- 1Tropical Research and Education Center, IFAS, University of Florida, Homestead, FL, United States
- 2Coral Reef Senior High School, Miami, FL, United States
Many soils including urban soils have high legacy soil phosphorus (P) due to repeated applications of P fertilizers, but a large portion legacy soil P is fixed by calcium in the calcareous soils. Phosphorus solubilizing bacteria (PSB) have the ability to transfer fixed (non-labile) soil P into bioavailable P. The aim of this study was to isolate P solubilizing bacteria from the rhizospheres of four local native plants [broomsedge bluestem (Andropogon virginicus), giant sword ferns (Nephrolepis biserrata), sawgrass (Cladium jamaicense), and sea ox-eye daisy (Borrichia frutescens)] grown in low bioavailable P calcareous soils and to determine their ability to solubilize P. A total of 44 strains of PSB were isolated with 15 of them being identified by sequencing the 16S rRNA genes as Bacillus flexus, Beijerinckia fluminensis, Enterobacter ludwigii, Enterobacter sp., and Pantoea cypripedii. After a 7-day incubation, these strains reduced pH to <4.27 and increased water-soluble P up to 588 mg L−1. Enterobacter ludwigii showed superior P solubilizing ability amount PSB isolated. Therefore, the isolated strains from the local native environment have the potential to thrive in local calcareous soils and possess strong ability to transform non-labile P into bioavailable forms for plants to uptake.
Introduction
Global food security depends on the availability of phosphorus (P) because it is a nonrenewable mineral resource and an essential nutrient for plants. Total reserves of high-grade phosphate rock are estimated to be about 67,000 metric tons and about 82% of annual P production (160 MT) worldwide are used as fertilizers (USGS, 2014). Phosphorus along with nitrogen (N) and potassium (K) are primary nutrients, also known as a macronutrients which are required in the largest amounts by plants. Phosphorus is essential for plant reproduction and is required for critical plant functions (e.g., nutrient transport, photosynthesis, respiration). In order to maintain high productivity of crops, inorganic and organic P fertilizers are applied at rates as high as 300 kg P2O5 ha−1 for commercial crops and even higher rates for residential landscape plants. The P use efficiency of crops from fertilizer application is estimated to be as low as 16% and crops grown in calcareous soils may have an even lower rate because applied P can be precipitated rapidly by calcium in calcareous soils (Dhillon et al., 2017). A majority of P applied as fertilizers is retained in soils and a small fraction of soil P enters groundwater by leaching, or surface water by runoff, which cause eutrophication of nearby water bodies. The annual application of P fertilizers beyond the uptake of plants results to the build-up of legacy soil P. A study showed that soil with bluegrass sod accumulated 1,291 kg P ha−1 after being fertilized for 45 years (Zhang et al., 2020). A soil sample (top 20 cm) collected from a vegetable farm in Miami-Dade County had 4,055 mg kg−1 P (equivalent to ∼8,000 kg ha−1) which is over ten-fold higher than native uncultivated soils in the area (Zhang et al., 2014).
Reducing use of inorganic fertilizers is the most effective approach to prevent P from entering water bodies. The recommendations of low application rates, new types of fertilizers (e.g., controlled release), and new application methods (e.g., fertigation) have been included in the best management practices (BMPs) for various commercial crops in many regions of the world (Grenon et al., 2021). In order to reduce P use for urban landscaping plants, many local governments in the U.S. passed local fertilizer ordinances to ban the use of either P containing fertilizers or any type of fertilizers during summer seasons. However, plants grow faster and require more nutrients, including P, due to increasing solar radiance, temperature, and rainfall. Even though many soils including urban soils have high legacy soil P, almost all legacy soil P is fixed by calcium in calcareous soils and iron/aluminum in acid soils and not available for plants (Van Zeghbroeck et al., 2021). Thus, it is necessary to transform legacy soil P from fixed forms into bioavailable forms for plants to use without applying additional inorganic P fertilizers.
Phosphorus solubilizing bacteria (PSB) are able to transfer non-labile soil P into plant available P by reducing pH (solubilization of precipitated P), chelating (prevention of P precipitation by cations), and mineralization (organic P with enzymes) (Mendoza-Arroyo et al., 2020). Slight changes of soil pH can significantly improve bioavailability of soil P in calcareous soils. However, a few studies have been conducted for isolating PSB from high pH soils, especially from natural areas with extreme low soil P. Our preliminary tests showed that two PSBs isolated from other regions did not survive in the extremely high calcium carbonate and high pH soils in South Florida. Therefore, our purpose of this study was to 1) isolate PSB strains from rhizosphere soil of four native plants grown in calcareous soils, 2) assess capabilities of P solubilization of these strains, and 3) identify these strains by sequence analysis of 16S rRNA genes.
Materials and Methods
Soil Sampling
Four samples of soils from uplands and wetlands were collected from the rhizospheres (∼1 mm thin layer of soil around plant roots) of 1) broomsedge bluestem (Andropogon virginicus) in uncultivated pineland, South Dade Agricultural area, Miami-Dade County, FL, 2) sawgrass (Cladium jamaicense) in a native area adjacent to Chekika Visitor Center, Everglades National Park, FL, 3) sword fern (Nephrolepis biserrata) in a natural area, Palmetto Bay, FL, and 4) sea ox-eye daisy (Borrichia frutescens) 20 m from seawater in the Deering Estate North Addition Preserve, Miami, FL. These native plants (naturally occurring in the area) grew in natural areas (never fertilized) with low bioavailable P and high pH calcareous soils. Therefore, the rhizospheres of these plants provided a condition for P solubilizing bacteria to transform non-labile P in soils into labile P available for plant uptake. For sampling, plants were slowly pulled out of the ground and soil loosely adhering to the roots were gently shaken off. Roots and attached soil were collected and stored at 4°C prior to processing for microbial isolation. Soils under bluestem and giant sword ferns are classified as Rockdale fine sandy loam in a1958 USDA soil survey and rock-plowed (the process to crush the oolitic limestone bedrock with a scarifying plow front-mounted on a large bulldozer) Rockdale soil was reclassified as Krome very gravelly loam (loamy-skeletal, carbonatic, hyper-thermic Lithic Udorthents) in a 1996 USDA soil survey. Soils under sawgrass and sea ox-eye daisy are Biscayne marl soil (Loamy, carbonatic, isohyperthermic, shallow Typic Fluvaquents) (USDA, 1996).
Analyses of soil samples followed the procedures by Hanlon et al. (1997). The soil moistures were measured by drying subsamples in an oven at 105°C. The second groups of subsamples were air-dried, ground, sieved (<2 mm), and stored for other analyses. Air-dried samples were then measured for pH and EC using a pH/EC meter (AR60, Fisher, Fisher Scientific, Fairlawn, NJ). Inorganic carbon was determined using a pressure-calcimeter. Soil organic matter (SOM) was determined by loss after ignition (550°C, 4 h). Ash from SOM measurement was digested in 6 M HCl for determining total P. Air-dried soils were extracted with the Mehlich-3 solution to determine plant available P. Both HCl and Mehlich-3 extracts were analyzed for P concentrations using the ascorbic acid method with a spectrophotometer (Beckman Instruments, Inc., Fullerton, CA). Total nitrogen (TN) was measured by dry combustion using an elemental combustion system (CN628, LECO, Saint Joseph, MI).
Bacterial Isolation and Screening
The PSB screenings were performed with the National Botanical Research Institute’s Phosphate (NBRIP) growth medium based on the modified method described by Liu et al. (2015). The NBRIP- bromophenol blue (BPB) agar was prepared with D-glucose (10 g L−1), ammonium sulfate [(NH4)2SO4, 0.1 g L−1], calcium phosphate [Ca3(PO4)2, 5 g L−1], magnesium chloride (MgCl2.6H2O, 5 g L−1], magnesium sulfate (MgSO4.7H2O, 0.25 g L−1), and potassium chloride (KCl, 0.2 g L−1). The pH indicator, bromophenol blue (BPB, 0.025 g L−1) was also added. The pH of the agar was adjusted to 7.2 before being autoclaved. All chemicals used for this experiment were ACS grade.
Two grams of each moist soil were placed in a 50-ml centrifuge tube. Sterile deionized water (30 ml) was added into the tube and the mixture was placed shaken on a vortex mixer for 2 minutes. The upper turbid phase was serially diluted (10−2–10−8), plated on NBRIP agar, and incubated at 25 ± 2°C. After a 3-days incubation, bacteria were evaluated for presence of halos around each colony. Halo zones were formed due to the color change of BPB in the medium. The acid-base indicator BPB changed from blue (pH > 4.6) to yellow (pH < 3). 44 strains that developed clear halo zones around their colonies were transferred to nutrient agar plates and stored in a walk-in cooler at −4°C before further analysis. Based on solubilization index (SI) values, 14 out of 44 strains were selected for testing capability of solubilizing P and for sequencing identification (Table S1). Two strains (SF-6b and SF-6w) were also selected for further evaluation even though they did not create halo zones but grew well on P-limited NBRIP-BPB agar medium.
Assessment of P Solubilizing Capability With NBRIP Plate Culture
Selected bacterial strains from stored cultures on the nutrient agar dish were transferred into 15 ml-centrifuge tubes containing 8 ml of nutrient broth. After incubation at 28°C and shaking at 140 rpm for 24 h, 4 ml of the solution was transferred into a cuvette. The optical density at 600 nm (OD600) of bacterial strains were measured using a spectrophotometer (SQ-4802, UNICO, Dayton, NJ). Each of the bacterial solutions were diluted to 0.5 OD600 and 1 ml of each of the diluted solutions was added into a 2 ml vial to begin the steps for the bacterial wash. The vial was then centrifuged for 1 min at 13,000 rpm, the excess liquid was discarded, and 1 ml of sterile distilled water was added into the vial. Each vial was then mixed well and the washing steps were repeated once.
After washing, the bacteria were inoculated by transferring 2 µL of the suspension [1–2 x 107 colony-forming units (CFU) mL−1] on the NBRIP-BPB plates. Each bacterial strain was plated on four plates as replicates. After 3 days, the diameters of both the colony and halo zone around the colony were measured using a digital caliper (CO 030300, Marathon, New Westminster, Canada). The brightness of each halo zone was also evaluated on a scale of 1–5, where 1 = very poor and 5 = excellent. The P solubilizing ability of each selected PSB was assessed using the solubilization index (SI). The SI was calculated using the following formula:
Assessment of P Solubilizing Capability With NBRIP Liquid Culture
Similar procedures for the plate cultures were used before adding bacterial strains into liquid media. Briefly, selected strain was inoculated into a 50-ml tube containing 35 ml of liquid NBRIP medium to achieve a final population of 1–2 x 107 CFU ml−1 and then incubated at 28°C with shaking at 150 rpm. Each strain was replicated three times. Five mL of suspensions were collected at 1, 3, 5, and 7 days during the incubation, filtered and analyzed for pH and water-soluble P (orthophosphate) using the same analytical methods described above for soil samples.
Bacterial 16S rRNA Gene Amplification and Sequencing
Bacterial strains were grown in 20 ml nutrient broth overnight at 28°C under continuous shaking at 120 rpm. Bacterial cells were collected by centrifuge and resuspended in 3 ml sterile water. Bacterial genomic DNA was isolated with the Wizard® Genomic DNA purification kit (Promega, Madison, WI) according to the manufacturer’s instructions. Genomic DNA samples were stored at −20°C. The 16S rRNA gene was amplified with universal primers 8F (5′AGAGTTTGATCCTGGCTCAG3′) and 1492R (5′GGTTACCTTGTTACGACTT3′). Each PCR reaction of 50 µl consisted of 5 µl buffer (10 × ), 1 µl dNTP (10 µM), 2.5 µl forward and reverse primers (10 µM), 0.2 µl Taq polymerase (5 unit µl−1), 1 µL genomic DNA, and 37.8 µl water. Water was added into the PCR reaction instead of genomic DNA as a negative control. PCR reaction components included an initial denaturation at 98°C for 30 s, one cycle; 35 cycles of 98°C for 10 s, 60°C for 30 s, and 72°C for 45 s; and a final extension at 72°C for 7 min. PCR products were visualized on 1% agarose gel after electrophoresis at 120 v for 30 min. A single PCR product of about 1,500 bp was amplified successfully from 15 out of 16 strains. The amplicons were isolated using QIAquick® PCR purification kit (Qiagen, Germantown, MD). The amplicons were sequenced at GeneWIZ from both ends (GeneWIZ, South Planfield, NJ). Sequence data were analyzed with BLAST and bacterial identity was inferred by comparing each sequence query to the available 16S rRNA gene sequences in GenBank with BLAST (NCBI, 2021). The top 10 matches were recorded as a potential identity for each sequence.
Results
Soils collected from the rhizospheres of four local native plants had different basic properties (Table 1). Soils from lowlands with vegetations of sawgrass and sea ox-eye daisy had high moistures (25–51%), high contents of calcium carbonate (20.56–44.44%), and high organic contents (2.27–4.72%). The highest salinity level (5,303 ug cm−1) was found in the rhizosphere soil of sea ox-eye daisy plants which are very close to saltwater. The rhizosphere of sword ferns had the lowest soil pH (5.5). All soilsfor this experiment had relatively low total P (230–360 mg kg−1) and very low Mechlich-3 extractable P (0.47–10.95 mg kg−1). The rockland soil of bluestem has the lowest total P and the soil of Everglade wetland has the lowest Mechlich-3 extractable P.
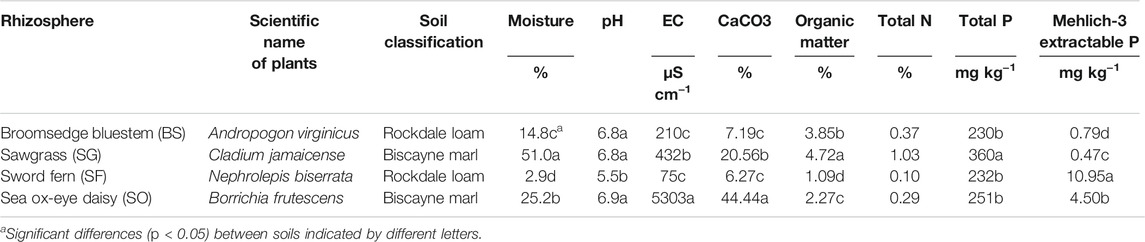
TABLE 1. Basic characteristics of soils collected from rhizospheres of native plants including bluestem (BS), sawgrass (SG), sword fern (SF), and sea ox-eye daisy (SO) in Miami-Dade County, Florida.
The testing of all soils resulted in a total of 44 strains which showed halo zones on NBRIP-BPB agar, and the numbers of these strains were different for four rhizosphere soils (Table 2). The soils with saline condition at sea ox-eye daisy site had the highest number of strains (17/plate) with halo zones, while the soil from the rhizosphere of bluestem at the rocky land site had the lowest number of strains (4/plate). The detected strains at the sword fern site had the largest colony diameters but the smallest halo zone and the lowest SI. On the other hand, strains isolated from sites of sawgrass and sea ox-eye daisy had relatively small colony diameter but had larger and brighter halo zones, and a higher SI.
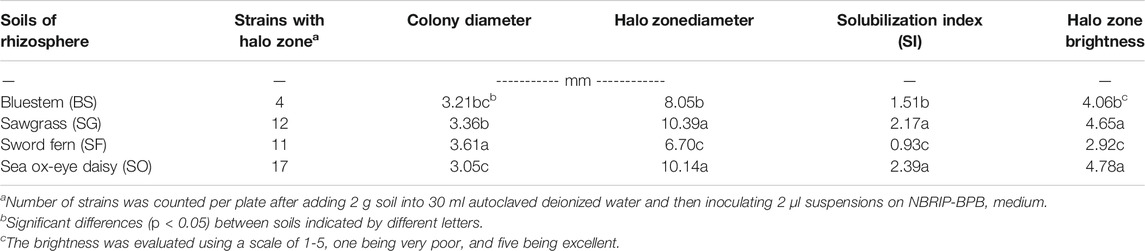
TABLE 2. Comparison of colony diameter, halo zone diameter, solubilization index (SI), and halo zone brightness for phosphorus solubilizing abilities of rhizosphere bacteria on NBRIP plates.
The 16 selected strains were processed for bacterial 16S rRNA gene amplification and sequencing (Table 3). The strain SO-10 was not identified because adequate DNA could not be obtained. Based on the best matches (90–99.6%) to sequences in the GenBank, five types of bacteria were identified. Three strains with halo zone formation on NBRIP agar medium were Enterobacter ludwigii from three sites (sawgrass, sword fern, and sea ox-eye daisy), Enterobacter sp. from the site of sea ox-eye daisy, and Pantoea cypripedii from the site of bluestem. Two strains without halo zone formation were identified as Bacillus flexus (the lowest match 90%) and Beijerinckia fluminensis from the rhizosphere of giant sword ferns.
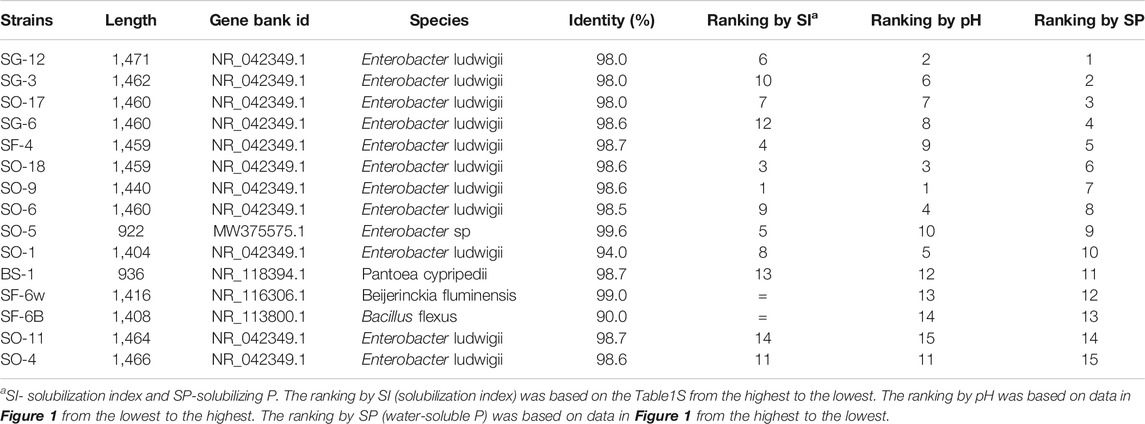
TABLE 3. Identification and rankings of selected PSB strains isolated from rhizospheres of native plants including bluestem (BS), sawgrass (SG), sword fern (SF), and sea ox-eye daisy (SO) in Miami-Dade County, Florida based on 16S rRNA gene sequence and GenBank with BLAST.
Phosphorus solubilizing capability of these strains were also tested in NBRIP liquid medium and the results were summarized based on types of bacteria (Figure 1). The pH values of the medium incubated with all strains changed dramatically just 1 day after inoculation and further decreased at a smaller rate during the rest of the incubation. Enterobacter ludwigii, Enterobacter sp., and Pantoea cypripedii had significantly greater pH reductions than Bacillus flexus and Beijerinckia fluminensis for the first day, and all of them had similar pH levels at day seven. Similarly, Enterobacter ludwigii, Enterobacter sp., and Pantoea cypripedii showed higher P solubilizing capabilities than the other two strains. Compared to the control, all strains demonstrated great potential for converting insoluble P to plant-available and water-soluble P. The best strain by ranking of water-soluble P was Enterobacter ludwigii from the site of sawgrass, while the best strain based on the ranking of solubilization index and changes of pH was also Enterobacter ludwigii but from the site of sea ox-eye daisy (Table 3). The amount of P solubilized by PSB was highly correlated to the reduction of solution pH with a high regression coefficient (R2 = 0.90) (Figure 1).
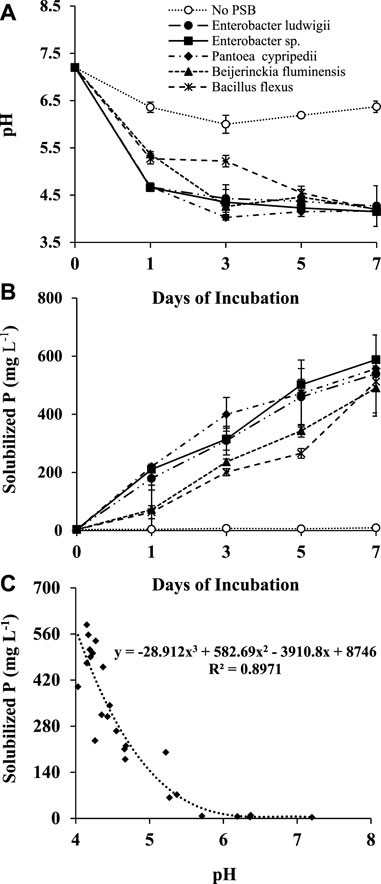
FIGURE 1. Changes of pH (A) and solubilized phosphorus (B) and relationship between them (C) in NBRIP liquid medium during a week-long incubation. The error bars indicate the standard errors of the means (n = 3).
Discussion
Phosphorus solubilizing bacteria (PSB) existed in all four low P soils and indicated that PSB play an important role in providing P to these native plants. Additionally, this research highlights the connection between local native plants and PSB suggesting that native plants play an important role in establishing and maintaining thriving PSB communities. More research needs to be conducted in the future in this area to better understand the relationship between these plants and the associated PSB. The strain Enterobacter ludwigii with the strongest P solubilizing capability was isolated from sawgrass rhizospheres which were collected from the Florida Everglades and had the lowest plant available P. The largest number of PSB were isolated from the rhizosphere of sea ox-eye daisy, which had the highest level of salinity. Our results agreed with the previous finding that the ideal condition for finding and isolating PSB was in soils with low bioavailable P, high salinity, and other stressful factors (Michas et al., 2021). The high correlation between the amount of P solubilized by PSB and the reduction of solution pH indicates that acidification of soils by PSB was the main mechanism for solubilizing P (Kalayu, 2019). Mendoza-Arroyo et al. (2020) reported that Enterobacter ludwigii reduced the solution pH to 4.2–4.9, which is similar to the findings of this study. Lowering soil pH could induce P fixation by Al and Fe. Fortunately, concentrations of these two cations are very low in the calcareous soils of the study area (Zhang et al., 2014). Bacterial soil acidification might also affect bioavailability of other nutrients or the nutrient balance in rhizosphere soils. However, these bacteria may not effectively solubilize Al and Fe bound P in acidic soils.
The genera of Enterobacter and Pantoea have been reported as PSB in a previous study by Collavino et al. (2010). Enterobacter ludwigii showed the ability to solubilize phosphorus (∼200–800 μg ml−1) through the production of organic acids (Mendoza-Arroyo et al., 2020). The formation of a halo zone by bacteria is considered as the standard method for screening PSB. However, our research showed that strains like Bacillus flexus and Beijerinckia fluminensis are often missed by such screening procedures because they did not produce enough acids at the beginning of the incubation, even though they lowered solution pH as much as other strains after 5 days of incubation. Beijerinckia fluminensis has not been reported for solubilizing inorganic phosphate in literature. The main contribution of this study is the successful isolation of these strains with strong P solubilizing ability from local native environments and the demonstration of their potential to thrive in local calcareous soils and convert non-labile P into a bioavailable form for plants to use.
The GenBank accession numbers of the 16S rRNA sequences for 15 strains in this paper are OL466878, OL466879, OL466880, OL466881, OL466882, OL466883, OL466884, OL466885, OL466886, OL466887, OL466888, OL466889, OL466890, OL466891, and OL466892.
Data Availability Statement
The datasets presented in this study can be found in online repositories. The names of the repository/repositories and accession number(s) can be found below: NCBI [accession: OL466878-OL466892].
Author Contributions
KL, JZ, and SZ contributed to conception and design of the study. QL trained KL for procedures used for the experiment and KL performed the experiment. KL and JZ performed the statistical analysis. KL wrote the first draft of the manuscript. All authors contributed to manuscript revision, read, and approved the submitted version.
Conflict of Interest
The authors declare that the research was conducted in the absence of any commercial or financial relationships that could be construed as a potential conflict of interest.
Publisher’s Note
All claims expressed in this article are solely those of the authors and do not necessarily represent those of their affiliated organizations, or those of the publisher, the editors and the reviewers. Any product that may be evaluated in this article, or claim that may be made by its manufacturer, is not guaranteed or endorsed by the publisher.
Acknowledgments
The senior author thanks Yuqing Fu and Guiqin Yu at the University of Florida for hands-on training of basic techniques of microbiology and chemical analysis.
Supplementary Material
The Supplementary Material for this article can be found online at: https://www.frontiersin.org/articles/10.3389/fenvs.2021.802563/full#supplementary-material
References
Collavino, M. M., Sansberro, P. A., Mroginski, L. A., and Aguilar, O. M. (2010). Comparison of In Vitro Solubilization Activity of Diverse Phosphate-Solubilizing Bacteria Native to Acid Soil and Their Ability to Promote Phaseolus Vulgaris Growth. Biol. Fertil. Soils 46, 727–738. doi:10.1007/s00374-010-0480-x
Dhillon, J., Torres, G., Driver, E., Figueiredo, B., and Raun, W. R. (2017). World Phosphorus Use Efficiency in Cereal Crops. Agron.j. 109, 1670–1677. doi:10.2134/agronj2016.08.0483
Grenon, G., Singh, B., De Sena, A., Madramootoo, C. A., von Sperber, C., Goyal, M. K., et al. (2021). Phosphorus Fate, Transport and Management on Subsurface Drained Agricultural Organic Soils: a Review. Environ. Res. Lett. 16, 013004. doi:10.1088/1748-9326/abce81
Hanlon, E. A., Gonzalez, J. S., and Bartos, J. M. (1997). IFAS Extension Soil Testing Laboratory (ESTL) and Analytical Research Laboratory (ARL) Chemical Procedures and Training Manual; Cir. 812, UF/IFAS Ext. Serv. (Gainesville, FL, USA: University of Florida).
Kalayu, G. (2019). Phosphate Solubilizing Microorganisms: Promising Approach as Biofertilizers. Int. J. Agron. 2019, 1–7. doi:10.1155/2019/4917256
Liu, Z., Li, Y. C., Zhang, S., Fu, Y., Fan, X., Patel, J. S., et al. (2015). Characterization of Phosphate-Solubilizing Bacteria Isolated from Calcareous Soils. Appl. Soil Ecol. 96, 217–224. doi:10.1016/j.apsoil.2015.08.003
Mendoza-Arroyo, G. E., Chan-Bacab, M. J., Aguila-Ramírez, R. N., Ortega-Morales, B. O., Canché Solís, R. E., Chab-Ruiz, A. O., et al. (2020). Inorganic Phosphate Solubilization by a Novel Isolated Bacterial Strain enterobacter Sp. Itcb-09 and its Application Potential as Biofertilizer. Agriculture 10, 383. doi:10.3390/agriculture10090383
Michas, A., Pastore, G., Chiba, A., Grafe, M., Clausing, S., Polle, A., et al. (2021). Phosphorus Availability Alters the Effect of Tree Girdling on the Diversity of Phosphorus Solubilizing Soil Bacterial Communities in Temperate Beech Forests. Front. For. Glob. Change 4, 696983. doi:10.3389/ffgc.2021.696983
NCBI (2021). GenBank. Bethesda (MD): National Library of Medicine US. Available from: https://www.ncbi.nlm.nih.gov/.
Van Zeghbroeck, J., Liu, G., Mylavarapu, R. S., and Li, Y. C. (2021). Phosphorus Management Strategies for Potato Production in Florida: A Review. Am. J. Potato Res. doi:10.1007/s12230-021-09851-2
Zhang, M., Li, C., Li, Y. C., and Harris, W. G. (2014). Phosphate Minerals and Solubility in Native and Agricultural Calcareous Soils. Geoderma 232-234, 164–171. doi:10.1016/j.geoderma.2014.05.015
Keywords: legacy soil phosphorus, Enterobacter ludwigii, Enterobacter sp., Pantoea cypripedii, Bacillu flexuss, Beijerinckia fluminensis
Citation: Li KS, Zeghbroeck J. V, Liu Q and Zhang S (2021) Isolating and Characterizing Phosphorus Solubilizing Bacteria From Rhizospheres of Native Plants Grown in Calcareous Soils. Front. Environ. Sci. 9:802563. doi: 10.3389/fenvs.2021.802563
Received: 26 October 2021; Accepted: 23 November 2021;
Published: 10 December 2021.
Edited by:
Tiequan Zhang, Harrow Research and Development Centre, CanadaReviewed by:
Jing Li, Chengdu University of Technology, ChinaPaula Godinho Ribeiro, Vale Technological Institute (ITV), Brazil
Zhi-Qing Lin, Southern Illinois University Edwardsville, United States
Nadeesha Ukwattage, University of Colombo, Sri Lanka
Copyright © 2021 Li, Zeghbroeck J., Liu and Zhang. This is an open-access article distributed under the terms of the Creative Commons Attribution License (CC BY). The use, distribution or reproduction in other forums is permitted, provided the original author(s) and the copyright owner(s) are credited and that the original publication in this journal is cited, in accordance with accepted academic practice. No use, distribution or reproduction is permitted which does not comply with these terms.
*Correspondence: Shouan Zhang, c3poYW5nMDAwN0B1ZmwuZWR1; Van Zeghbroeck J., anZhbnplZ2hicm9lY2tAdWZsLmVkdQ==