- 1Centre for Biogeochemistry in the Anthropocene, Department of Chemistry, University of Oslo, Oslo, Norway
- 2NILU-Norwegian Institute for Air Research, Kjeller, Norway
- 3Norwegian Institute for Water Research, Oslo, Norway
- 4Centre for Biogeochemistry in the Anthropocene, Department of Biosciences, University of Oslo, Oslo, Norway
- 5Aquatic Biology and Toxicology, Department of Biosciences, University of Oslo, Oslo, Norway
- 6Department of Natural Sciences, Mbeya University of Science and Technology, Mbeya, Tanzania
- 7Akvaplan-niva, Tromsø, Norway
- 8Institute for Marine Science, University of Dar es Salaam, Zanzibar, Tanzania
Temporal trends of industrial organic contaminants can show how environmental burdens respond to changes in production, regulation, and other anthropogenic and environmental factors. Numerous studies have documented such trends from the Northern Hemisphere, while there is very limited data in the literature from sub-Saharan Africa. We hypothesized that the temporal trends of legacy and contemporary industrial contaminants in sub-Saharan Africa could greatly differ from the regions in which many of these chemicals were initially produced and more extensively used. For this purpose, a dated sediment core covering six decades from a floodplain system in urban Dar es Salaam, Tanzania, was analysed. The samples were analysed for selected legacy persistent organic pollutants (POPs) [polychlorinated biphenyls (PCBs) and polybrominated biphenyl ethers (PBDEs)] and chemicals of emerging concern (CECs) [alternative brominated flame retardants (aBFRs), chlorinated paraffins (CPs), and dechloranes]. All groups of chemicals showed a steep increase in concentrations towards the uppermost sediment layers reflecting the more recent years. Concentrations of the individual compound groups in surface sediment were found in the order CPs >> aBFRs ∼ ∑25PBDEs > dechloranes ∼ ∑32PCBs. Time trends for the individual compounds and compound groups differed, with ∑32PCBs showing presence in sediments since at least the early 1960s, while some CECs first occurred in sediments corresponding to the last decade. Investigations into potential drivers for the observed trends showed that socioeconomic factors related to growth in population, economy, and waste generation have contributed to increasing concentrations of PBDEs, aBFRs, CPs, and Dechlorane Plus. Further monitoring of temporal trends of industrial organic contaminants in urban areas in the Global South is recommended.
Introduction
Legacy persistent organic pollutants (POPs), like polychlorinated biphenyls (PCBs) and polybrominated diphenyl ethers (PBDEs), have physicochemical properties which makes them persistent, bioaccumulative, toxic, and have a potential for long-range environmental transport. Some chemicals of emerging concern (CECs), like alternative brominated flame-retardants (aBFRs), chlorinated paraffins (CPs), and dechloranes, also share some of these properties. Time trends for production of these contaminants vary. PCBs and CPs have been produced since the 1930s, Dechlorane Plus (DP) from the 1960s, and PBDEs from the 1970s, while some aBFRs were not produced before the 1980s and 1990s (Breivik et al., 2002; Hoh et al., 2006; Covaci et al., 2011; Glüge et al., 2016; Abbasi et al., 2019). International regulation ensured an end to production of PCBs by the 1990s and PBDEs by the 2010s (Breivik et al., 2002; UNEP, 2017; Abbasi et al., 2019), while the CECs largely remain unregulated at an international level and production remains high or is increasing (Glüge et al., 2016; Xiong et al., 2019; Hansen et al., 2020). Both legacy POPs and CECs have been and are still used extensively in consumer products with different functions and user lifetimes. PCBs were applied in long-life electrical equipment, such as capacitors and transformers (Voogt and Brinkman, 1989), while PBDEs, aBFRs, CPs, and dechloranes have been, or still are used as flame retardants in e.g., electronics, building materials, and textiles. Many of the contemporary products containing CECs have shorter use lifetimes. Emissions to the environment may occur during production, but also during use of products containing legacy POPs and CECs, and from their disposal and recycling when these products reach their end of life.
Different histories for legacy POPs and CECs in terms of production, use, regulation, and waste disposal are thus likely to be reflected in long-term time trends of contaminant burdens in the physical environment. Research on time trends of these contaminants is therefore important to assess the effects of regulation and other emission-reducing measures, and to identify compounds that may merit further attention. Most studies on long-term temporal trends of POPs and CECs are from the Northern Hemisphere, where most of these chemicals have been more extensively produced and used. For instance, PCBs were mainly produced and/or used in North America, Europe, and Japan (Breivik et al., 2002). In these regions, environmental burdens peak around the time of peak production (Bogdal et al., 2008; Arp et al., 2011). Monitoring of atmospheric concentrations have documented declining primary emissions in recent decades (Schuster et al., 2010; Hung et al., 2016; Halvorsen et al., 2021; Wong et al., 2021).
However, elevated atmospheric concentrations of PCBs in some developing and newly industrialized regions in the Global South, including sub-Saharan Africa, indicate ongoing emissions (Klánová et al., 2009; Gioia et al., 2011). Unfortunately, studies on environmental levels of legacy POPs and CECs from sub-Saharan Africa are limited, particularly involving monitoring of long-term time trends (UNEP, 2021). The population and consumption is growing in urban areas in sub-Saharan Africa, though there is a low capacity for environmentally sound waste and e-waste handling. These regions are thus conceived to receive disproportionally large burdens of emissions at the end of the lifetime of products containing legacy POPs and CECs (Hoornweg and Bhada-Tata, 2012). Furthermore, elevated ambient temperatures may lead to enhanced emission of semi-volatile POPs and CECs from products in use and from waste (Breivik et al., 2011). These factors are likely to result in diverging long-term time trends compared to temperate regions in the industrialized Northern Hemisphere, indicating a need for further research in sub-Saharan Africa.
Studies assessing time trends are often based on monitoring of atmospheric levels. However, assessments of long-term time trends require monitoring over decades, yet atmospheric monitoring programs have only recently included CECs (Wong et al., 2021). Instead, sequentially settled and undisturbed sediments in e.g., fluvial floodplain systems may serve as archives documenting how levels of persistent contaminants in the physical environment have changed in response to changing emissions. This is possible as sediments function as a sink for legacy POPs and CECs, and individual sediment layers can be dated using radioisotopes (Korosi et al., 2015). The collection and analysis of sediment cores is therefore a relevant technique to assess long-term temporal trends of both legacy POPs and CECs in regions where long-term monitoring has been lacking.
The main objective of this study is to describe long-term temporal trends of selected legacy POPs and CECs in a dated sediment core from a sub-Saharan African urban region. Dar es Salaam, Tanzania, was chosen as the study area. This city has experienced growing consumption and population yet suffers from a limited capacity for handling municipal waste and e-waste (Yhdego, 2017; Mahenge et al., 2018). PCBs, PBDEs, aBFRs, CPs, and dechloranes were selected as target analytes as they represent divergent histories related to production, use, and international regulation. None of the selected chemicals are known to have been produced in the region. Their long-term trends in the dated sediment core are compared with results from similar studies from other parts of the world, with particular attention to potential differences between industrialized and developing regions. Finally, the likely socioeconomic and biogeochemical drivers affecting the observed trends are assessed.
Materials and Methods
Sampling Site
An urban area was targeted for sampling as the aim was to assess contaminants from local sources rather than contaminants from e.g., long-range transport. Sediment cores were thus collected from a tributary to the Msimbazi River in Tabata ward in Dar es Salaam (6° 49′ 17″ S, 39° 13′ 29″ E) (Figure 1) in February 2019. The size of the tributary river watershed is approximately 30 km2. Soil textures within the catchment are mainly sand and sandy clay (Igulu and Mshiu, 2020) while the sampled section of the riverbed mainly consisted of clay. A floodplain area was chosen for sampling since such terrains favour gravitational settling. The sediments are also less prone to be eroded due to the slow water movement (Heim and Schwarzbauer, 2013). Dar es Salaam has a wet tropical climate, with an annual rainfall of more than 1000 mm, mainly occurring during two rain seasons; the “long rains” in April-May and the “short rains” in October-November. Within the watershed, sources of legacy POPs and CECs to sediments are expected to include surface runoff, untreated wastewater, atmospheric deposition, and seepage from unsolicited scattered dumping of domestic waste.
Sample Collection
Two sediment cores, one for dating and one for legacy POPs and CECs analysis, were collected within ∼30 cm of each other using the inner tube (inner diameter 65 mm) of a gravity corer. Both cores were 39 cm in length, of which the top 15 cm were cut in 1 cm layers, while the lower sections were cut into 2 cm layers. Sectioning was done on-site, and slicing tools were rinsed in stream water between each slicing. Sediment layers were placed in pre-cleaned glass containers with aluminium foil-lined lids, transported in cooling bags, and subsequently stored in freezer after arrival at the laboratory.
Dating of Sediments
Layers from the sediment core collected for dating were shipped to GEL Laboratories, Charleston SC, United States, for radioisotope analysis and dating. 210Pb was determined by Alpha spectrometric analysis of its granddaughter in the 238U series, 210Po, which provide more accurate estimates of 210Pb compared to its direct analysis. 226Ra and 137Cs were determined using gamma spectrometry (Kim and Burnett, 1986). Sedimentation rate was modelled based on 210Pb that was not from the decay of 226Ra in the sediments (i.e., excess 210Pb) using the constant rate of supply (CRS) model and the constant flux: constant sedimentation (CF:CS) model as detailed in Appleby and Oldfield (1992).
Sample Preparation and Instrumental Analysis
Methods used for sample preparation and chemical analysis are detailed in the supplementary information (SI) and previously described for soils in Nipen et al. (2021). Only a summary is provided here. Approximately 2 g of wet sediment from each layer of the sediment core collected for legacy POPs and CECs analysis were dried and homogenized by mixing with sodium sulphate in a mortar. 13C labelled internal standards were added to each sediment layer before accelerated solvent extraction (ASE) using acetone/n-hexane (1:1). Approximately 2 g of activated copper powder was added to the extraction cells to remove elemental sulphur. Extracts were volume reduced, before concentrated sulphuric acid was added to remove lipids and other acid-labile potential instrumental interferences, followed by column chromatography clean-up with activated silica eluted with diethyl ether/n-hexane (1:9). The solvent was changed to isooctane, and volume reduced to 100 µl using a gentle stream of N2. A recovery standard of 1,2,3,4-tetrachloronapthalene was added before instrumental analysis.
Sediment concentrations of 32 PCBs, 25 PBDEs, and 14 aBFRs were determined using gas chromatography high-resolution mass spectrometry (GC-HRMS) (AutoSpec, Waters, Manchester, United Kingdom). Concentrations of 34 short chain CPs (SCCPs) congener groups, 28 medium chain CPs (MCCPs) congener groups, and nine dechlorane compounds were analysed using gas chromatography quadrupole time-of-flight high-resolution mass spectrometry (GC/Q-TOF) (Agilent, Santa Clara, United States). CPs were quantified using a pattern deconvolution procedure (Bogdal et al., 2015). A full inventory of congeners, congener groups, and acronyms used in the text is provided in Supplementary Table S1.
Water and organic matter (OM) content in sediment layers were determined gravimetrically by loss on drying at 110°C and loss on ignition (LOI) at 550°C, respectively. OM was determined by LOI followed by correction for loss of structural water in clay (Hoogsteen et al., 2015). Water content, which varied from 31% in the lowest sediment layer to 75% in the top layer, was used to calculate dry weight (dw) concentrations and bulk density.
Quality Assurance
All glassware were baked overnight at 450°C and rinsed with acetone and n-hexane before use. Components for ASE cells were sonicated in acetone twice, followed by sonication in n-hexane. High purity grade solvents were used throughout laboratory procedures. Field blanks (N = 3) and method blanks (N = 5) were analysed along with the sediment samples. Average blank levels were typically low, except for DBDPE, SCCPs, and MCCPs. High concentrations of these compounds in sediment samples ensured average blank concentrations only represented 6, 9, and 4% of average detected sample concentrations, respectively. Detailed information on blank levels is provided in Supplementary Table S2. Sample concentrations were blank corrected based on field and method blanks run with each sample batch. No significant difference was detected between field and method blanks (p < 0.05), so both were used to determine method detection limits (LODs). LODs were determined as three times the standard deviation of the blanks normalized to the average sample amount. LODs ranged between 0.0028 and 0.069 ng/g dw for PCBs, 0.0002 and 0.19 ng/g dw for PBDEs, and 0.0011 and 0.043 ng/g dw for aBFRs other than DBDPE. DBDPE had a higher detection limit of 1.7 ng/g dw. LOD for both SCCPs and MCCPs were 22 ng/g dw. No dechloranes were detected in blanks. Instrument detection limits, which ranged from 0.0041 to 0.083 ng/g dw, were therefore used for these compounds. For PCBs, PBDEs, and aBFRs two isotope m/z ratios were analysed for each compound, and results were to be excluded if the ratio diverged from the theoretical isotope ratio by more than 20%. However, no diverging ratios were found in samples with concentrations above the LODs.
Recoveries of internal standards were acceptable for the methods used. They varied between 48 ± 13% and 59 ± 6% for PCBs, 29 ± 7% and 51 ± 10% for PBDEs, 35 ± 11% and 48 ± 11% for aBFRs, 79 ± 15% and 90 ± 21% for dechloranes, and 71 ± 18% for CPs. Samples with internal standard recovery <15% were re-analysed. All legacy POPs and CECs were quantified using the isotope dilution principle, hence intrinsically correcting for recovery. The standard reference material SRM 1944 of an urban marine sediment, from the National Institute for Standards and Technology, was analysed for PCBs and PBDEs with 92 ± 14% and 89 ± 14% compliance, respectively.
Results and Discussion
Sediment Core Dating
The sediment layers contained low activities of excess 210Pb, rendering core dating challenging. However, a reasonably good exponential decay of 210Pb was measured in a mid-section of the core (8–20 cm depth). For this section, the sediment accumulation rate (SAR) was determined to be 0.27 ± 0.07 cm/year using the CRS model. This corresponds well with SAR determined using the CF:CS model (0.18 ± 0.03 cm/year). Dates for the top- and mid-section of the core were thus assigned based on the rate of sedimentation (CRS model) in the mid-section of the core, covering the time interval 1962 to 2019. Sediment layers below the mid-section of the core did not show exponential decay of 210Pb. This section could therefore not be dated with any confidence and is thus referred to as pre-1962. Activities of 137Cs were generally below or near detection limits and could thus not confirm the dating.
Concentrations and Time Trends of Legacy POPs and CECs
Temporal trends in concentrations of ∑32PCBs, ∑25PBDEs, aBFRs, CPs, and dechloranes with estimated age of sediment layers are shown in Figure 2. Concentrations and congener profiles in each sediment layer are provided in Supplementary Table S6 and Supplementary Figures S4–S8. All analysed PCBs and PBDEs congeners, and both SCCPs and MCCPs, were detected in one or more sediment layer. Of the 14 analysed aBFRs, the following were detected in one or more sediment layers: DBDPE, BTBPE, TBPH, ATE, a-TBECH, b-TBECH, g/d-TBECH, HBB, and PBBZ. Of these, only three (DBDPE, BTBPE, and TBPH) were detected in notable amounts (concentrations >0.1 ng/g dw) and with discernible time trends and are therefore included in further discussions. Of the nine analysed dechloranes, only DP (syn- and anti-isomers), Dec 602, and Dec 603 were detected. Overall, the highest concentrations were found for CPs, followed by aBFRs and ∑25PBDEs with concentrations two orders of magnitude lower than CPs, and dechloranes and ∑32PCBs with concentrations three orders of magnitude lower than CPs.
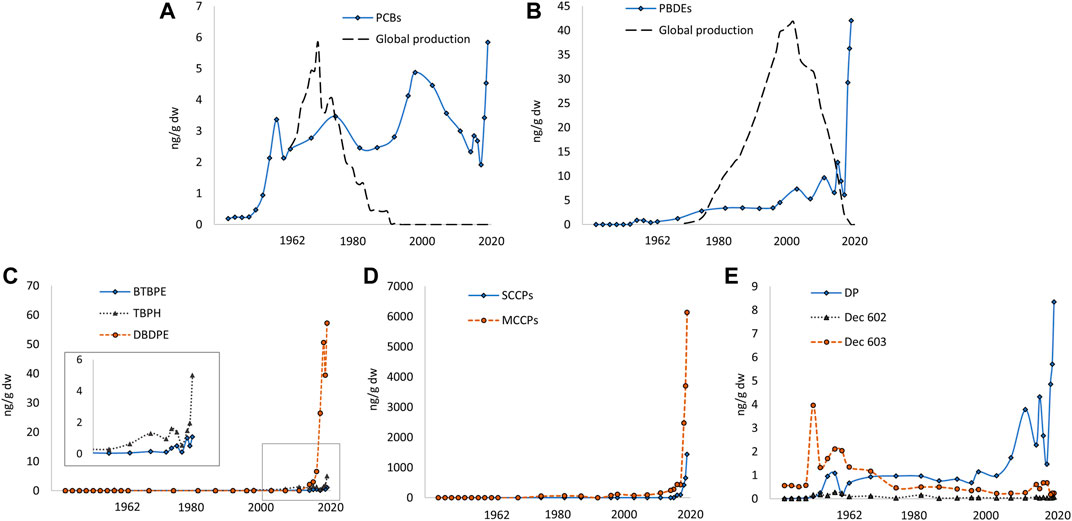
FIGURE 2. A,B) Concentration of ∑32PCBs and ∑25PBDEs, respectively, in sediments layers dated from before 1962–2019, along with trend in global production for PCBs from Breivik et al. (2002), and trend in global production for PBDEs from Abbasi et al. (2019). (C–E) Concentration of aBFRs, CPs, and dechloranes, respectively, in sediments layers dated from before 1962–2019.
Most of the legacy POPs and CECs showed steep increases in concentrations in sediment layers corresponding to the late 2010s. This trend was particularly notable for the CECs, with CPs and aBFRs showing 10–15 times increase from sediment layers corresponding to 2016/2017 to 2019. Of the dechloranes, DP increased five times in the same layers, but the levels of Dec 602 and Dec 603 remained relatively constant. Of the legacy POPs, ∑25PBDEs concentrations increased seven times, while ∑32PCBs concentrations tripled. Although the increase in ∑32PCBs in the late 2010s was small compared to some CECs, it is notable considering that the global production of PCBs peaked five decades ago (Breivik et al., 2002).
Tables 1, 2 lists the approximate year of peak concentration in sediment cores from this study and other studies for comparison, organized by region (developing and newly industrialized countries (Table 1) and industrialized countries (Table 2)). Temporal trends for the compound groups are discussed below, followed by a discussion of potential drivers for the observed increasing trends.
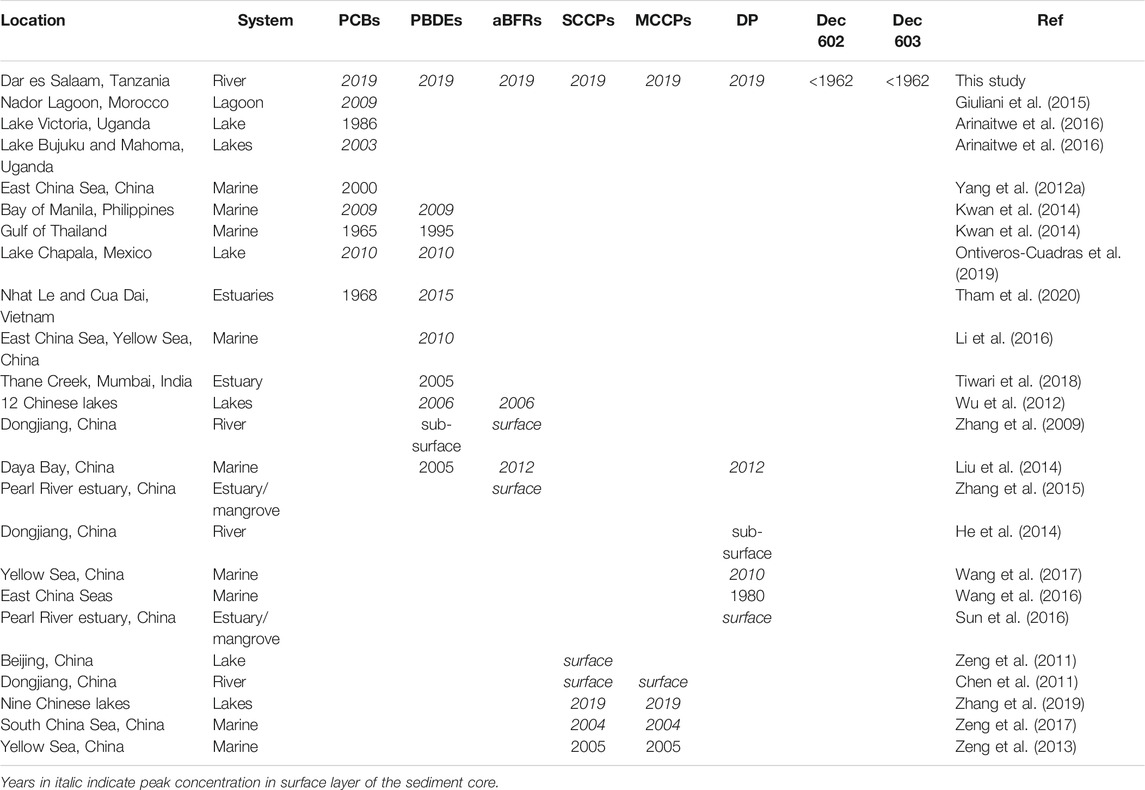
TABLE 1. Approximate year of peak concentration of PCBs, PBDEs, aBFRs, CPs, and dechloranes in the studied sediment core and in sediment cores from developing and newly industrialized regions, compiled from literature.
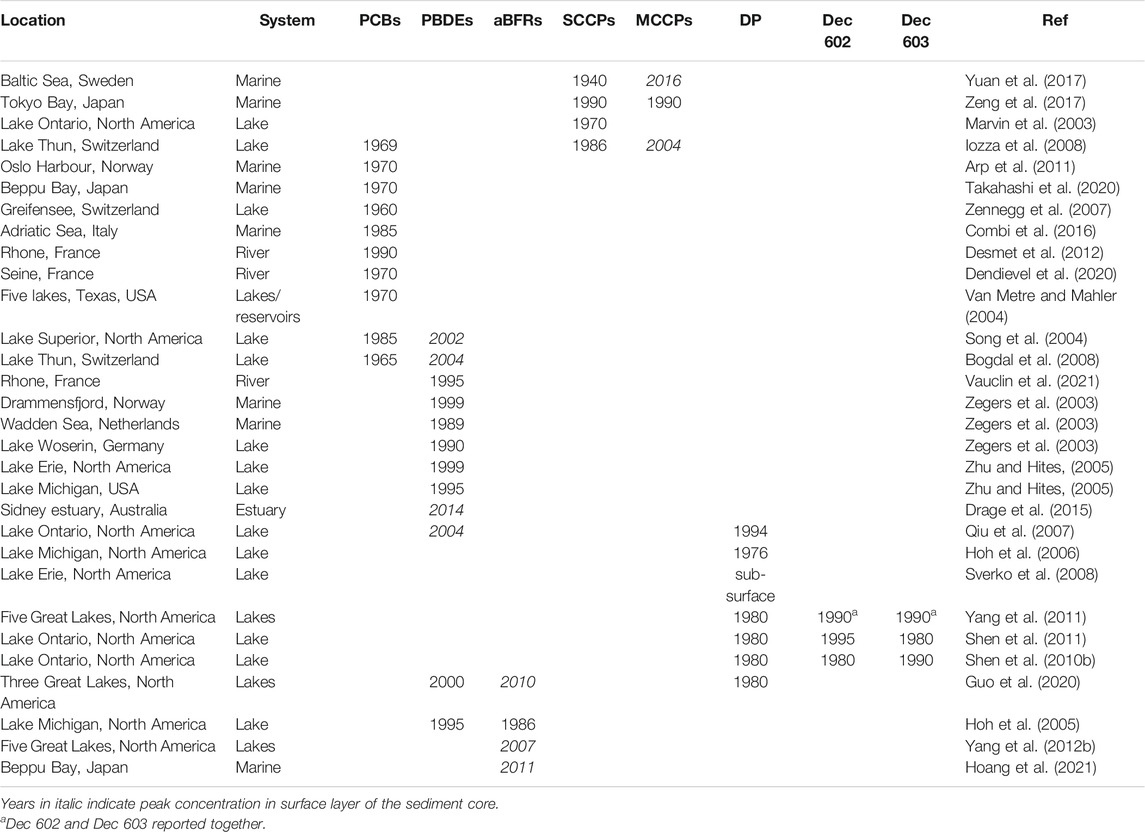
TABLE 2. Approximate year of peak concentration of PCBs, PBDEs, aBFRs, CPs, and dechloranes in sediment cores from regions which have remained industrialized for an extended time, compiled from literature.
Legacy POPs
∑32PCB concentrations varied between 0.19 ng/g dw in the deepest sediment layer and 5.8 ng/g dw in the surface sediment layer (Figure 2A). Subsurface peaks of ∑32PCBs were found in layers corresponding to pre-1962 (3.4 ng/g dw), the 1970s (3.5 ng/g dw), and the late-1990s (4.9 ng/g dw). The dominating congeners in the sediments were PCB-138 (on average 15%), PCB-153 (13%), PCB-149 (9.3%), PCB-101 (8.3%), PCB-118 (7.8%), PCB-180 (7.4%), PCB-28 (4.2%), and PCB-31 (3.0%). Individual PCB congeners had temporal trends which differed from each other (Illustrated for PCB-28 and PCB-180 in Figure 3). Concentrations of PCB-118, PCB-138, and PCB-180 peaked in subsurface layers, and the increase between 2017 and 2019 was less than factor 2. Lower chlorinated (e.g., PCB-28 and PCB-52) and higher chlorinated (e.g., PCB-206 and PCB-209) congeners peaked instead in the upper layers of the core, where concentrations increased by factors between 3 and 5 from layers corresponding to 2017–2019. The contribution of higher chlorinated congeners to ∑32PCB was however minor (Supplementary Figure S4). Lower chlorinated congeners are less persistent, and they reached peak production later compared to higher chlorinated congeners (Breivik et al., 2002). Both these factors may affect comparative time trends.
The temporal trend in ∑32PCBs (Figure 2A) in the studied sediment core was not consistent with trends found in sediment cores from industrialized countries. The PCBs loading in industrialized regions generally peak around 1970 (Table 2), reflecting the trends in global PCBs production (Figure 2A). In Tanzania, PCBs were mainly used in closed systems (e.g., electrical transformers) (IPEN, 2005) where emissions are likely to occur during or after decommissioning. This, in combination with long use-lives of transformers, may explain the comparatively low peak in PCBs concentrations observed in the 1970s followed by a higher peak in the late 1990s (Figure 2A). In this study, as in several other studies from developing and newly industrialized regions (Table 1), the highest PCBs concentrations are found in upper most recent layers of sediments. We attribute this to emissions from products and waste containing PCBs, causing delayed emissions compared to industrialized regions. For example, a Chinese study reported a bimodal peak in PCBs in a dated sediment core, with the first peak corresponding to the 1970s, and the second (higher) peak to the 2000s. These peaks were explained by PCBs production and e-waste processing, respectively (Yang H. et al., 2012).
∑25PBDE concentrations ranged from below LOD in the deeper sediment layers to 42 ng/g dw in the surface layer (Figure 2B). Concentrations increased from the 1970s to around 3 ng/g dw in the 1980s, where it remained relatively constant until it increased again from the 2000s. From its first detection onwards, BDE-209, the main congener of the decaBDE mixture, dominated the ∑25PBDE concentrations, comprising on average 81% of the total (range 71–90%). The relative contribution of BDE-209 increased with sediment depth (p < 0.05). This was an unexpected trend considering that penta- and octaBDE were regulated and phased out of production a decade earlier than decaBDE (Abbasi et al., 2019). Time trends for congeners associated with pentaBDE mixtures and octaBDE mixtures were relatively similar (Supplementary Figure S5). ∑25PBDEs and ∑32PCBs were strongly correlated (p < 0.001), and several individual PBDE and PCB congeners were also correlated (Supplementary Table S7). PBDEs are not known to have been produced before around 1970. Nevertheless, several PBDE congeners were detected in pre-1962 layers, though at low levels. Similar findings have been reported for PBDEs in sediment cores collected in China, the UK, and North America, with PBDEs detected in sediments dated to the 1960s and earlier (Wu et al., 2012; Liu et al., 2014; Yang et al., 2016; Da et al., 2019; Guo et al., 2020). The reason for detection of PBDEs in sediment layers corresponding to dates prior to its production is unknown. Potential explanations include contamination during core extrusion, uncertainty in dating of the older layers, or bioturbation of the sediments. These factors are also of relevance to the other analysed compound groups.
According to sediment core data from Western Europe, the environmental levels of PBDEs levelled off or decreased from the late 1990s and early 2000s (Bogdal et al., 2008; Vauclin et al., 2021). On the other hand, some studies from other industrialized countries conducted in the early 2000s and 2010s, documented increasing sediment concentrations of PBDEs (Table 2). This may reflect the continued presence of PBDEs in the anthroposphere. Contrary to flattening trends of PBDEs in Western Europe, and similarly to our study, Ontiveros-Cuadras et al. (2019) found increasing PBDEs concentrations in sediment cores from Mexico (Table 1). Li et al. (2016) also found increasing trends of PBDEs in sediment cores from the East China Sea. They explain this with shifts in industrial production towards China and import of waste containing PBDEs. The recent steep increase in PBDEs is therefore likely best explained by increasing disposal and recycling of waste products containing PBDEs.
CECs
The concentrations of DBDPE, BTBPE and TBPH ranged from <1.7 to 57, <0.01 to 1.1, and <0.06–5.0 ng/g dw, respectively (Figure 2C). DBDPE was first detected in sediment layers corresponding to 2014 and increased up to 57 ng/g dw in the surface layer. Studies on sediment cores from North America (Yang R. et al., 2012), Japan (Hoang et al., 2021), and China (Zhang et al., 2009; Wu et al., 2012; Zhang et al., 2015) show similar increasing concentrations of DBDPE (Tables 1, 2), though DBDPE was generally detected in layers down to deposits from the 1980s or 1990s. BTBPE was detectable in the sediment down to layers corresponding to the early 1980s, though concentrations increased from the late 1990s, and reached the highest concentration in layers corresponding to the late 2010s. Sediment cores from North America show BTBPE concentrations increasing from the 1970s, and levelling off between the mid-1980s and the early 2000s (Hoh et al., 2005; Yang R. et al., 2012). In a study from China, however, BTBPE concentrations were generally found to continue increasing to the upper sediment layers (Zhang et al., 2015). TBPH was detected down to sediment layers corresponding to the late 1990s. Concentrations remain relatively constant between 1 and 2 ng/g dw through the 2010s and increase to 5 ng/g dw in the surface sediment layer. A study from South China reported similar increasing concentrations of TBPH in sediments (Liu et al., 2014). The delayed appearance of DBDPE, BTBPE, and TBPH in the sediments from Dar es Salaam, compared to studies from North America and China, is likely due to differences in industrial activity. DBDPE, BTBPE, and TBPH all correlated strongly with ∑25PBDEs and individual PBDEs congeners. BTBPE and TBPH also showed strong correlations to ∑32PCBs (p < 0.001) (Supplementary Table S7).
MCCPs dominated over SCCPs with concentrations varying from <22 to 6,200 and to 1,400 ng/g dw, respectively. The highest CPs concentrations were found in the surface sediment layer, with strongest increase occurring between 2017 and 2019 (Figure 2D). MCCPs were detected in all sediment layers corresponding to the mid-1990s onwards, while SCCPs were first detected in layers corresponding to the mid-2010s. Time trends of SCCPs and MCCPs were strongly correlated to ∑25PBDEs and aBFRs (p < 0.001) (Supplementary Table S7). In sediment cores from Western Europe, SCCPs concentrations peaked around the 1980s and 1990s, while MCCPs generally show continued increasing trends (Iozza et al., 2008; Yuan et al., 2017). In sediment cores from China, which has sustained high CPs production, both SCCPs and MCCPs concentrations in general show the highest concentrations in surface layers (Zeng et al., 2012; Zhang et al., 2019) (Tables 1, 2). There is no known production of CPs in Tanzania, so the continued increasing trend is likely connected to the increased use and disposal of CPs containing products, possibly in combination with increased applications of CPs in metal industry. Elevated levels of CPs have been measured in air and soil in and around Dar es Salaam. Spatial trends suggested that municipal waste and e-waste were important sources (Nipen et al., 2021). Surface sediment concentrations of SCCPs and MCCPs were comparable to or higher than the highest soil concentration found in urban areas (1600 and 3200 ng/g dw, respectively, (Nipen et al., 2021). Moreover, congener group profiles of CPs in sediments were more comparable to urban soil than to urban air (SI Text 2).
DP was the dominant dechlorane compound in the sediment (Figure 2E), with concentrations ranging from <0.02 to 8.4 ng/g dw. Dec 602 and Dec 603 concentrations ranged from <0.01 to 0.27, and 0.18–4.0 ng/g dw, respectively. DP was detected in pre-1962 layers and remained stable at around 1 ng/g dw until the early 2000s. From the mid-2000s, concentrations fluctuated over a general increase to peak concentration in the surface layer. As far as we are aware, this study is the first to report sediment core based long-term time trends for dechloranes from outside regions where they are produced (the North American Great Lakes region and China). In the Great Lakes region, DP concentrations have generally increased from the 1960s or 1970s, usually peaking in the 1980s or 1990s, although some sediment cores showed continued increasing trends into the upper layers (Qiu et al., 2007; Shen et al., 2010b; Shen et al., 2011; Yang et al., 2011) (Table 2). In China, the vertical profile of DP in sediments show increasing trends towards the surface layers (Table 1). Dec 603 concentration in the studied sediment peaked in layers corresponding to pre-1962 (Figure 2E), followed by comparatively low concentrations in subsequent sediment layers. In sediment cores from the Great Lakes region, Dec 603 increased from the 1950s or 1960s, and peaked around the 1980s or 1990s (Shen et al., 2010b; Shen et al., 2011) (Table 2). Shen et al. (2010a) describe Dec 603 as an impurity in the historically used pesticides Aldrin and Dieldrin. They observed spatial trends of Dec 603 in sediments in the Great Lakes corresponding to pesticide use. Historic pesticide use is also a plausible explanation for the trends for Dec 603 in this study (SI Text 3). The levels of Dec 602 also peaked in pre-1962 sediment layers (Figure 2E). The Dec 602 peak was followed by declining concentrations, with stable low concentrations around or just below 0.05 ng/g dw from the mid-1980s up to the surface sediment layer. In sediment cores from the Great Lakes region, Dec 602 concentrations increased from the 1960s or 1970s and peaked around the 1980s and 1990s (Shen et al., 2010b; Shen et al., 2011). Dec 602 only showed a significant correlation with Dec 603 (p < 0.01), while correlations between Dec 603 and other CECs and legacy POPs were generally negative. Although DP showed strong positive correlations with both the legacy POPs (∑32PCBs and ∑25PBDEs) and the other CECs (aBFRs and CPs) (p < 0.001 for all), the continued presence of DP in sediments over decades demonstrate that it is not a “new” contaminant in this region.
Spatial trends and elevated levels of DP in air and soil in Dar es Salaam suggest e-waste as an important source (Nipen et al., 2021). The levels of DP in the surface sediment layer were higher than the highest concentrations found in urban soil (5.0 ng/g dw). Dec 602 and Dec 603 concentrations in surface sediment (0.07 and 0.2 ng/g dw, respectively) were comparable to the highest soil concentrations found in urban areas (0.05 and 0.3 ng/g dw, respectively). Moreover, the study by Nipen et al. suggested that the Dec 603 sources were of a historic nature, which is consistent with the findings in this study.
Potential Drivers for Time Trends of Legacy POPs and CECs
There are several conceptual explanations for the recent increasing trends in POPs and CECs, both in terms of the socioeconomic development in the region and due to shifts in environmental pressures on biogeochemical processes.
Growth in urban population and especially in per capita Gross Domestic Product (GDP) (Figure 4A) imply growth in consumption and general waste generation (Hoornweg and Bhada-Tata, 2012). Further, the very strong growth in mobile and broadband subscriptions, along with the amount of e-waste generated in the region, show that consumption has been particularly strong for electronics during the last decade. Strong correlations were found between these socioeconomic parameters and sediment concentrations of ∑25PBDEs, aBFRs, DP, and CPs (p < 0.001). Dec 602, Dec 603, and ∑32PCBs were not correlated with any of the socioeconomic parameters, although some individual congeners (PCB-28 and PCB-206, p < 0.001) were (Supplementary Table S8). Strong temporal correlations between socioeconomic parameters and the level of flame retardants (PBDEs, aBFRs, DP, along with CPs) in the sediment layers, suggest that increases in consumption and waste generation of consumer goods containing these additives contribute to the increase in these pollutants during the late 2010s. PCB-28 and PCB-206 have been reported to occur as unintentional by-products in pigment production (Anh et al., 2020). Consequently, use and disposal of pigment-containing consumer products may be a contributing factor for the increasing trend for these congeners in the 2010s. These findings suggest that inadequate handling and recycling of waste has led to increasing pollutant levels in the urban environment.
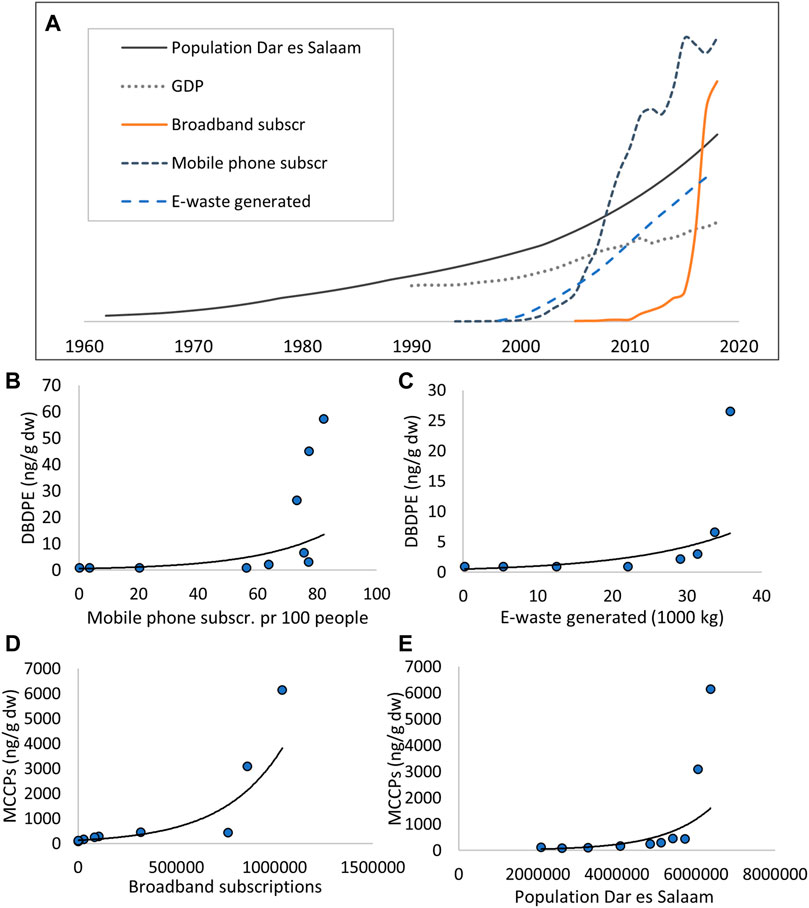
FIGURE 4. (A) Time trends of some socioeconomic factors in Tanzania (GDP, broadband subscriptions, mobile phone subscriptions, e-waste generated) and Dar es Salaam (population). For comparison purposes data have been converted to unitless numbers by normalizing using the mean. Original data for population, GDP per capita, Broadband subscriptions, and mobile phone subscriptions are gathered from the World Bank (WorldBank, 2021). E-waste data are from the Tanzanian National Beaureu of Statistics (NBS, 2019). (B,C) Concentrations of DBDPE plotted against mobile phone subscriptions and e-waste from 1998 to 2019 (e-waste data only available to 2017). (D,E): Concentrations of MCCPs plotted against broadband subscriptions and population in Dar es Salaam from 1998 to 2019.
The exponential rather than linear relationship between CECs and socioeconomic factors (Figures 4B–E) suggests that additional factors may contribute synergistically to increasing concentrations. Elevated concentrations of organic pollutants in sediment layers may be due to erosion of contaminated topsoil or other contaminated surface reservoirs within the watershed caused by intense rainfall and flooding events (Lu et al., 2016). Correlations of rainfall versus legacy POPs and CECs were investigated for two different time intervals (1983–2018 and 2010–2018), using rainfall data from the Tanzanian Meteorological Authority (TMA, 2021). The longer and shorter time intervals were chosen based on the likelihood of legacy POPs and CECs being present in topsoil, respectively. No significant correlations were found for the time interval 1983–2018 (Supplementary Table S9). For the time interval 2010 to 2018, the rainfall episodes between 2010 and 2012 were less intense, around average between 2013 and 2016, and stronger than average in 2017 and 2018. For the time interval 2010 to 2018, CPs and DBDPE were significantly correlated with rainfall (p < 0.05 and p < 0.01, respectively). Similarities in CPs congener group profiles in soil from urban areas and sediment, as well as comparable concentrations, support that increased erosion of contaminated soil has contributed to the increasing trend of CPs in sediments.
Strong associations are expected between OM content and the hydrophobic legacy POPs and CECs (Supplementary Figure S11). OM content in the sediment varied between 1% in the lowest sediment layer to 9% in the top layer. The OM content was strongly positively correlated to sediment concentration of most PCB and PBDE congeners, ∑32PCBs, ∑25PBDE, TBPH, BTBPE, DP, and MCCPs (p < 0.001). OM content was also significantly correlated to PCB-209, DBDPE, and SCCPs (p < 0.05), while there were no or negative correlations between OM and Dec 602 and Dec 603. However, the main features of trends of legacy POPs and CECs, including strong increases in the late 2010s and subsurface peaks for PCBs, remain when concentrations are normalized to OM content (Supplementary Figure S12). If OM content was the main explanatory factor governing the time trends, there would have been a “smoothing out” of the concentration differences between the sediment layers. The trend in OM content is therefore not likely to be the main driver for the observed temporal trends.
Overall, these findings suggest that the increasing trends in legacy POPs and CECs in sediment layers corresponding to the late 2010s are mainly a consequence of increased primary emissions from products and waste in connection with the rapid socioeconomic development in the region. However, secondary emissions in the form of remobilized surface reservoirs of legacy POPs and CECs are likely to have contributed to the increasing trends, particularly for CPs and DBDPE.
Concluding Remarks
This study showed that concentrations of both legacy POPs and CECs, particularly CPs and DBDPE, have increased notably in sediment layers corresponding to the late 2010s. Other studies from industrialized regions, as well as other developing and newly industrialized regions, in general also show increasing trends for these CECs. This suggests that particular attention should be given to these compounds in future monitoring studies, both regionally and internationally. Comparisons of time trends of legacy POPs showed that the flattening and declining trends seen in most industrialized regions, as a response to regulation and emission controls, are not reflected in the studied region, nor in most other developing and newly industrialized regions. This underlines the importance of improved emissions controls in waste handling and recycling in these regions. This should be further prioritized at a regional level, but also in international regulatory efforts relating to trade in products, used products, and waste that contain these compounds.
More studies of sediment cores are needed to further document historical emission trends in developing and newly industrialized regions. Monitoring of atmospheric concentrations of legacy POPs and CECs in urban areas in these regions may also be an important additional asset to assess the effect of emissions reductions, as atmospheric concentrations respond more rapidly to changes in emissions.
Data Availability Statement
The raw data supporting the conclusions of this article will be made available by the authors, without undue reservation.
Author Contributions
MN, RV, PB-N, KBo, and KBr conceptualized the study, MN wrote the original draft, while RV, PB-N, KBo, EM, AB, MS, GC, AM, and KBr contributed to review and editing of the manuscript. Formal analysis was conducted by MN. Investigation was conducted by MN, EM, AB, and GC. Supervision was provided by RV, PB-N, KBo, MS, and KBr, and KBo was project administrator.
Funding
The AnthroTox project is funded through the University of Oslo Life:Science initiative and the Norwegian Ministry of Education and Research.
Conflict of Interest
The authors declare that the research was conducted in the absence of any commercial or financial relationships that could be construed as a potential conflict of interest.
Publisher’s Note
All claims expressed in this article are solely those of the authors and do not necessarily represent those of their affiliated organizations, or those of the publisher, the editors and the reviewers. Any product that may be evaluated in this article, or claim that may be made by its manufacturer, is not guaranteed or endorsed by the publisher.
Acknowledgments
This study was conducted as part of the AnthroTox project, a convergence environment combining social and natural sciences to study anthropogenic toxicants. We thank Signe Mikkelsen for contributions during fieldwork. Modelling work for sediment dating was carried out by W. C. Burnett, Florida State University, for GEL Laboratories.
Supplementary Material
The Supplementary Material for this article can be found online at: https://www.frontiersin.org/articles/10.3389/fenvs.2021.805544/full#supplementary-material
References
Abbasi, G., Li, L., and Breivik, K. (2019). Global Historical Stocks and Emissions of PBDEs. Environ. Sci. Technol. 53, 6330–6340. doi:10.1021/acs.est.8b07032
Anh, H. Q., Watanabe, I., Minh, T. B., and Takahashi, S. (2020). Unintentionally Produced Polychlorinated Biphenyls in Pigments: An Updated Review on Their Formation, Emission Sources, Contamination Status, and Toxic Effects. Sci. Total Environ. 755, 142504. doi:10.1016/j.scitotenv.2020.142504
Appleby, P., and Oldfield, F. (1992). “Applications of lead-210 to Sedimentation Studies,” in Uranium-Series Disequilibrium: Applications to Earth, Marine, and Environmental Sciences. 2nd Edn. Oxford: Clarendon Press.
Arinaitwe, K., Rose, N. L., Muir, D. C. G., Kiremire, B. T., Balirwa, J. S., and Teixeira, C. (2016). Historical Deposition of Persistent Organic Pollutants in Lake Victoria and Two Alpine Equatorial Lakes from East Africa: Insights into Atmospheric Deposition from Sedimentation Profiles. Chemosphere 144, 1815–1822. doi:10.1016/j.chemosphere.2015.10.061
Arp, H. P. H., Villers, F., Lepland, A., Kalaitzidis, S., Christanis, K., Oen, A. M. P., et al. (2011). Influence of Historical Industrial Epochs on Pore Water and Partitioning Profiles of Polycyclic Aromatic Hydrocarbons and Polychlorinated Biphenyls in Oslo Harbor, Norway, Sediment Cores. Environ. Toxicol. Chem. 30 (4), 843–851. doi:10.1002/etc.466
Bogdal, C., Alsberg, T., Diefenbacher, P. S., MacLeod, M., and Berger, U. (2015). Fast Quantification of Chlorinated Paraffins in Environmental Samples by Direct Injection High-Resolution Mass Spectrometry with Pattern Deconvolution. Anal. Chem. 87 (5), 2852–2860. doi:10.1021/ac504444d
Bogdal, C., Schmid, P., Kohler, M., Müller, C. E., Iozza, S., Bucheli, T. D., et al. (2008). Sediment Record and Atmospheric Deposition of Brominated Flame Retardants and Organochlorine Compounds in Lake Thun, Switzerland: Lessons from the Past and Evaluation of the Present. Environ. Sci. Technol. 42 (18), 6817–6822. doi:10.1021/es800964z
Breivik, K., Gioia, R., Chakraborty, P., Zhang, G., and Jones, K. C. (2011). Are Reductions in Industrial Organic Contaminants Emissions in Rich Countries Achieved Partly by Export of Toxic Wastes? Environ. Sci. Technol. 45 (21), 9154–9160. doi:10.1021/es202320c
Breivik, K., Sweetman, A., Pacyna, J., and Jones, K. (2002). Towards a Global Historical Emission Inventory for Selected PCB Congeners - A Mass Balance Approach1. Global Production and Consumption. Sci. Total Environ. 290, 181–198. doi:10.1016/s0048-9697(01)01075-0
Chen, M.-Y., Luo, X.-J., Zhang, X.-L., He, M.-J., Chen, S.-J., and Mai, B.-X. (2011). Chlorinated Paraffins in Sediments from the Pearl River Delta, South China: Spatial and Temporal Distributions and Implication for Processes. Environ. Sci. Technol. 45 (23), 9936–9943. doi:10.1021/es202891a
Combi, T., Miserocchi, S., Langone, L., and Guerra, R. (2016). Polychlorinated Biphenyls (PCBs) in Sediments from the Western Adriatic Sea: Sources, Historical Trends and Inventories. Sci. Total Environ. 562, 580–587. doi:10.1016/j.scitotenv.2016.04.086
Da, C., Wang, R., Ye, J., and Yang, S. (2019). Sediment Records of Polybrominated Diphenyl Ethers (PBDEs) in Huaihe River, China: Implications for Historical Production and Household Usage of PBDE-Containing Products. Environ. Pollut. 254, 112955. doi:10.1016/j.envpol.2019.07.123
Dendievel, A.-M., Mourier, B., Coynel, A., Evrard, O., Labadie, P., Ayrault, S., et al. (2020). Spatio-Temporal Assessment of the Polychlorinated Biphenyl (PCB) Sediment Contamination in Four Major French River Corridors (1945-2018). Earth Syst. Sci. Data 12 (2), 1153–1170. doi:10.5194/essd-12-1153-2020
Desmet, M., Mourier, B., Mahler, B. J., Van Metre, P. C., Roux, G., Persat, H., et al. (2012). Spatial and Temporal Trends in PCBs in Sediment Along the Lower Rhône River, France. Sci. Total Environ. 433, 189–197. doi:10.1016/j.scitotenv.2012.06.044
Drage, D., Mueller, J. F., Birch, G., Eaglesham, G., Hearn, L. K., and Harrad, S. (2015). Historical Trends of PBDEs and HBCDs in Sediment Cores from Sydney Estuary, Australia. Sci. Total Environ. 512-513, 177–184. doi:10.1016/j.scitotenv.2015.01.034
Gioia, R., Eckhardt, S., Breivik, K., Jaward, F. M., Prieto, A., Nizzetto, L., et al. (2011). Evidence for Major Emissions of PCBs in the West African Region. Environ. Sci. Technol. 45 (4), 1349–1355. doi:10.1021/es1025239
Giuliani, S., Piazza, R., El Moumni, B., Polo, F. P., Vecchiato, M., Romano, S., et al. (2015). Recognizing Different Impacts of Human and Natural Sources on the Spatial Distribution and Temporal Trends of PAHs and PCBs (Including PCB-11) in Sediments of the Nador Lagoon (Morocco). Sci. Total Environ. 526, 346–357. doi:10.1016/j.scitotenv.2015.04.057
Glüge, J., Wang, Z., Bogdal, C., Scheringer, M., and Hungerbühler, K. (2016). Global Production, Use, and Emission Volumes of Short-Chain Chlorinated Paraffins - A Minimum Scenario. Sci. Total Environ. 573, 1132–1146. doi:10.1016/j.scitotenv.2016.08.105
Guo, J., Li, Z., Ranasinghe, P., Rockne, K. J., Sturchio, N. C., Giesy, J. P., et al. (2020). Halogenated Flame Retardants in Sediments from the Upper Laurentian Great Lakes: Implications to Long-Range Transport and Evidence of Long-Term Transformation. J. Hazard. Mater. 384, 121346. doi:10.1016/j.jhazmat.2019.121346
Hansen, K. M., Fauser, P., Vorkamp, K., and Christensen, J. H. (2020). Global Emissions of Dechlorane Plus. Sci. Total Environ. 742, 140677. doi:10.1016/j.scitotenv.2020.140677
He, M.-J., Luo, X.-J., Wu, J.-P., Chen, S.-J., Wei, S.-Q., and Mai, B.-X. (2014). Isomers of Dechlorane Plus in an Aquatic Environment in a Highly Industrialized Area in Southern China: Spatial and Vertical Distribution, Phase Partition, and Bioaccumulation. Sci. Total Environ. 481, 1–6. doi:10.1016/j.scitotenv.2014.02.025
Heim, S., and Schwarzbauer, J. (2013). Pollution History Revealed by Sedimentary Records: A Review. Environ. Chem. Lett. 11 (3), 255–270. doi:10.1007/s10311-013-0409-3
Hoang, A. Q., Aono, D., Watanabe, I., Kuwae, M., Kunisue, T., and Takahashi, S. (2021). Contamination Levels and Temporal Trends of Legacy and Current-Use Brominated Flame Retardants in a Dated Sediment Core from Beppu Bay, Southwestern Japan. Chemosphere 266, 129180. doi:10.1016/j.chemosphere.2020.129180
Hoh, E., Zhu, L., and Hites, R. A. (2006). Dechlorane Plus, a Chlorinated Flame Retardant, in the Great Lakes. Environ. Sci. Technol. 40 (4), 1184–1189. doi:10.1021/es051911h
Hoh, E., Zhu, L., and Hites, R. A. (2005). Novel Flame Retardants, 1,2-Bis(2,4,6-Tribromophenoxy)ethane and 2,3,4,5,6-Pentabromoethylbenzene, in United States' Environmental Samples. Environ. Sci. Technol. 39 (8), 2472–2477. doi:10.1021/es048508f
Hoogsteen, M. J. J., Lantinga, E. A., Bakker, E. J., Groot, J. C. J., and Tittonell, P. A. (2015). Estimating Soil Organic Carbon Through Loss on Ignition: Effects of Ignition Conditions and Structural Water Loss. Eur. J. Soil Sci. 66 (2), 320–328. doi:10.1111/ejss.12224
Hoornweg, D., and Bhada-Tata, P. (2012). What a Waste: A Global Review of Solid Waste Management. Urban Development Series Knowledge Papers2012, No. 15. Washington, D.C.: World Bank.
Hung, H., Katsoyiannis, A. A., Brorström-Lundén, E., Olafsdottir, K., Aas, W., Breivik, K., et al. (2016). Temporal Trends of Persistent Organic Pollutants (POPs) in Arctic Air: 20 Years of Monitoring under the Arctic Monitoring and Assessment Programme (AMAP). Environ. Pollut. 217, 52–61. doi:10.1016/j.envpol.2016.01.079
Igulu, B., and Mshiu, E. (2020). The Impact of an Urbanizing Tropical Watershed to the Surface-Runoff. Glob. J. Environ. Sci. Manage. 6 (2), 245–260. doi:10.22034/gjesm.2020.02.09
Iozza, S., Müller, C. E., Schmid, P., Bogdal, C., and Oehme, M. (2008). Historical Profiles of Chlorinated Paraffins and Polychlorinated Biphenyls in a Dated Sediment Core from Lake Thun (Switzerland). Environ. Sci. Technol. 42 (4), 1045–1050. doi:10.1021/es702383t
IPEN (2005). Hotspot Report for Pattern of Practices: PCBs Sources and Releases in Tanzania. Dar es Salaam: The International POPs Elimination Project.
Kim, K. H., and Burnett, W. C. (1986). Uranium-Series Growth History of a Quaternary Phosphatic Crust from the Peruvian continental Margin. Chem. Geology. Isotope Geosci. section 58 (3), 227–244. doi:10.1016/0168-9622(86)90012-6
Klánová, J., Čupr, P., Holoubek, I., Borůvková, J., Přibylová, P., Kareš, R., et al. (2009). Monitoring of Persistent Organic Pollutants in Africa. Part 1: Passive Air Sampling Across the Continent in 2008. J. Environ. Monit. 11 (11), 1952–1963. doi:10.1039/b913415h
Korosi, J. B., Cheng, W., and Blais, J. M. (2015). “Organic Pollutants in Sediment Core Archives,” in Environmental Contaminants. Editors J. Blais, M. Rosen, and J Smol (Dordrecht: Springer), 161–185. doi:10.1007/978-94-017-9541-8_8
Kwan, C. S., Takada, H., Boonyatumanond, R., Kato, Y., Mizukawa, K., Ito, M., et al. (2014). Historical Occurrences of Polybrominated Diphenyl Ethers and Polychlorinated Biphenyls in Manila Bay, Philippines, and in the Upper Gulf of Thailand. Sci. total Environ. 470-471, 427–437. doi:10.1016/j.scitotenv.2013.09.076
Li, Y., Lin, T., Hu, L., Feng, J., and Guo, Z. (2016). Time Trends of Polybrominated Diphenyl Ethers in East China Seas: Response to the Booming of PBDE Pollution Industry in China. Environ. Int. 92-93, 507–514. doi:10.1016/j.envint.2016.04.033
Liu, H.-H., Hu, Y.-J., Luo, P., Bao, L.-J., Qiu, J.-W., Leung, K. M. Y., et al. (2014). Occurrence of Halogenated Flame Retardants in Sediment off an Urbanized Coastal Zone: Association with Urbanization and Industrialization. Environ. Sci. Technol. 48 (15), 8465–8473. doi:10.1021/es500660z
Lu, Q., Futter, M. N., Nizzetto, L., Bussi, G., Jürgens, M. D., and Whitehead, P. G. (2016). Fate and Transport of Polychlorinated Biphenyls (PCBs) in the River Thames Catchment - Insights from a Coupled Multimedia Fate and Hydrobiogeochemical Transport Model. Sci. Total Environ. 572, 1461–1470. doi:10.1016/j.scitotenv.2016.03.029
Lunder Halvorsen, H., Bohlin-Nizzetto, P., Eckhardt, S., Gusev, A., Krogseth, I. S., Moeckel, C., et al. (2021). Main Sources Controlling Atmospheric Burdens of Persistent Organic Pollutants on a National Scale. Ecotoxicology Environ. Saf. 217, 112172. doi:10.1016/j.ecoenv.2021.112172
Mahenge, R. S., Ulanga, P., Malabeja, M., Pilly, J., and Massoy, W. (2018). Performance Audit Report on Electronic Waste Management. Available at: https://www.nao.go.tz/uploads/ELECTRONIC-WASTE-MANAGEMENT-2018.pdf. (Accessed March, 2021).
Marvin, C. H., Painter, S., Tomy, G. T., Stern, G. A., Braekevelt, E., and Muir, D. C. G. (2003). Spatial and Temporal Trends in Short-Chain Chlorinated Paraffins in Lake Ontario Sediments. Environ. Sci. Technol. 37 (20), 4561–4568. doi:10.1021/es0345064
NBS (2019). in National E-Waste Statistics Report (Dodoma, Tanzania, Bonn, Germany: V.S. National Bureau of Statistics and United Nations University. National Bureau StatisticsUnited Nations University, ViE – SCYCLE).
Nipen, M., Vogt, R. D., Bohlin-Nizzetto, P., Borgå, K., Mwakalapa, E. B., Borgen, A. R., et al. (2021). Spatial Trends of Chlorinated Paraffins and Dechloranes in Air and Soil in a Tropical Urban, Suburban, and Rural Environment. Environ. Pollut. 292, 118298. doi:10.1016/j.envpol.2021.118298
Ontiveros-Cuadras, J. F., Ruiz-Fernández, A. C., Sanchez-Cabeza, J.-A., Sericano, J., Pérez-Bernal, L. H., Páez-Osuna, F., et al. (2019). Recent History of Persistent Organic Pollutants (PAHs, PCBs, PBDEs) in Sediments from a Large Tropical lake. J. Hazard. Mater. 368, 264–273. doi:10.1016/j.jhazmat.2018.11.010
Qiu, X., Marvin, C. H., and Hites, R. A. (2007). Dechlorane Plus and Other Flame Retardants in a Sediment Core from Lake Ontario. Environ. Sci. Technol. 41 (17), 6014–6019. doi:10.1021/es070810b
Schuster, J. K., Gioia, R., Breivik, K., Steinnes, E., Scheringer, M., and Jones, K. C. (2010). Trends in European Background Air Reflect Reductions in Primary Emissions of PCBs and PBDEs. Environ. Sci. Technol. 44 (17), 6760–6766. doi:10.1021/es101009x
Shen, L., Reiner, E. J., Helm, P. A., Marvin, C. H., Hill, B., Zhang, X., et al. (2011). Historic Trends of Dechloranes 602, 603, 604, Dechlorane Plus and Other Norbornene Derivatives and Their Bioaccumulation Potential in Lake Ontario. Environ. Sci. Technol. 45 (8), 3333–3340. doi:10.1021/es104328r
Shen, L., Reiner, E. J., MacPherson, K. A., Kolic, T. M., Helm, P. A., Richman, L. A., et al. (2010a). Dechloranes 602, 603, 604, Dechlorane Plus, and Chlordene Plus, a Newly Detected Analogue, in Tributary Sediments of the Laurentian Great Lakes. Environ. Sci. Technol. 45 (2), 693–699. doi:10.1021/es1027844
Shen, L., Reiner, E. J., MacPherson, K. A., Kolic, T. M., Sverko, E., Helm, P. A., et al. (2010b). Identification and Screening Analysis of Halogenated Norbornene Flame Retardants in the Laurentian Great Lakes: Dechloranes 602, 603, and 604. Environ. Sci. Technol. 44 (2), 760–766. doi:10.1021/es902482b
Song, W., Ford, J. C., Li, A., Mills, W. J., Buckley, D. R., and Rockne, K. J. (2004). Polybrominated Diphenyl Ethers in the Sediments of the Great Lakes. 1. Lake Superior. Environ. Sci. Technol. 38 (12), 3286–3293. doi:10.1021/es035297q
Sun, Y.-X., Zhang, Z.-W., Xu, X.-R., Hao, Q.-W., Hu, Y.-X., Zheng, X.-B., et al. (2016). Spatial and Vertical Distribution of Dechlorane Plus in Mangrove Sediments of the Pearl River Estuary, South China. Arch. Environ. Contam. Toxicol. 71 (3), 359–364. doi:10.1007/s00244-016-0296-2
Sverko, E., Tomy, G. T., Marvin, C. H., Zaruk, D., Reiner, E., Helm, P. A., et al. (2008). Dechlorane Plus Levels in Sediment of the Lower Great Lakes. Environ. Sci. Technol. 42 (2), 361–366. doi:10.1021/es0710104
Takahashi, S., Anh, H. Q., Watanabe, I., Aono, D., Kuwae, M., and Kunisue, T. (2020). Characterization of Mono- to Deca-Chlorinated Biphenyls in a Well-Preserved Sediment Core from Beppu Bay, Southwestern Japan: Historical Profiles, Emission Sources, and Inventory. Sci. Total Environ. 743, 140767. doi:10.1016/j.scitotenv.2020.140767
Tham, T. T., Anh, H. Q., Phuong, B. T., Trinh, L. T., Thuy, N. T. T., Yen, N. T. H., et al. (2020). Contamination Status and Temporal Trends of Persistent Toxic Substances in Sediment Cores from Coastal Areas of central Vietnam. Mar. Pollut. Bull. 156, 111222. doi:10.1016/j.marpolbul.2020.111222
Tiwari, M., Sahu, S. K., Bhangare, R. C., Ajmal, P. Y., and Pandit, G. G. (2018). Polybrominated Diphenyl Ethers (PBDEs) in Core Sediments from Creek Ecosystem: Occurrence, Geochronology, and Source Contribution. Environ. Geochem. Health 40 (6), 2587–2601. doi:10.1007/s10653-018-0125-9
TMA (2021). Rainfall Anomalies Between 1983 and 2018. Available at: http://maproom.meteo.go.tz/maproom/ (Accessed September 2021).
UNEP (2021). Global Monitoring Plan for Persistent Organic Pollutants: Third Regional Monitoring Report - Africa Region. Available at: http://chm.pops.int/Implementation/GlobalMonitoringPlan/MonitoringReports/tabid/525/ (Accessed October 29, 2021).
UNEP (2017). Stockholm Convention on Persistent Organic Pollutants (POPs). Available at: www.pops.int/TheConvention/Overview/TextoftheConvention/tabid/2232/Default.aspx: UNEP (Accessed September 02, 2021).
Van Metre, P. C., and Mahler, B. J. (2004). Contaminant Trends in Reservoir Sediment Cores as Records of Influent Stream Quality. Environ. Sci. Technol. 38 (11), 2978–2986. doi:10.1021/es049859x
Vauclin, S., Mourier, B., Dendievel, A.-M., Marchand, P., Vénisseau, A., Morereau, A., et al. (2021). Temporal Trends of Legacy and Novel Brominated Flame Retardants in Sediments along the Rhône River Corridor in France. Chemosphere 271, 129889. doi:10.1016/j.chemosphere.2021.129889
Voogt, P., and Brinkman, U. (1989). Production, Properties and Usage of Polychlorinated Biphenyls. Halogenated biphenyls, terphenyls, naphthalenes, dibenzodioxins Relat. Prod. (2nd edn) 3–45. doi:10.1016/b978-0-444-81029-8.50005-9
Wang, G., Peng, J., Hao, T., Feng, L., Liu, Q., and Li, X. (2017). Effects of Terrestrial and Marine Organic Matters on Deposition of Dechlorane Plus (DP) in Marine Sediments from the Southern Yellow Sea, China: Evidence from Multiple Biomarkers. Environ. Pollut. 230, 153–162. doi:10.1016/j.envpol.2017.06.061
Wang, G., Peng, J., Hao, T., Liu, Y., Zhang, D., and Li, X. (2016). Distribution and Region-specific Sources of Dechlorane Plus in Marine Sediments from the Coastal East China Sea. Sci. Total Environ. 573, 389–396. doi:10.1016/j.scitotenv.2016.08.090
Wong, F., Hung, H., Dryfhout-Clark, H., Aas, W., Bohlin-Nizzetto, P., Breivik, K., et al. (2021). Time Trends of Persistent Organic Pollutants (POPs) and Chemicals of Emerging Arctic Concern (CEAC) in Arctic Air from 25 Years of Monitoring. Sci. Total Environ. 775, 145109. doi:10.1016/j.scitotenv.2021.145109
WorldBank (2021). The World Bank in Tanzania [Online]. Available at: http://www.worldbank.org/en/country/tanzania/overview (Accessed January 2021).
Wu, F., Guo, J., Chang, H., Liao, H., Zhao, X., Mai, B., et al. (2012). Polybrominated Diphenyl Ethers and Decabromodiphenylethane in Sediments from Twelve Lakes in China. Environ. Pollut. 162, 262–268. doi:10.1016/j.envpol.2011.11.014
Xiong, P., Yan, X., Zhu, Q., Qu, G., Shi, J., Liao, C., et al. (2019). A Review of Environmental Occurrence, Fate, and Toxicity of Novel Brominated Flame Retardants. Environ. Sci. Technol. 53 (23), 13551–13569. doi:10.1021/acs.est.9b03159
Yang, C., Rose, N. L., Turner, S. D., Yang, H., Goldsmith, B., Losada, S., et al. (2016). Hexabromocyclododecanes, Polybrominated Diphenyl Ethers, and Polychlorinated Biphenyls in Radiometrically Dated Sediment Cores from English Lakes, ∼ 1950-present. Sci. Total Environ. 541, 721–728. doi:10.1016/j.scitotenv.2015.09.102
Yang, H., Zhuo, S., Xue, B., Zhang, C., and Liu, W. (2012a). Distribution, Historical Trends and Inventories of Polychlorinated Biphenyls in Sediments from Yangtze River Estuary and Adjacent East China Sea. Environ. Pollut. 169, 20–26. doi:10.1016/j.envpol.2012.05.003
Yang, R., Wei, H., Guo, J., and Li, A. (2012b). Emerging Brominated Flame Retardants in the Sediment of the Great Lakes. Environ. Sci. Technol. 46 (6), 3119–3126. doi:10.1021/es204141p
Yang, R., Wei, H., Guo, J., McLeod, C., Li, A., and Sturchio, N. C. (2011). Historically and Currently Used Dechloranes in the Sediments of the Great Lakes. Environ. Sci. Technol. 45 (12), 5156–5163. doi:10.1021/es201019m
Yhdego, M. (2017). From a Sanitary Landfill to a Dump Site: Pugu Kinyamwezi Community Curse in Dar Es Salaam, Tanzania. Dar es Salaam, Tanzania: ERC-Environmental Resources Consultancy. doi:10.13140/RG.2.2.27828.04483
Yuan, B., Brüchert, V., Sobek, A., and de Wit, C. A. (2017). Temporal Trends of C8-C36 Chlorinated Paraffins in Swedish Coastal Sediment Cores Over the Past 80 Years. Environ. Sci. Technol. 51 (24), 14199–14208. doi:10.1021/acs.est.7b04523
Zegers, B. N., Lewis, W. E., Booij, K., Smittenberg, R. H., Boer, W., De Boer, J., et al. (2003). Levels of Polybrominated Diphenyl Ether Flame Retardants in Sediment Cores from Western Europe. Environ. Sci. Technol. 37 (17), 3803–3807. doi:10.1021/es034226o
Zeng, L., Chen, R., Zhao, Z., Wang, T., Gao, Y., Li, A., et al. (2013). Spatial Distributions and Deposition Chronology of Short Chain Chlorinated Paraffins in Marine Sediments Across the Chinese Bohai and Yellow Seas. Environ. Sci. Technol. 47 (20), 11449–11456. doi:10.1021/es402950q
Zeng, L., Lam, J. C. W., Horii, Y., Li, X., Chen, W., Qiu, J.-W., et al. (2017). Spatial and Temporal Trends of Short- and Medium-Chain Chlorinated Paraffins in Sediments off the Urbanized Coastal Zones in China and Japan: A Comparison Study. Environ. Pollut. 224, 357–367. doi:10.1016/j.envpol.2017.02.015
Zeng, L., Wang, T., Wang, P., Liu, Q., Han, S., Yuan, B., et al. (2011). Distribution and Trophic Transfer of Short-Chain Chlorinated Paraffins in an Aquatic Ecosystem Receiving Effluents from a Sewage Treatment Plant. Environ. Sci. Technol. 45 (13), 5529–5535. doi:10.1021/es200895b
Zeng, L., Zhao, Z., Li, H., Wang, T., Liu, Q., Xiao, K., et al. (2012). Distribution of Short Chain Chlorinated Paraffins in Marine Sediments of the East China Sea: Influencing Factors, Transport and Implications. Environ. Sci. Technol. 46 (18), 9898–9906. doi:10.1021/es302463h
Zennegg, M., Kohler, M., Hartmann, P. C., Sturm, M., Gujer, E., Schmid, P., et al. (2007). The Historical Record of PCB and PCDD/F Deposition at Greifensee, A Lake of the Swiss Plateau, Between 1848 and 1999. Chemosphere 67 (9), 1754–1761. doi:10.1016/j.chemosphere.2006.05.115
Zhang, C., Chang, H., Wang, H., Zhu, Y., Zhao, X., He, Y., et al. (2019). Spatial and Temporal Distributions of Short-, Medium-, and Long-Chain Chlorinated Paraffins in Sediment Cores from Nine Lakes in China. Environ. Sci. Technol. 53 (16), 9462–9471. doi:10.1021/acs.est.8b07296
Zhang, X.-L., Luo, X.-J., Chen, S.-J., Wu, J.-P., and Mai, B.-X. (2009). Spatial Distribution and Vertical Profile of Polybrominated Diphenyl Ethers, Tetrabromobisphenol A, and Decabromodiphenylethane in River Sediment from an Industrialized Region of South China. Environ. Pollut. 157 (6), 1917–1923. doi:10.1016/j.envpol.2009.01.016
Zhang, Z.-W., Sun, Y.-X., Sun, K.-F., Xu, X.-R., Yu, S., Zheng, T.-L., et al. (2015). Brominated Flame Retardants in Mangrove Sediments of the Pearl River Estuary, South China: Spatial Distribution, Temporal Trend and Mass Inventory. Chemosphere 123, 26–32. doi:10.1016/j.chemosphere.2014.11.042
Keywords: temporal trends, urban environment, PCBs, PBDEs, flame retardants, dechloranes, chlorinated paraffins
Citation: Nipen M, Vogt RD, Bohlin-Nizzetto P, Borgå K, Mwakalapa EB, Borgen AR, Schlabach M, Christensen G, Mmochi AJ and Breivik K (2022) Increasing Trends of Legacy and Emerging Organic Contaminants in a Dated Sediment Core From East-Africa. Front. Environ. Sci. 9:805544. doi: 10.3389/fenvs.2021.805544
Received: 30 October 2021; Accepted: 20 December 2021;
Published: 07 January 2022.
Edited by:
Jabir Hussain Syed, COMSATS University Islamabad Pakistan, PakistanReviewed by:
Begoña Jiménez, Spanish National Research Council (CSIC), SpainQinghui Huang, Tongji University, China
Copyright © 2022 Nipen, Vogt, Bohlin-Nizzetto, Borgå, Mwakalapa, Borgen, Schlabach, Christensen, Mmochi and Breivik. This is an open-access article distributed under the terms of the Creative Commons Attribution License (CC BY). The use, distribution or reproduction in other forums is permitted, provided the original author(s) and the copyright owner(s) are credited and that the original publication in this journal is cited, in accordance with accepted academic practice. No use, distribution or reproduction is permitted which does not comply with these terms.
*Correspondence: Maja Nipen, bWFqYW5pcEBramVtaS51aW8ubm8=, bW5pQG5pbHUubm8=