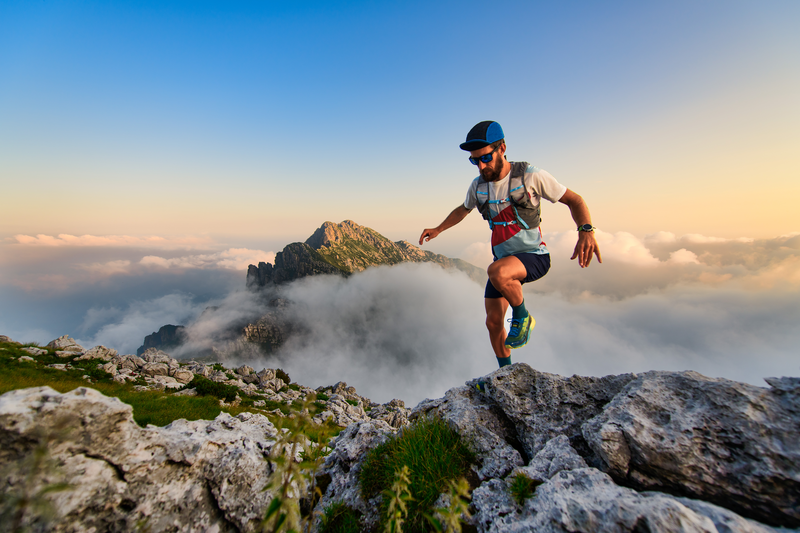
94% of researchers rate our articles as excellent or good
Learn more about the work of our research integrity team to safeguard the quality of each article we publish.
Find out more
ORIGINAL RESEARCH article
Front. Environ. Sci. , 21 December 2021
Sec. Water and Wastewater Management
Volume 9 - 2021 | https://doi.org/10.3389/fenvs.2021.799264
This article is part of the Research Topic Environmental Monitoring and Remediation Using Microbiotechnology View all 19 articles
Zero valent iron (ZVI) particles were embedded into porous materials to avoid aggregation and separation problems in the controlled synthesis process. To investigate the adsorption mechanism of crystal violet and methylene blue, activated carbon (AC) and AC-based ZVI extraction by solid-phase and liquid-phase reduced approaches was conducted. Characterization methods of specific surface area, scanning electron microscopy (SEM), and x-ray diffractograms (XRD) were used to elucidate the structure of adsorbents, and the adsorption capacities of crystal violet and methylene blue were obtained under experimental conditions of various pH values (2.0–10.0), adsorption times (0–72 h), and temperatures (30–50°C). The adsorption of crystal violet/methylene blue was controlled by both chemisorption and reduction. The adsorption processes were fitted to a pseudo-second-order kinetic model, and that of reduction kinetics was suitable to pseudo-first-order kinetic model. The thermodynamic study revealed that the adsorption of crystal violet and methylene blue was endothermic and spontaneous, and the adsorption isotherms fitted well to the Langmuir model. Different adsorption capacities of crystal violet and methylene blue on various adsorbents were found, indicating that both the properties of adsorbents (pore size, specific surface area, and chemical functional groups) and the structures of adsorbates had significant effect on the removal of dye molecules.
Water pollution, a known universal crisis, might cause a reduction in the population, or extinction, of living things; reduce the value of environmental resources; and pose a threat to ecological balance (Iqbal et al., 2019; Efimov et al., 2019; Li et al., 2020). The release of dye in wastewater generated from different industries has been considered to be an important source of water pollution (Bilal et al., 2016a; Nouren et al., 2017). These dyes pose a serious threat to the ecological environment since they are extremely stable and non-biodegradable, and their accumulation always leads to poor oxygenation of the water environment by preventing the photosynthesis of photosynthetic organisms (Spadaro et al., 1992; Xu et al., 2018). Moreover, most dyes are toxic, mutagenic, and carcinogenic, leading to serious harm for aquatic animals and human health (Bilal et al., 2016a; Bilal et al., 2016b; Ramamoorthy et al., 2020). Therefore, it is quite significant to carry out the remediation of dyes in wastewater to clean the water environment and safeguard human health (Abbas et al., 2018; Amin et al., 2020).
To impede the discharge and pollution of dye in wastewater, plenty of treatment methods have been continuously improved to remove these dyes from wastewater (Chen et al., 2017; Saber-Samandari et al., 2017). Considering economics, applicability, and removal efficacy, adsorption is suggested as the most popular technique (Fernandes et al., 2010; Xu et al., 2014; Rasalingam et al., 2015), in which the selection of adsorbents is the key factor affecting removal efficiency (Fernandes et al., 2010; Zhang et al., 2020b; Liu et al., 2014). Activated carbon (AC) is known as the most effective adsorbent; however, the expensive cost of AC restricts its wide utilization. Recently, a variety of bio-based activated carbons derived from agricultural wastes such as fruit peel, crop straw, coconut shell, and vegetable residues have been synthesized to make the adsorption process more feasible and cost-effective (Mishra et al., 2021). Moreover, iron-based porous materials have been developed to be effective adsorbents for toxic dye removal from wastewater (Zhang et al., 2010; Wang et al., 2012), mainly because of the unique redox potential of zero valent iron (ZVI) combined with the high surface area and large reaction sites of porous adsorbents (Kerkez et al., 2014). Previous studies demonstrated that iron-modified montmorillonite could effectively adsorb crystal violet (CV) (Guz et al., 2014), and iron nanoparticles decorated onto three-dimensional graphene could rapidly and efficiently degrade azo dye (Wang et al., 2015).
However, the application of ZVI in actual water treatment is subject to certain restrictions, because it tends to agglomerate and is easily oxidized (Liu et al., 2007; Fan et al., 2016). Therefore, many studies have been initiated to search for different matrices to overcome the iron particle aggregation, such as adding iron on montmorillonite for the adsorption of toxic cationic dyes (Wang et al., 2017; Liu et al., 2018) and adding iron nanoparticles onto three-dimensional graphene to degrade azo dyes (Liu et al., 2018). It could be found that two different approaches, namely, solid-phase and liquid-phase iron direct reduction technology (Liu et al., 2007; Wang et al., 2017; Liu et al., 2018), are the main approaches to prepare ZVI covered on adsorbents for effectively removing toxic dyes in wastewater. Using liquid-phase iron direct reduction technology, many materials were used as matrices to fix nZVI including kaolinite (Liu et al., 2007), activated carbon (Liu et al., 2018), graphene (Wang et al., 2017), and palygorskite (Ngulube et al., 2019), which exhibited an excellent dye removal efficiency. Based on solid-phase iron direct reduction technology, different kinds of iron-based adsorbents with the ZVI particles embedded into matrixes could be prepared, overcoming the lack of instability of ZVI synthesized in the liquid-phase direct reduction approach (Wang et al., 2017; Zheng et al., 2019).
In order to research the removal efficiency and purification mechanism of dyes onto AC embedded with ZVI extracted by solid/liquid-phase direct reduction approach, a series of AC supported ZVI adsorbents were synthesized and used to remove CV and methylene blue (MB) at various pH values, adsorption times, and temperatures. CV and MB were chosen as the representation of dyes because of the wide area of applications and similar molecular weights/different molecular structures. In this study, palm kernel shells as agricultural wastes were used to prepare AC adsorbents owing to their low ash content, high in carbon and volatile content (Ajeng et al., 2021). The higher heating value of palm kernel shells was beneficial for the solid-phase iron direct reduction technology (Wang et al., 2017; Bazargan et al., 2018). Thus, AC adsorbents were used as reductants to prepare the ZVI in the solid-phase iron direct reduction process and as matrices to fix nZVI in the liquid-phase iron direct reduction process. Adsorption data were interpreted by using adsorption kinetics, adsorption isotherms, and thermodynamic models to calculate the adsorption capacities and thermodynamic parameters.
Palm kernel shell (C 50.2%, H 6.2%, O 40.8%, and N 0.3%), and iron ore tailings (Fe2O3 27.47%, Al2O3 6.44%, SiO2 22.44%, CaO 1.95%, MgO 3.07%, and S2O3 4.61%) were sieved through 500-mesh screens and dried in an oven at 80°C for 24 h. Analytical grade dye molecules (CV and MB), iron chloride (FeCl3·6H2O), ethanol, sodium borohydride (NaBH4), and sodium hydroxide (NaOH) were purchased from Beijing Chemical Reagents Company (Beijing, China). Water used in this experiment was deionized water.
AC from palm kernel shell (PAC, 1#) and ZVI adsorbents produced through the solid-phase direct reduction approach (PAC-mZVI, 2#) were prepared as shown in Figure 1. In the preparation of PAC, palm kernel shell was heated in the absence of oxygen at a heating rate of 10°C min−1, a heating temperature of 800°C, and a calcining time of 40 min, to maintain its weight and produce a loose porous structure. Schematic of the preparation steps is shown in Figure 1.
The solid-phase direct reduction approach was chosen to produce ZVI to prepare PAC-mZVI (2#), in which iron ore tailings were reduced by palm kernel shell to ZVI during the sintering process at high temperature, as shown in the chemical reaction below:
According to a previous study (Wang et al., 2017), the liquid-phase direct reduction approach was chosen to produce nanoscale ZVI to prepare PAC-nZVI (3#) adsorbents, in which Fe3+ was reduced by NaBH4 to ZVI on the surface of PAC as shown in the chemical reaction below:
Iron chloride solution (FeCl3) saturated PAC adsorbents and sodium borohydride solution (NaBH4) were shaken at 120 r min−1 under a temperature of 30°C to reduce Fe3+ to ZVI. The optimal experimental factors influencing the amount of ZVI covered on the surface of PAC were selected at a shaking time of 1 h, a molar ratio of Fe3+:BH4−at 1:3, and a mass ratio of Fe3+:PAC adsorbent at 1:5.
The surface area and pore size were detected according to nitrogen adsorption–desorption isotherms using the Autsorb-1 (Quantachrome, United States). The morphological characteristics and chemical components of these adsorbents were determined using scanning electron microscopy (SEM, JSM-6610 LV, Jeol, Japan) and x-ray diffractograms (XRD, 08 Advance Davinci, Bruker, Germany), respectively. Zeta potentials were determined by a Zeta Probe apparatus (Colloidal-Dynamics, United States).
Dye solution (100 ml) with an initial concentration of 50–1,000 mg L−1 and an adsorbent dose of 0.6 g were added into glass conical flasks to carry out the adsorption experiments at the constant speed of 120 rpm. The desired pH value was adjusted at the range of 2–10. Adsorption kinetic studies were carried out at various adsorption times (0–72 h). The removal efficiencies (R) and adsorption capacities (Qt) were calculated by Eqs 1, 2 (Mwamulima et al., 2018). Adsorption isotherm studies were conducted with different initial concentrations of dye solution (50, 100, 200, 400, 600, 800, and 1,000 mg L−1) at temperatures of 30, 40, and 50°C. The concentrations of residual CV and MB in aqueous solution were detected by UV spectrophotometry.
where C0 (mg L−1) is the initial concentration, Ct (mg L−1) is the concentration at time t, V (L) is the volume of the solution, and m (g) is the adsorbent weight.
Nitrogen adsorption method was used to determine the pore diameter, pore volume, and surface area of adsorbents in Table 1. Compared with palm kernel shell, PAC, PAC-mZVI, and PAC-nZVI had a much larger surface area and micropore area, which might lead to the deduction that abundant micropores were generated in the process of biomass pyrolysis chemical reaction of palm kernel shell at high temperature (Guo et al., 2016). Moreover, the calcining temperature in the preparation of PAC-mZVI adsorbents had a significant impact on the reduction process, which largely determined the micropore area and pore size. The surface area of PAC-nZVI adsorbents was higher than the other three adsorbents, showing that the adsorbents prepared by the liquid-phase reduction approach had the largest BET surface area.
SEM and EDX images of PAC, PAC-mZVI, and PAC-nZVI are shown in Figure 2. It could be clearly found that the surface of these adsorbents was rough; meanwhile, numerous pores existed in these adsorbents. This could be attributed to the fact that the organic matter in palm kernel shell generated volatile CO, CO2, and hydrogen during the sintering process (Guo et al., 2016). These volatile gases escaped from the inside of adsorbents; then, the pores were formed in this process. Moreover, PAC-nZVI adsorbents contained more pores compared with PAC-mZVI adsorbents, which might be attributed to the cementation effect of ZVI particle in the solid-phase reduction process (Wang et al., 2017). Combined with EDX analysis, it could be found that ZVI was generated in the solid-phase reduction reaction with the reductants of palm kernel shell and liquid-phase reduction reaction process in aqueous solution. As shown in Figure 2C, newly formed ZVI particles in the liquid-phase reduction reaction process were uniformly dispersed throughout the matrix, owing to the advantages of the liquid-phase iron reduction approach.
FIGURE 2. SEM images of (A1) PAC, (B1) PAC-mZVI, and (C1) PAC-nZVI and EDX images of (A2) PAC, (B2) PAC-mZVI, and (C2) PAC-nZVI.
XRD analyses were chosen to identify various phases and phase transformations for PAC, PAC-mZVI, and PAC-nZVI adsorbents. As depicted in Figure 3A, the main component of PAC adsorbents was carbon. As for PAC-mZVI in Figure 3B, hematite (Fe2O3) and ZVI were found as well as the carbon that PAC contained, indicating that palm kernel shells were translated to PAC, and iron ore tailings were partly reduced to ZVI in the solid reduction process with palm kernel shell as reductants (Man et al., 2014; Wang et al., 2017). As shown in Figure 3C, PAC-nZVI adsorbents mainly contained carbon and ZVI. Compared with PAC-mZVI adsorbents, ZVI was the unique form of iron element in PFB-nZVI adsorbents, confirming that Fe3+ was directly reduced to ZVI covered on porous adsorbents in liquid-phase reduction approach (Liu et al., 2018; Mwamulima et al., 2018).
FIGURE 3. X-ray diffractograms of three different adsorbents. (A) PAC, (B) PAC-mZVI, and (C) PAC-nZVI.
Removal efficiencies of CV and MB by PAC, PAC-mZVI, and PAC-nZVI adsorbents were researched at different pH values, and effects of pH value on adsorption capacity are shown in Figure 4. The adsorption process was significantly affected by the changing of pH value, mainly because the existence forms of functional groups on the adsorbents were changed under different acid and alkali conditions (Wang et al., 2017; Liu et al., 2018; Mwamulima et al., 2018). As shown in Figure 4, removal efficiencies of dye molecules increased with the increase of pH value at the initial stage, then gradually decreased after the maximum. Meanwhile, we could find that removal efficiencies of CV by these three different adsorbents reached the maximum when pH value was approximately at 6.0, 6.0 and 8.0, respectively.
FIGURE 4. Effects of pH on the removal of CV (A) and MB (B) by PAC, PAC-mZVI, and PAC-nZVI adsorbents.
This phenomenon could be attributed to the influence of structure characteristics and the point of zero charge (pHpzc) of adsorbents. As reported in previous literature, the surface of adsorbent had a positive charge at pH < pHpzc, negative charge at pH > pHpzc, and net zero charge at pH = pHpzc, respectively (Hammed et al., 2016). In this study, pHpzc of PAC, PAC-mZVI, and PAC-nZVI adsorbents was approximately 5.5, 7.0, and 8.0, respectively. At lower pH, excess H+ might compete with CV/MB molecule for adsorption sites, inhibiting the adsorption process of CV and MB onto adsorbents. Moreover, electrostatic repulsion existed between adsorbents and dye molecules, which would be also largely adverse to the adsorption of CV/MB onto adsorbents. Generally, cationic dyes were quite easily absorbed onto adsorbents with negative charges owing to the attraction of positive and negative charges. It could be concluded that excess OH− at higher pH promoted the formation of iron hydroxide, which could occupy the reactive sites on adsorbents to decrease the reduction reaction of CV/MB in this experiment (Chen et al., 2013).
The adsorption capacities of CV and MB at 30°C are shown in Figure 5. The adsorption processes of CV/MB were generally fast at the initial stage and then leveled off with time, which was attributed to the fact that huge amounts of active vacant sites on these adsorbents were gradually occupied. As depicted in Figures 5A,B, the adsorption of CV and MB on PAC-nZVI reached equilibrium at approximately 50 h, which was slightly longer than other adsorbents. The reason was that the specific surface area of PAC-nZVI adsorbents was larger than that of other adsorbents and a large number of pores existed in the interior of the adsorbents. Moreover, the adsorption capacity of PAC-nZVI adsorbents for CV and MB arrived at 97 mg g−1 and 43 mg g−1, which was higher than that of other adsorbents. This could be attributed to the fact that both adsorption and reduction reactions were included in the removal processes of CV and MB by these three different adsorbents. PAC-nZVI adsorbents contained higher concentration of ZVI compared with that of other adsorbents, generating more active sites to promote the increase in adsorption capacity. Besides, it could be found that the absorption of MB reached the equilibrium faster than that of CV, which could be attributed to the fact that the triangular shape of CV was not beneficial when entering smaller pores compared with the chain shape of MB.
FIGURE 5. Effects of contact time on the removal of CV (A) and MB (B) by PAC, PAC-mZVI, and PAC-nZVI adsorbents.
Changes in adsorption capacity of CV and MB under different temperatures are depicted in Figure 6. It could be found that the adsorption capacity of CV/MB increased with the increase of temperature, indicating that the adsorption processes of dye molecules were endothermic reaction. Moreover, the phenomenon could also be explained by the fact that high temperature could promote the increase in pore size and surface area to enlarge the adsorption capacity (Liu et al., 2018). When the temperature arrived at 50°C, the adsorption capacity of PAC-nZVI for CV and MB arrived at approximately 110 and 73 mg g−1, respectively. Moreover, the change rate of adsorption capacity with time increased as the reaction temperature increased. It might be attributed to the increase in the mobility of dye molecules in aqueous solution and the formation of reactive sites on the interface of adsorbents under higher temperature (Liu et al., 2018; Mwamulima et al., 2018).
FIGURE 6. Effects of temperature on the removal of CV and MB by PAC, PAC-mZVI, and PAC-nZVI adsorbents.
Lagergren pseudo-first-order rate (Eq. 3) (Lagergren, 1898), pseudo-second-order rate (Eq. 4) (Ho and McKay, 1999), and Weber-Morris diffusion model (Eq. 5) (Weber and Morris, 1963) were chosen to explain the kinetics of CV and MB onto these three adsorbents, which could be described as:
where qe (mg g−1) and qt (mg g−1) are the adsorption capacities at equilibrium and time t, respectively. k1 (1/h), k2 (1/h), kint, and b are the constants in pseudo-first-order, pseudo-second-order, and intraparticle diffusion, respectively.
The kinetic parameters (r2, Qe, K1, and K2 values) are shown in Table 2. According to the previous literature, the optimal fitting model could be selected based on the parameter of r2 value (Wang et al., 2017). Thus, it could be found that adsorption of CV/MB onto these three adsorbents fitted the pseudo-second-order rate equations well due to the relatively better r2 values compared with that of the pseudo-first-order kinetic model. Based on the qe values calculated from the pseudo-second-order kinetics model, PAC-nZVI adsorbent showed the highest adsorption capacities at equilibrium compared with other adsorbents. These values of qe had quite good agreement with experimental results. Thus, we could deduce that chemisorption was the rate-controlling step in the removal process of CV/MB onto PAC and PAC-based ZVI adsorbents (Liu et al., 2018; Mwamulima et al., 2018).
TABLE 2. Kinetic parameters for the adsorption of CV and MB dye on PAC and PAC-based ZVI adsorbents.
In order to further analyze the adsorption process, the Weber-Morris diffusion model was chosen to discuss the relationship between qt and t0.5 based on the intraparticle diffusion theory. If the intraparticle diffusion was a rate-limiting step, the plot of qt against t0.5 would yield a straight line with a slope of kint. As depicted in Figures 7A, B, the linear plot of qt vs. t0.5 yielded a straight line, indicating that intraparticle diffusion was a rate-limiting step. Moreover, the linear plot did not pass through the origin, illustrating that the molecular diffusion controlled the adsorption reaction rate to some extent. Thus, it could be concluded that two processes, namely, chemisorption and diffusion, affected the removal process of these dye molecules.
FIGURE 7. Intraparticle model for the adsorption for the adsorption of CV and MB onto PAC, PAC-mZVI, and PAC-nZVI adsorbents.
Based on the above research results, adsorption reaction of CV/MB by PAC and PAC-based ZVI adsorbents included adsorption and chemical reduction (Chen et al., 2013; Wang et al., 2017; Ezzatahmadi et al., 2017). Pseudo-first-order and -second-order reduction kinetic models in Eqs 6, 7 were selected to study the reduction process (Kerkez et al., 2014).
where C0 (mg g−1) and Ct (mg g−1) are the concentration at the initial time and at time t; kobs (h−1) and k2 (h−1) are the rate constant of pseudo-first-order and second-order reaction, respectively.
Reduction kinetics parameters are shown in Table 3. Based on the reduction kinetics parameters, the pseudo-first-order kinetics model provided a better match of the experiment results of CV/MB dye, because of the relatively better r2 values compared with that of the pseudo-second-order kinetic model. This indicated that CV/MB reacted with ZVI in the interface of the adsorbents through a solid–liquid reaction (Wang et al., 2017; Liu et al., 2018). Moreover, the reduction processes of CV/MB by these three adsorbents were endothermic, considering the reduction kinetic rate (kobs) increased with the increase of temperature from 30 to 50°C. This was due to the fact that higher temperature could promote dye molecule transfer from aqueous solution to the surface of adsorbents (Adesemuyi et al., 2020).
Taking PAC-mZVI adsorbent for example, kobs increased from 0.0716 to 0.0827 h−1 when the temperature increased from 30 to 50°C. It was depicted that the Kobs value of PAC-mZVI adsorbents was slightly higher, indicating that the chemical reduction reaction happened faster than that of the other two adsorbents. Based on the parameter values shown in Tables 2, 3, it could be deduced that both physical adsorption and chemical reaction might handle the removal processes of CV/MB onto these adsorbents. Meanwhile, we could also find that the adsorption process of CV/MB dye was slightly faster than the reduction process by the comparison of the parameters of K1 and Kobs (Chen et al., 2013).
Adsorption isotherms could reveal the relationship of adsorption capacity of adsorbents and the concentration of adsorbates in aqueous solution, when the adsorption process reached equilibrium at a certain temperature. In this study, the Langmuir equation in Eq. 8 (Langmuir, 1918) and the Freundlich equation in Eq. 9 (Freundlich, 1906) were chosen to study the adsorption isotherms. The Langmuir equation supposed that adsorption was limited to monolayer coverage, while Freundlich isotherms assumed that the adsorption surface was heterogeneous and multilayer adsorption might be possible.
where Ce (mg L−1) is the concentration of adsorbates at equilibrium, qmax (mg g−1) is the maximum adsorption capacity, and KF and n are constants related to adsorption capacity and intensity, respectively. Based on Eqs 8, 9, it could be found that the near-perfect linear fitting of 1/qe vs. 1/Ce belonged to the Langmuir model, while that of logqe versus logCe obeyed the Freundlich model. If the value of 1/n was less than 1, it might be attributed to the favorability of adsorption (Inbaraj and Chen., 2011; Fan et al., 2017).
The adsorption isotherms are depicted in Figure 8, and relevant adsorption isotherm parameters (b, Qm, 1/n, Kf) are shown in Table 4. Considering the relatively better r2 values, we could find that adsorption isotherms of CV/MB matched well with the Langmuir model. Consequently, adsorption processes of CV/MB onto PAC and PAC-based ZVI adsorbents were homogeneous and occurred at a monolayer region on the adsorbents. For the adsorption of CV dye, the Qm value of PAC-nZVI adsorbents was the greatest compared with other adsorbents. As for MB, the Qm value of PAC-nZVI adsorbents was 68, 79, and 99 mg g−1 at 30, 40, and 50°C, depicting the greatest adsorption capacity. Moreover, RL values of CV and MB were less than 1, confirming that it was favorable for CV and MB adsorbed onto these adsorbents under this reaction condition (Inbaraj and Chen., 2011).
FIGURE 8. Adsorption isotherms of CV and MB adsorbed onto PAC and PAC-based ZVI adsorbents, (A1) CV by PAC; (A2) MB by PAC; (B1) CV by PAC-mZVI; (B2) MB by PAC-mZVI; (C1) CV by PAC-nZVI; (C2) MB by PAC-nZVI.
To analyze the effects of temperature on the adsorption, thermodynamic parameters including ΔH, ΔS, and ΔG were calculated based on the adsorption isotherms. The specific calculation method is shown as Eqs 10, 11, respectively.
The parameter values of ΔH, ΔS, and ΔG are shown in Table 5. As noted, ΔG values of three different adsorbents decreased with the increase in temperature, indicating that the adsorption of CV/MB happened spontaneously. Meanwhile, ΔH values were all greater than zero, attributing to the fact that CV/MB adsorbed on adsorbents showed endothermic reactions. Moreover, values of ΔS in the adsorption processes were positive, which could further reveal the increasing randomness at the interface of solid/solution (Zhang et al., 2020a).
TABLE 5. Thermodynamic parameters for the adsorption of CV and MB on PAC and PAC-based ZVI adsorbents.
CV and MB in water solution were firstly adsorbed on the surface of adsorbents, and then transported into the interior pores of adsorbents through pore diffusion. During the diffusion process, both the structural features of adsorbents and adsorbates had significant effects on the adsorption of dye molecules. When the molecular size of adsorbents was smaller than pore size, adsorbates could enter the internal pores for further adsorption action. Since the molecular structures of MB (line-shaped) and CV (fork-shaped) were different, the co-influence of the pore size of adsorbents and the structure of adsorbates on the removal processes is exhibited in Figure 9A.
CV and MB in the internal pores of adsorbents were finally adsorbed on the active sites of adsorbents, in which CV/MB was partly reduced by ZVI on the adsorbents. According to our previous research (Wang et al., 2017; Liu et al., 2018), CV was cleaved to two parts to generate new products via electrochemical reduction; however, MB reacted to its reduction state. The adsorption capacities of CV on these adsorbents were largely higher than that of MB as demonstrated in the experimental results, which might be attributed to the new products reduced from CV with smaller molecular size. Moreover, it could be deduced that specific surface area was also an important factor in the adsorption processes. The adsorption sites on the adsorbents increased with the increase in specific surface area. Based on the above discussion, the possible removal mechanisms of CV/MB from aqueous solutions on PAC and PAC-based ZVI adsorbents are proposed in Figure 9.
Adsorption of CV/MB on PAC and PAC-based ZVI adsorbents indicated that ZVI could efficiently remove cationic dyes from aqueous solution. The removal processes of CV/MB on adsorbents were significantly affected by the changing pH value, reaction time, and temperature. The adsorption capacities of CV/MB were fast in the initial stages and finally leveled off with time. The adsorption capacity increased with the increase in temperature. The adsorption processes of CV and MB onto these adsorbents all fitted well with the pseudo-second-order rate equations, and chemisorption was the rate-controlling step. The pseudo-first-order reduction kinetic model provided a better match of the adsorption process, indicating that dye molecules reacted with ZVI in the interface of adsorbents through a solid–liquid reaction, and the reduction process was endothermic. The adsorption isotherms obeyed the Langmuir model, indicating that CV/MB onto these adsorbents were homogeneous and occurred within a monolayer region on the surface of adsorbents. Compared with solid-phase iron direct reduction technology, PAC-nZVI adsorbents prepared by liquid-phase iron direct reduction technology showed higher adsorption capacity owing to the larger BET surface area and more ZVI particles. Furthermore, it was found that the properties of adsorbents (pore size, specific surface area, and chemical functional groups) and the structure characteristics of adsorbates had significant effects on the adsorption efficiency of dye molecules.
The original contributions presented in the study are included in the article/supplementary material. Further inquiries can be directed to the corresponding author.
YW compiled and analyzed output data, and designed and wrote the first version of the manuscript. TC designed the study and managed the funding acquisition. XZ and TM conducted the experiments. All authors edited and approved the final version of this manuscript.
This study was financially supported by China Postdoctoral Science Foundation (grant number 2017M622301).
The authors declare that the research was conducted in the absence of any commercial or financial relationships that could be construed as a potential conflict of interest.
All claims expressed in this article are solely those of the authors and do not necessarily represent those of their affiliated organizations, or those of the publisher, the editors, and the reviewers. Any product that may be evaluated in this article, or claim that may be made by its manufacturer, is not guaranteed or endorsed by the publisher.
The authors would like to thank Gang Zhou’s team at Shandong University of Science and Technology who helped with the sampling and analysis.
Abbas, M., Adil, M., Ehtisham-ul-Haque, S., Munir, B., Yameen, M., Ghaffar, A., et al. (2018). Vibrio Fischeri Bioluminescence Inhibition Assay for Ecotoxicity Assessment: A Review. Sci. Total Environ. 626, 1295–1309. doi:10.1016/j.scitotenv.2018.01.066
Adesemuyi, M. F., Adebayo, M. A., Akinola, A. O., Olasehinde, E. F., Adewole, K. A., and Lajide, L. (2020). Preparation and Characterisation of Biochars from Elephant Grass and Their Utilisation for Aqueous Nitrate Removal: Effect of Pyrolysis Temperature. J. Environ. Chem. Eng. 8, 104507. doi:10.1016/j.jece.2020.104507
Ajeng, A. A., Abdullah, R., Junia, A., Lau, B. F., Ling, T. C., and Ismail, S. (2021). Evaluation of palm Kernel Shell Biochar for the Adsorption of Bacillus Cereus. Phys. Scr. 96, 105004. doi:10.1088/1402-4896/ac0f3b
Amin, M., Chetpattananondh, P., and Khan, M. N. (2020). Ultrasound Assisted Adsorption of Reactive Dye-145 by Biochars from marine Chlorella Sp. Extracted Solid Waste Pyrolyzed at Various Temperatures. J. Environ. Chem. Eng. 8, 104403. doi:10.1016/j.jece.2020.104403
Bazargan, A., Rough, S. L., and McKay, G. (2018). Fine Tuning of Process Parameters for Improving Briquette Production from palm Kernel Shell Gasification Waste. Environ. Tech. 39, 931–938. doi:10.1080/09593330.2017.1317835
Bilal, M., Iqbal, M., Hu, H., and Zhang, X. (2016a). Mutagenicity and Cytotoxicity Assessment of Biodegraded Textile Effluent by Ca-Alginate Encapsulated Manganese Peroxidase. Biochem. Eng. J. 109, 153–161. doi:10.1016/j.bej.2016.01.020
Bilal, M., Iqbal, M., Hu, H., and Zhang, X. (2016b). Mutagenicity, Cytotoxicity and Phytotoxicity Evaluation of Biodegraded Textile Effluent by Fungal Ligninolytic Enzymes. Water Sci. Technol. 73, 2332–2344. doi:10.2166/wst.2016.082
Chen, J., Leng, J., Yang, X., Liao, L., Liu, L., and Xiao, A. (2017). Enhanced Performance of Magnetic Graphene Oxide-Immobilized Laccase and its Application for the Decolorization of Dyes. Molecules 22, 221. doi:10.3390/molecules22020221
Chen, Z., Wang, T., Jin, X., Chen, Z., Megharaj, M., and Naidu, R. (2013). Multifunctional Kaolinite-Supported Nanoscale Zero-Valent Iron Used for the Adsorption and Degradation of crystal Violet in Aqueous Solution. J. Colloid Interf. Sci. 398, 59–66. doi:10.1016/j.jcis.2013.02.020
Efimov, M. N., Vasilev, A. A., Muratov, D. G., Baranchikov, A. E., and Karpacheva, G. P. (2019). IR Radiation Assisted Preparation of KOH-Activated Polymer-Derived Carbon for Methylene Blue Adsorption. J. Environ. Chem. Eng. 7, 103514. doi:10.1016/j.jece.2019.103514
Ezzatahmadi, N., Ayoko, G. A., Millar, G. J., Speight, R., Yan, C., Li, J., et al. (2017). Clay-supported Nanoscale Zero-Valent Iron Composite Materials for the Remediation of Contaminated Aqueous Solutions: a Review. Chem. Eng. J. 312, 336–350. doi:10.1016/j.cej.2016.11.154
Fan, M., Li, T., Hu, J., Cao, R., Wu, Q., Wei, X., et al. (2016). Synthesis and Characterization of Reduced Graphene Oxide-Supported Nanoscale Zero-Valent Iron (nZVI/rGO) Composites Used for Pb(II) Removal. Materials 9, 687. doi:10.3390/ma9080687
Fan, S., Wang, Y., Wang, Z., Tang, J., Tang, J., and Li, X. (2017). Removal of Methylene Blue from Aqueous Solution by Sewage Sludge-Derived Biochar: Adsorption Kinetics, Equilibrium, Thermodynamics and Mechanism. J. Environ. Chem. Eng. 5, 601–611. doi:10.1016/j.jece.2016.12.019
Fernandes, A. N., Almeida, C. A. P., Debacher, N. A., and Sierra, M. M. D. S. (2010). Isotherm and Thermodynamic Data of Adsorption of Methylene Blue from Aqueous Solution onto Peat. J. Mol. Struct. 982, 62–65. doi:10.1016/j.molstruc.2010.08.006
Freundlich, H. (1907). Über die Adsorption in Lösungen. Z. Phys. Chem. 57U, 385–470. doi:10.1515/zpch-1907-5723
Guo, D., Zhu, L., Guo, S., Cui, B., Luo, S., Laghari, M., et al. (2016). Direct Reduction of Oxidized Iron Ore Pellets Using Biomass Syngas as the Reducer. Fuel Process. Tech. 148, 276–281. doi:10.1016/j.fuproc.2016.03.009
Guz, L., Curutchet, G., Torres Sánchez, R. M., and Candal, R. (2014). Adsorption of crystal Violet on Montmorillonite (Or Iron Modified Montmorillonite) Followed by Degradation through Fenton or Photo-Fenton Type Reactions. J. Environ. Chem. Eng. 2, 2344–2351. doi:10.1016/j.jece.2014.02.007
Hammed, A. K., Dewayanto, N., Du, D., Ab Rahim, M. H., and Nordin, M. R. (2016). Novel Modified ZSM-5 as an Efficient Adsorbent for Methylene Blue Removal. J. Environ. Chem. Eng. 4, 2607–2616. doi:10.1016/j.jece.2016.05.008
Ho, Y. S., and McKay, G. (1999). Pseudo-second Order Model for Sorption Processes. Process Biochem. 34, 451–465. doi:10.1016/S0032-9592(98)00112-5
Int, C., Iqbal, M., Nisar, J., Nazir, A., and Qamar, A. Z. (2019). Bioassays Based on Higher Plants as Excellent Dosimeters for Ecotoxicity Monitoring: a Review. Chem. Int. 5, 1–80. doi:10.31221/osf.io/z2ynm
Kerkez, D. V., Tomašević, D. D., Kozma, G., Bečelić-Tomin, M. R., Prica, M. D., Rončević, S. D., et al. (2014). Three Different clay-supported Nanoscale Zero-Valent Iron Materials for Industrial Azo Dye Degradation: a Comparative Study. J. Taiwan Inst. Chem. Eng. 45, 2451–2461. doi:10.1016/j.jtice.2014.04.019
Lagergren, S. (1898). Zur theorie der sogenannten adsorption gelöster stoffe, Kungliga Svenska Vetenskapsakademiens. Handl 24, 1–39.
Langmuir, I. (1918). The Adsorption of Gases on Plane Surfaces of Glass, Mica and Platinum. J. Am. Chem. Soc. 40, 1361–1403. doi:10.1021/ja02242a004
Li, Z., Chen, Z., Zhu, Q., Song, J., Li, S., and Liu, X. (2020). Improved Performance of Immobilized Laccase on Fe3O4@C-Cu2+ Nanoparticles and its Application for Biodegradation of Dyes. J. Hazard. Mater. 399, 123088. doi:10.1016/j.jhazmat.2020.123088
Liu, J., Wang, Y., Fang, Y., Mwamulima, T., Song, S., and Peng, C. (2018). Removal of crystal Violet and Methylene Blue from Aqueous Solutions Using the Fly Ash-Based Adsorbent Material-Supported Zero-Valent Iron. J. Mol. Liquids 250, 468–476. doi:10.1016/j.molliq.2017.12.003
Liu, S., Lim, M., and Amal, R. (2014). TiO2-coated Natural Zeolite: Rapid Humic Acid Adsorption and Effective Photocatalytic Regeneration. Chem. Eng. Sci. 105, 46–52. doi:10.1016/j.ces.2013.10.041
Liu, Y., Phenrat, T., and Lowry, G. V. (2007). Effect of TCE Concentration and Dissolved Groundwater Solutes on NZVI-Promoted TCE Dechlorination and H2 Evolution. Environ. Sci. Technol. 41, 7881–7887. doi:10.1021/es0711967
Man, Y., Feng, J. X., Li, F. J., Ge, Q., Chen, Y. M., and Zhou, J. Z. (2014). Influence of Temperature and Time on Reduction Behavior in Iron Ore-Coal Composite Pellets. Powder Tech. 256, 361–366. doi:10.1016/j.powtec.2014.02.039
Mishra, P., Singh, K., and Dixit, U. (2021). Adsorption, Kinetics and Thermodynamics of Phenol Removal by Ultrasound-Assisted Sulfuric Acid-Treated Pea (Pisum Sativum) Shells. Sust. Chem. Pharm. 22, 100491. doi:10.1016/j.scp.2021.100491
Mwamulima, T., Zhang, X., Wang, Y., Song, S., and Peng, C. (2018). Novel Approach to Control Adsorbent Aggregation: Iron Fixed Bentonite-Fly Ash for Lead (Pb) and Cadmium (Cd) Removal from Aqueous media. Front. Environ. Sci. Eng. 12, 2. doi:10.1007/s11783-017-0979-6
Ngulube, T., Gumbo, J. R., Masindi, V., and Maity, A. (2019). Preparation and Characterisation of High Performing Magnesite-Halloysite Nanocomposite and its Application in the Removal of Methylene Blue Dye. J. Mol. Struct. 1184, 389–399. doi:10.1016/j.molstruc.2019.02.043
Nourein, S., Bhatti, H., Iqbal, M., Bibi, I., Zafar, N., Iqbal, D., et al. (2017). Redox Mediators Assisted-Degradation of Direct Yellow 4. Pol. J. Environ. Stud. 26, 2885–2890. doi:10.15244/pjoes/68564
Ramamoorthy, M., Ragupathy, S., Sakthi, D., Arun, V., and Kannadasan, N. (2020). Synthesis of SnO2 Loaded on Corn Cob Activated Carbon for Enhancing the Photodegradation of Methylene Blue under Sunlight Irradiation. J. Environ. Chem. Eng. 8, 104331. doi:10.1016/j.jece.2020.104331
Rasalingam, S., Peng, R., and Koodali, R. T. (2015). An Insight into the Adsorption and Photocatalytic Degradation of Rhodamine B in Periodic Mesoporous Materials. Appl. Catal. B: Environ. 174-175, 49–59. doi:10.1016/j.apcatb.2015.02.040
Saber-Samandari, S., Saber-Samandari, S., Joneidi-Yekta, H., and Mohseni, M. (2017). Adsorption of Anionic and Cationic Dyes from Aqueous Solution Using Gelatin-Based Magnetic Nanocomposite Beads Comprising Carboxylic Acid Functionalized Carbon Nanotube. Chem. Eng. J. 308, 1133–1144. doi:10.1016/j.cej.2016.10.017
Spadaro, J. T., Gold, M. H., and Renganathan, V. (1992). Degradation of Azo Dyes by the Lignin-Degrading Fungus Phanerochaete Chrysosporium. Appl. Environ. Microbiol. 58, 2397–2401. doi:10.1016/1050-1738(93)90025-210.1128/aem.58.8.2397-2401.1992
Stephen Inbaraj, B., and Chen, B. H. (2011). Dye Adsorption Characteristics of Magnetite Nanoparticles Coated with a Biopolymer Poly(γ-Glutamic Acid). Bioresour. Tech. 102, 8868–8876. doi:10.1016/j.biortech.2011.06.079
Wang, J.-Q., Liu, Y.-H., Chen, M.-W., Xie, G.-Q., Louzguine-Luzgin, D. V., Inoue, A., et al. (2012). Rapid Degradation of Azo Dye by Fe-Based Metallic Glass Powder. Adv. Funct. Mater. 22, 2567–2570. doi:10.1002/adfm.201103015
Wang, W., Cheng, Y., Kong, T., and Cheng, G. (2015). Iron Nanoparticles Decoration onto Three-Dimensional Graphene for Rapid and Efficient Degradation of Azo Dye. J. Hazard. Mater. 299, 50–58. doi:10.1016/j.jhazmat.2015.06.010
Wang, Y., López-Valdivieso, A., Zhang, T., Mwamulima, T., Zhang, X., Song, S., et al. (2017). Preparation of Microscale Zero-Valent Iron-Fly Ash-Bentonite Composite and Evaluation of its Adsorption Performance of crystal Violet and Methylene Blue Dyes. Environ. Sci. Pollut. Res. 24, 20050–20062. doi:10.1007/s11356-017-9426-2
Weber, W. J., and Morris, J. C. (1963). Kinetics of Adsorption on Carbon from Solution. J. Sanit. Engrg. Div. 89, 31–59. doi:10.1061/JSEDAI.0000430
Xu, C.-H., Zhu, L.-j., Wang, X.-H., Lin, S., and Chen, Y.-m. (2014). Fast and Highly Efficient Removal of Chromate from Aqueous Solution Using Nanoscale Zero-Valent Iron/activated Carbon (nZVI/AC). Water Air Soil Pollut. 225, 1845. doi:10.1007/s11270-013-1845-1
Xu, H.-M., Sun, X.-F., Wang, S.-Y., Song, C., and Wang, S.-G. (2018). Development of Laccase/graphene Oxide Membrane for Enhanced Synthetic Dyes Separation and Degradation. Sep. Purif. Tech. 204, 255–260. doi:10.1016/j.seppur.2018.04.036
Zhang, J., Zhang, T., Liang, X., Wang, Y., Shi, Y., Guan, W., et al. (2020a). Efficient Photocatalysis of CrVI and Methylene Blue by Dispersive Palygorskite-Loaded Zero-Valent Iron/carbon Nitride. Appl. Clay Sci. 198, 105817. doi:10.1016/j.clay.2020.105817
Zhang, L., Yao, L., Ye, L., Long, B., Dai, Y., and Ding, Y. (2020b). Benzimidazole-based Hyper-Cross-Linked Polymers for Effective Adsorption of Chlortetracycline from Aqueous Solution. J. Environ. Chem. Eng. 8, 104562. doi:10.1016/j.jece.2020.104562
Zhang, X., Lin, S., Lu, X.-Q., and Chen, Z.-l. (2010). Removal of Pb(II) from Water Using Synthesized Kaolin Supported Nanoscale Zero-Valent Iron. Chem. Eng. J. 163, 243–248. doi:10.1016/j.cej.2010.07.056
Keywords: water pollution, activated carbon, zero valent iron, crystal violet, methylene blue
Citation: Wang Y, Chen T, Zhang X and Mwamulima T (2021) Removal Study of Crystal Violet and Methylene Blue From Aqueous Solution by Activated Carbon Embedded Zero-Valent Iron: Effect of Reduction Methods. Front. Environ. Sci. 9:799264. doi: 10.3389/fenvs.2021.799264
Received: 21 October 2021; Accepted: 25 November 2021;
Published: 21 December 2021.
Edited by:
Lean Zhou, Changsha University of Science and Technology, ChinaReviewed by:
Rui Hou, South China Sea Institute of Oceanology (CAS), ChinaCopyright © 2021 Wang, Chen, Zhang and Mwamulima. This is an open-access article distributed under the terms of the Creative Commons Attribution License (CC BY). The use, distribution or reproduction in other forums is permitted, provided the original author(s) and the copyright owner(s) are credited and that the original publication in this journal is cited, in accordance with accepted academic practice. No use, distribution or reproduction is permitted which does not comply with these terms.
*Correspondence: Tiantian Chen, Y2hlbnRpYW4wODE5QDE2My5jb20=
Disclaimer: All claims expressed in this article are solely those of the authors and do not necessarily represent those of their affiliated organizations, or those of the publisher, the editors and the reviewers. Any product that may be evaluated in this article or claim that may be made by its manufacturer is not guaranteed or endorsed by the publisher.
Research integrity at Frontiers
Learn more about the work of our research integrity team to safeguard the quality of each article we publish.