- 1Laboratório de Fisiologia de Plantas Sob Estresse, Departamento de Botânica, Setor de Ciências Biológicas, Universidade Federal do Paraná, Curitiba, Brazil
- 2Fundação Ezequiel Dias, Belo Horizonte, Brazil
- 3Departamento de Zoologia, Instituto de Ciências Biológicas, Universidade Federal de Minas Gerais, Belo Horizonte, Brazil
- 4Ecotoxicology of Aquatic Microorganisms Laboratory, EcotoQ, GRIL, TOXEN, Department of Biological Sciences, Université Du Québec à Montréal, Montréal, QC, Canada
This study investigated the occurrence and risk assessment of ten pharmaceutical products and two herbicides in the water of rivers from the Doce river watershed (Brazil). Of the 12 chemicals studied, ten (acyclovir, amoxicillin, azithromycin, ciprofloxacin, enrofloxacin, fluoxetine, erythromycin, sulfadiazine, sulfamethoxazole, glyphosate and aminomethylphosphonic acid) had a 100% detection rate. In general, total concentrations of all target drugs ranged from 4.6 to 14.5 μg L−1, with fluoroquinolones and sulfonamides being the most representative classes of pharmaceutical products. Herbicides were found at concentrations at least ten times higher than those of the individual pharmaceutical products and represented the major class of contaminants in the samples. Most of the contaminants studied were above concentrations that pose an ecotoxicological risk to aquatic biota. Urban wastewater must be the main source of contaminants in waterbodies. Our results show that, in addition to the study of metal in water (currently being conducted after the Fundão dam breach), there is an urgent need to monitor emerging contaminant in waters from Doce river watershed rivers, as some chemicals pose environmental risks to aquatic life and humans due to the use of surface water for drinking and domestic purposes by the local population. Special attention should be given to glyphosate, aminomethylphosaphonic acid, and to ciprofloxacin and enrofloxacin (whose concentrations are above predicted levels that induce resistance selection).
Introduction
On the 5th of November 2015, one of the biggest environmental tragedies in the world occurred in the municipality of Mariana, MG (Brazil): the collapse of the Fundão dam, belonging to Samarco (a joint venture of Brazilian Vale and Anglo-Australian BHP Billiton), was responsible for releasing about 50 million m3 of mining waste into the environment (Porto, 2016). The disaster, classified as very large and sudden (due to the severity of negative impacts caused), directly affected about 663.2 km of one of the most important Brazilian rivers (Doce river), which stretches between the states of Minas Gerais and Espírito Santo (IBAMA, 2015). Among the environmental impacts caused by the silt wave of tailings, the destruction of permanent protected areas and native vegetation of the Atlantic Forest and above all, the impact on aquatic ecosystems should be highlighted.
The spoil heaps of the Fundão dam quarry flooded the district of Bento Rodrigues, however, it was dammed by the Risoleta Neves hydroelectric power plant. This was practically the only area of floodplain affected by the disaster. The material deposits in the area were considered an ecological time bomb due to their potential to release metals into the environment, including water—although this remains controversial in the literature (Queiroz et al., 2018). After the disaster, monitoring the water quality of the Doce river became a priority to track the potential impact on the disaster on aquatic environment. In addition, this study is important because the Doce river water is used to supply several cities in the states of Minas Gerais and Espírito Santo. However, the main focus of these studies was to evaluate the metal concentrations in the water. As far as we know, there have been no studies that have evaluated organic contamination of the water by emerging contaminants such as pesticides and personal and pharmaceutical products. In addition to mining activities, the Doce river watershed experiences continuous discharges of untreated wastewater, as well as contamination from agriculture (e.g., fertilizers and pesticides) and inadequate disposal of municipal waste (ANA, 2015) which are inevitably reflected in the presence of these emerging contaminants in the water. Once in the aquatic environment, drug and pesticide residues can cause potential environmental risks by affecting aquatic organisms and, in the case of antibiotics, promoting the spread of antibiotic-resistant genes (Gomes et al., 2017; Gomes et al., 2019; Mendes et al., 2021). In addition, the use of contaminated water for crop irrigation can lead to the accumulation of pesticides and pharmaceuticals in crops and their uptake into the food web (Gomes et al., 2019; Gomes et al., 2020b). Here, we tracked concentrations of pharmaceuticals and pesticides in water from rivers in the Doce river Watershed from 2018 to 2019. We wanted to draw attention to the need to focus water investigations on the presence of novel contaminants that may affect water and environmental safety, in addition to metals.
Materials and Methods
Study Area
The study area includes the Doce river drainage watershed, in the state of Minas Gerais (Brazil) (Figure 1). The region has approximately 199,000 inhabitants, mainly located in the urban area of the cities of Ouro Preto (74,558 inhabitants) and Mariana (61,228 inhabitants) (IBGE, 2020). Samples were collected from four sites with pronounced human activities in vicinity of the collecting point (Supplementary Tables S1, S2): In the Carmo River, samples were collected in the Mariana downtown area, typically characterized by urban discharges (Figure 1, site 1), and near the small town of Acaiaca (3,994 inhabitants) (Figure 1, site 2), which is surrounded by some agricultural fields with extensive livestock (mainly) and eucalyptus plantations. In the Gualaxo do Norte River, the samples were collected in an area surrounded by agricultural fields (mainly arable); the water also receives effluents from mining in SAMARCO iron ore mining (Figure 1, site 3). Finally, samples from the Doce river were collected near Risoleta Neves dam (Figure 1, site 4). The Doce river is formed by the confluence of the Piranga and Carmo rivers and, also receives urban runoff from the city of Ponte Nova via the Piranga river (Figure 1; Supplementary Tables S1, S2).
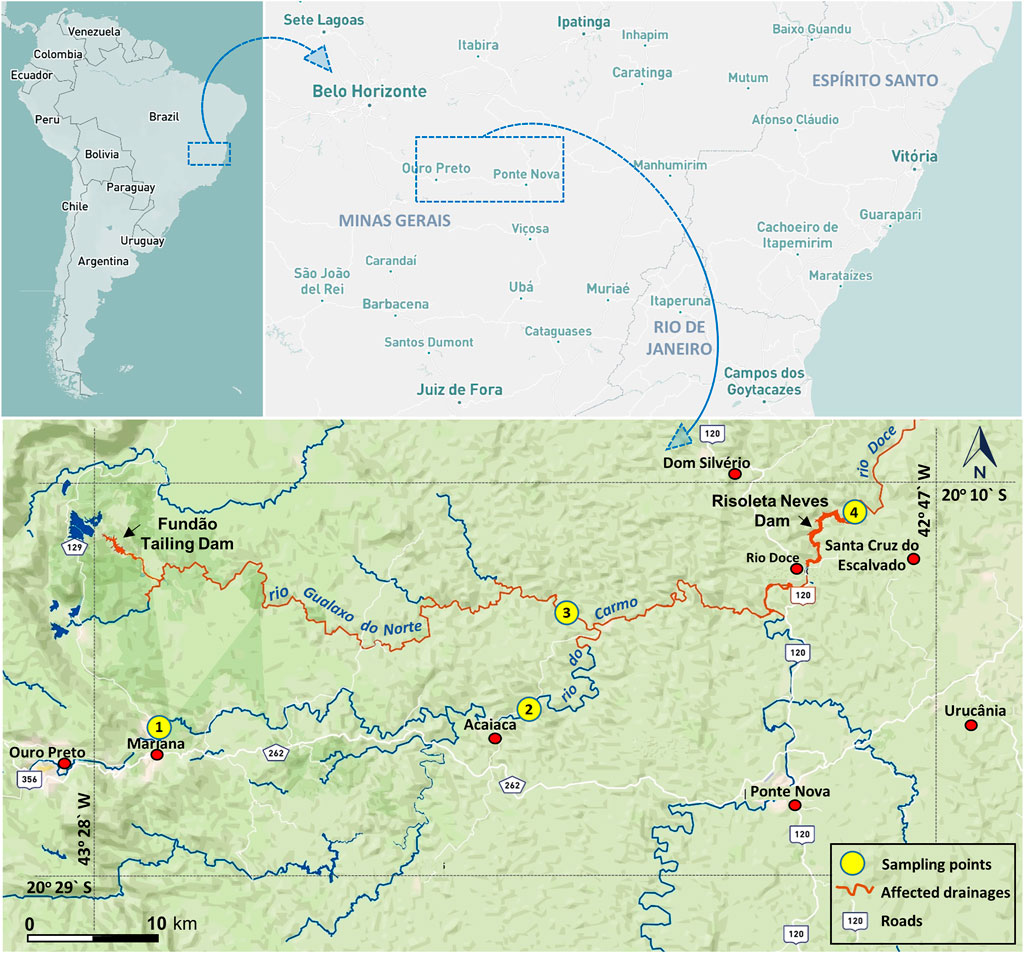
FIGURE 1. Sampling sites along the Rio Doce watershed, Minas Gerais, Brazil. Site 1 in the Carmo River in the Mariana district, characterized by urban discharges; Site 2 in the Carmo River near its surrounding agricultural fields (mainly extensive cattle ranching and eucalyptus plantations); Site 3 in the Gualaxo do Norte River, surrounded by agricultural fields (mainly arable), into which wastewater in SAMARCO iron ore mining; and Site 4 in the Doce river which is formed by the junction of the Piranga and Carmo Rivers, also receiving urban discharges from the city of Ponte Nova through the Piranga River.
Selection of the Studied Chemicals
Pharmaceuticals (acyclovir, amoxicillin, azithromycin, ciprofloxacin, doxycycline, enrofloxacin, fluoxetine, erythromycin, sulfadiazine and sulfamethoxazole) were selected based on their abundance in on surface waters worldwide (Grill et al., 2016; Bertram et al., 2018; Beatriz et al., 2020; Gupta et al., 2021). Antibiotics such as amoxicillin (β-lactam), azithromycin and erythromycin (macrolides), ciprofloxacin and enrofloxacin (fluoroquinolones), doxycycline (tetracyclines), sulfamethoxazole and sulfadiazine (sulfonamides) are among the most commonly used in human and animal treatment, aquaculture and as feed additives (Giang et al., 2015). Acyclovir is one of the most effective and widely used anti-herpes agents (Mucsi et al., 1992; Gupta et al., 2021) and, fluoxetine is one of the most commonly prescribed antidepressants (Bertram et al., 2018). Glyphosate, on the other hand is the most commonly used herbicide in the world (Gomes et al., 2014) and is frequently used in the fields surrounding the sampling sites. Conversely, aminomethylphosphonic acid (AMPA) is the major metabolite of glyphosate, which is formed in the environment mainly through the degradation of the herbicide by microbes (Brock et al., 2019). In addition, organic phosphonates used in both industrial and domestic applications (detergents, flame retardants, corrosion inhibitors, anti-limescale agents and in the textile industry) are also sources of AMPA in aquatic ecosystems (Levine et al., 2015; Grandcoin et al., 2017).
Sampling Campaign and Preparation
Sampling was conducted in June 2018 (total precipitation from 0.2 to 0.6 mm/average flow rate 3.98 to 48.54 m3/s), November 2018 (total precipitation from 146.1 to 210.1 mm/average flow rate 9.73 to 118.26 m3/s), and April 2019 (total precipitation from 108.0 to 142.8 mm/average flow rate 5.95 to 102.75 m3/s) (Supplementary Tables S3, S4). All sampling equipment was thoroughly cleaned with 70% ethanol before fieldwork and then washed with deionized water. Three water samples (5,000 ml) were collected at 50 m intervals at each point. The surface water samples were collected in sterile amber glass bottles. Samples were stored in ice (4°C) until arrival at the laboratory and then filtered through glass fiber membranes (0.45 µm, Millipore). Samples were then separated for evaluation of drugs (acyclovir, amoxicillin, azithromycin, ciprofloxacin, doxycycline, enrofloxacin, fluoxetine, norfloxacin, erythromycin, sulfamethoxazole, and sulfadiazine) and herbicides [glyphosate and aminomethylphosphonic acid (AMPA)]. The pH of the samples was adjusted to 6.5 and 2.5 for the drug and herbicide analyses, respectively.
For the drug analyses, the filtered water samples (500 ml) were concentrated by solid-phase extraction (SPE) using a Visiprep™ SPE Vaccum manifold (Sigma-Aldrish, Brazil) with 200 mg, 3 ml−1 Phenomenex Strata-X® cartridges (Torrance, California, United States). SPE conditions were the same as those described by to Beatriz et al. (2020). Cartridges were conditioned with 4 ml methanol followed by 6 ml ultrapure water and analytes were eluted in 4 ml methanol. For herbicide evaluation, samples were concentrated using C18 cartridges (500 mg/6 ml; Applied Separations, United States) previously conditioned with 15 ml of acidified water (pH 2.5) and 5 ml of methanol (Mendes et al., 2021). The cartridges containing the samples were eluted with 3 ml of 50% methanol in water (v/v). For both antibiotics and herbicides, the eluate was dried in a SpeedVac device (RC1010, Thermo), and the residues resuspended in the mobile phase (water and acetonitrile in a 50:50 v/v ratio with 0.1% formic acid and 5 µM ammonium formate for antibiotics and 5 mM ammonium acetate in water for herbicides).
Chromatographic Analyses
Analyses were performed using a LC-MS/MS system consisting of a Xevo TQD triple quadrupole mass spectrometer (Waters) with electrospray (ESI) ionisation source and an HPLC Varian SYS-LC-240-E with autosampler. Drugs were evaluated following (Beatriz et al., 2020), while glyphosate and AMPA were evaluated following (Gomes et al., 2015). For the drugs, chromatographic separations were performed using a 4.6 mm × 150 mm, 5 µm particle size Zorbax Eclipse XDB-C8 column (Agilente, Milford, United States) using water as phase A and acetonitrile/water (95:5 v/v) as phase B, both containing 0.1% formic acid and 5 mM ammonium formate. For the herbicides, chromatographic separations were performed using an Ascentis® C18 column (Sigma-Aldrich, Brazil) with a mobile phase consisting of 5 mM ammonium acetate in water (phase A) and 5 mM ammonium acetate in methanol (phase B), both pH 7.0. Mass spectrometry analyses were performed in positive and negative ion modes for antibiotics and herbicides, respectively. Acyclovir (ACY), amoxicillin (AMO), azithromycin (AZI), ciprofloxacin (CIP), doxycycline (DOX), enrofloxacin (ENR), fluoxetine (FLU), erythromycin (ERY), sulfadiazine (SDZ), sulfamethoxazole (SMX), glyphosate (GLY) and AMPA (Sigma-Aldrich, Canada) in analytical grade were used to construct the calibration curves. Standard stock solutions (1,000 μg ml−1) of these compounds were prepared using different compositions of methanol, water and acetonitrile, with formic acid and ammonium formate, depending on solubility. The six-point calibration curves showed good linearity for the analytes (r2 ≥ 0.95; p < 0.0001). Each sample batch included three blanks, three standards, and three fortified samples (for quality control). The recoveries for all compounds were greater than 87%. The limit of detection (LOD) and limit of quantification (LOQ) of each analyte are listed in Table 1.
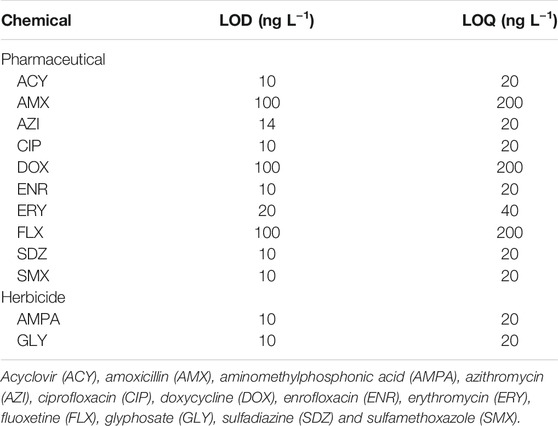
TABLE 1. Limit of detection (LOD) and limit of quantification (LOQ) of drugs and herbicides evaluated using a LC-MS/MS (Gomes et al., 2015; Beatriz et al., 2020).
Ecological Risk Assessment
The predicted no effect concentration (PNEC) was estimated using the ecological structure-activity relationships (ECOSAR) model (Moore et al., 2003) and was calculated by dividing the no-observed effect concentration (NOEC) found in the literature by an assessment factor (AF) of 1,000, which represents chronic toxicity (Ikem et al., 2017).
Hazard quotient (HQ) was used to assess the environmental risk of chemicals and their potential to cause adverse effects in the environment (Carlsson et al., 2006) and was calculated as follows:
Where PNEC is the predicted no-effect concentration (from literature) and MEC is the measured environmental concentration. For MEC, the mean of the concentrations found over time (n = 9) for each collection site was used.
Statistical Analyses
Results were expressed as the average of three replicates. Statistical analyses were performed using JMP 10.0 software (SAS Institute Inc.). Results were subjected to normality (Shapiro–Wilk) and homogeneity (Bartlett) tests and then statistically analyzed. Univariate repeated measures ANOVA, with time as a within-subject factor and sites as the main effect, were used to analyze differences in chemical concentrations during the sampling period. The sphericity of the data was tested using Mauchly’s criteria to determine if the univariate F-tests were valid for within-subject effects. If F-tests were invalid, the Greenhouse-Geisser test was used to estimate epsilon (ε). Contrast analysis was used when there were significant differences in the variables examined.
Results
Occurrence of Pharmaceutical Products on Surface Waters
With the exception of DOX, all surface water samples were contaminated with the tested drugs (Figure 2). The highest concentrations of antibiotics were found in CIP (up to 4,854.6 ng L−1), followed by SMX (up to 9,640 ng L−1). The concentrations of DOX (up to 2.25 ng L−1) were lower (or were not detected) compared to the other drugs (Table 2). With the exception of ERY and FLX (p > 0.05), a significant interaction (p < 0.05) between time and site of sampling was observed for the drugs (Table 2). Regardless of the sampling date, the concentrations of ACY, AMX, CIP, SDZ, SMX (except for the last sampling date) were higher and ENR concentration was lower at site one than at the other sampling sites (Figure 2). High concentrations of ACY, AZI, CIP, SDZ and SMX were detected in the water samples from site one on the first sampling date and lower concentrations of ACY, AMX, ENR, ERY, and SDZ were detected on the second sampling date compared to the other sampling dates (Figure 2). CIP concentrations decreased and ENR concentrations increased over time in the site 2 water samples (Figure 2). In addition, ERY, SDZ, and SMX concentrations were lower in site 2 water samples at the second time point compared to the other sampling time points (Figure 2). With the exception of ENR, whose concentration decreased at the second sampling time point, and ACY, whose concentration increased at the last sampling time point, the concentrations of the other drugs in the water samples from Site three did not differ significantly (p < 0.05) over time (Figure 2). When compared over time of collection, the concentrations of ACY, AZI, and SDZ were higher in the water samples from Site four on the first sampling date and the concentrations of AMX and ERY were lower on the second sampling date (Figure 2).
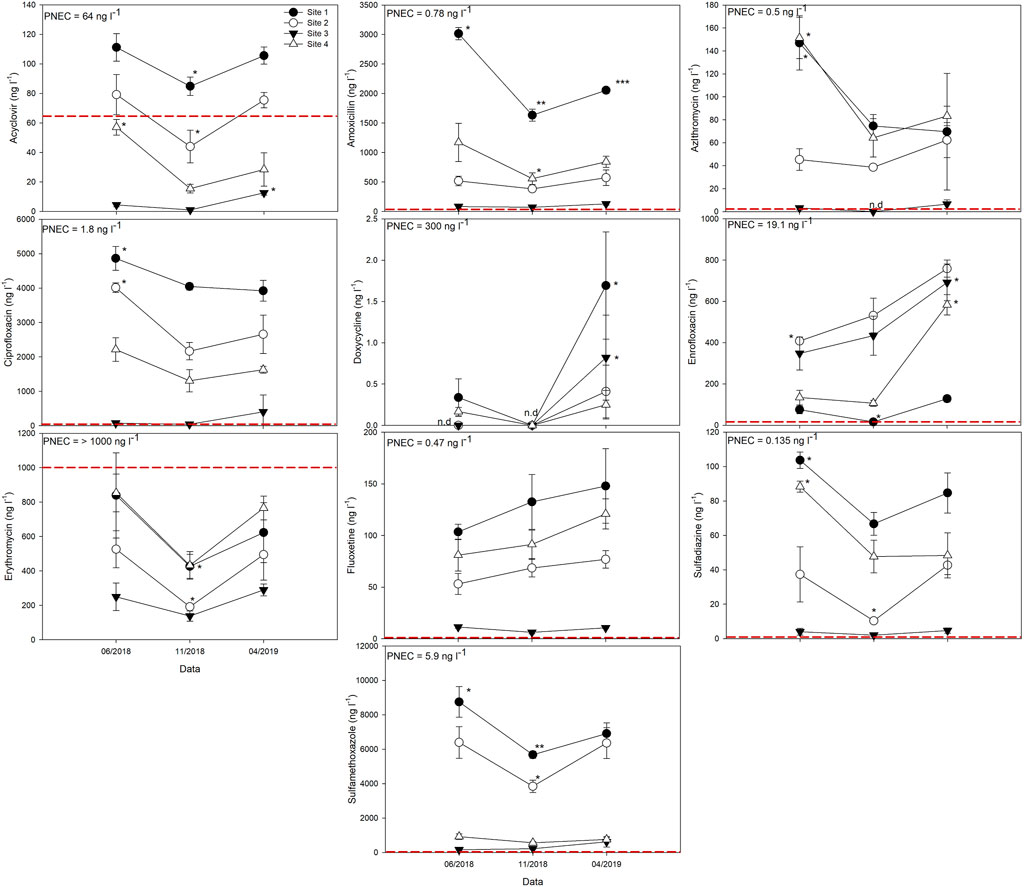
FIGURE 2. Concentrations of pharmaceutical target products in surface water samples from rivers of the Doce river watershed, Minas Gerais, Brazil. Values are means ± SD of three replicates. Values marked with * differ significantly (p < 0.05) within the same sampling site by the contrast test. Predicted no effect concentration (PNEC) is shown as dotted red line. NOEC = concentration with no observed effect.
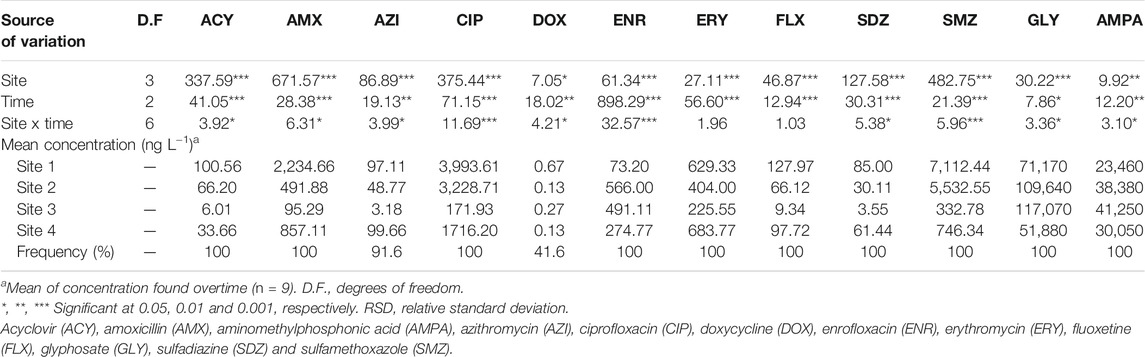
TABLE 2. Repeated-measure ANOVA for the effects of the site of sampling and time on the concentration of chemicals in surface water of rivers of Rio Doce Basin.
Occurrence of Glyphosate and AMPA in Surface Waters
All surface water samples were contaminated with GLY and AMPA (Figure 3). For GLY and AMPA, a significant interaction (p < 0.05) was observed between time and site of sampling (Table 2). Higher concentrations of these chemicals were observed in samples from sites 2 and three compared to samples from sites one and 2 on the first day of sampling. Glyphosate and AMPA concentrations increased over time in samples from sites 1 and 2, respectively (Figure 3). At site 3, AMPA concentrations were lower on the second sampling date (Figure 3). Glyphosate and AMPA concentrations in samples from site four did not differ over time (Figure 3).
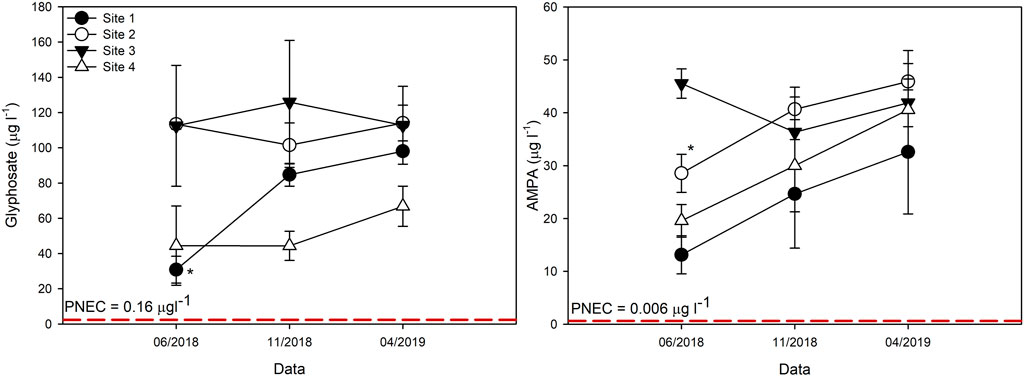
FIGURE 3. Concentrations of glyphosate and AMPA in surface water samples from rivers of the Doce river watershed, Minas Gerais, Brazil. Values are means ± SD of three replicates. Values marked with *, differ significantly (p < 0.05) within the same sampling site by the contrast test. Predicted no effect concentration (PNEC) is shown as dotted red line. NOEC = concentration with no observed effect.
Ecological Risk Assessment
With the exception of ACY, DOX, ERY, the observed concentrations of the chemicals were higher than their calculated PNEC(ecotox) (Figures 2, 3 and Table 3). For ACY, all concentrations observed at site one were greater than the calculated PNEC(ecotox); for site 2, only the concentrations found on the first and last sampling dates were greater than the calculated PNEC(ecotox). At all sites, the observed concentrations of AZI, DOX, ERY, and SMZ were lower than the PNEC(resi. sel) (Table 3). Only the HQ of DOX and ERY were lower than one for all sites. At sites 3 and 4, HQ < 1 was also observed for ACY (Table 3).
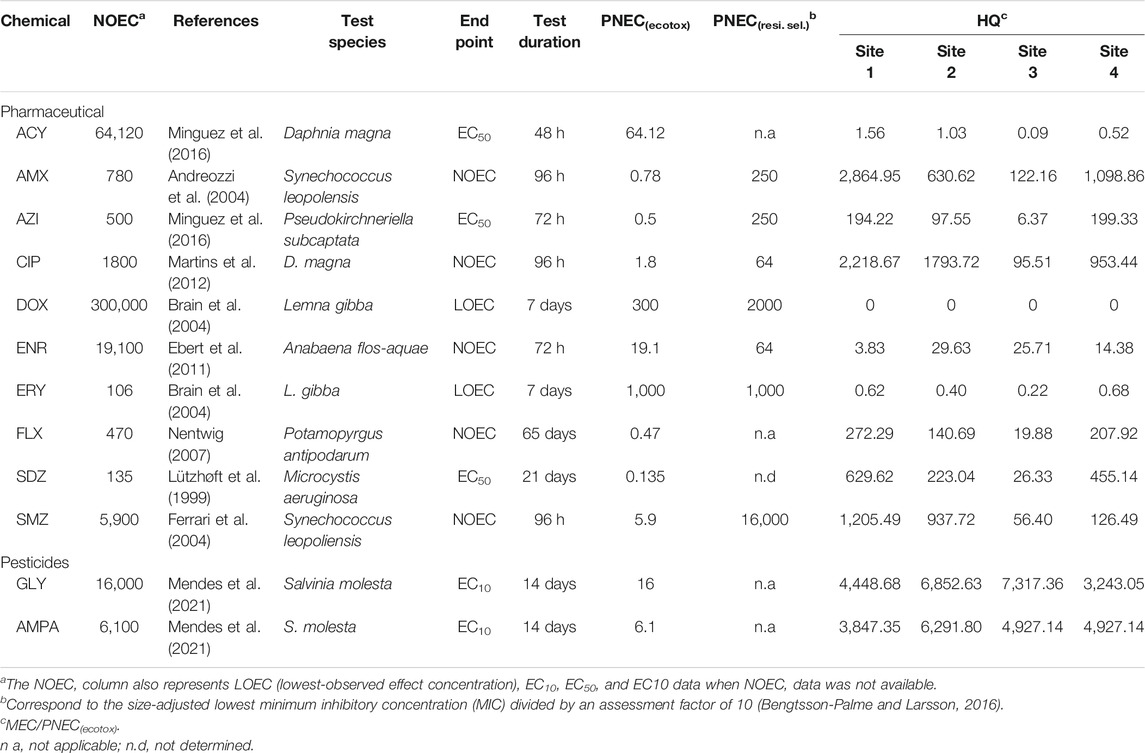
TABLE 3. No-observed effect concentration (NOEC), the predicted no effect concentration based on ecotoxicological data [PNEC(ecotox)], predicted no effect concentration for antibiotic-resistance selection [PNEC(resi. sel.)] and hazard quotient (HQ) (in ngl−1).
Discussion
Of the ten pharmaceutical products studied, all had a 100% detection rate, except for AZI (91.6%) and DOX (41.6%) (Table 3). In general, the total concentration of all target pharmaceutical ranged from 4,595.40 to 14,478.59 ng L−1, with fluoroquinolones (CIP + ENR) and sulfonamides (SDZ + SMX) accounting for 28.08–49.42% and 17.57–53.28%, respectively. Based on the average proportion at all sites, the proportion of different pharmaceuticals was as follows: Fluoroquinolones ≥ Sulfonamides > Macrolides (AZI + ERY) (5.01–14.05%) > β-Lactam (AMX) (4.05–18.65%) > antiretrovirals (ACY) (1.5–1.9%) antidepressants (FLU) (0–4.6%) > tetracyclines (DOX, <0.1%).
Among the fluoroquinolones, CIP was the most frequently detected antibiotic, regardless of the site and time of sampling (Figure 2), which is not surprising since CIP is the most commonly prescribed fluoroquinolone worldwide (Andreu et al., 2007) whose bactericidal effect is based on inhibition of DNA replication by inhibition of bacterial DNA topoisomerase and DNA-gyrase. CIP has been detected in milligram amounts in sewage sludge (Golet et al., 2003; Martínez-Carballo et al., 2007). However, in water samples, the detected concentrations are lower. In untreated hospital wastewater, CIP concentrations ranged from 1,100 to 44,000 ng L−1 in Vietnam and 388–578 ng L−1 in Malaysia (Duong et al., 2008; Thai et al., 2018). In urban wastewater, CIP was previously detected at concentrations ranging from 242 to 415 ng L−1 in China (Low et al., 2021) and in municipal landfills, concentrations ranged from 60.2 to 4,482 ng L−1 (Wu et al., 2015). In Brazilian surface waters, CIP concentrations were below 0.41 ng L−1 in the Atibaia River (São Paulo) (Locatelli et al., 2011) and ranged from 180 to 340 ng L−1 in rivers from the four largest hydrographic catchments of the city of Curitiba (Paraná) (Beatriz et al., 2020). The higher CIP concentration in surface waters observed in our study must be related to the lack of wastewater treatment (present in the other Brazilian cities cited) and the direct discharge of urban wastewater into the waters of Doce river watershed rivers. Similarly, a CIP concentration of 15,000 ng L−1 was observed in surface water in South Africa (Agunbiade and Moodley, 2014). Indeed, samples from sites under the influence of direct urban discharges (sites 1, 2 and 4) had high concentrations of the antibiotics compared to sites without urban proximity (site 3). Interestingly, ENR concentrations were lower in samples from site one compared to the other sites (Figure 2). This antibiotic is used in veterinary medicine (Rusu et al., 2015), and indeed high ENR concentrations were found at sites near livestock (sites 2 and 3). It is important to note that ENR can be degraded to CIPRO through biotransformation (Walters et al., 2010), which could contribute to the CIP concentrations in water at sites 2 and 3.
In the group of sulfonamides, SMX were detected higher concentrations (from 332.78 to 7,112.44 ng L−1) than SDZ (from 3.55 to 61.44 ng L−1) (Figure 2; Table 3). In rivers near the city of Curitiba (Brazil), SXM was found at concentration of 1859 ng L−1, while SDZ were reported at concentration of 27 ng L−1 (Beatriz et al., 2020). In South Africa, SMX was detected at concentrations of 7,300 (Matongo et al., 2015) and 14,000 ng L−1 in surface waters (Ngumba et al., 2016). In Kenya, concentrations of up to 40,000 ng L−1 have been observed in river waters (K’oreje et al., 2016) while SDZ has been detected in concentrations of up to 40 ng L−1 in rivers from Nigeria (Oluwatosin et al., 2016). In China, up to 764.6 ng SMX l−1 has been detected in rivers (Chen and Zhou, 2014). Sulfonamides are bacteriostatic antibiotics that interfere with folic acid synthesis and are mainly used for acne and urinary tract infections—justifying their high concentrations in rivers near cities (sites 1 and 2). In China, sulfonamides were the major class of antibiotics found in rivers. SDZ and SMZ were detected at 100% and had mean concentrations of 259.6 and 7.6 ng L−1, respectively (Chen and Zhou, 2014).
Macrolides such as AZI and ERY inhibit bacterial protein biosynthesis, while the β-lactam AMX acts by binding to penicillin-binding proteins, resulting in the activation of autolytic enzymes in the bacterial cell wall. These antibiotics are used for both human and veterinary purposes. This may explain why macrolide and β-lactam concentrations were lowest at site 4, where there are no direct urban discharges and where crop cultivation is the main activity in the environment (Figure 2). Among macrolides, ERY was observed at high concentrations in our study, regardless of time and sites of collection (Table 3; Figure 2). ERY concentrations up to 20,000 ng L−1 and 1,000 ng L−1 were observed in surface waters in South Africa (Matongo et al., 2015) and Nigeria (Oluwatosin et al., 2016), respectively. AZI concentrations ranged up to 650 ng L−1 in Brazilian rivers (Beatriz et al., 2020) and up to 30 ng L−1 in South African rivers (Módenes et al., 2017), while it was not detected in Nigerian rivers (Oluwatosin et al., 2016). As for β-lactam, AMX has been detected in concentrations up to 99.4 mg L−1 in wastewater in Egypt (Abou-Elela and El-Khateeb, 2015). In Brazil, this antibiotic has been detected at concentrations up to 1,570 ng L−1 in rivers from the Curitiba region (Beatriz et al., 2020) and up to 1,284 ng L−1 in rivers from the state of S o Paulo (Locatelli et al., 2011).
Data on the concentrations of ACY and FLX are scarce in the literature. These drugs are used to treat human, which justifies their high concentrations in areas with urban runoff (Figure 2). ACY is generally used as the first choice in the treatment of viral infections such as herpes simplex, Varicella zoster, herpes zoster, herpes labialis and acute herpetic keratitis (OʼBrien and Campoli-Richards, 1989). In Brazilian rivers (Curitiba, Paraná), concentrations ranged from ACY to 990 ng L−1 (Beatriz et al., 2020). In Germany, ACY concentrations in river water ranged up to 190 ng L−1 (Prasse et al., 2010). Average concentrations of FLX in surface waters concentrations ranged from 12 to 1,400 ng L−1 worldwide (Kolpin et al., 2002; Christensen et al., 2009). In Brazil, FLX concentrations in streams of Curitiba were as high as 620 ng L−1 (Beatriz et al., 2020). FLX is primarily used to treat depression, but also helps treat other mental disorders such as obsessive-compulsive disorder, bulimia nervosa, and panic syndrome, and is one of the most commonly prescribed psychotropic drugs in Brazil (Quintana et al., 2015). As with some other drugs studied, we found relatively high concentrations of pharmaceuticals in the waters of the Doce river watershed (Figure 2). Seasonal aspects could have influenced the results obtained. For example, the concentrations of ACY, AMX, AZI, CIP, ERY, SDZ and SDX were high at some sites on the first sampling date which corresponding to the dry season. During a low-precipitation period, water flow decreases and, assuming that pollution sources are constant, dilution effects must play a central role in the occurrence and concentrations of pharmaceuticals in the water samples sampled (Locatelli et al., 2011).
The flow of a river is the result of complex natural processes that occur at the catchment scale and are largely influenced by precipitation (Yunus and Nakagoshi, 2004). Changes in streamflow affect water quality (Caruso, 2001) and pollution of rivers increases when streamflow is low, due to low dilution capacity (Yunus and Nakagoshi, 2004). We clearly observed the influence of rainfall on flow (Supplementary Table S4) and concentration of the studied drugs (Figure 1). At least for two of the sampling sites, the concentrations of the analyzed drugs (except for DOX and ENR) were higher when precipitations were the lowest (June 2018). The higher concentrations of DOX and ENR at the higher rainfall levels (294 mm in November 2018 and 108 mm in April 2019), indicate that the source of these drugs increased during rainy season. This could be due to increased seepage and runoff (Yunus and Nakagoshi, 2004) or to the increased use of these drugs during the rainy season.
Although high concentrations of pharmaceuticals were found in the water samples, the most worrying results are associated with the observed GLY and AMPA concentrations (Figure 3). GLY and AMPA contamination levels exceeded those observed for pharmaceutical products by several times. These contaminants were observed at concentrations ranging from 51.88 to 117.07 and 23.46–41.25 μg L−1, respectively, indicating that herbicides are the main source of contamination in the rivers studied. In the Paraná River watershed (Brazil), GLY concentrations ranged from 0.4 to 91.91 μg L−1 (Mendonça, 2018), while AMPA was detected at concentrations up to 14.78 μg L−1 (Da Silva et al., 2003). In another study, glyphosate concentrations up to 100 μg L−1 and AMPA concentrations up to 50 μg L−1 were detected in the Arroio Passo do Pilão watershed (Brazil). GLY is not only used in crops and eucalyptus plantations but is also widely used for weed control in Brazilian cities, and its use is often unregulated. Therefore, it is possible that GLY concentrations >80 μg L−1 at sites under urban areas (Figure 3). In addition to GLY, the observed concentrations of AMPA in water samples must be derived from its use in industry and household products (such as detergents). (Levine et al., 2015; Grandcoin et al., 2017). Unlike pharmaceuticals, concentrations of GLY and AMPA were not affected by pluviosity (except for sites one and 2 for glyphosate and AMPA, respectively). Considering the dilution effect of high pluviosity and river flow on river pollutants (Yunus and Nakagoshi, 2004), we hypothesize that herbicide use was increased during rainy season. In fact, glyphosate uses in Brazil is declining from April to September, as the herbicide is mainly used during the rainy season, when crops are growing (Dias et al., 2021). At concentrations as low as 5 μg L−1, the herbicide glyphosate reduced algal diversity in phytoplankton communities of freshwater streams (Smedbol et al., 2018) and the EC10 value for GLY and AMPA in the macrophyte Salvinia molesta was 16 and 6.1 μg L−1, respectively (Mendes et al., 2021). Therefore, it is reasonable to assume that the concentrations of these herbicides found in the Doce river watershed could trigger an alteration of aquatic life, and to assess the potential risk of these chemicals (along with the pharmaceutical products evaluated), we conducted a risk assessment.
PNEC values are based on toxicological data from the literature. In this study, we selected the NOEC of species representative of the those found in Brazil to calculate the PNEC(ecotox), using an assessment factor of 1,000, which represents chronic toxicity (Ikem et al., 2017). If the reported concentrations in the environment are higher than the PNEC, there is a toxicological risk to the environment. With the exception of ACY, DOX, ERY, the concentration of all other chemicals studied poses a potential toxicological risk. In the case of ACY, the observed concentrations at site one are also of toxicological concern. The risk level is generally classified into four groups: no risk (HQ < 0.01), low risk (0.01 ≤ HQ ≤ 0.1), medium risk (0.1 ≤ HQ ≤ 1), and high risk (HQ > 1) (Rodriguez-Mozaz et al., 2020). Only DOX had a HQ < 0.01, and did not pose an ecotoxicological risk to the aquatic environment. For the site 3, ACY poses a low risk (HQ = 0.09), and for site 4, ACY poses a moderate risk (HQ = 0.52). Similarly, ERY poses a medium risk (HQ < 1) to aquatic life, regardless of sampling locations. However, for all other chemicals sampled, HQ was greater than 1 (and can reach 7,317.36), representing a high ecotoxicological risk to the aquatic environment. The mean value of HQ for Cd, Pb, Cr, Zn, Cu and As in the Doce river ranged from 226.30 to 841.60 (Gabriel et al., 2020). Although the HQ indices for these metals and metalloids represent a high ecotoxicological risk, they are lower than the HQ calculated here for some chemicals (i.e., AMX, CIP, GLY, and AMPA) (Table 3). These results demonstrate the urgent need to consider emerging contaminants (and not just metals) in risk assessment, given the importance of these chemicals to aquatic ecosystems. It is also important to note that concentrations of AMX, CIP, and ENR are high than the proposed PNEC for resistance selection. Antimicrobial resistance is an emerging concern, as the spread of resistance genes is a global problem with direct detrimental effects on the economy and public health (Kent et al., 2020). Moreover, very few studies have investigated the toxicity of drug mixtures in natural water samples. For example, Gomes et al. (2020a) observed interactive effects of AMX, ENR, and oxytetracycline on Lemna minor plants, which demonstrates the importance of evaluating both the isolated and integrative toxic effects of chemicals. Clearly, toxicological testing involving exposure to a cocktail of multiple drugs is needed, especially in highly contaminated surface water (Anh et al., 2021), as noted here.
The main objective of this study was to draw attention to the presence of considerable amounts of emergent contaminants in the waters of the Rio Doce basin, which, among other contaminants, such as trace elements, can limit aquatic life. Our data also suggest that environmental factors, especially pluviosity (and its effect on water flow), play an important role in the concentrations of chemicals found in the water. The fate of organic contaminants is influenced by the physicochemical and biological properties of the water and sediments. Indeed, temperature, pH, microbial activity and light conditions may affect the availability of the contaminants (Moncmanová, 2007) and alter their rates of degradation, sorption, and bioaccumulation. Therefore, we cannot comment on the exact contribution of an upstream source to the concentration of chemicals along the river (downstream sites). To this end, studies with isotopically labeled chemicals would permit to elucidate the fate as well as the specific role of anthropogenic activities on concentrations of emerging contaminants in the Rio Doce basin rivers. However, in a climate change scenario, we pointed out the possible increased of toxicological impacts of contaminants. As a result of rising temperatures, increased drought, El-Niño Southern Oscillation, and reduced pluviosity (Caruso, 2001), there may be low water flow and increased concentrations and harmful effects of chemicals on aquatic ecosystems.
Conclusion
Through sampling and analysis, the concentrations and distribution of 12 contaminants (pharmaceutical products and herbicides) were determined at four different sites in rivers of the Doce river watershed. Although the concentrations detected were within the range of those observed in other emerging countries, the sampled waters were highly contaminated, especially by the herbicide GLY and its metabolite AMPA. The risk assessment analysis conducted here shows that most of the chemicals assessed are present at concentrations above the PNEC(ecotox) level, posing a potential threat to the aquatic environment. In addition, several antibiotic concentrations are higher than those known to cause antibiotic resistance, particularly those in the fluoroquinolone class. The concentrations of chemicals studied were related to human activities in vicinity of the sampling sites, but the lack of water treatment in urban areas could be the main cause of river contamination. Based on the HQ index, the risk assessment approach provided useful guidance on which chemicals needs to our priority attention for future control and remediation. In this context, particular attention needs to be give to GLY, AMPA, fluoroquinolones and sulfonamides. The results show that there is an urgent need to monitor the presence of emerging contaminants in water, which, in addition to metals (the main target in the study of water quality in the rivers of the Doce river watershed), may pose risk to the environment and humans due to the frequent use of surface water for drinking and domestic purposes by the local population.
Data Availability Statement
The raw data supporting the conclusion of this article will be made available by the authors, without undue reservation.
Author Contributions
MG, FV, and PJ conceived and designed the experiments, gave technical support and conceptual advice. MG, JB, RK, and PJ performed chemical analysis. MG, JB, and PJ wrote manuscript. FV provided technical and editorial assistance. All authors read and approved the manuscript.
Funding
This research was financed in part by the Coordenação de Aperfeiçoamento de Pessoal de Nível Superior—Brasil (CAPES)—Financial Code 001; by the Conselho Nacional de Desenvolvimento Científico e Tecnológico (CNPq, Brazil) through a strategic grant "REDES—Remediation of the Rio Doce Basin: potential of the aquatic and terrestrial biota" (Grant number 88881.118082/ 2016-01) and “Contaminação dos sistemas lóticos e produtos alimentares por antimicrobianos: da aquisição à prevenção da resistência em microrganismos (Grant number 406190/2018-6) awarded to MG; and the Natural Sciences and Engineering Research Council of Canada (Grant number RGPIN-2017-06210) awarded to PJ, MG received research productivity scholarship from CNPq.
Conflict of Interest
The authors declare that the research was conducted in the absence of any commercial or financial relationships that could be construed as a potential conflict of interest.
Publisher’s Note
All claims expressed in this article are solely those of the authors and do not necessarily represent those of their affiliated organizations, or those of the publisher, the editors and the reviewers. Any product that may be evaluated in this article, or claim that may be made by its manufacturer, is not guaranteed or endorsed by the publisher.
Supplementary Material
The Supplementary Material for this article can be found online at: https://www.frontiersin.org/articles/10.3389/fenvs.2021.801599/full#supplementary-material
References
Abou-Elela, S., and El-Khateeb, M. (2015). Performance Evaluation of Activated Sludge Process for Treating Pharmaceutical Wastewater Contaminated with B-Lactam Antibiotics. J. Ind. Pollut. Control. 31, 1–5.
Agunbiade, F. O., and Moodley, B. (2014). Pharmaceuticals as Emerging Organic Contaminants in Umgeni River Water System, KwaZulu-Natal, South Africa. Environ. Monit. Assess. 186, 7273–7291. doi:10.1007/s10661-014-3926-z
ANA (2015). Encarte Especial sobre a Bacia do Rio Doce: Rompimento da Barragem em Mariana/MG. Brasília: DF. Brazil.
Andreozzi, R., Caprio, V., Ciniglia, C., de Champdoré, M., Lo Giudice, R., Marotta, R., et al. (2004). Antibiotics in the Environment: Occurrence in Italian STPs, Fate, and Preliminary Assessment on Algal Toxicity of Amoxicillin. Environ. Sci. Technol. 38, 6832–6838. doi:10.1021/es049509a
Andreu, V., Blasco, C., and Picó, Y. (2007). Analytical Strategies to Determine Quinolone Residues in Food and the Environment. Trac Trends Anal. Chem. 26, 534–556. doi:10.1016/j.trac.2007.01.010
Anh, H. Q., Le, T. P. Q., Da Le, N., Lu, X. X., Duong, T. T., Garnier, J., et al. (2021). Antibiotics in Surface Water of East and Southeast Asian Countries: A Focused Review on Contamination Status, Pollution Sources, Potential Risks, and Future Perspectives. Sci. Total Environ. 764, 142865. doi:10.1016/j.scitotenv.2020.142865
Beatriz, B., Raquel de O, V., Mariana, M. F., Victor, C., Allan, M. J., Josiane, M. F. S., et al. (2020). HPLC-MS/MS Method for Quantification of Pharmaceuticals in Subtropical Rivers and Water Treatment Plants in Brazil. J. Environ. Sci. Public Health 04, 390–408. doi:10.26502/jesph.96120109
Bengtsson-Palme, J., and Larsson, D. G. J. (2016). Concentrations of Antibiotics Predicted to Select for Resistant Bacteria: Proposed Limits for Environmental Regulation. Environ. Int. 86, 140–149. doi:10.1016/j.envint.2015.10.015
Bertram, M. G., Ecker, T. E., Wong, B. B. M., O'Bryan, M. K., Baumgartner, J. B., Martin, J. M., et al. (2018). The Antidepressant Fluoxetine Alters Mechanisms of Pre- and post-copulatory Sexual Selection in the Eastern Mosquitofish (Gambusia holbrooki). Environ. Pollut. 238, 238–247. doi:10.1016/j.envpol.2018.03.006
Brain, R. A., Johnson, D. J., Richards, S. M., Sanderson, H., Sibley, P. K., and Solomon, K. R. (2004). Effects Of 25 Pharmaceutical Compounds To Lemna Gibba Using A Seven-Day Static-Renewal Test. Environ. Toxicol. Chem. 23, 371. doi:10.1897/02-576
Brock, A. L., Rein, A., Polesel, F., Nowak, K. M., Kästner, M., and Trapp, S. (2019). Microbial Turnover of Glyphosate to Biomass: Utilization as Nutrient Source and Formation of AMPA and Biogenic NER in an OECD 308 Test. Environ. Sci. Technol. 53, 5838–5847. doi:10.1021/acs.est.9b01259
Carlsson, C., Johansson, A.-K., Alvan, G., Bergman, K., and Kühler, T. (2006). Are Pharmaceuticals Potent Environmental Pollutants? Sci. Total Environ. 364, 67–87. doi:10.1016/j.scitotenv.2005.06.035
Caruso, B. S. (2001). Regional River Flow, Water Quality, Aquatic Ecological Impacts and Recovery from Drought. Hydrological Sci. J. 46, 677–699. doi:10.1080/02626660109492864
Chen, K., and Zhou, J. L. (2014). Occurrence and Behavior of Antibiotics in Water and Sediments from the Huangpu River, Shanghai, China. Chemosphere 95, 604–612. doi:10.1016/j.chemosphere.2013.09.119
Christensen, A. M., Markussen, B., Baun, A., and Halling-Sørensen, B. (2009). Probabilistic Environmental Risk Characterization of Pharmaceuticals in Sewage Treatment Plant Discharges. Chemosphere 77, 351–358. doi:10.1016/j.chemosphere.2009.07.018
Da Silva, M. D., Peralba, M. D. C. R., and Mattos, M. L. T. (2003). Determinação De Glifosato E Ácido Aminometilfosfônico Em Águas Superficiais Do Arroio Passo Do Pilão. Pes 13, 19–28. doi:10.5380/pes.v13i0.3161
Dias, M., Rocha, R., and Soares, R. (2021). Down the River: Glyphosate Use in Agriculture and Birth Outcomes of Surrounding Populations. Available at: https://www.insper.edu.br/wp-content/uploads/2021/04/Dias-Rocha-Soares-2020.12.30.pdf (Accessed September 20, 2021).
Duong, H. A., Pham, N. H., Nguyen, H. T., Hoang, T. T., Pham, H. V., Pham, V. C., et al. (2008). Occurrence, Fate and Antibiotic Resistance of Fluoroquinolone Antibacterials in Hospital Wastewaters in Hanoi, Vietnam. Chemosphere 72, 968–973. doi:10.1016/j.chemosphere.2008.03.009
Ebert, I., Bachmann, J., Kühnen, U., Küster, A., Kussatz, C., Maletzki, D., et al. (2011). Toxicity of the Fluoroquinolone Antibiotics Enrofloxacin and Ciprofloxacin to Photoautotrophic Aquatic Organisms. Environ. Toxicol. Chem. 30, 2786–2792. doi:10.1002/etc.678
Ferrari, B., Mons, R., Vollat, B., Fraysse, B., Paxéus, N., Lo Giudice, R., et al. (2004). Environmental Risk Assessment of Six Human Pharmaceuticals: Are the Current Environmental Risk Assessment Procedures Sufficient for the protection of the Aquatic Environment? Environ. Toxicol. Chem. 23, 1344. doi:10.1897/03-246
Gabriel, F. A., Silva, A. G., Queiroz, H. M., Ferreira, T. O., Hauser‐Davis, R. A., and Bernardino, A. F. (2020). Ecological Risks of Metal and Metalloid Contamination in the Rio Doce Estuary. Integr. Environ. Assess. Manag. 16, 655–660. doi:10.1002/ieam.4250
Golet, E. M., Xifra, I., Siegrist, H., Alder, A. C., and Giger, W. (2003). Environmental Exposure Assessment of Fluoroquinolone Antibacterial Agents from Sewage to Soil. Environ. Sci. Technol. 37, 3243–3249. doi:10.1021/es0264448
Gomes, G. L. G. C., Carbonari, C. A., Velini, E. D., Trindade, M. L. B., and Silva, J. R. M. (2015). Extraction and Simultaneous Determination of Glyphosate, AMPA and Compounds of the Shikimic Acid Pathway in Plants. Planta Daninha 33, 295–304. doi:10.1590/0100-83582015000200015
Gomes, M. P., Gonçalves, C. A., de Brito, J. C. M., Souza, A. M., da Silva Cruz, F. V., Bicalho, E. M., et al. (2017). Ciprofloxacin Induces Oxidative Stress in Duckweed (Lemna Minor L.): Implications for Energy Metabolism and Antibiotic-Uptake Ability. J. Hazard. Mater. 328, 140–149. doi:10.1016/j.jhazmat.2017.01.005
Gomes, M. P., Moreira Brito, J. C., Cristina Rocha, D., Navarro-Silva, M. A., and Juneau, P. (2020a). Individual and Combined Effects of Amoxicillin, Enrofloxacin, and Oxytetracycline on Lemna Minor Physiology. Ecotoxicology Environ. Saf. 203, 111025. doi:10.1016/j.ecoenv.2020.111025
Gomes, M. P., Rocha, D. C., Moreira de Brito, J. C., Tavares, D. S., Marques, R. Z., Soffiatti, P., et al. (2020b). Emerging Contaminants in Water Used for maize Irrigation: Economic and Food Safety Losses Associated with Ciprofloxacin and Glyphosate. Ecotoxicology Environ. Saf. 196, 110549. doi:10.1016/j.ecoenv.2020.110549
Gomes, M. P., Smedbol, E., Chalifour, A., Hénault-Ethier, L., Labrecque, M., Lepage, L., et al. (2014). Alteration of Plant Physiology by Glyphosate and its By-Product Aminomethylphosphonic Acid: an Overview. J. Exp. Bot. 65, 4691–4703. doi:10.1093/jxb/eru269
Gomes, M. P., Tavares, D. S., Richardi, V. S., Marques, R. Z., Wistuba, N., Moreira de Brito, J. C., et al. (2019). Enrofloxacin and Roundup Interactive Effects on the Aquatic Macrophyte Elodea canadensis Physiology. Environ. Pollut. 249, 453–462. doi:10.1016/j.envpol.2019.03.026
Grandcoin, A., Piel, S., and Baurès, E. (2017). AminoMethylPhosphonic Acid (AMPA) in Natural Waters: Its Sources, Behavior and Environmental Fate. Water Res. 117, 187–197. doi:10.1016/j.watres.2017.03.055
Grill, G., Khan, U., Lehner, B., Nicell, J., and Ariwi, J. (2016). Risk Assessment of Down-The-drain Chemicals at Large Spatial Scales: Model Development and Application to Contaminants Originating from Urban Areas in the Saint Lawrence River Basin. Sci. Total Environ. 541, 825–838. doi:10.1016/j.scitotenv.2015.09.100
Gupta, A., Vyas, R. K., and Gupta, A. B. (2021). Occurrence of Acyclovir in the Aquatic Environment, its Removal and Research Perspectives: A Review. J. Water Process Eng. 39, 101855. doi:10.1016/j.jwpe.2020.101855
IBGE (2020). Censo Demográfico. Available at: https://cidades.ibge.gov.br/brasil/m (Accessed September 20, 2021).
Ikem, A., Lin, C.-H., Broz, B., Kerley, M., and Thi, H. L. (2017). Occurrence of Enrofloxacin in Overflows from Animal Lot and Residential Sewage Lagoons and a Receiving-Stream. Heliyon 3, e00409. doi:10.1016/j.heliyon.2017.e00409
K'oreje, K. O., Vergeynst, L., Ombaka, D., De Wispelaere, P., Okoth, M., Van Langenhove, H., et al. (2016). Occurrence Patterns of Pharmaceutical Residues in Wastewater, Surface Water and Groundwater of Nairobi and Kisumu City, Kenya. Chemosphere 149, 238–244. doi:10.1016/j.chemosphere.2016.01.095
Kent, A. G., Vill, A. C., Shi, Q., Satlin, M. J., and Brito, I. L. (2020). Widespread Transfer of mobile Antibiotic Resistance Genes within Individual Gut Microbiomes Revealed through Bacterial Hi-C. Nat. Commun. 11, 4379. doi:10.1038/s41467-020-18164-7
Kolpin, D. W., Furlong, E. T., Meyer, M. T., Thurman, E. M., Zaugg, S. D., Barber, L. B., et al. (2002). Pharmaceuticals, Hormones, and Other Organic Wastewater Contaminants in U.S. Streams, 1999−2000: A National Reconnaissance. Environ. Sci. Technol. 36, 1202–1211. doi:10.1021/es011055j
Levine, S. L., von Mérey, G., Minderhout, T., Manson, P., and Sutton, P. (2015). Aminomethylphosphonic Acid Has Low Chronic Toxicity to Daphnia magna and Pimephales promelas. Environ. Toxicol. Chem. 34, 1382–1389. doi:10.1002/etc.2940
Locatelli, M. A. F., Sodré, F. F., and Jardim, W. F. (2011). Determination of Antibiotics in Brazilian Surface Waters Using Liquid Chromatography-Electrospray Tandem Mass Spectrometry. Arch. Environ. Contam. Toxicol. 60, 385–393. doi:10.1007/s00244-010-9550-1
Low, K., Chai, L., Lee, C., Zhang, G., Zhang, R., Vahab, V., et al. (2021). Prevalence and Risk Assessment of Antibiotics in Riverine Estuarine Waters of Larut and Sangga Besar River, Perak. J. Ocean. Limnol. 39, 122–134. doi:10.1007/s00343-020-9246-y
Lützhøft, H.-C. H., Halling-Sørensen, B., and Jørgensen, S. E. (1999). Algal Toxicity of Antibacterial Agents Applied in Danish Fish Farming. Arch. Environ. Contam. Toxicol. 36, 1–6. doi:10.1007/s002449900435
Martínez-Carballo, E., González-Barreiro, C., Scharf, S., and Gans, O. (2007). Environmental Monitoring Study of Selected Veterinary Antibiotics in Animal Manure and Soils in Austria. Environ. Pollut. 148, 570–579. doi:10.1016/j.envpol.2006.11.035
Martins, N., Pereira, R., Abrantes, N., Pereira, J., Gonçalves, F., and Marques, C. R. (2012). Ecotoxicological Effects of Ciprofloxacin on Freshwater Species: Data Integration and Derivation of Toxicity Thresholds for Risk Assessment. Ecotoxicology 21, 1167–1176. doi:10.1007/s10646-012-0871-x
Matongo, S., Birungi, G., Moodley, B., and Ndungu, P. (2015). Occurrence of Selected Pharmaceuticals in Water and Sediment of Umgeni River, KwaZulu-Natal, South Africa. Environ. Sci. Pollut. Res. 22, 10298–10308. doi:10.1007/s11356-015-4217-0
Mendes, E. J., Malage, L., Rocha, D. C., Kitamura, R. S. A., Gomes, S. M. A., Navarro-Silva, M. A., et al. (2021). Isolated and Combined Effects of Glyphosate and its By-Product Aminomethylphosphonic Acid on the Physiology and Water Remediation Capacity of Salvinia Molesta. J. Hazard. Mater. 417, 125694. doi:10.1016/j.jhazmat.2021.125694
Mendonça, C. F. R. (2018). Determinação de glifosato e AMPA nas águas superficiais da Bacia do Paraná 3. Master’s thesis. Araraquara, Brazil: Universidade Estadual Paulista “Júlio de Mesquita Filho”.
Minguez, L., Pedelucq, J., Farcy, E., Ballandonne, C., Budzinski, H., and Halm-Lemeille, M.-P. (2016). Toxicities of 48 Pharmaceuticals and Their Freshwater and marine Environmental Assessment in Northwestern France. Environ. Sci. Pollut. Res. 23, 4992–5001. doi:10.1007/s11356-014-3662-5
Módenes, A. N., de Oliveira, A. P., Espinoza-Quiñones, F. R., Trigueros, D. E. G., Kroumov, A. D., and Bergamasco, R. (2017). Study of the Involved Sorption Mechanisms of Cr(VI) and Cr(III) Species onto Dried Salvinia Auriculata Biomass. Chemosphere 172, 373–383. doi:10.1016/j.chemosphere.2017.01.038
Moncmanová, A. (2007). “Environmental Factors that Influence the Deterioration of Materials,” in Environmental Deterioration of Materials (Lisbon, Portugal: WIT Press), 1–25. doi:10.2495/978-1-84564-032-3/01
Moore, D. R. J., Breton, R. L., and MacDonald, D. B. (2003). A Comparison of Model Performance for Six Quantitative Structure-Activity Relationship Packages that Predict Acute Toxicity to Fish. Environ. Toxicol. Chem. 22, 1799. doi:10.1897/00-361
Mucsi, I., Gyulai, Z., and Béládi, I. (1992). Combined Effects of Flavonoids and Acyclovir against Herpesviruses in Cell Cultures. Acta Microbiol. Hung. 39, 137–147.
Nentwig, G. (2007). Effects of Pharmaceuticals on Aquatic Invertebrates. Part II: The Antidepressant Drug Fluoxetine. Arch. Environ. Contam. Toxicol. 52, 163–170. doi:10.1007/s00244-005-7190-7
Ngumba, E., Gachanja, A., and Tuhkanen, T. (2016). Occurrence of Selected Antibiotics and Antiretroviral Drugs in Nairobi River Basin, Kenya. Sci. Total Environ. 539, 206–213. doi:10.1016/j.scitotenv.2015.08.139
Nguyen Dang Giang, C., Sebesvari, Z., Renaud, F., Rosendahl, I., Hoang Minh, Q., and Amelung, W. (2015). Occurrence and Dissipation of the Antibiotics Sulfamethoxazole, Sulfadiazine, Trimethoprim, and Enrofloxacin in the Mekong Delta, Vietnam. PLoS One 10, e0131855–24. doi:10.1371/journal.pone.0131855
OʼBrien, J. J., and Campoli-Richards, D. M. (1989). Acyclovir. Drugs 37, 233–309. doi:10.2165/00003495-198937030-00002
Oluwatosin, O., Adekunle, B., Obih, U., and Arne, H. (2016). Quantification of Pharmaceutical Residues in Wastewater Impacted Surface Waters and Sewage Sludge from Lagos, Nigeria. J. Environ. Chem. Ecotoxicol. 8, 14–24. doi:10.5897/JECE2015.0364
Porto, M. F. S. (2016). A tragédia da mineração e Do desenvolvimento no Brasil : desafios para a saúde coletiva. Cad. Saude Publica 32, 1–3. doi:10.1590/0102-311x00211015
Prasse, C., Schlüsener, M. P., Schulz, R., and Ternes, T. A. (2010). Antiviral Drugs in Wastewater and Surface Waters: A New Pharmaceutical Class of Environmental Relevance? Environ. Sci. Technol. 44, 1728–1735. doi:10.1021/es903216p
Queiroz, H. M., Nóbrega, G. N., Ferreira, T. O., Almeida, L. S., Romero, T. B., Santaella, S. T., et al. (2018). The Samarco Mine Tailing Disaster: A Possible Time-Bomb for Heavy Metals Contamination? Sci. Total Environ. 637-638, 498–506. doi:10.1016/j.scitotenv.2018.04.370
Quintana, M. I., Andreoli, S. B., Peluffo, M. P., Ribeiro, W. S., Feijo, M. M., Bressan, R. A., et al. (2015). Psychotropic Drug Use in São Paulo, Brazil - an Epidemiological Survey. PLoS One 10, e0135059. doi:10.1371/journal.pone.0135059
Rodriguez-Mozaz, S., Vaz-Moreira, I., Varela Della Giustina, S., Llorca, M., Barceló, D., Schubert, S., et al. (2020). Antibiotic Residues in Final Effluents of European Wastewater Treatment Plants and Their Impact on the Aquatic Environment. Environ. Int. 140, 105733. doi:10.1016/j.envint.2020.105733
Rusu, A., Hancu, G., and Uivaroşi, V. (2015). Fluoroquinolone Pollution of Food, Water and Soil, and Bacterial Resistance. Environ. Chem. Lett. 13, 21–36. doi:10.1007/s10311-014-0481-3
Smedbol, É., Gomes, M. P., Paquet, S., Labrecque, M., Lepage, L., Lucotte, M., et al. (2018). Effects of Low Concentrations of Glyphosate-Based Herbicide Factor 540 on an Agricultural Stream Freshwater Phytoplankton Community. Chemosphere 192, 133–141. doi:10.1016/j.chemosphere.2017.10.128
Thai, P. K., Ky, L. X., Binh, V. N., Nhung, P. H., Nhan, P. T., Hieu, N. Q., et al. (2018). Occurrence of Antibiotic Residues and Antibiotic-Resistant Bacteria in Effluents of Pharmaceutical Manufacturers and Other Sources Around Hanoi, Vietnam. Sci. Total Environ. 645, 393–400. doi:10.1016/j.scitotenv.2018.07.126
Walters, E., McClellan, K., and Halden, R. U. (2010). Occurrence and Loss over Three Years of 72 Pharmaceuticals and Personal Care Products from Biosolids-Soil Mixtures in Outdoor Mesocosms. Water Res. 44, 6011–6020. doi:10.1016/j.watres.2010.07.051
Wu, D., Huang, Z., Yang, K., Graham, D., and Xie, B. (2015). Relationships between Antibiotics and Antibiotic Resistance Gene Levels in Municipal Solid Waste Leachates in Shanghai, China. Environ. Sci. Technol. 49, 4122–4128. doi:10.1021/es506081z
Keywords: antimicrobials, herbicide, aquatic toxicology, ecological risk assesment, glyphosate
Citation: Gomes MP, Brito JCM, Vieira F, Kitamura RSA and Juneau P (2022) Emerging Contaminants in Streams of Doce River Watershed, Minas Gerais, Brazil. Front. Environ. Sci. 9:801599. doi: 10.3389/fenvs.2021.801599
Received: 25 October 2021; Accepted: 10 December 2021;
Published: 12 January 2022.
Edited by:
Erick Bandala, Desert Research Institute (DRI), United StatesReviewed by:
Oscar Manuel Rodriguez, Centro de Innovación Aplicada en Tecnologías Competitivas (CIATEC), MexicoYeongkwon Son, Desert Research Institute (DRI), United States
Copyright © 2022 Gomes, Brito, Vieira, Kitamura and Juneau. This is an open-access article distributed under the terms of the Creative Commons Attribution License (CC BY). The use, distribution or reproduction in other forums is permitted, provided the original author(s) and the copyright owner(s) are credited and that the original publication in this journal is cited, in accordance with accepted academic practice. No use, distribution or reproduction is permitted which does not comply with these terms.
*Correspondence: Marcelo Pedrosa Gomes, bWFyY2Vsby5nb21lc0B1ZnByLmJy