1 was provisionally assigned to B. thuringiensis (GenBank accession MW551565.1).
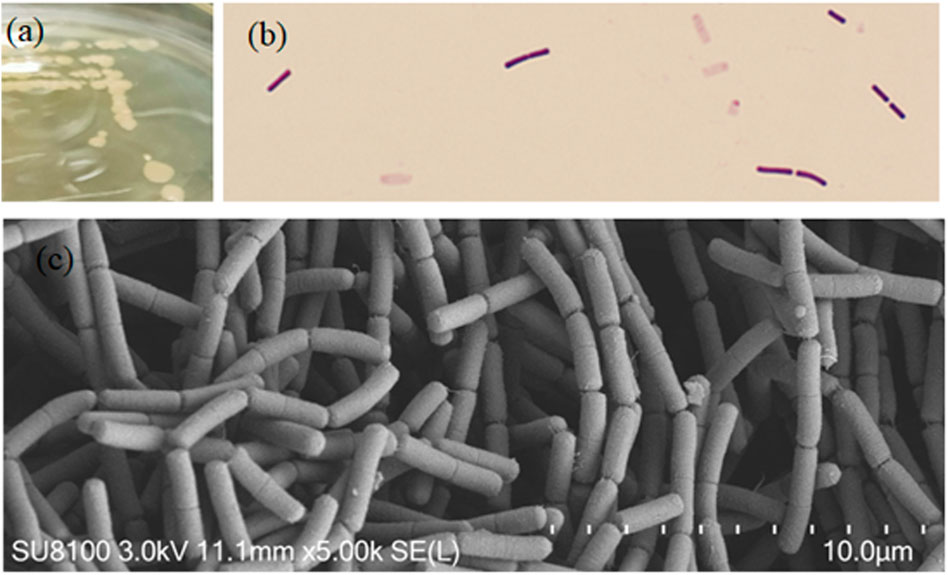
FIGURE 1. The morphologies of the strain EM-A1. Colony on LB plates (A), gram staining reaction by optical microscope (B), and cells under the scanning electron microscope (C).
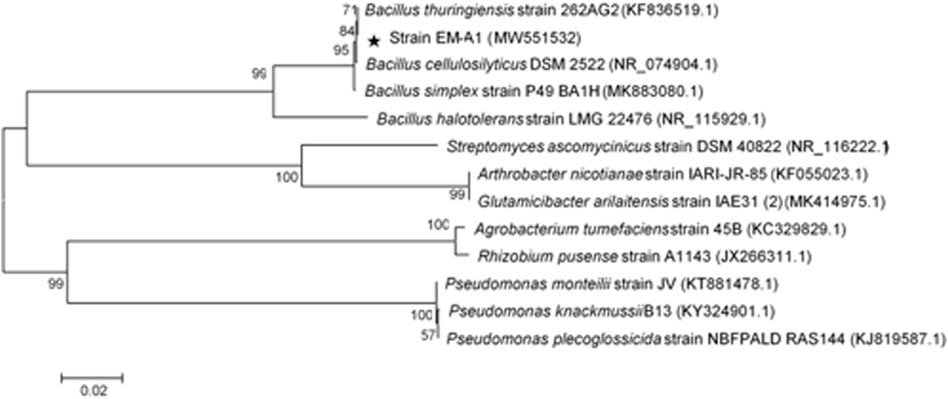
FIGURE 2. Neighbor-joining phylogenetic tree of the strain EM-A1 and related bacteria based on 16S rRNA sequence comparisons.
Analysis of N Removal Characteristics of Strain EM-A1
To determine whether the bacteria have the ability of nitrification, NH4+-N (20 mg/L) was selected as the N source in the BM. After 48 h incubation, NH4+-N was completely removed from the BM, with corresponding TN removal of 56.31% (Figure 3). These results showed that strain EM-A1 could effectively perform heterotrophic nitrification to remove NH4+-N. Meanwhile, no NO2−-N generation was observed during the NH4+-N oxidation process, while high NO3−-N concentration (8.32 ± 0.08 mg/L) was accumulated. The transformation of NH4+-N to NO3−-N indicated that the strain EM-A1 was capable of heterotrophic nitrification. The NO3−-N accumulation from NH4+-N oxidation was inconsistent with some studies, in which NH4+-N was first converted into NO2−-N and then into NO3−-N by heterotrophic nitrifying bacteria such as Pseudomonas sp. HXF1 (Hu et al., 2021) and Pseudomonas guganensis strain 4-n-1 (Motamedi and Jafari, 2020). In addition, the low TN removal efficiency by strain EM-A1 was possibly because of NO3−-N accumulation (Liu et al., 2015).
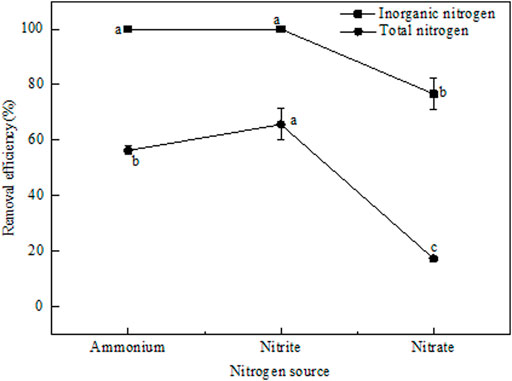
FIGURE 3. Nitrogen removal efficiency of strain EM-AI under aerobic conditions using ammonium and nitrite as sole nitrogen source. Values are presented as the means ± SD of the results of three replicates (error bars). Different letters indicate significant differences between treatments at p < 0.05.
Subsequently, the denitrification capacity of strain EM-A1 was measured by using NO2−-N or NO3−-N as the sole N source. When NO2−-N was selected as the only N source in BM, the NO2−-N was completely removed within 48 h, and no NH4+-N was observed, but NO3−-N (2.65 ± 0.52 mg/L) was observed during the process of NO2−-N transformation. These results were inconsistent with several reported strains, such as Pseudomonas mendocina IHB602 (Hong et al., 2020), Pseudomonas taiwanensis J488 (He et al., 2020), Streptomyces mediolani EM-B2 (He et al., 2021), and Acinetobacter sp. ND7 (Xia et al., 2020), in which no accumulation of NO3−-N was observed during the NO2−-N reduction process. When NO3−-N was selected as only N source, the NO3−-N removal efficiency was 76.67% after 48 h cultivation, and the residual concentration of NO3−-N was 5.74 ± 0.73 mg/L. Meanwhile, NO2−-N accumulation was hardly detected during NO3−-N removal, which contradicted the theory that NO2−-N accumulation is inevitable during biological treatment of wastewater (Zou et al., 2014; Zhao et al., 2018; Lang et al., 2020). Overall, these results showed that strain EM-A1 is a heterotrophic nitrifying-aerobic denitrifying bacterium that can efficiently remove low concentrations of NH4+-N, NO2−-N, and NO3−-N within 48 h.
Effects of Different Factors on NH2OH and TN Removal Capacity
Effect of Inoculum Concentration on NH2OH and TN Removal
The inoculum concentration is an important factor in biological N removal. Low inoculum concentrations will fail to achieve high N removal, while excessive inoculum concentrations might lead to bacterial death because of competition for nutrients (Ye et al., 2016). The NH2OH and TN removal efficiencies increased with increasing inoculum concentration (Figure 4A). When the inoculum concentration increased from 0.35 × 108 to 0.42 × 108 CFU, the removal efficiencies of NH2OH and TN increased from 71.14 and 6.67% to 84.19 and 11.76%, respectively. When the inoculum concentration increased to 0.58 × 108 CFU, the NH2OH and TN concentration decreased to 2.6 ± 0.68 mg/L and 18.02 ± 0.53 mg/L. The removal efficiencies of NH2OH and TN showed an increasing trend and reached the maximum values of 87.93 and 28.04%, respectively. Meanwhile, the accumulation of nitrite was only 0.92 ± 0.04 mg/L, which was different from the heterotrophic nitrifying bacteria Pseudomonas putida Y-9, which transformed the whole NH2OH to NO2− (Huang et al., 2019). A different relationship was observed between inoculum concentration and bacterial denitrification for high inoculum concentration. When the inoculum concentration was increased from 0.58 × 108 CFU to 0.68 × 108 CFU, the TN removal efficiency decreased significantly to 14% (p < 0.05). Therefore, to maintain the effective removal of NH2OH and TN, the inoculum concentration of strain EM-A1 needs to be maintained at 0.58 × 108 CFU.
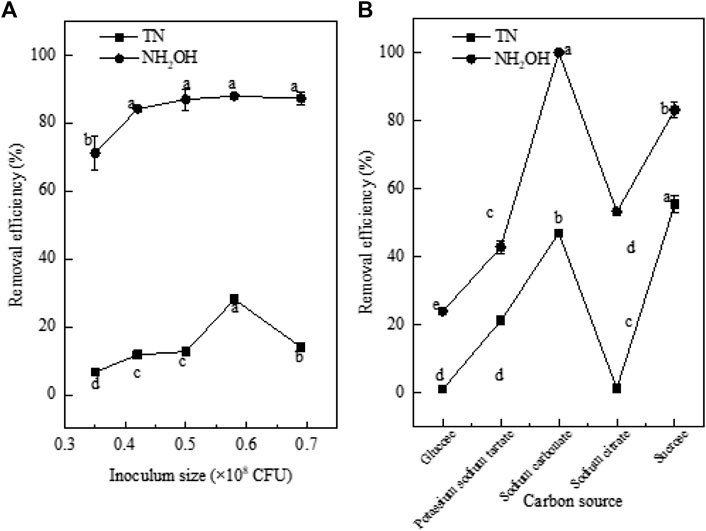
FIGURE 4. Effect of factors on hydroxylamine removal ability of strain EM-A1. Inoculum size (A), carbon source (B). Values are presented as the means ± SD of the results of three replicates (error bars). Different letters indicate significant differences between treatments at p < 0.05.
Effect of C Source on NH2OH and TN Removal
A C source is necessary for cell growth and metabolism of microorganisms, and different C sources directly affect the N removal ability of heterotrophic nitrifying bacteria (Ren et al., 2014). In this experiment, the effects of five C sources on the removal of NH2OH and TN by strain EM-A1 were studied. The bacterium could use a wide range of C sources, but only sodium carbonate and sucrose significantly promoted NH2OH removal (Figure 4B). When in a medium with sodium carbonate as the sole carbon source, whether inoculated or not, the removal efficiencies of hydroxylamine and total nitrogen were 100%, so we can infer that the removal of nitrogen is not related with bacteria. In addition to sodium carbonate, the NH2OH (83.1%) and TN (55.32%) removal efficiencies were highest when sucrose was used as the C source. After inoculation of 91 h, the levels of NH2OH and TN kept about 4.55 ± 0.88 mg/L and 12.36 ± 1.68 mg/L, respectively. There was little accumulation of NH4+-N (5.72 ± 0.22 mg/L), NO2−-N (0.05 ± 0.0.1 mg/L), and NO3−-N (1.64 ± 0.02 mg/L) observed during NH2OH oxidation with growth on sucrose. Therefore, sucrose was selected as the optimal C source for EM-A1. This result was consistent with that of Bacillus licheniformis FP6 (Nie et al., 2013), but was different from those for Bacillus methylotrophicus strain L7 (Zhang et al., 2012), Pseudomonas aeruginosa P-1 (Wei et al., 2021), Pseudomonas stutzeri GEP-01 (Gao et al., 2020), for which the optimal C sources were sodium succinate, glucose, and sodium citrate, respectively. These experimental results showed that carbohydrate was more beneficial to N removal than organic substances for strain EM-A1.
NH2OH and TN Removal at Different Temperature
Temperature is not only an important factor for maintaining enzyme activity but also has a great impact on various metabolic processes in cells (Huang, 2009). Previous reports showed that low temperature would reduce or even completely inhibit the growth, activity, and function of relevant ammonia-oxidizing bacteria, and eventually lead to failure of the nitrification process (Taylor and Bishop, 1989). In this research, the capacity of strain EM-A1 to remove NH2OH and TN at different temperatures was investigated. The N removal of strain EM-1 occurred over a wide temperature range (from 5°C to 30°C; Figure 5A). Increasing the temperature significantly enhanced the NH2OH and TN removal efficiencies. The NH2OH removal efficiency showed a gentle upward trend (from 19.25 to 46.04%) The temperature was changed from 5°C to 20°C, which was consistent with the theory that low temperature is not conducive to bacterial nitrification (He et al., 2018). When temperature rose from 20°C to 30°C, the NH2OH and TN removal was continuously enhanced, and the maximum NH2OH (93.44%) and TN (67.18%) removal efficiencies were observed at 30°C. Meanwhile, the low concentrations of NH2OH (1.43 ± 0.03 mg/L) and TN (7.73 ± 0.18 mg/L) were detected at the end of cultivation. When the temperature was increased from 5°C to 30°C, the concentrations of inorganic N (ammonium, nitrite, and nitrate) were increased from 2.54 ± 0.21 mg/L to 6.29 ± 0.3 mg/L, which might be because the higher temperature was more conducive to NH2OH conversion by strain EM-A1. The NH2OH and TN removal efficiency dropped sharply if the temperature exceeded 30°C, whether inoculated with strain EM-A1 or not. This phenomenon exhibited that high NH2OH removal efficiency at 35°C was connected with its instability at high temperature, rather than inoculation of strain EM-A1. Above all, the optimum temperature for strain EM-A1 is 30°C, which was consistent with several reports that the optimum temperature is 30–35°C (Shammas, 1986; Hammer and Knight, 1994).
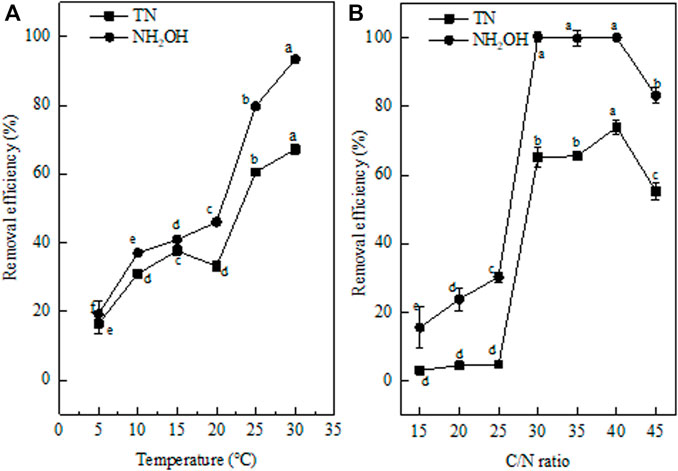
FIGURE 5. Effect of factors on hydroxylamine removal ability of strain EM-A1. Temperature (A), C/N ratio (B). Values are presented as the means ± SD of the results of three replicates (error bars). Different letters indicate significant differences between treatments at p < 0.05.
NH2OH and TN Removal Under Different C/N Ratios
Sufficient C is necessary for microbial growth (Yan et al., 2002). To explore the optimal C/N ratio for strain EM-A1, C/N ratios (15, 20, 25, 30, 35, 40, and 45) were tested. As shown in Figure 5B, the C/N ratio obviously affected the N removal capacity of strain EM-A1 (p < 0.05). When the C/N ratio was 15, the NH2OH (3.1%) and TN removal efficiencies (15.61%) were lowest, which might be because there was insufficient C for heterotrophic growth (Wan et al., 2017). When the C/N ratio was changed from 20 to 35, the NH2OH and TN removal efficiencies have expanded from 4.51 and 23.84% to 65.49 and 99.78%, respectively (p < 0.05). This showed that increasing the C/N ratio significantly improved the N removal ability of strain EM-A1. The removal efficiencies of NH2OH and TN were increased to the maximum of 100 and 73.91%, respectively, when the C/N ratio was 40. Only 6.94 ± 0.12 mg/L of TN was left in the medium. There was about C inorganic N (NH4+-N, NO2—N, and NO3−-N) accumulation during the NH2OH oxidation process, which indicated that NH2OH was mainly transformed to gaseous N (Liu et al., 2019; He et al., 2020). The result was consistent with the theory that heterotrophic nitrification bacteria usually require high concentrations of organic C for nitrification (Li et al., 2021; Zhang et al., 2021). However, this does not mean that the higher C/N ratios are more favorable for the nitrification ability of bacteria. When C/N ratio rose to 45, the removal efficiencies of NH2OH and TN decreased to 55.32 and 83.10%, respectively. Therefore, the optimum C/N ratio for strain EM-A1 was 40, which indicated that strain EM-A1 was more suitable for wastewater with high C content.
Effect of pH on Removal of Hydroxylamine and Total Nitrogen
According to previous reports, pH affects the absorption of nutrients and emission of membrane for bacteria by affecting cell membrane potential and emission of metabolites (Miao, 2011). The pH range suitable for nitrification by nitrifying bacteria is 7.9–9.0, and nitrification can be inhibited at pH values lower than 6.0 (Wang et al., 2003). Consequently, it is essential to explore the influence of pH on heterotrophic nitrification. As shown in Figure 6A, the removal efficiencies of NH2OH and TN increased with increasing pH. When the pH was 6.52, strain EM-A1 had the lowest removal efficiencies of NH2OH and TN, which were 25.31 and 0.1%, respectively. When the pH rose from 6.52 to 8.56, the removal efficiencies of NH2OH and TN significantly improved to 47.84 and 23.72%, respectively. These results showed that higher pH was more beneficial for NH2OH and TN removal by strain EM-A1. When the pH increased from 8.56 to 9.33, the NH2OH and TN removal efficiencies increased rapidly, and maximum NH2OH and TN removal efficiencies (100 and 71.86%) were observed when the pH was 9.33. Only 5.79 ± 0.63 mg/L of TN was not removed. However, after 91 h incubation, the NO2−-N accumulation peaked a maximum value of 2.22 ± 0.08 mg/L, and the concentration of NH4+-N was lowest (2.08 ± 0.08 mg/L) at pH 9.33. These aforementioned results suggested that a strong alkaline environment was more conducive to nitrification by strain EM-A1, which is inconsistent with previous studies, where the optimal pH of nitrification was 7.85 and 8–9 (Hans, 1994; Jiang et al., 2007; Chen et al., 2015). Strain EM-A1 is an alkali-resisting bacterium.
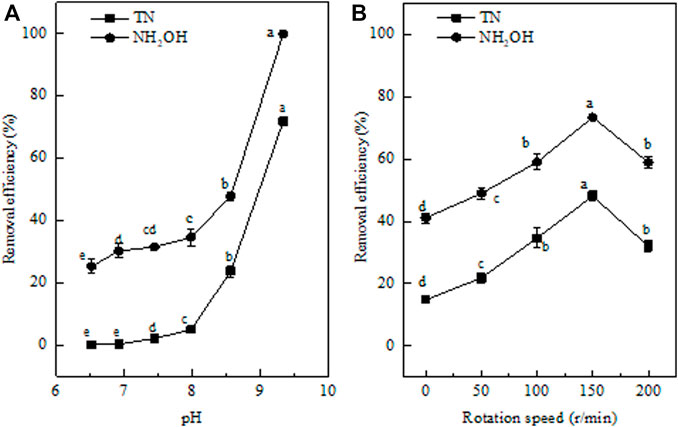
FIGURE 6. Effect of factors on hydroxylamine removal ability of strain EM-A1. pH (A), rotation speed (B). Values are presented as the means ± SD of the results of three replicates (error bars). Different letters indicate significant differences between treatments at p < 0.05.
The NH2OH and TN Removal at Different DO
To explore the influence of DO on the N removal ability of strain EM-A1, different shaker rotation speeds (0, 50, 100, 150, and 200 rpm) were measured. The N removal ability of strain EM-A1 was decreased with the increasing rotation speeds (Figure 6B).
For instance, when the rotation speed was stationary, the lowest NH2OH and TN removal efficiencies were achieved of 41.16 and 14.83%, respectively. The inorganic nitrogen of NO2−-N (0.80 ± 0.04 mg/L), NO3−-N (0.39 ± 0.36 mg/L), and NH4+-N (6.73 ± 0.0.15 mg/L) were accumulated. The relative high level of NH4+-N production in NH2OH oxidation at 0 rpm was inconsistent with a previous report (Liu and Wang, 2013). As the further increase of the speed was from 50 to 100 rpm, the NH2OH removal efficiency increased from 48.99 to 59.07%. The highest NH2OH and TN removal efficiencies of 73.42 and 48.2% were obtained when the speed climbed to 150 rpm. In such condition, the residual of NH2OH and TN was 7 ± 0.57 mg/L and 14.58 ± 0.47 mg/L, respectively. This further confirmed that high DO condition was more conductive to strain EM-A1 to perform heterotrophic nitrification and cell growth, which was also reported by several studies (Wang et al., 2007; Lei et al., 2019; Ouyang et al., 2020).
Nitrogen Balance Analysis in Process of Heterotrophic Nitrification and Aerobic Denitrification
The discrepancy results of the nitrogen balance among, NH4+-N, NO3—N, and NO2−-N were shown in Table 1. When NH4+-N was used as the sole nitrogen source, about 29.34% ± 0.18 of initial TN was removed as the nitrogenous gas after 24 h incubation. Only 0.72 ± 0.06 mg/L of NH4+ was remained in the nitrification system. There were low concentrations of NH2OH, NO3−—N, and organic-N accumulated. The nitrite was not detected even though the NH2OH was produced as 5.47 mg/L, which was different from that there was about 0.05–2.22 mg/L nitrite generation during the NH2OH oxidation process. This may be that a small amount of accumulation nitrite was rapidly converted into nitrogenous gas. Therefore, the nitrification pathway could be inferred as NH4+-N →NH2OH → NO3−-N→ NO2−-N → nitrogenous gas. When the NO3−-N and NO2−-N were separately used as the sole nitrogen, the nitrogen loss efficiency reached 26.71 ± 0.36% and 23.72 ± 0.88%, respectively. The nitrogen loss efficiency was conspicuously higher than that of 14.88% by Paracoccus denitrificans Z195 (Zhang et al., 2020) and 19.63% by Stenotrophomonas maltophilia DQ01 (Jia et al., 2019) when they were processed with nitrate alone. The NO2−-N accumulation was detected during NO2−-N transformation process. Thus, the denitrification pathway could be deduced as NO3−-N→ NO2−-N → nitrogenous gas.
Enzyme Analysis
To further analyze the nitrogen removal mechanism of strain EM-A1, AMO, HAO, NR, and NiR activities were examined. After cell lysis of strain EM-A1, the specific activities of AMO, HAO, NR, and NIR were detected as 0.37, 0.88, 0.45, and 0.70 U/mg protein, respectively (Table 1). Medhi et al. (2017) found that the extracellular-specific activities of AMO, NR, and NIR were 0.07, 0.023, and 0.122 U/mg protein, while they were 0.01, 0.006, and 0.01 U/mg protein intracellularly under aerobic condition in a strain of Paracoccus denitrificans ISTOD1. The specific activities of AMO, NR, and NIR in strain EM-A1 were greater than the sum of the intracellular and extracellular related specific activities in Paracoccus denitrificans ISTOD1. Additionally, the specific HAO activity (0.88 U/mg protein) in strain EM-A1 was higher than those detected in A. calcoaceticus HNR (0.051 U/mg protein) (Zhao et al., 2010a) and Pseudomonas PB16 (0.47 U/mg protein) (Jetten et al., 1997). The target heterotrophic nitrification and aerobic denitrification enzymes were successfully expressed and detected, which further confirmed that strain EM-A1 could be capable of full heterotrophic nitrification and aerobic denitrification. Combined with the results of nitrogen balance analysis, the possible pathway for nitrogen removal was identical to that of NH4+-N → NH2ON → NO2−-N → NO3−-N→ NO2−-N →N2O → N2, which was performed by B. thuringiensis WXN-23 (Xu et al., 2021).
Conclusion
B. thuringiensis EM-A1 was isolated from the biogas digester by using NH2OH as the sole N source. The optimal conditions for NH2OH removal were sucrose, C/N 40, temperature 30°C, pH 9.58, inoculant concentration of 0.58 × 108 CFU, and rotation speed of 150 rpm. B. thuringiensis EM-A1 efficiently removed low concentrations (20 mg/L) of NH4+-N, NO2−-N, and NO3−-N within 48 h. Combined with the results of intermediates accumulation, nitrogen balance analysis, specific activities of AMO, HAO, NR, and NIR, the possible pathway for nitrogen removal by strain EM-A1 was NH4+-N → NH2ON → NO2−-N → NO3−-N→ NO2−-N → nitrogen gas. Therefore, strain EM-A1 could be a promising candidate for inorganic nitrogen removal from wastewater.
Data Availability Statement
The datasets presented in this study can be found in online repositories. The names of the repository/repositories and accession number(s) can be found below: GenBank (accession number: MW551532).
Author Contributions
TW: Investigation, Experimental design, Experimental operation, Data calculation, Paper writing. MC: Investigation, Experimental design, Data calculation, Experimental operation, Figure construction. XL: Investigation, Experimental operation, Data calculation. FC: Experimental operation. TH: Investigation, Experimental idea, Conceptualization, Funding acquisition, Writing-review, Paper revisions. ZL: Investigation.
Funding
This work was supported by the Guizhou Province Science and Technology Planning Project (Qianke He Foundation-ZK (2021) General 233), the National Natural Science Foundation of China (No. 42007223), the Natural Science Research Young Talents Project of Provincial Education Department (No. Guizhou Education and KY word (2021) 086), Gui Da Zhuan Ji He Zi (2019) 04 and Gui Da cultivation (2019)50, the Science and Technology plan Project of Guizhou Province (Support of Guizhou Science and Technology Cooperation (2021)193). Undergraduate “SRT Plan” project, (Guida SRT (2021) No. 271) to TW.
Conflict of Interest
The authors declare that the research was conducted in the absence of any commercial or financial relationships that could be construed as a potential conflict of interest.
Publisher’s Note
All claims expressed in this article are solely those of the authors and do not necessarily represent those of their affiliated organizations, or those of the publisher, the editors, and the reviewers. Any product that may be evaluated in this article, or claim that may be made by its manufacturer, is not guaranteed or endorsed by the publisher.
References
Chen, M., Ding, C., He, T., Zhang, M., and Wu, Q. (2022). Efficient Hydroxylamine Removal through Heterotrophic Nitrification by Novel Bacterium Glutamicibacter Arilaitensis EM-H8. Chemosphere 288 (Pt 1), 132475. doi:10.1016/j.chemosphere.2021.132475
Chen, M., Wang, W., Feng, Y., Zhu, X., Zhou, H., Tan, Z., et al. (2014). Impact Resistance of Different Factors on Ammonia Removal by Heterotrophic Nitrification-Aerobic Denitrification Bacterium Aeromonas Sp. HN-02. Bioresour. Technol. 167, 456–461. doi:10.1016/j.biortech.2014.06.001
Chen, Q., and Ni, J. (2012). Ammonium Removal by Agrobacterium Sp. LAD9 Capable of Heterotrophic Nitrification-Aerobic Denitrification. J. Biosci. Bioeng. 113 (5), 619–623. doi:10.1016/j.jbiosc.2011.12.012
Chen, Q., and Ni, J. (2011). Heterotrophic Nitrification-Aerobic Denitrification by Novel Isolated Bacteria. J. Ind. Microbiol. Biotechnol. 38 (9), 1305–1310. doi:10.1007/s10295-010-0911-6
Chen, W., Ding, X. L., He, Y. L., Mo, Y., Xu, L. J., and Gao, S. F. (2011). Isolation and Identification of Nitrite Degrading Bacteria and Their Degradation Characteristics. Environ. Sci. Technol. 34 (S2), 37–41.
Chen, Z. H., Xu, S. S., Li, X., and Liu, F. X. (2015). The Influence of pH on the Efficiency of Anammox Reaction. Environ. Prot. Chem. industry 35 (2), 121–126.
Feng, S., Xie, S., Zhang, X., Yang, Z., Ding, W., Liao, X., et al. (2012). Ammonium Removal Pathways and Microbial Community in GAC-Sand Dual media Filter in Drinking Water Treatment. J. Environ. Sci. 24 (9), 1587–1593. doi:10.1016/s1001-0742(11)60965-0
Frear, D. S., and Burrell, R. C. (1955). Spectrophotometric Method for Determining Hydroxylamine Reductase Activity in Higher Plants. Anal. Chem. 27 (10), 1664–1665. doi:10.1021/ac60106a054
Gao, J., Zhu, T., Liu, C., Zhang, J., Gao, J., Zhang, J., et al. (2020). Ammonium Removal Characteristics of Heterotrophic Nitrifying Bacterium Pseudomonas Stutzeri GEP-01 with Potential for Treatment of Ammonium-Rich Wastewater. Bioproc. Biosyst Eng 43 (6), 959–969. doi:10.1007/s00449-020-02292-x
Guo, L., Chen, Q., Fang, F., Hu, Z., Wu, J., Miao, A., et al. (2013). Application Potential of a Newly Isolated Indigenous Aerobic Denitrifier for Nitrate and Ammonium Removal of Eutrophic lake Water. Bioresour. Technol. 142, 45–51. doi:10.1016/j.biortech.2013.05.021
Hammer, D. A., and Knight, R. L. (1994). Designing Constructed Wetlands for Nitrogen Removal. Water Sci. Technol. 29 (4), 15–27. doi:10.2166/wst.1994.0148
Hans, B. (1994). Use of Constructed Wetlands in Water Pollution Control: Historical Development, Present Status, and Future Perspectives. Water Sci. Technol. 30 (8), 209–223. doi:10.2166/wst.1994.0413
He, T., Ye, Q., Sun, Q., Cai, X., Ni, J., Li, Z., et al. (2018). Removal of Nitrate in Simulated Water at Low Temperature by a Novel Psychrotrophic and Aerobic Bacterium, Pseudomonas Taiwanensis Strain J. Biomed. Res. Int. 2018, 4984087. doi:10.1155/2018/4984087
He, T., Xie, D., Ni, J., Li, Z., and Li, Z. (2020). Nitrous Oxide Produced Directly from Ammonium, Nitrate and Nitrite during Nitrification and Denitrification. J. Hazard. Mater. 388, 122114. doi:10.1016/j.jhazmat.2020.122114
He, T. X., Wu, Q. F., Ding, C. Y., Chen, M. P., and Zhang, M. M. (2021). Hydroxylamine and Nitrite are Removed Effectively by Streptomyces Mediolani Strain EM-B2. Ecotoxicol. Environ. Saf. 224, 112693.
Heil, J., Vereecken, H., and Brüggemann, N. (2016). A Review of Chemical Reactions of Nitrification Intermediates and Their Role in Nitrogen Cycling and Nitrogen Trace Gas Formation in Soil. Eur. J. Soil Sci. 67 (1), 23–39. doi:10.1111/ejss.12306
Hong, P., Wu, X., Shu, Y., Wang, C., Tian, C., Wu, H., et al. (2020). Bioaugmentation Treatment of Nitrogen-Rich Wastewater with a Denitrifier with Biofilm-Formation and Nitrogen-Removal Capacities in a Sequencing Batch Biofilm Reactor. Bioresour. Technol. 303, 122905. doi:10.1016/j.biortech.2020.122905
Hu, X. F., Su, J. F., Ali, A. J., Wang, Z., and Wu, Z. Z. (2021). Heterotrophic Nitrification and Biomineralization Potential of Pseudomonas Sp. HXF1 for the Simultaneous Removal of Ammonia Nitrogen and Fluoride from Groundwater. Bioresour. Technol. 323, 124608. doi:10.1016/j.biortech.2020.124608
Huang, X., Li, W., Zhang, D., and Qin, W. (2013). Ammonium Removal by a Novel Oligotrophic Acinetobacter Sp. Y16 Capable of Heterotrophic Nitrification-Aerobic Denitrification at Low Temperature. Bioresour. Technol. 146, 44–50. doi:10.1016/j.biortech.2013.07.046
Huang, X., Xu, Y., He, T., Jia, H., Feng, M., Xiang, S., et al. (2019). Ammonium Transformed into Nitrous Oxide via Nitric Oxide by Pseudomonas Putida Y-9 under Aerobic Conditions without Hydroxylamine as Intermediate. Bioresour. Technol. 277, 87–93. doi:10.1016/j.biortech.2019.01.040
Huang, Y. (2009). Study on Metabolism Pathway of Nitrogen Removel by a New Strain Shanghai Jiao. Shanghai: Tong University.
Jain, R. M., Mody, K. H., Keshri, J., and Jha, B. (2014). Biological Neutralization and Biosorption of Dyes of Alkaline Textile Industry Wastewater. Mar. Pollut. Bull. 84 (1-2), 83–89. doi:10.1016/j.marpolbul.2014.05.033
Jetten, M. S. M., de Bruijn, P., and Kuenen, J. G. (1997). Hydroxylamine Metabolism in Pseudomonas PB16 Involvement of a Novel. Antoni. Leeuw 71, 69–74. doi:10.1023/a:1000145617904
Jia, Y., Zhou, M., Chen, Y., Luo, J., and Hu, Y. (2019). Carbon Selection for Nitrogen Degradation Pathway by Stenotrophomonas Maltophilia: Based on the Balances of Nitrogen, Carbon and Electron. Bioresour. Technol. 294, 122114. doi:10.1016/j.biortech.2019.122114
Jiang, A. X. (2000). The Mechanism and Countermeasures of Nitrogen Pollution in Water Environment. China's Popul. Resour. Environ. 10 (S2), 77–78.
Jiang, T. S., Yang, Q., Shang, H. T., Gan, Y. P., Bai, Y., and Wang, H. C. (2007). The Influence of Temperature and pH Value on Activated Sludge Method for Nitrogen and Phosphorus Removal. Chin. J. Environ. Eng. 1 (9), 10–14.
Lang, X., Li, Q., Ji, M., Yan, G., and Guo, S. (2020). Isolation and Niche Characteristics in Simultaneous Nitrification and Denitrification Application of an Aerobic Denitrifier, Acinetobacter Sp. YS2. Bioresour. Technol. 302, 122799. doi:10.1016/j.biortech.2020.122799
Lei, X., Jia, Y., Chen, Y., and Hu, Y. (2019). Simultaneous Nitrification and Denitrification without Nitrite Accumulation by a Novel Isolated Ochrobactrum Anthropic LJ81. Bioresour. Technol. 272, 442–450. doi:10.1016/j.biortech.2018.10.060
Li, D., Liu, R., Cui, X., He, M., Zheng, S., Du, W., et al. (2021). Co-culture of Bacteria and Microalgae for Treatment of High Concentration Biogas Slurry. J. Water Process Eng. 41, 102014. doi:10.1016/j.jwpe.2021.102014
Liu, G., and Wang, J. (2013). Long-term Low Do Enriches and Shifts Nitrifier Community in Activated Sludge. Environ. Sci. Technol. 47 (10), 5109–5117. doi:10.1021/es304647y
Liu, Y., Ai, G.-M., Wu, M.-R., Li, S.-S., Miao, L.-L., and Liu, Z.-P. (2019). Photobacterium Sp. NNA4, an Efficient Hydroxylamine-Transforming Heterotrophic Nitrifier/aerobic Denitrifier. J. Biosci. Bioeng. 128 (1), 64–71. doi:10.1016/j.jbiosc.2018.12.014
Liu, Y., Hu, T., Song, Y., Chen, H., and Lv, Y. (2015). Heterotrophic Nitrogen Removal by Acinetobacter Sp. Y1 Isolated from Coke Plant Wastewater. J. Biosci. Bioeng. 120 (5), 549–554. doi:10.1016/j.jbiosc.2015.03.015
Medhi, K., Singhal, A., Chauhan, D. K., and Thakur, I. S. (2017). Investigating the Nitrification and Denitrification Kinetics under Aerobic and Anaerobic Conditions by Paracoccus Denitrificans ISTOD1. Bioresour. Technol. 242, 334–343. doi:10.1016/j.biortech.2017.03.084
Miao, L. (2011). Isolation of Heterotrophic Nitrifying Bacteria and Characters of Removal of Ammonia. Changsha: Central South University.
Motamedi, H., and Jafari, M. (2020). Screening Heterotrophic Ammonia Removal and Aerobic Denitrifying Bacteria from Wastewater of Ammonia Production Units of a Petrochemical Industry. Curr. Microbiol. 77 (9), 2207–2214. doi:10.1007/s00284-020-02065-5
Nie, H. H., Zhao, Q. F., Li, C. H., Wang, Y. N., and Zhang, D. M. (2013). Isolation, Identification and Denitrification Characteristics of a Strain of Nitrogenous Nitrogen Removal Bacteria. Microbiol. China 40 (11), 2146–2155.
Ouyang, L., Wang, K., Liu, X., Wong, M. H., Hu, Z., Chen, H., et al. (2020). A Study on the Nitrogen Removal Efficacy of Bacterium Acinetobacter Tandoii MZ-5 from a Contaminated River of Shenzhen, Guangdong Province, China. Bioresour. Technol. 315, 123888. doi:10.1016/j.biortech.2020.123888
Poot, V., Hoekstra, M., Geleijnse, M. A. A., van Loosdrecht, M. C. M., and Pérez, J. (2016). Effects of the Residual Ammonium Concentration on NOB Repression during Partial Nitritation with Granular Sludge. Water Res. 106, 518–530. doi:10.1016/j.watres.2016.10.028
Ren, Y.-X., Yang, L., and Liang, X. (2014). The Characteristics of a Novel Heterotrophic Nitrifying and Aerobic Denitrifying Bacterium, Acinetobacter Junii YB. Bioresour. Technol. 171, 1–9. doi:10.1016/j.biortech.2014.08.058
Shammas, N. K. (1986). Interactions of Temperature, pH, and Biomass on the Nitrification Process. Journal (Water Pollution Control Federation) 58 (1), 52–59. doi:10.2307/25042841
Soler-Jofra, A., Pérez, J., and van Loosdrecht, M. C. M. (2021). Hydroxylamine and the Nitrogen Cycle: A Review. Water Res. 190, 116723. doi:10.1016/j.watres.2020.116723
Su, J. f., Ma, M., Huang, T. l., Ma, F., Shao, S. c., Lu, J. s., et al. (2016). Characteristics of Autotrophic and Heterotrophic Denitrification by the Strain Pseudomonas Sp. H117. Geomicrobiology J. 34 (1), 45–52. doi:10.1080/01490451.2015.1137661
Taylor, E. T., and Bishop, P. L. (1989). Distribution and Role of Bacterial Nitrifying Populations in Nitrogen Removal in Aquatic Treatment Systems. Pergamon 23 (8), 947–955. doi:10.1016/0043-1354(89)90167-x
Wan, W., He, D., and Xue, Z. (2017). Removal of Nitrogen and Phosphorus by Heterotrophic Nitrification-Aerobic Denitrification of a Denitrifying Phosphorus-Accumulating Bacterium Enterobacter cloacae HW-15. Ecol. Eng. 99, 199–208. doi:10.1016/j.ecoleng.2016.11.030
Wang, H. Y., Ma, F., Su, J. F., Zuo, W., Zhang, X. X., and Zhang, J. (2007). The Effect of Different Carbon Sources and Carbon-Nitrogen Ratios on the Denitrification Performance of an Aerobic Denitrifying Bacteria. Acta Scientiae (06), 968–972.
Wang, Q., and He, J. (2020). Complete Nitrogen Removal via Simultaneous Nitrification and Denitrification by a Novel Phosphate Accumulating Thauera Sp. Strain SND5. Water Res. 185, 116300. doi:10.1016/j.watres.2020.116300
Wang, X. W., Kong, Q. X., Jin, M., Song, N., Zheng, J. L., Gu, C. Q., et al. (2003). Effect of pH and Aeration on Nitrification of Nitrifying Bacteria. J. Prev. Med. Chin. PLA 21, 319–321.
Wei, R., Hui, C., Zhang, Y., Jiang, H., Zhao, Y., and Du, L. (2021). Nitrogen Removal Characteristics and Predicted Conversion Pathways of a Heterotrophic Nitrification-Aerobic Denitrification Bacterium, Pseudomonas aeruginosa P-1. Environ. Sci. Pollut. Res. 28 (6), 7503–7514. doi:10.1007/s11356-020-11066-7
Wu, Y. (2018). Study on Nitrogen Status of Urban Surface Water and Nitrogen Utilization Mechanism of Aquatic Plants. Tianjin: Tianjin University.
Xia, L., Li, X., Fan, W., and Wang, J. (2020). Heterotrophic Nitrification and Aerobic Denitrification by a Novel Acinetobacter Sp. ND7 Isolated from Municipal Activated Sludge. Bioresour. Technol. 301, 122749. doi:10.1016/j.biortech.2020.122749
Xing, C. Y., Fan, Y. C., Chen, X., Guo, J. S., Shen, Y., Yan, P., et al. (2020). The Effect of Hydroxylamine on Anammox Sludge Community. Environ. Sci. 41 (7), 3365–3372. doi:10.13227/j.hjkx.201911244
Yan, N., Jin, X. B., and Zhang, J. Q. (2002). Comparison of Methanol and Glucose as Carbon Sources in the Denitrification Process. J. Shanghai Normal Univ. (Natural Sci. Edition) 31 (03), 41–44. doi:10.13227/j.hjkx.201911244
Ye, J., Zhao, B., An, Q., and Huang, Y.-S. (2016). Nitrogen Removal by Providencia Rettgeri Strain YL with Heterotrophic Nitrification and Aerobic Denitrification. Environ. Technol. 37 (17), 2206–2213. doi:10.1080/09593330.2016.1146338
Zhang, D., Li, W., Huang, X., Qin, W., and Liu, M. (2013). Removal of Ammonium in Surface Water at Low Temperature by a Newly Isolated Microbacterium Sp. Strain SFA13. Bioresour. Technol. 137, 147–152. doi:10.1016/j.biortech.2013.03.094
Zhang, H., Li, S., Ma, B., Huang, T., Qiu, H., Zhao, Z., et al. (2020). Nitrate Removal Characteristics and 13C Metabolic Pathways of Aerobic Denitrifying Bacterium Paracoccus Denitrificans Z195. Bioresour. Technol. 307, 123230. doi:10.1016/j.biortech.2020.123230
Zhang, M., Pan, L., Su, C., Liu, L., and Dou, L. (2021). Simultaneous Aerobic Removal of Phosphorus and Nitrogen by a Novel Salt-Tolerant Phosphate-Accumulating Organism and the Application Potential in Treatment of Domestic Sewage and Aquaculture Sewage. Sci. Total Environ. 758, 143580. doi:10.1016/j.scitotenv.2020.143580
Zhang, Q.-L., Liu, Y., Ai, G.-M., Miao, L.-L., Zheng, H.-Y., and Liu, Z.-P. (2012). The Characteristics of a Novel Heterotrophic Nitrification-Aerobic Denitrification Bacterium, Bacillus Methylotrophicus Strain L7. Bioresour. Technol. 108, 35–44. doi:10.1016/j.biortech.2011.12.139
Zhang, Q. L., Wang, H., Zhang, L. Q., Li, J., and Tang, X. Q. (2008). The Impact of Nitrate Nitrogen Pollution in Drinking Water on Human Health. Groundwater 30 (01), 57–59.
Zhao, B., Cheng, D. Y., Tan, P., An, Q., and Guo, J. S. (2018). Characterization of an Aerobic Denitrifier Pseudomonas Stutzeri Strain XL-2 to Achieve Efficient Nitrate Removal. Bioresour. Technol. 250, 564–573. doi:10.1016/j.biortech.2017.11.038
Zhao, B., He, Y. L., Hughes, J., and Zhang, X. F. (2010a). Heterotrophic Nitrogen Removal by a Newly Isolated Acinetobacter Calcoaceticus HNR. Bioresour. Technol. 101 (14), 5194–5200. doi:10.1016/j.biortech.2010.02.043
Zhao, B., He, Y. L., and Zhang, X. F. (2010b). Nitrogen Removal Capability through Simultaneous Heterotrophic Nitrification and Aerobic Denitrification byBacillussp. LY. Environ. Technol. 31 (4), 409–416. doi:10.1080/09593330903508922
Keywords: hydroxylamine removal, heterotrophic nitrification, aerobic denitrification, hydroxylamine oxidoreductase, nitrogen balance
Citation: Wang T, Chen M, Liang X, Chen F, He T and Li Z (2022) The Alkali-Tolerant Bacterium of Bacillus thuringiensis EM-A1 Can Effectively Perform Heterotrophic Nitrification and Aerobic Denitrification. Front. Environ. Sci. 9:818316. doi: 10.3389/fenvs.2021.818316
Received: 19 November 2021; Accepted: 21 December 2021;
Published: 19 January 2022.
Edited by:
Likun Wang, Chinese Academy of Sciences (CAS), ChinaReviewed by:
Lei Yang, Xi’an University of Architecture and Technology, ChinaAng Li, Harbin Institute of Technology, China
Copyright © 2022 Wang, Chen, Liang, Chen, He and Li. This is an open-access article distributed under the terms of the Creative Commons Attribution License (CC BY). The use, distribution or reproduction in other forums is permitted, provided the original author(s) and the copyright owner(s) are credited and that the original publication in this journal is cited, in accordance with accepted academic practice. No use, distribution or reproduction is permitted which does not comply with these terms.
*Correspondence: Tengxia He, dHhoZUBnenUuZWR1LmNu