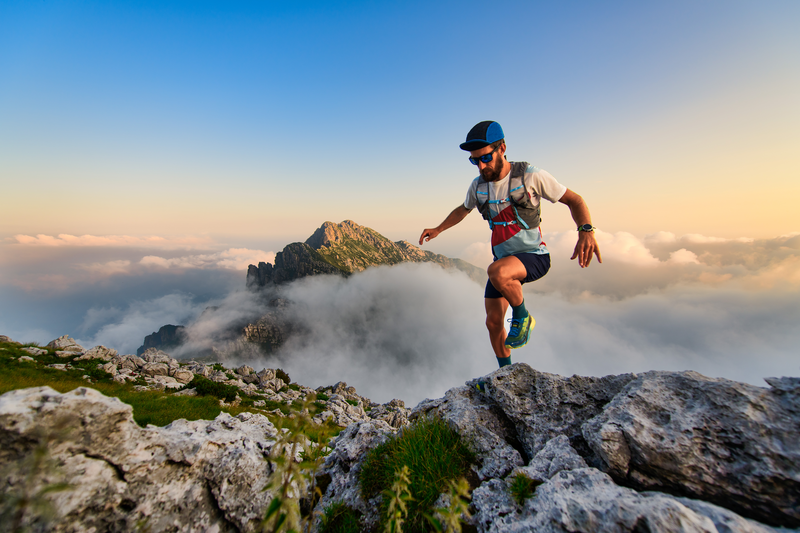
94% of researchers rate our articles as excellent or good
Learn more about the work of our research integrity team to safeguard the quality of each article we publish.
Find out more
ORIGINAL RESEARCH article
Front. Environ. Sci. , 20 January 2022
Sec. Soil Processes
Volume 9 - 2021 | https://doi.org/10.3389/fenvs.2021.798383
Split application of nitrogen (N) fertilizers during different crop growth stages to fulfill the crop N requirements reduces soil mineral N concentrations and improves the efficiency of crop N fertilizer use, and can decrease nitrous oxide (N2O) emission from the soil. However, inconsistent results regarding N2O emissions have been reported in rainfed areas. Furthermore, few long-term studies have explained the effects of split N application on soil methane (CH4) flux, thus limiting complete assessment of the effects of split N application on total greenhouse gas (GHG) emissions. Therefore, long-term monitoring is urgently required to understand the impacts of split N application on GHG emissions in rainfed areas. In this study, a 6-year field experiment was conducted in a rainfed maize (Zea mays L.) field in Northeast China. The experiment included three treatments: no N application representing control (CK), single application at the sowing stage of maize (SU), and split N at the sowing and jointing stages at a ratio of 1: 2 (SF). Between the sowing and jointing stages, N2O emissions were significantly higher in SU than in SF. However, high N2O emissions were observed in SF for 1 month after N application at the jointing stage possibly because the time of N application coincided with optimum precipitation and soil temperature conditions, which stimulated N2O emissions. Overall, the total N2O emissions showed no significant difference between SU and SF. During the study period, split application of N fertilizer did not significantly affect the cumulative CH4 flux. Compared to CK, the yield-scaled GWP in SF treatment increased by 18.7% (p < 0.05). Ammonia (NH3) volatilization in SF was 272% higher than that in SU. The findings indicated that split N application exhibited an environmental risk by increasing the yield-scaled GWP and NH3 emissions in the field. Thus, this study suggested that single N application applied in the sowing stage should be employed in rainfed fields to mitigate the yield-scaled GWP and NH3 emissions, and maintain efficient maize yields.
Nitrous oxide (N2O) is a potent greenhouse gas (GHG) with a global warming potential (GWP) that is 265 times more than that of carbon dioxide (CO2) in a 100-year timescale; moreover, it significantly destroys the stratospheric ozone layer (IPCC, 2013). Therefore, reducing N2O emissions is urgently required. Fertilized soil is a primary source of N2O (Smith et al., 1998; Thompson et al., 2019); however, using suitable agricultural management measures can effectively reduce N2O emissions (Shi et al., 2013).
In Northeast China, maize fields comprise 42.2% of the total cropped area (Yearbook, 2019). However, maize requires high inputs of N fertilizers and, thus, is an important source of atmospheric N2O (Liu et al., 2011). A meta-analysis indicated that maize exhibited high emission factors (EF) compared with other crop types (Cayuela et al., 2017). Maize requires low N amounts during its early growth stages but high N amounts for several weeks during the growing season (Abendroth et al., 2011). During the period of first few months from N application to rapid N uptake, N2O is majorly lost. Delaying the application of N fertilizers to maize during the growing season can effectively reduce N2O emissions in rainfed systems (Dell et al., 2014). Using the DNDC (DeNitrification-DeComposition) model, Li et al. (2012) suggested that N2O emissions can be mitigated with the increase in the number of N fertilizer applications from spring maize fields in Northeast China. Split N applications during the growing season could improve the balance between soil N availability and crop N demand, and, subsequently, reduce the soil N amount available for conversion to N2O (Venterea and Coulter, 2015; Schwenke and Haigh, 2019). However, previous studies on the effects of split application of N fertilizer on N2O emissions are still inconclusive, with reports of increase (Venterea and Coulter, 2015), decrease (Aita et al., 2015; Schwenke et al., 2016), and no change in the N2O emissions (Zebarth et al., 2012; Venterea et al., 2016). Differences in the amount and time of precipitation between the single and split N fertilizer applications under rainfed agricultural conditions could contribute to these disparate results (Drury et al., 2012; Yu et al., 2016). Furthermore, difficulty in predicting the precipitation amount and distribution in rainfed areas and its significant impacts on N2O emissions served as a challenge to draw consistent conclusions during short-term monitoring of N2O emissions in rainfed areas (Su et al., 2021). In addition, increased N application rate (100 kg N ha−1) gradually increased methane (CH4) absorption in upland soils (Aronson and Helliker, 2010). Contrary to previous studies, recent studies indicated that the upland soil should be a source of CH4 emission (Jiang C. M. et al., 2017; Lan et al., 2020). Therefore, the authors of this study could not draw a conclusion on the response of CH4 flux to conventional N application (180 kg N ha−1) in upland cropping systems in the study area. Thus, increased upland field studies were required to reduce the uncertainty of the effects of N application on soil CH4 fluxes.
Fertilization of agricultural soil is an important anthropogenic source of ammonia (NH3), which is a secondary source of N2O (Gao et al., 2013). Therefore, simultaneous measurements of N2O and NH3 can provide valuable insights on the effect of split N application on N losses. However, the effects of split application of urea on both N2O and NH3 emission have not been studied extensively in rainfed areas.
In this study, we aimed at examining the effects of split application of N fertilizer on N2O and CH4 emissions, maize yield, and yield-scaled GWP in a continuously rainfed maize field in Shenyang City, Northeast China, through long-term field experiments. Additionally, in the sixth year of the study, NH3 emissions were determined to evaluate the effects of split application of N fertilizer on NH3 loss. We hypothesize that split application of N fertilizer could mitigate N2O emissions, but might increase NH3 losses from this rainfed maize field.
The experiments were performed from May 2010 to April 2016 in a rainfed maize field at the Shenyang Agro-ecological Experimental Station (41°31′N, 123°22′E), Chinese Academy of Sciences, Liaoning Province, China. The mean annual precipitation of the study site is approximately 680 mm, with more than 80% of the annual precipitation concentrated from May to September. The mean annual temperature is 7.5°C. The soil is classified as Luvisol (World Reference Base) or Alfisol (Soil Taxonomy). The soil texture is silt loam (clay, 20.4%; silt, 50.1%; sand, 28.9%). The soil physical and chemical properties in the top 20 cm were as follows: soil bulk density, 1.25 g cm−3; soil pH, 5.8; total N content, 0.95 g kg−1; organic carbon content, 8.52 g kg−1; Olsen-P, 21.9 mg kg−1; and NH4OAc-K, 111.0 mg kg−1.
The experimental design consisted of three replicates of a randomized complete block, with an area of 20 m2 (4 m × 5 m) for each plot. The experimentation included three treatments: no N fertilizer application (CK), single application of 180 kg N ha−1 of urea at the sowing stage (SU), and N fertilizer application with the same rate and type as SU in two splits (SF). The SF treatment included the application of 60 kg N ha−1 and 120 kg N ha−1 at the sowing and jointing stages of maize, respectively. Calcium superphosphate (90 kg P2O5 ha−1) and potassium chloride (90 kg K2O ha−1) were applied during sowing. Furthermore, maize (Zea mays L., variety of fuyou #9) were planted in early May with a density of 45,000 plants ha−1, were seeded at 37 cm intervals, were not irrigated, and were harvested by the end of September. At harvest, maize yield and aboveground biomass yield were measured by harvesting all plants (20 m2) in each plot. After harvest of aboveground biomass, they were manually removed.
Soil-to-atmosphere N2O/CH4 fluxes were measured using static chambers (Dong et al., 2021), which consisted of a base chamber (56 cm × 28 cm × 10 cm) and a removable top chamber (56 cm × 28 cm × 20 cm), both made of stainless steel. Prior to sowing, the base chambers were inserted 10 cm into the soil in the center of a row, and were maintained in the same place throughout the study period except during tillage, which was performed once a year in April.
Gas samples were collected with a 50-ml syringe, equipped with a three-way stopcock, at 20 min intervals (t0, t20, and t40) between 9:00 and 11:00 a.m. after the chambers were closed. Subsequently, the collected samples were injected into 12 ml vacuum vials fitted with butyl rubber stoppers, and were analyzed using a gas chromatograph (Agilent 7890A, Shanghai, China) equipped with an electron capture detector and a hydrogen flame ionization detector. Gas samples were collected every 2–6 days during the growing seasons and every 7–15 days during the nongrowing seasons. The growing and nongrowing seasons were from May to September and from October to April of the following year, respectively.
The NH3 volatilization was measured using a modified vented chamber method as described by Li et al. (2019). The chamber is made up of PVC pipes with a height of 15 cm and a diameter of 15 cm. Two round sponges with 16 cm in diameter and 2 cm in thickness were placed in each chamber. Before use, the sponges were moistened with 15 ml of phosphate/glycerol solution (5% phosphate and 4% glycerol). One sponge was placed 5 cm away from the soil surface to absorb NH3 volatilized from the soil. Another sponge was placed on the top of the chamber to absorb the NH3 volatilized from the air entering the chamber through the vent. NH3 in the phosphate solution of each sponge in the chamber was determined by shaking 300 ml of 1 M KCl for 60 min. Ammonium ions were qualitified with a continuous flow analyzer (Alliance, Futura, France). The sponge in the chamber at the sowing stage of maize was replaced and sampled every day during 4–7 days after sowing, at 2–4 days intervals during the second and third week, and thereafter every 7 days until NH3 became undetectable. During the topdressing period, NH3 was observed continuously for 9 days. Next, NH3 was monitored once a week.
During measurements of gas fluxes, the soil temperature at 5 cm of soil depth was simultaneously recorded using a bent stem thermometer. Soil moisture was monitored at a depth of 0–5 cm using time domain reflectometry and was expressed as water-filled pore space (WFPS). Daily precipitation and air temperature data were acquired from the weather station of the Shenyang experimental station of ecology, Shenyang City.
The N2O/CH4 fluxes were calculated using a linear regression of concentration versus time during the chamber closure period. Cumulative N2O/CH4 emissions were calculated from May to April of the following year by adding the average values of the two adjacent fluxes, and multiplying the sum by the interval time (Ding et al., 2011). The GWP was measured with 265 kg of CO2 and 28 kg of CO2 for 1 kg of both N2O and CH4, respectively, while the yield-scaled GWP (kg CO2 t−1 yield) was calculated by dividing the GWP by the maize yield (Mosier et al., 2006; Van Groenigen et al., 2010). NH3 loss from the soil was calculated by the following formula:
Here, G represents NH3 captured by the sponge during each sampling (mg N), a is the cross-sectional area of the chamber (m2), d is the sampling duration (d), and 10–2 is the conversion coefficient. The loss rate (%) of NH3 was determined by dividing the cumulative NH3 emissions (kg N ha−1) with the amount of N applied (kg N ha−1).
Differences in N2O, CH4, and NH3 emissions were tested using one-way analysis of variance (ANOVA) followed by Tukey’s test with p = 0.05 as the significance level. Furthermore, two-way ANOVA was used to examine whether there were significant differences of the N2O flux, CH4 flux, GWP, maize yields, and yield-scaled GWP among the three treatments in the experimental years. Analyses were performed using the Statistical Package for the Social Sciences (SPSS) 18.0 for windows (SPSS, Chicago, IL, United States).
The air temperature ranged from −22.8°C to 28.3°C (Figure 1), and the annual mean air temperatures were 7.9, 8.3, 7.7, 8.1, 9.5, and 9.3°C during 2010–2015, respectively. The soil temperature trends were consistent with the air temperature changes. The soil temperature at 5 cm depth ranged between −14.0°C and 35.0°C (Figure 1). In May 2012, a dry period persisted from May 3 to 29, during which 7.6 mm of precipitation was recorded, and the soil WFPS at 0–5 cm depth decreased to 24% on May 28. In May 2015, a heavy precipitation event (61.5 mm) occurred on May 12, and the total precipitation was 71.9 mm. During July 5–24 2015, precipitation was not recorded, and the soil WFPS gradually declined to 10% on July 24. Furthermore, heavy precipitation events occurred in August 2010, August 2012, and July 2013 (Figure 2). During 2010–2015, the total annual precipitation for each year was 1,075, 645, 910, 690, 409, and 627 mm, respectively, with a mean annual precipitation of 680 mm, indicating that 2010 and 2012 witnessed heavy precipitation, while 2014 witnessed extreme droughts. During the nongrowing period in 2012, 230.3 mm of precipitation was recorded, which was the highest during the study period.
The N2O emission peaks during 2010–2015 were 84.8, 27.7, 99.6, 23.8, 96.5, and 50.6 μg N m−2 h−1 for the SU treatment, respectively, and 249.8, 62.6, 93.6, 33.9, 172.2, and 160.5 μg N m−2 h−1 for the SF treatment, respectively, with the maximum daily N2O fluxes observed after top-dressing in the SF treatment. The highest peaks in the SF treatment appeared on July 28, 2010 (249.8 μg N m−2 h−1) after precipitation (76.7 mm on July 20 and 21, 2010) following N fertilizer top-dressing at the jointing stage. The maximal peak of N2O emissions in 2010 was 7 times higher than that in 2013 (33.9 μg N m−2 h−1). Moreover, N2O emissions associated with freezing and thawing were observed in 2012–2013 (Figure 3).
FIGURE 3. Seasonal dynamics of N2O emissions in the CK, SU, and SF treatments from May 2010 to April 2016. The bars represent the standard deviations of the means (n = 3).
Among the three treatments, cumulative N2O emissions were significantly higher in 2010 than that in other years between the sowing and jointing stages in the CK and SF treatments (p < 0.05). Compared with the SF treatment, significantly higher N2O emissions were observed in the SU treatment between the sowing and jointing stages during 2011, 2012, 2014, and 2015 (p < 0.05, Table 1). Because of higher N application in the SU treatment than in the SF treatment during maize sowing, N2O emissions were 107.6% greater in the SU treatment vs SF treatment between the sowing and jointing stages. Except for 2012, the N2O emissions did not differ between the CK and SF treatments during 2010–2015 between the sowing and jointing stages (Table 1). Furthermore, during the 6-year study period, a significantly linear relationship between the soil N2O emissions between the sowing and jointing stages and N input was observed (Figure 4). The N2O emissions in 1 month after top-dressing were 29.8–53.1% of the annual cumulative N2O emissions in the SF treatment. Compared with the SU treatment, the N2O emissions increased by 169.4% in the SF treatment in the month after top-dressing (Table 1). However, no significant difference was observed during 2010–2015 between the CK and SU treatments in 1 month after top-dressing (Table 1).
TABLE 1. Cumulative N2O emission and N2O direct emission factor (EF) as affected by different treatments.
FIGURE 4. Relationship between the cumulative N2O emission between the sowing and jointing stages and the N applied.
The CK treatment exhibited the lowest N2O emissions, with an average flux of 0.466 kg N ha−1 during the 6 years (Table 1). The annual cumulative N2O emissions from the SU treatment were 0.343–2.539 kg N ha−1, with extremely high N2O emissions observed in 2010 (Table 1). No significant differences were observed between SU and SF in average N2O emissions across the 6-year observation.
The CH4 flux did not exhibit seasonal or annual trends, and each treatment represented either a small sink or a small source, with the CH4 magnitude ranging from −60.5 to 46.0 μg m−2 h−1 (Figure 5). Negative flux days accounted for 70.7% of the total sampling days across all treatments. The CH4 flux was positively correlated with soil WFPS (p < 0.01). Furthermore, soil CH4 uptake between the sowing and jointing stages was significantly in linear relation with the N input (Figure 6).
FIGURE 5. Seasonal dynamics of CH4 fluxes in the CK, SU, and SF treatments from May 2010 to April 2016. The bars represent the standard deviations of the means (n = 3).
FIGURE 6. Relationship between the cumulative CH4 absorption between the sowing and jointing stages and the N applied.
Compared with CK, CH4 absorption increased by 40.3% in SU in the maize growing season. Total CH4 uptakes in the CK, SU, and SF treatments were −0.33, −0.38, and −0.35 kg CH4-C ha−1 year−1, respectively. Two-way ANOVA results indicated that treatments had no significant effect on the cumulative CH4 flux at the annual scale (Table 2, p > 0.05).
Maize yield in the SU and SF treatments was not significant (Table 3, p > 0.05). The GWP of SU and SF were significantly higher than that of CK (Table 3, p < 0.05). The yield-scaled GWP were 21.4, 22.9, and 25.3 kg CO2 eq t−1 grain for CK, SU, and SF, respectively. Compared to CK, the yield-scaled GWP in SF treatment increased by 18.7% (Table 3, p < 0.05).
TABLE 3. Mean annual CH4 and N2O emissions and their estimated global warming potentials (GWP), yield, and yield-scaled GWP over six annual cycles from May 2010 to April 2016.
During sowing, the maximum NH3 fluxes were observed after 3 d of basal fertilizer application (Figure 7). After 1 month of the basal fertilizer application, NH3 flux reached the background level. However, during the jointing stage, NH3 flux was the highest (approximately 2.51 kg N ha−1 d−1) immediately after fertilization. The NH3 emission fluxes persisted for approximately 1 week at the maize jointing stage. Furthermore, NH3 volatilization in the SF treatment accounted for 16.7% of the total N applied during this stage. Although NH3 volatilization during top-dressing persisted for a short period, the intensity of NH3 volatilization was high. Thus, the cumulative NH3 loss from SF was 272% more than that from SU.
FIGURE 7. Dynamics of ammonia volatilization under different treatments. The bars represent the standard deviations of the means (n = 3).
The inter-annual N2O emissions significantly fluctuated during the study period. Similar results were reported by previous studies (Du et al., 2006; Scheer et al., 2008; Song et al., 2009; Zhang et al., 2014). Since the rates and methods of N application were identical each year, the variations in the inter-annual N2O emissions could be strongly related to fluctuations in the amount and distribution of precipitation.
The cumulative N2O emissions were lower during 2011/2012, 2012/2013, 2013/2014, and 2015/2016 between the sowing and jointing stages (Table 1) probably because of low precipitation and its low distribution a week prior to maize sowing till May end. Precipitation along with fertilization promoted N2O emissions because of an available C source and anaerobic conditions required for denitrification. Although precipitation during a week before sowing till the end of May 2015 was 69.4 mm, heavy precipitation events (61.5 mm, May 12) occurred after fertilization. Anaerobic conditions caused by heavy rainfall events could reduce N2O to nitrogen (N2), thus potentially explaining the low N2O emissions between the sowing and jointing stages in 2015.
In 2015, after a prolonged dry period (20 d), the soil WFPS at 0–5 cm depth was 10% (July 24); additionally, the precipitation (24.2 mm) on July 25 induced a large N2O flux in the SF treatment (160 μg N m−2 h−1). Pulse of N2O fluxes following rewetting were commonly observed in croplands, tropical forests, and grazed pastures (Davidson, 1992; Van Haren et al., 2005; Barton et al., 2008; Kim et al., 2010). The mechanisms underlying this phenomenon may be due to the enhanced metabolic activities of microorganisms, gas displacement, and reduced diffusivity (Kim et al., 2010).
Cumulative N2O emissions for the SU treatment in 2010 were approximately 7 times more than those in 2013 because 2010 received the highest precipitation (998 mm, 47% more than the mean annual precipitation). However, heavy precipitation did not always induce higher N2O emissions. This was particularly evident in the last month of the growing season (August 2012), which received 315.2 mm of precipitation. These results were consistent with the results of Liu et al. (2011). In this study, low N2O emissions in the presence of high precipitation could be explained by low soil nitrate concentrations (2.32 mg N kg−1), which were lower than the threshold required for denitrification (Dobbie and Smith, 2003). Therefore, this means that soil nitrate content could be a limiting factor of N2O emissions.
Despite lower annual precipitation in 2014 than in 2013, cumulative N2O emissions in SU in 2014 were approximately 2.6 times more than those in 2013 possibly because of the time of the precipitation events. The precipitation in May 2013 (15.4 mm) contributed to the low N2O emissions in 2013 between the sowing and jointing stages. Although the precipitation in July 2013 after top-dressing (228 mm) was the highest during the study period, only a relatively small peak of emissions occurred after top-dressing in the SF treatment (Figure 3). Thus, the timing of the precipitation events and its precipitation distribution strongly affected N2O emissions (Burton et al., 2008).
In the nongrowing season, N2O emissions played an important role in annual N2O budgets and were increased by significantly high precipitation. For example, during significantly high precipitation in the nongrowing season of 2012–2013, N2O emissions from the freeze–thaw cycles contributed 43% to the annual N2O budgets in the SU treatment during 2012–2013. Therefore, during development and designing of N2O mitigation strategies, emissions associated with the nongrowing season should be considered. Moreover, we speculated that the heavy precipitation and soil temperature along with split application would cause NH3, nitrogen oxide (NO), and NO3−-N losses. Pulse emission of NO after fertilization and precipitation/irrigation contributed to 88% of the annual NO emissions (Cui et al., 2012). Thus, studies are required to simultaneously quantify N2O, NH3, NO, and NO3−-N losses that can improve the estimation of complete impacts of split application of fertilizers on nitrogen losses.
Our results showed that split fertilization significantly reduced the cumulative N2O emissions by 51.8% compared with single fertilization between the sowing and jointing stages. The acquired significant linear relationship between the soil N2O emissions (between the sowing and jointing stages) and N input, which is consistent with previous studies (Bouwman, 1996; Kim et al., 2013), was adopted by IPCC (2019) to estimate the N2O emissions in managed agricultural soils. However, nonlinear responses of N2O emissions to N fertilizer could be observed when high amount of N fertilizer was applied (Takeda et al., 2021).
During the study period, N2O emission pulse persisted for 1 month after top-dressing (Figure 3). The combined effect of N fertilization and heavy precipitation were major drivers of N2O emissions (Zhang et al., 2014). Thus, the increase in N2O emissions after top-dressing offsets the benefits of split application during the early growth stages of maize (Aita et al., 2015).
No significant difference in the annual N2O emissions was observed between the SF and SU treatments (p > 0.05). In contrast to our hypothesis, split fertilizer application did not mitigate N2O emissions. Similar results were observed by Wang et al. (2016), who reported no signficant differences in N2O emissions between the split N application and single N application in a rainfed maize–wheat rotation system in the semiarid Loess Plateau. Additionally, Venterea et al. (2016) observed no significant differences between single urea application and split application on the N2O emission in a rainfed maize field during a 2-year study period. Thus, factors, such as soil moisture content, temperature, and C availability, that affect N2O production may be comparatively more important in regulating N2O flux than the crop stage (Venterea et al., 2016).
Based on the findings, we concluded that the single N fertilizer application was a better alternative than the two split applications in mitigating the N2O emissions in the rainfed maize fields in Northeast China. In the same region, Jiang et al. (2017) reported 1.42 kg N2O-N ha−1 emissions in three split applications with N applied at a rate of 150 kg N ha−1 during the maize growing season. Thus, this type of N application emitted more N2O (1.42 vs. 1.02 kg N2O-N ha−1) despite having low rates of N application (150 vs. 180 kg N ha−1). In addition, single fertilizations could reduce the fuel expenses and mitigate CO2 emissions from tractors that are generally used for fertilizer application (Huérfano et al., 2015). Therefore, compared with split fertilization, single N application can reduce the farming costs, conserve the environment, and maintain high maize yields.
In this study, the average EF in the SU (0.308%) and SF (0.376%) were much lower than 1% as suggested by IPCC (2006) probably because of low soil organic carbon (SOC) content (Wang et al., 2016). Stehfest and Bouwman (2006) concluded that N2O emissions were significantly lower from soils with SOC <3%. A review by Cayuela et al. (2017) indicated that soil under dry Mediterranean conditions, with an average annual precipitation >450 mm, exhibited an EF of 0.32%. Australia uses a country-specific EF of 0.2% for N fertilizer application in rainfed agriculture (Schwenke et al., 2016). The present study conducted in a rainfed area exhibited an EF that was lower than that in irrigated regions, such as North China Plain (0.61–0.77%), probably because of the absence of irrigation and low precipitation (Jiang J. et al., 2017). Previous studies conducted in Northeast China and Loess Plateau, which were under rainfed conditions, indicated that the EF in these regions was similar to that observed in our study site (Chen et al., 2014; Wang et al., 2016), and exhibited a substantial impact on estimating the total national N2O emissions inventories from the cropping systems (Cayuela et al., 2017). The EF estimated in the present study could assist in quantifying the contribution of maize fields in rainfed systems to the national N2O emission inventories in China.
The absence of distinct seasonal trends of CH4 flux estimated in this study was also observed in other studies (Liu et al., 2015; Wang et al., 2015; Fan et al., 2021). Soil WFPS only explained 3.3% of the variations in CH4 fluxes in the CK treatment. Additionally, emission or uptake of CH4 was controlled by several factors, such as soil texture, soil nutrients, and microbial activity (Carter et al., 2011; Dijkstra et al., 2013; Ran et al., 2017; Fan et al., 2021).
Some studies have indicated that N fertilizer application exceeding 100 kg ha−1 year−1 can inhibit CH4 absorptions (Aronson and Helliker, 2010). In this study, the cumulative CH4 absorptions between the sowing and jointing stages increased with increasing N fertilization. Furthermore, low or no fertilization inhibited the growth of methanotrophs, whereas high fertilization promoted the growth and activity of methanotrophs and improved the methane oxidation capacity (Wang et al., 2015; Ran et al., 2017).
There was no significant difference in maize grain yields between single application of urea at the sowing stage and two split applications at the sowing and jointing stages (p > 0.05), which was consistent with the previous study (Venterea et al., 2016). Therefore, in the local maize planting mode, the single application at the seeding stage can be used instead of the traditional application at the seeding stage and jointing stage, which can not only save labor costs but also effectively reduce greenhouse gas emissions. Single fertilization can be adopted and used by farmers in maize cultivation through extensive field trials and demonstrations. However, splitting N application has also been reported to increased crop yield by previous studies (Wang et al., 2016; Du et al., 2019; Lu et al., 2021). The differences between these studies may be related to the different amount and times of fertilization, as well as the differences in soil properties, climate, and other factors. With the increased times of split N fertilizer, there generally may be an increase in maize yields, but it may also increase greenhouse gas emissions, inevitably increasing labor costs. Therefore, it is necessary to further study the effects of fertilization frequency and the application rate on GHG and NH3 emissions, as well as their relationship with yields. In the present study, the yield-scaled GWP ranged from 4.42 to 31.58 kg CO2 eq Mg−1 in the N-fertilized treatment throughout the study period except 2010 (significantly high N2O emissions were observed in 2010 because of high precipitation). Jiang C. M. et al. (2017) reported 430 kg CO2 eq−1 ha−1 and 43.9 kg CO2 eq Mg−1 of GWP and yield-scaled GWP of maize, respectively. The relatively small values of GWP in our study could be because of low fertilizer splits [twice in our study vs. thrice in the study by Jiang et al. (2017)] and high maize yields. Moreover, GWP was significantly lower than that reported by Ma et al. (2013), who observed 610–620 kg CO2-eq Mg−1 of grain in a rice–wheat rotation field in eastern China probably due to high N application (480 kg−1 N ha−1) and high CH4 emissions in rice fields.
The NH3 volatilization loss was significantly higher in the basal stage than in the top-dressed urea application. The difference in NH3 loss between the basal and top-dressed fertilization may be associated with high air temperature in the top-dressed fertilization (17°C vs. 25°C) and precipitation (4.9 mm on May 18 and 2 mm on May 19 vs. 2.5 mm on June 27 and 11.8 mm on June 29), which accelerated urea hydrolysis, thereby increasing the soil NH4+-N content (21 mg N kg−1 dry soil). The NH3 EF of the SF treatment was 16.7%, which was higher than that observed by Li et al. (2019), who used ammonium sulfate as a N fertilizer. Thus, the increased NH3 and N2O losses during top-dressing may affect the maize yield.
Split application of urea initially decreased N2O emissions between the sowing and jointing stages, but an increase in N2O emissions was observed after top-dressing in the SF treatment, that offset the benefits of early mitigation of N2O. During the study period, no significant differences were observed between SU and SF in N2O emission. However, split application of urea significantly increased NH3 losses compared with single application. Moreover, no significant difference in the maize yield was observed between the SU and SF treatments. From the perspective of environment, single fertilization is more suitable than split fertilization in study area.
The original contributions presented in the study are included in the article/Supplementary Material; further inquiries can be directed to the corresponding authors.
WY and HX contributed to conceptualization; HS and SK contributed to methodology; DD and SK contributed to investigation; DD contributed to writing—original draft preparation; WY and HS contributed to writing—review and editing; DD and SK contributed to visualization; WY contributed to supervision; HX and DD contributed to project administration; HX and DD contributed to funding acquisition. All authors have read and agreed to the published version of the manuscript.
This study was financially supported by the Non-Profit Research Foundation for Agriculture (201103039), the National Key Research and Development Program of China (2020YFA0907800), Open Research Project of Shouguang Facilities Agriculture Center in Institute of Applied Ecology (2018SG-S-02), and the Natural Science Research Programme of Huai’an (HAB202055) and Huai’an Excellent Youth Science Foundation.
The authors declare that the research was conducted in the absence of any commercial or financial relationships that could be construed as a potential conflict of interest.
All claims expressed in this article are solely those of the authors and do not necessarily represent those of their affiliated organizations, or those of the publisher, the editors, and the reviewers. Any product that may be evaluated in this article, or claim that may be made by its manufacturer, is not guaranteed or endorsed by the publisher.
Abendroth, L. J., Elmore, R. W., Boyer, M. J., and Marlay, A. S. K. (2011). Corn Growth and Development. Ames, IA: Iowa State University Extension.
Aita, C., Schirmann, J., Pujol, S. B., Giacomini, S. J., Rochette, P., Angers, D. A., et al. (2015). Reducing Nitrous Oxide Emissions from a maize-wheat Sequence by Decreasing Soil Nitrate Concentration: Effects of Split Application of Pig Slurry and Dicyandiamide. Eur. J. Soil Sci. 66, 359–368. doi:10.1111/ejss.12181
Aronson, E. L., and Helliker, B. R. (2010). Methane Flux in Non-wetland Soils in Response to Nitrogen Addition: a Meta-Analysis. Ecology 91, 3242–3251. doi:10.1890/09-2185.1
Barton, L., Kiese, R., Gatter, D., Butterbach-Bahl, K., Buck, R., Hinz, C., et al. (2008). Nitrous Oxide Emissions from a Cropped Soil in a Semi-arid Climate. Glob. Change Biol. 14, 177–192. doi:10.1111/j.1365-2486.2007.01474.x
Bouwman, A. F. (1996). Direct Emission of Nitrous Oxide from Agricultural Soils. Nutr. Cycl. Agroecosyst. 46, 53–70. doi:10.1007/bf00210224
Burton, D. L., Zebarth, B. J., Gillam, K. M., and MacLeod, J. A. (2008). Effect of Split Application of Fertilizer Nitrogen on N2O Emissions from Potatoes. Can. J. Soil Sci. 88, 229–239. doi:10.4141/cjss06007
Carter, M. S., Ambus, P., Albert, K. R., Larsen, K. S., Andersson, M., Priemé, A., et al. (2011). Effects of Elevated Atmospheric CO2, Prolonged Summer Drought and Temperature Increase on N2O and CH4 Fluxes in a Temperate Heathland. Soil Biol. Biochem. 43, 1660–1670. doi:10.1016/j.soilbio.2011.04.003
Cayuela, M. L., Aguilera, E., Sanz-Cobena, A., Adams, D. C., Abalos, D., Barton, L., et al. (2017). Direct Nitrous Oxide Emissions in Mediterranean Climate Cropping Systems: Emission Factors Based on a Meta-Analysis of Available Measurement Data. Agric. Ecosyst. Environ. 238, 25–35. doi:10.1016/j.agee.2016.10.006
Chen, Z., Ding, W., Luo, Y., Yu, H., Xu, Y., Müller, C., et al. (2014). Nitrous Oxide Emissions from Cultivated Black Soil: A Case Study in Northeast China and Global Estimates Using Empirical Model. Glob. Biogeochem. Cycles 28, 1311–1326. doi:10.1002/2014gb004871
Cui, F., Yan, G., Zhou, Z., Zheng, X., and Deng, J. (2012). Annual Emissions of Nitrous Oxide and Nitric Oxide from a Wheat-maize Cropping System on a silt Loam Calcareous Soil in the North China Plain. Soil Biol. Biochem. 48, 10–19. doi:10.1016/j.soilbio.2012.01.007
Davidson, E. A. (1992). Pulses of Nitric Oxide and Nitrous Oxide Flux Following Wetting of Dry Soil: An Assessment of Probable Sources and Importance Relative to Annual Fluxes. Ecol. Bulletins, 149–155.
Dell, C. J., Han, K., Bryant, R. B., and Schmidt, J. P. (2014). Nitrous Oxide Emissions with Enhanced Efficiency Nitrogen Fertilizers in a Rainfed System. Agron.j. 106, 723–731. doi:10.2134/agronj2013.0108
Dijkstra, F. A., Morgan, J. A., Follett, R. F., and LeCain, D. R. (2013). Climate Change Reduces the Net Sink of CH4 and N2O in a Semiarid Grassland. Glob. Change Biol. 19, 1816–1826. doi:10.1111/gcb.12182
Ding, W. X., Yu, H. Y., and Cai, Z. C. (2011). Impact of Urease and Nitrification Inhibitors on Nitrous Oxide Emissions from Fluvo-Aquic Soil in the North China Plain. Biol. Fertil. Soils 47, 91–99. doi:10.1007/s00374-010-0504-6
Dobbie, K. E., and Smith, K. A. (2003). Impact of Different Forms of N Fertilizer on N2O Emissions from Intensive Grassland. Nutr. Cycl. Agroecosyst. 67, 37–46. doi:10.1023/a:1025119512447
Dong, D., Yang, W., Sun, H., Kong, S., and Xu, H. (2021). Nitrous Oxide Emissions in Response to Long-Term Application of the Nitrification Inhibitor DMPP in an Acidic Luvisol. Appl. Soil Ecol. 159, 103861. doi:10.1016/j.apsoil.2020.103861
Drury, C. F., Reynolds, W. D., Yang, X. M., McLaughlin, N. B., Welacky, T. W., Calder, W., et al. (2012). Nitrogen Source, Application Time, and Tillage Effects on Soil Nitrous Oxide Emissions and Corn Grain Yields. Soil Sci. Soc. America J. 76, 1268–1279. doi:10.2136/sssaj2011.0249
Du, R., Lu, D., and Wang, G. (2006). Diurnal, Seasonal, and Inter-annual Variations of N2O Fluxes from Native Semi-arid Grassland Soils of Inner Mongolia. Soil Biol. Biochem. 38, 3474–3482. doi:10.1016/j.soilbio.2006.06.012
Du, X., Kong, L., Xi, M., and Zhang, X. (2019). Split Application Improving Sweetpotato Yield by Enhancing Photosynthetic and Sink Capacity under Reduced Nitrogen Condition. Field Crops Res. 238, 56–63.
Fan, Y., Hao, X., Carswell, A., Misselbrook, T., Ding, R., Li, S., et al. (2021). Inorganic Nitrogen Fertilizer and High N Application Rate Promote N2O Emission and Suppress CH4 Uptake in a Rotational Vegetable System. Soil Tillage Res. 206, 104848. doi:10.1016/j.still.2020.104848
Gao, J., Zhang, H., Cao, X., Ding, J., Yu, G., and Xu, H. (2013). Characteristics and Kinetics of Ammonia and N2O Emissions of Aged Refuse Irrigated from Landfill Leachate. Waste Manage. 33, 1229–1236. doi:10.1016/j.wasman.2013.02.008
Huérfano, X., Fuertes-Mendizábal, T., Duñabeitia, M. K., González-Murua, C., Estavillo, J. M., and Menéndez, S. (2015). Splitting the Application of 3,4-dimethylpyrazole Phosphate (DMPP): Influence on Greenhouse Gases Emissions and Wheat Yield and Quality under Humid Mediterranean Conditions. Eur. J. Agron. 64, 47–57. doi:10.1016/j.eja.2014.11.008
IPCC (2006). “2006 IPCC Guidelines for National Greenhouse Gas Inventories,” in Volume 4 Agricultural, Forestry and Other Land Use. Editors H. S. Eggleston, L. Buendia, K. Miwa, T. Ngara, and K. Tanabe (Japan: IGES), 11.11–11.54.
IPCC (2013). Climate Change 2013: The Physical Science Basis: Working Group Ⅰ Contribution to the Fifth Assessment Report of the Intergovernmental Panel on Climate Change. UK.
IPCC (2019). “Climate Change 2019. Refinement to the 2006 IPCC Guidelines for National Greenhouse Gas Inventories,” in Volume 4 Agriculture, Forestry and Other Land Use. Editors D. Gómez, and W. Irving (Cambridge, United Kingdom and New York, NY, USA: Cambridge University Press).
Jiang, C. M., Yu, W. T., Ma, Q., Xu, Y. G., and Zou, H. (2017). Alleviating Global Warming Potential by Soil Carbon Sequestration: A Multi-Level Straw Incorporation experiment from a maize Cropping System in Northeast China. Soil Tillage Res. 170, 77–84. doi:10.1016/j.still.2017.03.003
Jiang, J., Wang, R., Wang, Z., Guo, S., and Ju, X. (2017). Nitrous Oxide and Methane Emissions in spring maize Field in the Semi-arid Regions of Loess Plateau. CLEAN. – Soil Air Water 45, 1500271. doi:10.1002/clen.201500271
Kim, D.-G., Hernandez-Ramirez, G., and Giltrap, D. (2013). Linear and Nonlinear Dependency of Direct Nitrous Oxide Emissions on Fertilizer Nitrogen Input: A Meta-Analysis. Agric. Ecosyst. Environ. 168, 53–65. doi:10.1016/j.agee.2012.02.021
Kim, D.-G., Mishurov, M., and Kiely, G. (2010). Effect of Increased N Use and Dry Periods on N2O Emission from a Fertilized Grassland. Nutr. Cycl Agroecosyst 88, 397–410. doi:10.1007/s10705-010-9365-5
Lan, T., Li, M., Han, Y., Deng, O., Tang, X., Luo, L., et al. (2020). How Are Annual CH4, N2O, and NO Emissions from rice-wheat System Affected by Nitrogen Fertilizer Rate and Type. Appl. Soil Ecol. 150, 103469. doi:10.1016/j.apsoil.2019.103469
Li, H., Qiu, J.-j., Wang, L.-g., Xu, M.-y., Liu, Z.-q., and Wang, W. (2012). Estimates of N2O Emissions and Mitigation Potential from a Spring Maize Field Based on DNDC Model. J. Integr. Agric. 11, 2067–2078. doi:10.1016/s2095-3119(12)60465-1
Li, J., Yang, H., Zhou, F., Zhang, X., Luo, J., Li, Y., et al. (2019). Effects of maize Residue Return Rate on Nitrogen Transformations and Gaseous Losses in an Arable Soil. Agric. Water Manage. 211, 132–141. doi:10.1016/j.agwat.2018.09.049
Liu, L., Hu, C., Yang, P., Ju, Z., Olesen, J. E., and Tang, J. (2015). Effects of Experimental Warming and Nitrogen Addition on Soil Respiration and CH4 Fluxes from Crop Rotations of winter Wheat-Soybean/fallow. Agric. For. Meteorology 207, 38–47. doi:10.1016/j.agrformet.2015.03.013
Liu, Y. T., Li, Y. E., Wan, Y. F., Chen, D. L., Gao, Q. Z., Li, Y., et al. (2011). Nitrous Oxide Emissions from Irrigated and Fertilized spring maize in Semi-arid Northern China. Agric. Ecosyst. Environ. 141, 287–295. doi:10.1016/j.agee.2011.03.002
Lu, J., Hu, T., Zhang, B., Wang, L., Yang, S., Fan, J., et al. (2021). Nitrogen Fertilizer Management Effects on Soil Nitrate Leaching, Grain Yield and Economic Benefit of Summer maize in Northwest China. Agric. Water Manage. 247, 106739. doi:10.1016/j.agwat.2021.106739
Ma, Y. C., Kong, X. W., Yang, B., Zhang, X. L., Yan, X. Y., Yang, J. C., et al. (2013). Net Global Warming Potential and Greenhouse Gas Intensity of Annual rice-wheat Rotations with Integrated Soil-Crop System Management. Agric. Ecosyst. Environ. 164, 209–219. doi:10.1016/j.agee.2012.11.003
Mosier, A. R., Halvorson, A. D., Reule, C. A., and Liu, X. J. (2006). Net Global Warming Potential and Greenhouse Gas Intensity in Irrigated Cropping Systems in Northeastern Colorado. J. Environ. Qual. 35, 1584–1598. doi:10.2134/jeq2005.0232
Ran, Y., Xie, J., Xu, X., Li, Y., Liu, Y., Zhang, Q., et al. (2017). Warmer and Drier Conditions and Nitrogen Fertilizer Application Altered Methanotroph Abundance and Methane Emissions in a Vegetable Soil. Environ. Sci. Pollut. Res. 24, 2770–2780. doi:10.1007/s11356-016-8027-9
Scheer, C., Wassmann, R., Kienzler, K., Ibragimov, N., and Eschanov, R. (2008). Nitrous Oxide Emissions from Fertilized, Irrigated Cotton (Gossypium Hirsutum L.) in the Aral Sea Basin, Uzbekistan: Influence of Nitrogen Applications and Irrigation Practices. Soil Biol. Biochem. 40, 290–301. doi:10.1016/j.soilbio.2007.08.007
Schwenke, G. D., and Haigh, B. M. (2019). Can Split or Delayed Application of N Fertiliser to Grain Sorghum Reduce Soil N2O Emissions from Sub-tropical Vertosols and Maintain Grain Yields. Soil Res. 57, 859–874. doi:10.1071/sr19080
Schwenke, G. D., Herridge, D. F., Scheer, C., Rowlings, D. W., Haigh, B. M., and McMullen, K. G. (2016). Greenhouse Gas (N2O and CH4) Fluxes under Nitrogen-Fertilised Dryland Wheat and Barley on Subtropical Vertosols: Risk, Rainfall and Alternatives. Soil Res. 54, 634–650. doi:10.1071/sr15338
Shi, Y., Wu, W., Meng, F., Zhang, Z., Zheng, L., and Wang, D. (2013). Integrated Management Practices Significantly Affect N2O Emissions and Wheat-maize Production at Field Scale in the North China Plain. Nutr. Cycl. Agroecosyst. 95, 203–218. doi:10.1007/s10705-013-9558-9
Smith, K. A., Thomson, P. E., Clayton, H., McTaggart, I. P., and Conen, F. (1998). Effects of Temperature, Water Content and Nitrogen Fertilisation on Emissions of Nitrous Oxide by Soils. Atmos. Environ. 32, 3301–3309. doi:10.1016/s1352-2310(97)00492-5
Song, C., Xu, X., Tian, H., and Wang, Y. (2009). Ecosystem-atmosphere Exchange of CH4 and N2O and Ecosystem Respiration in Wetlands in the Sanjiang Plain, Northeastern China. Glob. Change Biol. 15, 692–705. doi:10.1111/j.1365-2486.2008.01821.x
Stehfest, E., and Bouwman, L. (2006). N2O and NO Emission from Agricultural fields and Soils under Natural Vegetation: Summarizing Available Measurement Data and Modeling of Global Annual Emissions. Nutr. Cycl. Agroecosyst. 74, 207–228. doi:10.1007/s10705-006-9000-7
Su, C., Kang, R., Huang, W., and Fang, Y. (2021). Temporal Patterns of N2O Fluxes from a Rainfed Maize Field in Northeast China. Front. Environ. Sci. 9. doi:10.3389/fenvs.2021.668084
Takeda, N., Friedl, J., Rowlings, D., De Rosa, D., Scheer, C., and Grace, P. (2021). Exponential Response of Nitrous Oxide (N2O) Emissions to Increasing Nitrogen Fertiliser Rates in a Tropical Sugarcane Cropping System. Agric. Ecosyst. Environ. 313, 107376. doi:10.1016/j.agee.2021.107376
Thompson, R. L., Lassaletta, L., Patra, P. K., Wilson, C., Wells, K. C., Gressent, A., et al. (2019). Acceleration of Global N2O Emissions Seen from Two Decades of Atmospheric Inversion. Nat. Clim. Chang. 9, 993–998. doi:10.1038/s41558-019-0613-7
Van Groenigen, J. W., Velthof, G. L., Oenema, O., Van Groenigen, K. J., and Van Kessel, C. (2010). Towards an Agronomic Assessment of N2O Emissions: a Case Study for Arable Crops. Eur. J. Soil Sci. 61, 903–913. doi:10.1111/j.1365-2389.2009.01217.x
Van Haren, J. L. M., Handley, L. L., Biel, K. Y., Kudeyarov, V. N., McLain, J. E. T., Martens, D. A., et al. (2005). Drought-induced Nitrous Oxide Flux Dynamics in an Enclosed Tropical forest. Glob. Change Biol. 11, 1247–1257. doi:10.1111/j.1365-2486.2005.00987.x
Venterea, R. T., Coulter, J. A., and Dolan, M. S. (2016). Evaluation of Intensive “4R” Strategies for Decreasing Nitrous Oxide Emissions and Nitrogen Surplus in Rainfed Corn. J. Environ. Qual. 45, 1186–1195. doi:10.2134/jeq2016.01.0024
Venterea, R. T., and Coulter, J. A. (2015). Split Application of Urea Does Not Decrease and May Increase Nitrous Oxide Emissions in Rainfed Corn. Agron.j. 107, 337–348. doi:10.2134/agronj14.0411
Wang, L., Pan, Z., Xu, H., Wang, C., Gao, L., Zhao, P., et al. (2015). The Influence of Nitrogen Fertiliser Rate and Crop Rotation on Soil Methane Flux in Rain-Fed Potato fields in Wuchuan County, China. Sci. Total Environ. 537, 93–99. doi:10.1016/j.scitotenv.2015.08.003
Wang, S., Luo, S., Li, X., Yue, S., Shen, Y., and Li, S. (2016). Effect of Split Application of Nitrogen on Nitrous Oxide Emissions from Plastic Mulching maize in the Semiarid Loess Plateau. Agric. Ecosyst. Environ. 220, 21–27. doi:10.1016/j.agee.2015.12.030
Yu, Y., Zhao, C., and Jia, H. (2016). Ability of Split Urea Applications to Reduce Nitrous Oxide Emissions: A Laboratory Incubation experiment. Appl. Soil Ecol. 100, 75–80. doi:10.1016/j.apsoil.2015.12.009
Zebarth, B. J., Snowdon, E., Burton, D. L., Goyer, C., and Dowbenko, R. (2012). Controlled Release Fertilizer Product Effects on Potato Crop Response and Nitrous Oxide Emissions under Rain-Fed Production on a Medium-Textured Soil. Can. J. Soil Sci. 92, 759–769. doi:10.4141/cjss2012-008
Keywords: maize, N2O, split application, NH3 volatilization, CH4, greenhouse gas emissions, luvisol
Citation: Dong D, Yang W, Sun H, Kong S and Xu H (2022) Effects of Split Application of Urea on Greenhouse Gas and Ammonia Emissions From a Rainfed Maize Field in Northeast China. Front. Environ. Sci. 9:798383. doi: 10.3389/fenvs.2021.798383
Received: 20 October 2021; Accepted: 24 December 2021;
Published: 20 January 2022.
Edited by:
Rosa Francaviglia, Council for Agricultural and Economics Research (CREA), ItalyReviewed by:
José L. S. Pereira, Instituto Politecnico de Viseu, PortugalCopyright © 2022 Dong, Yang, Sun, Kong and Xu. This is an open-access article distributed under the terms of the Creative Commons Attribution License (CC BY). The use, distribution or reproduction in other forums is permitted, provided the original author(s) and the copyright owner(s) are credited and that the original publication in this journal is cited, in accordance with accepted academic practice. No use, distribution or reproduction is permitted which does not comply with these terms.
*Correspondence: Weichao Yang, eWFuZ3dlaWNoYW9AaWFlLmFjLmNu; Hui Xu, eHVodWlAaWFlLmFjLmNu
Disclaimer: All claims expressed in this article are solely those of the authors and do not necessarily represent those of their affiliated organizations, or those of the publisher, the editors and the reviewers. Any product that may be evaluated in this article or claim that may be made by its manufacturer is not guaranteed or endorsed by the publisher.
Research integrity at Frontiers
Learn more about the work of our research integrity team to safeguard the quality of each article we publish.