Corrigendum: Functional Flows in Groundwater-Influenced Streams: Application of the California Environmental Flows Framework to Determine Ecological Flow Needs
- 1Center for Watershed Sciences, University of California, Davis, Davis, CA, United States
- 2California Department of Fish and Wildlife, Water Branch, West Sacramento, CA, United States
- 3Department of Wildlife, Fish and Conservation Biology, University of California, Davis, Davis, CA, United States
- 4The Nature Conservancy, Water Division, Sacramento, CA, United States
- 5Department of Environmental Science, Policy and Management, University of California, Berkeley, Berkeley, CA, United States
- 6Biology Department, Southern California Coastal Water Research Project, Costa Mesa, CA, United States
Environmental flows, or the practice of allocating water in river systems for ecological purposes, is a leading strategy for conserving aquatic species and improving river health. However, consideration of surface-groundwater connectivity is seldom addressed in environmental flow development due to a lack of methodologies that account for groundwater contributions to instream flow. Groundwater-influenced streams have been identified as key refugia for native biota under a rapidly changing climate. These ecosystems are anticipated to be more resistant to climate change because groundwater input buffers the adverse effects of low flows and high temperatures, particularly in the dry season. Less understood, however, is the relative contribution of groundwater inputs to streamflow and how these surface-groundwater water interactions should be accounted for in environmental flow assessments and management actions. In order to assess ecological flow needs in groundwater-influenced streams, we applied the California Environmental Flows Framework (CEFF) in two river systems in California, United States. The Little Shasta River and the lower Cosumnes River are representative of many groundwater-influenced streams throughout the semi-arid western United States. Historically, perennial streamflow once sustained diverse native aquatic species in these ecosystems, but water withdrawals for irrigated agriculture has resulted in periodic stream dewatering. We found CEFF was useful in quantifying ecological flow needs for seasonal components of the flow regime that support ecosystem functionality. In particular, CEFF offered flexibility to incorporate information on the seasonal and spatial dimensions of groundwater influences in the development of ecological flow targets. The focus on ecosystem functions in CEFF, and ability to account for groundwater influences on those functions, creates opportunities for integrated surface-groundwater management strategies that support the recovery and protection of streamflows in groundwater-influenced streams.
1 Introduction
Development of river systems for human use is ubiquitous across the globe (Lehner et al., 2011; Grill et al., 2019; Cooley et al., 2021) and has resulted in drastic reductions in freshwater biodiversity and ecosystem services (Vorosmarty et al., 2010; Reid et al., 2019). Recent global biodiversity initiatives explicitly call for actions that restore freshwater ecosystem processes, improve water quality, accelerate environmental flow implementation, and protect critical habitats (Tickner et al., 2020a; van Rees et al., 2021). Environmental flows, or the practice of allocating water in river systems for ecological purposes, is a leading strategy for conserving aquatic species and improving river health (Horne et al., 2017). Environmental flows are often implemented in regulated rivers through re-operation of large dams, but less attention has been given to rivers where flow is affected by other water management activities, including diversions from surface waters, springs, and groundwater sources. In particular, existing environmental flow programs and methodologies rarely account for the influence of groundwater withdrawals on river flows, despite well-recognized interactions between surface water and groundwater in many river systems (Rohde et al., 2017). Moreover, groundwater management programs are typically focused on urban or agricultural uses and rarely account for environmental water needs of groundwater dependent ecosystems (GDEs), defined as “terrestrial, aquatic, and coastal ecosystems that require access to, replenishment or benefit from, or otherwise rely on subsurface stores of water to function or persist” (Howard and Merrifield, 2010). New environmental flow assessment approaches are needed that consider surface-groundwater interactions and incorporate the role of groundwater in supporting the health of groundwater-influenced streams and their associated GDEs.
In California, one of the most geographically diverse states in the United States, groundwater-influenced streams are found throughout climatically variable regions and across varying geologies (Howard and Merrifield, 2010). In these streams, groundwater discharge via surface springs or shallow subsurface flow provides dry season baseflow critical for sustaining aquatic habitat when precipitation is low or lacking. Groundwater inputs typically create cool water upwelling in streams when hot temperatures and low flows in the dry season can limit instream productivity and physiologically stress fish and other organisms (Cunjak, 1988; Davidson et al., 2010). Conversely, during the wet season, groundwater can have a warming effect on physiological stressful low-temperature conditions (Davidson et al., 2010). Groundwater-influenced streams have also been shown to provide highly productive rearing habitat for salmon and other native fishes in California because of their naturally higher levels of nutrients, including nitrate and phosphate (Lusardi et al., 2016; Lusardi et al., 2020). The combination of optimal thermal regimes, high productivity, and stable hydrologic conditions make groundwater-influenced streams critical refugia for coldwater species in arid and semi-arid environments such as California (Lusardi et al., 2021).
Despite their high conservation value, most groundwater-influenced streams have been highly altered by human activities (Gomez-Baggethun et al., 2019; Tickner et al., 2020b). Because of their reliable flows and high water quality, groundwater-influenced streams serve as valuable water supplies for agricultural and municipal uses. As a result, surface water diversions, groundwater pumping, and drainage of riparian wetlands are ubiquitous and have substantially impacted groundwater-influenced stream habitats. Diversions from surface springs and groundwater pumping have also contributed to widespread flow depletion across the United States (Jasechko et al., 2021), particularly in the dry season when groundwater contributes a substantial portion of baseflow and when aquatic ecosystems are already stressed by high temperatures and low flows (Zipper et al., 2019). There is an urgent need to prevent further degradation of groundwater-influenced streams and their associated GDEs and to implement actions to restore and protect the surface water and groundwater sources that sustain environmental flows.
Many environmental flow assessments focus on developing flows that support the needs of one or more key aquatic species, such as PHABSIM for assessing hydraulic habitat requirements for salmon (Milhous et al., 1989; Spence and Hickley, 2000), with little consideration of other important ecological factors such as temperature or nutrient concentrations that can be strongly influenced by groundwater contributions. In contrast, holistic approaches go beyond the needs of single species and consider the role of flow variability on ecosystem processes and aquatic community response. For example, the Ecological Limits of Hydrologic Alteration (ELOHA) framework is an approach to identify ecological flow needs using relationships between flow and multiple ecological outcomes, including single species responses but also indicators of biotic community health (Poff et al., 1997). However, such approaches require high-quality coupled data on biological and flow conditions and may overlook mediating factors that can alter flow-ecology relationships, such as altered channel morphology or water quality impairments. To overcome these limitations, river scientists have called for a “functional flows approach” to freshwater ecosystem management. The functional flows approach aims to manage and restore discrete components of the natural hydrograph that support key ecosystem functions and drive geomorphic and ecological processes (Yarnell et al., 2015). By focusing on key seasonal flow components such as the spring snowmelt recession or peak flood flows, the functional flows approach holistically addresses the needs of all aquatic species that are adapted to the natural seasonal variability in flow, but does not require the high density of data needed to develop ecological-flow relationships. Rather, the approach considers how flows interact with physical channel conditions, floodplains, sediment regimes, thermal regimes, and other physical processes, including groundwater connectivity, to support critical ecosystem functions (Yarnell and Thoms).
Here, we describe an application of the California Environmental Flows Framework (CEFF), which uses a functional flows approach to determine environmental flow needs, in two groundwater-influenced streams in California. We demonstrate how the influence of groundwater on stream functions can be incorporated in CEFF through 1) an evaluation of groundwater sources contributing to streamflow, 2) consideration of channel morphology controls on surface-groundwater interactions, and 3) assessment of groundwater effects on stream water quality. We also discuss management actions that could be expected to sustain surface-groundwater interactions that are critical to stream ecosystem health. CEFF and other holistic environmental flow assessments that account for influences of groundwater are likely to become increasingly important for restoring the ecological health of rivers and maintaining ecosystem resilience in the face of climate change.
2 Methods
2.1 California Environmental Flows Framework
The California Environmental Flows Framework (CEFF) is a structured process for setting environmental flow standards following a functional flows approach. Functional flows are components of the natural flow regime that sustain the biological, physical, and chemical processes upon which native freshwater species depend (Yarnell et al., 2015; Grantham et al., 2020). The functional flows approach is founded on the principles of the natural flow regime paradigm (Poff et al., 1997), but recognizes specific dimensions of flow variability and their interactions with the landscape as being particularly important for supporting ecosystem processes. Unlike other environmental flow assessments, a functional flows approach does not rely on single species flow needs to determine appropriate flows, but rather focuses on the natural ranges of specific flow components that drive ecosystem functions, such as the spring snowmelt recession that provides spawning cues for fish or peak flood flows that provide channel-floodplain connectivity, and recommends preservation of those flow ranges as ecological flow standards. For California, five functional flow components have been identified that support key ecosystem functions—fall pulse flow, wet-season baseflow, peak flows, spring recession flow, and dry-season baseflow—each of which are quantified by a suite of functional flow metrics describing their magnitude, timing, frequency, and duration (Yarnell et al., 2020). CEFF provides a process for defining the ranges of these key flow components, taking into consideration potential mediating factors such as channel conditions, water quality conditions, and biologic interactions, and then developing environmental flow recommendations that balance multiple water uses (Stein et al., 2021).
CEFF is organized into three sections (Stein et al., 2021). In section A, initial ecological flow needs—flows broadly protective of ecosystem health and expected to support critical ecologic functions and native aquatic and riparian communities—are estimated from predicted natural ranges of functional flows using hydrologic modeling methods (see (Grantham et al.), this issue, for more information on the modeling approach). In section B, ecological flow needs may be revised if physical, chemical, or biological process have been altered and natural ranges of functional flows would no longer support ecologic functions. Revised ecological flow needs are based on additional site-specific information that describes the relationship between functional flow components and ecosystem response. For example, consideration of channel geomorphology (e.g. floodplain connectivity in incised channels) may require adjustments to the ecological flow ranges for peak flows to ensure inundated floodplain habitat and associated functions are provided. While flow needs for individual species of management or regulatory interest (e.g. endangered salmon) may also be evaluated to confirm that the functional flows provide suitable flow requirements, adjustments to the ecological flow ranges should not be made to meet only the singular needs of a species of interest (Obester et al., 2021). Rather, a range in flow variability for all key flow components should be retained to ensure ecosystem functionality is met. Section C of CEFF provides guidance on determining environmental flows—flows that consider both ecological flow needs and human water demands—and offers suggestions for implementing and adaptively managing environmental flows over time (Stein et al., 2021).
In this paper, we highlight how groundwater influences were addressed in application of CEFF to the Little Shasta River, a spring-influenced stream in northern California, and the lower Cosumnes River, a floodplain groundwater-influenced river in central California. At both study sites, we followed the guidance under CEFF sections A and B to determine ecological flow needs supportive of ecosystem functionality and provide ecological considerations for future development of environmental flow recommendations by watershed stakeholders via section C (CWQMC-EFW, 2021). Following section A guidance, we downloaded the predicted natural ranges of functional flows (quantified as a suite of functional flow metrics) for each study site from the California Natural Flows database (https://rivers.codefornature.org). Metrics are expressed as a range of values expected to occur at each location of interest under natural conditions over a long-term period of record (10 or more years), developed from models that rely on a network of reference gages in the region (Grantham et al., this issue). We then evaluated factors that may contribute to, or limit the effectiveness of, the natural range of functional flow metrics in supporting ecosystem functions to determine whether the range of metrics for any flow component should be refined per guidance in section B. In particular, we assessed the potential for contributions of groundwater to enhance surface flows at each study site, especially during the dry season when runoff from precipitation is limited or lacking and flows are often sustained by groundwater inputs. We evaluated existing studies and knowledge of known groundwater inputs, such as discrete spring volumes, and determined whether these contributions should be considered in our estimates of the natural range of dry season baseflow magnitude or other functional flow components.
Per guidance in section B, we also evaluated the potential of non-flow factors, including physical habitat and water quality, to affect the relationship between natural functional flows, surface-groundwater interactions, and ecosystem functions. For example, altered channel morphology, such as channel incision, can limit the functionality of several key functional flow components by modifying flow hydraulics and the spatial and temporal interactions of water and the landscape. Deeply incised channels require higher peak flows to inundate the floodplain during the wet season and to provide floodplain connectivity, riparian recruitment, and habitat availability for native fish during the spring flow recession (Yarnell et al., 2015). Reduced floodplain connectivity can limit functions such as groundwater recharge that support gaining stream conditions and extended riparian soil moisture for GDEs, and highly incised channels can cause groundwater levels to fall below riparian vegetation rooting depths, resulting in the loss of riparian vegetation and habitat (Loheide and Booth, 2011; Barlow and Leake, 2012). We evaluated existing studies and information available on channel morphology at each site to assess whether altered channel conditions may prevent floodplain inundation or decrease baseflow due to water loss (drainage) to the surrounding subsurface, and thus require higher peak flow magnitudes or higher dry season baseflow magnitudes, respectively, to achieve functionality.
Additionally, we reviewed existing studies on water quality conditions at each study site to determine whether groundwater contributions may affect water temperature or water quality conditions such that increased or decreased baseflow may be needed during the dry season. While groundwater-influenced streams provide reliable water supply during the dry season, they are particularly notable for providing high water quality with limited contaminants from their adjacent wetlands and deep aquifer sources (Lusardi et al., 2021). Groundwater-derived baseflows also typically provide relatively cool water during the dry season and warm water during the wet season, helping to mitigate physiologically stressful seasonal extremes in temperature (Davidson et al., 2010). In addition, spring-fed systems in particular provide high naturally derived nutrient levels that support high aquatic productivity relative to surface-dominated streamflows (Lusardi et al., 2016). During the dry season especially, considerations of baseflow volume alone may be insufficient to support suitable habitat conditions for aquatic biota as the quality of water, including temperature and nutrient conditions, are critical to species persistence and success.
The outcomes from section A and section B analyses determine ecological flow needs at each study site, which can then be used by watershed stakeholders seeking to develop environmental flow recommendations following guidance under section C.
2.2 Study Sites
2.2.1 Little Shasta River
The Shasta River, a large tributary to the Klamath River, was historically one of the most productive salmon streams in California (National Resources Council, 2004). Groundwater from cold, nutrient-rich springs provided nearly optimal aquatic habitat conditions that supported robust populations of Chinook salmon (Oncorhynchus tshawytscha) and coho salmon (Oncorhynchus kisutch). More than a century of aquatic and riparian habitat degradation along the Shasta River and its tributaries has resulted in dramatic declines of wild salmon populations, including upper Klamath/Trinity spring-run Chinook and the federally threatened Southern Oregon/Northern California Coast coho salmon (Moyle, 2002; Moyle et al., 2017). Diversion of surface and groundwater resources in support of irrigated agricultural activities throughout the Shasta Basin, including the Little Shasta River tributary, reduced the quantity and quality of cold-water habitat during juvenile rearing and adult migration in summer and autumn. Historical adjudication of water rights did not consider the quality and quantity of water necessary to support native fishes. As a result, surface water allocations prioritize agricultural and other human water use, with limited water remaining in the environment to support ecological functions needed by salmon and other aquatic species. While progress had been made reconciling ecological water needs and human uses in some of the highest priority reaches, stream flows are insufficient for supporting healthy ecosystem conditions in most of the Shasta River.
The Little Shasta River plays a vital role in the recovery of native fishes in the Shasta River watershed, and thus is of great interest to the regulatory community and other stakeholders. Originating at 1830 m in elevation and extending approximately 41.7 km west from the Cascade Mountains of northern California until its confluence with the Shasta River within the lower Klamath River basin (Figure 1), the Little Shasta River contributes to riverine habitat diversity within the broader Shasta River watershed because of its mixed source hydrology. While the mainstem Shasta River receives the majority of its flows from productive groundwater springs emerging from volcanic terrain, the Little Shasta River derives its streamflow from both surface runoff (snowmelt and wet season rainfall) over predominantly volcanic and metavolcanic terrain and groundwater fed from several springs. Three distinct stream reaches—headwaters, foothills, and bottomlands—have been identified in the Little Shasta River that reflect different geomorphic and hydrologic conditions (McBain and Trush, 2013) (Figure 1). The steeper and higher elevation forested headwaters are fed by surface runoff from winter rainfall and spring snowmelt and control the hydrologic and thermal regime of the river. The foothills reach is dominated by herbaceous and shrub land cover with a lower gradient (<4%) and wider channel, creating more diverse channel habitats, with flow that is fed by the headwaters and supplemented by discrete groundwater-fed springs. The bottomlands reach is the lowest gradient (<1%), dominated by agricultural and herbaceous land cover and exhibiting wide shallow channels with limited habitat complexity that creates warmer water temperatures and supports extensive riparian wetlands.
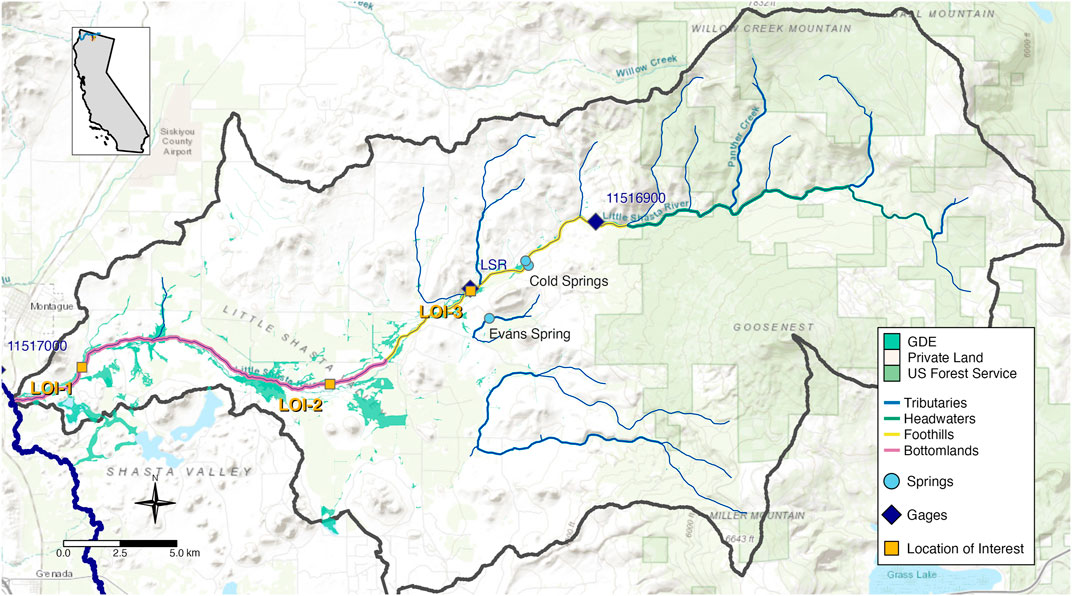
FIGURE 1. The Little Shasta River watershed, tributary to the Shasta River in Northern California. Streamlines reflect differing geomorphic and hydrologic conditions, including small tributaries and three primary stream reaches: headwaters, foothills, and bottomlands. Groundwater dependent ecosystems (GDEs) are shown as green shaded polygons. Locations of interest are shown as orange squares, and flow gages are shown as blue diamonds. Background images shows topographic map with elevation contours and private versus public (US Forest Service) land designation. LOI 3 is coincident with the LSR flow gage. USGS gage 11516900 in the upper watershed is no longer active; USGS gage 11517000 on the main Shasta River just upstream of the Little Shasta confluence is currently active.
Multiple groundwater springs and seeps contribute baseflow to the Little Shasta River and its tributaries throughout the upper headwaters reach and near Table Rock at the eastern edge of the Little Shasta Valley where porous volcanic rocks overlay less permeable Quaternary alluvium (Figure 2). Historical flow data prior to agricultural development and spring diversions are unavailable for the Little Shasta watershed, but information on spring discharge volumes and monthly flows dating back to the early decades of the 20th century can be found from Shasta Watermaster reports and was also summarized in Nichols et al. (2016). These historical accounts indicated that, collectively, springs contributed in excess of 20 cfs to the Little Shasta River (Adams et al., 1912). These spring-fed baseflows are augmented by surface runoff from winter rainfall and spring snowmelt in the headwaters, which contribute mean monthly flows ranging from less than 5 cfs during the dry season (typically June-October) to over 50 cfs during the wet season (Nov-May) and annual peak flows of 200–800 cfs (historical data, USGS gage 11516900). Together, rainfall and snowmelt provided seasonal hydrologic variability on top of the stable, cool groundwater-supported baseflows throughout the year.
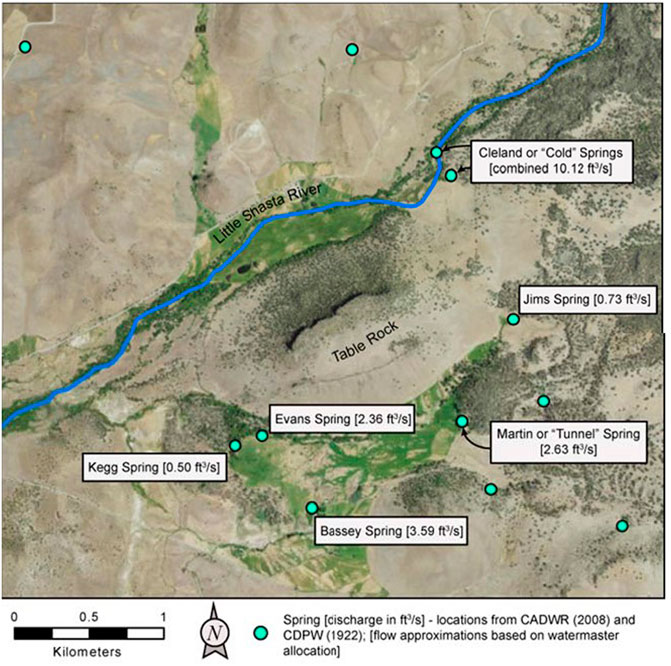
FIGURE 2. Springs and estimated historic discharges (in cubic feet per second) based on early 20th century watermaster reports (reproduced from Nichols et al., 2016). Evans spring and Cold springs are shown on Figure 1, for location reference.
Downstream of the foothills reach, the low gradient bottomlands reach includes multiple GDEs and wetlands, supported by both local groundwater sources and upstream baseflow contributions (Figure 1). Historically, these low-lying wetlands likely supported a diverse aquatic community throughout the year with a variety of warm surface-water and cool groundwater-influenced habitats through which native fish migrated during spring, summer, and autumn. Nutrients from upstream springs likely contributed to primary and secondary productivity in the bottomland reach, supporting higher order consumers such as steelhead trout (Oncorhynchus mykiss) and coho salmon (Lusardi et al., 2020). However, agricultural development, surface diversions, and groundwater pumping have now disconnected most of the lower Little Shasta River into isolated pools during the irrigation season, limiting access to high-quality, cold-water habitat in the foothills reach that may be conducive to supporting juvenile coho salmon (Nichols et al., 2016). Thus, restoration of each of the three reaches in the Little Shasta River, including both environmental flow allocations and physical habitat improvements, is considered a high priority for conserving threatened populations of anadromous fishes and the diverse aquatic and riparian communities in the watershed (Lukk et al., 2019).
To assess the flows needed to support ecological functions within the Little Shasta River, three locations of interest (LOI) were chosen that represented varying flow and habitat conditions within the watershed. The foothills reach (LOI 3) has an active stream gage and is downstream of Cold Springs (Figure 1). Flow in this reach is provided by surface runoff from the headwaters and spring flow from several key discrete groundwater sources. Inputs from the cold-water springs provide suitable temperatures and high nutrients for primary and secondary production crucial for rearing native fish, particularly under warming climate conditions that may adversely affect stream temperature conditions and limit salmonid habitat suitability (Isaak et al., 2015; Isaak et al., 2018). Two additional locations of interest were chosen in the upper and lower portions of the bottomlands reach where effects from surface diversions, agricultural use, and groundwater levels influence wetland habitat and streamflow during various seasons (LOI 2 and LOI 1, Figure 1). LOI 2 occurs where the stream transitions into the bottomlands reach and shallow groundwater interacts with surface water conditions and adjacent wetland habitat. LOI 1 is at the downstream end of the bottomlands reach, where the cumulative influence of runoff and groundwater accretion occurs in the watershed near its confluence with the Shasta River.
2.2.2 Cosumnes River
The Cosumnes River is the largest undammed river on the west side of the Sierra Nevada range in California. Located between the American and Mokelumne River watersheds and originating at 2,315 m in elevation, the Cosumnes River flows from the granite-dominated forested Sierra Nevada mountains 130 km westward to the San Francisco-Bay Delta via its confluence with the Mokelumne River in the Central Valley (Figure 3). The lower Cosumnes River in the Central Valley is a low gradient alluvial floodplain stream that is supported by unregulated surface runoff (winter rainfall and spring snowmelt) from the upper mountainous watershed and a complex of shallow perched aquifers and a deep expansive aquifer underlaying the entire Central Valley (Robertson-Bryan, 2006). The lower river and its connected floodplain include extensive GDEs, including the largest remaining Central Valley riparian forest, that support a diverse native fish assemblage and hundreds of species of migratory birds (Kleinschmidt Associates, 2008). In addition, the lower watershed supports thousands of acres of productive agricultural land and several local communities.
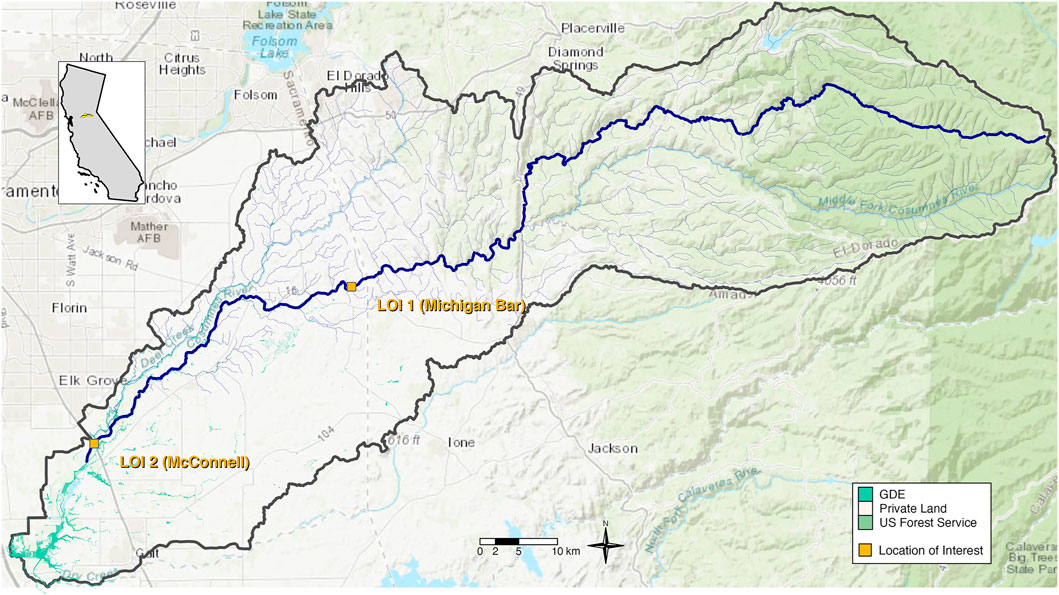
FIGURE 3. The lower Cosumnes River watershed in Northern California. Groundwater dependent ecosystems (GDEs) are shown as green shaded polygons. Locations of interest are shown as orange squares. Background images shows topographic map with elevation contours and private versus public (US Forest Service) land designation. LOI 1 is coincident with USGS gage 11335000 in the upper reach and is currently active. LOI 2 is coincident with USGS gage 11336000 in the lower reach and is not currently active.
Prior to European settlement in the mid-19th century, the lower Cosumnes river system was comprised of a series of shallow anastomosing fluvial channels grading into a complex of stream channels, seasonal marshes, and “lagunitas” or perennial floodplain lakes near the confluence with the San Francisco-Bay Delta that supported a wealth of biodiversity (Wiener, 2021). However, agricultural development in the late 1800s and early 1900s leveled the floodplains, leveed the main stream channel, and converted the river system into a deepened single channel corridor with little floodplain connectivity. Decades of groundwater overdraft and uncoordinated stream diversions have contributed to diminished river flows in the lower Central Valley reaches, particularly in the summer and autumn dry season when some reaches periodically go dry (Wiener, 2021). For anadromous fish species, such as Central Valley Fall-run Chinook salmon (Oncorhynchus tshawytscha) and Central Valley steelhead trout (Oncorhynchus mykiss) that are key conservation priorities, a lack of suitable flows in the channel for migration and spawning in autumn and winter and limited access to an inundated floodplain for juvenile rearing in spring has contributed to precipitous population declines (National Marine Fisheries Service, 2014). As a result, resource agencies, agricultural entities, and other stakeholders are working under the auspices of the Sustainable Groundwater Management Act to address groundwater basin aquifer sustainability, characterize surface-groundwater interactions, understand the related GDEs, and measure groundwater recharge and use. However, integration between groundwater and surface water hydrology is needed in order to inform the development of environmental flow standards supportive of the ecological functionality required by anadromous fish and other aquatic and riparian species.
Unlike other Sierra Nevada watersheds, the Cosumnes River is not impacted by large dams that capture runoff and sediment. As such, the flow regime in the lower watershed reflects winter rain-dominated surface runoff with the influence of snowmelt from the upper watershed in spring, particularly in wetter colder years when the Sierra snowpack is more substantial. Summer baseflows in the dry season are sustained by a combination of low flows from the upper watershed and groundwater contributions from shallow perched aquifers and the larger underlying deep aquifer when conditions are appropriate.
Currently, the lower Cosumnes River can be described as three contiguous stream reaches with slightly differing conditions, constraints, and opportunities with regard to surface-groundwater interactions and flow functionality. The upper reach extends from the base of the forested foothills where the river emerges into the Central Valley to about 13 km downstream where herbaceous and shrub land cover dominates and channel gradient further decreases (Figure 3). Levees are less ubiquitous in this upper reach allowing for river adjustment during high flows and local in-channel deposition of sediments contributing to channel diversity. Channel flows seasonally connect to the primary underlying aquifer such that groundwater levels range from 0 m to approximately 30 m below ground surface (bgs) depending on the time of year and extent of river flow (Wiener, 2021). Low groundwater levels in late summer and autumn in particular contribute to drying of the stream channel in this losing reach such that when fall precipitation begins, elevated streamflows are often ‘lost’ to the underlying channel sediments until enough flow has saturated the subsurface and local groundwater levels have increased enough to limit seepage losses supporting higher sustained river flow. The middle reach of the lower Cosumnes River extends through predominantly pasture and agricultural land across the valley floodplain for approximately 27 km to the Highway 99 crossing where floodplain connectivity increases and tidal influences from the Delta downstream begin to affect flow conditions. Throughout the middle valley reach, channel levees are frequent, the river is deeply incised, and only the largest floods inundate the floodplain in the few locations with levee setbacks. Channel flows are fully disconnected from the primary aquifer, which is typically 12–30 m bgs throughout the year; however, seepage from the channel during winter and spring flows saturate the adjacent channel areas helping to support riparian vegetation and recharge local shallow perched aquifers (Wiener, 2021). Similar to the upper reach, channel flows in autumn only become sustained when enough flow has saturated the local subsurface and remains high enough in volume to overcome channel seepage rates. The lower stream reach extends downstream of the highway about 18 km to the river confluence with the Delta. The river is less incised in this reach with fewer levees that are set-back or breached from floodplain restoration projects to allow for more frequent floodplain inundation at lower flows. At its most downstream end, the river is comprised of multiple tidally-influenced channels that shift across the lower floodplain and support the most diverse aquatic and riparian habitat in the lower watershed. Due to higher groundwater levels (<10 m bgs) partially controlled by tidal backwater influences in the Delta, surface flows in this lower reach are seasonally connected to the primary underlying aquifer and support riparian and wetland vegetation throughout the floodplain. Frequent floodplain inundation during high flows contributes large volumes of water to groundwater recharge helping to maintain elevated groundwater levels and surface water-groundwater connectivity throughout much of the year.
To assess ecological flow needs within the lower Cosumnes River, two locations of interest were selected that represent various habitat conditions within the lower watershed. One location of interest (LOI 2) was chosen at the transition from the upper to middle reach where an active stream gage is located (Figure 3), providing long-term daily flow dating from 1908 to present. This upper location characterized the river where channel incision is high and groundwater levels are low, but perched aquifers help to support riparian vegetation and GDEs adjacent to the channel. A second location of interest (LOI 1) was chosen at the transition from the middle to lower reach, where floodplain restoration projects have increased floodplain connectivity and past research provides additional information on local groundwater conditions (Figure 3). This downstream location characterized surface flow, groundwater conditions, and habitats supportive of the extensive GDEs located throughout the lower watershed. A previously maintained flow gage was also located at this lower location, providing daily flow data from 1942 to 1982.
3 Results
Comprehensive descriptions of the environmental flow assessments following CEFF are provided in technical reports for the Little Shasta River (Yarnell et al., 2021) and for the Lower Cosumnes River (Yarnell and Obester, 2021). Here we provide a summary of the outcomes from CEFF sections A and B at each study site, with a focus on how groundwater influences were accounted for in the determination of ecological flow needs that support stream functionality.
3.1 Accounting for Groundwater Contributions
The natural range of functional flows estimated from models in CEFF section A reflected a flow regime dominated by surface runoff hydrology. These models include predictor variables that characterize the climatic and physical characteristics of the contributing watershed area, including precipitation, temperature, geology, elevation, and drainage area (Grantham et al.). Although baseflow contributions are potentially accounted for in a groundwater recharge index in the models (Wolock, 2003), the predicted baseflow components were generally underestimated at the two study sites, indicating that the models were not capturing the effects of local groundwater or spring contributions. For example, at LOI 3 in the foothills reach of the Little Shasta River, predicted dry season baseflow magnitude ranged from 1–20 cfs, averaging 9 cfs (Table 1), which reflected the range in surface water runoff across wet and dry years. However, additional year-round groundwater discharge of 10 cfs from Cold Spring, just upstream of LOI 3 (Figure 2), would nearly double the estimates of natural summer baseflow. Downstream at LOI 2 and LOI 3 (Figure 1), cumulative additions of up to 20 cfs from upstream springs (Figure 2) would also substantially increase dry season and wet season baseflows. To account for groundwater contributions not reflected in the models, we added these discrete spring volumes to the predicted dry season and wet season baseflow magnitudes for the Little Shasta River in Section B. At LOI 3 in the foothills reach (Figure 1), we added 10 cfs from Cold Spring located just upstream (Table 1), and an additional 20 cfs to each of the two LOIs in the bottomlands reach, reflecting the cumulative spring contributions from the upstream headwaters and foothills reaches.
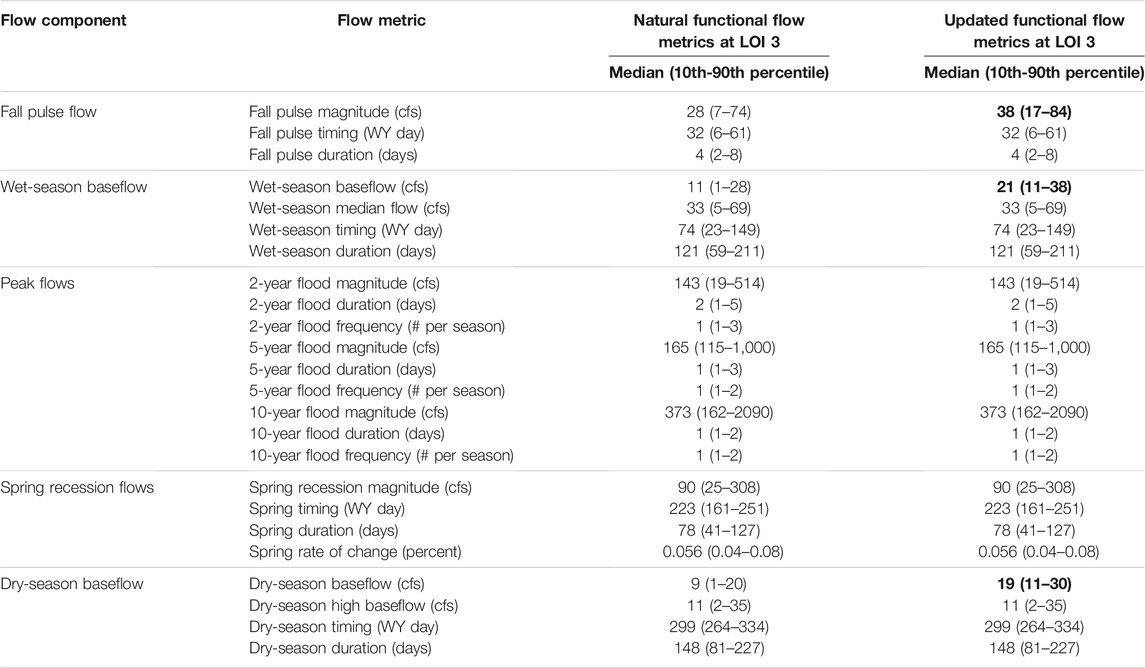
TABLE 1. Natural functional flow metrics from CEFF Section A and updated functional flow metrics from Section B accounting for spring contributions at LOI 3 in the foothills reach of the Little Shasta River. Values reflect medians and 10th–90th percentiles of functional flow metrics for all water year types combined. Values that were updated are in bold. Magnitude metrics are expressed in cubic feet per second (cfs) and timing metrics are expressed in day of Water Year, where day 1 = October 1).
We also evaluated the potential for subsurface groundwater inputs from locally adjacent high groundwater levels to support and sustain baseflow conditions during the dry season at each site. Although limited data was available to quantify the interactions between surface flow, groundwater, and the associated GDEs in both the lower reaches of the Little Shasta River and the lower Cosumnes River, groundwater modeling results from ongoing studies in both basins indicated that portions of these streams vary between gaining and losing conditions as they traverse their respective valleys. In the Little Shasta River, modeled losses to or gains from groundwater appear to be small relative to spring contributions (pers comm, L. Foglia), but additional study will provide insight to whether gaining reaches may prolong higher baseflow duration, support higher soil moisture in riparian areas, and contribute to healthier conditions for GDEs. Thus, no further adjustments accounting for subsurface flow were made at this time to the dry season baseflow magnitudes in the Little Shasta River (Table 1). Similarly, adjacent perched aquifers in the lower Cosumnes River may contribute to higher baseflow and extended baseflow duration during the dry season. Previous studies on subsurface stratigraphy, groundwater elevations, and surface water-groundwater connectivity in the lower Cosumnes watershed have linked lowered groundwater elevations to disconnection of surface flows in the channel, but the contributions of discrete local perched aquifers remains unknown (Wiener 2021). Thus, we chose to retain the predicted dry season baseflow magnitudes from section A as ecological flow needs in the lower Cosumnes River until further study can be completed (Table 2). Ongoing and new research in both study basins will help further quantify surface-groundwater connections and aid in determining groundwater levels needed to support stream functionality during the dry season. These future studies will also inform whether dry season baseflows should be adjusted in the future to account for shallow subsurface groundwater contributions, particularly in the lower Cosumnes River.
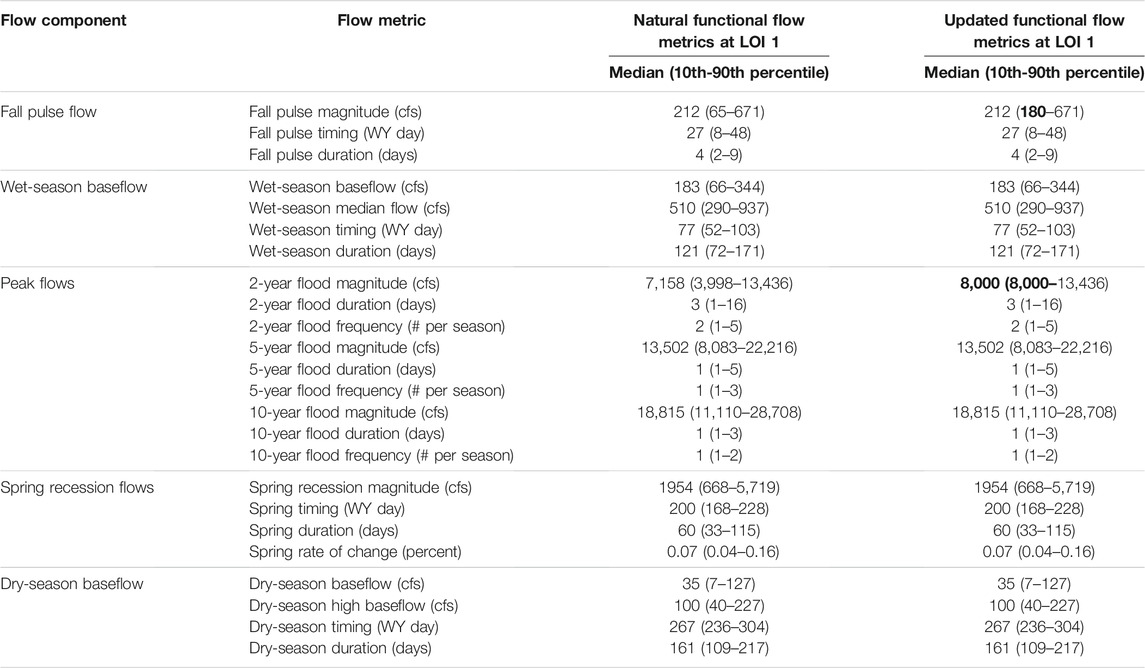
TABLE 2. Natural functional flow metrics from CEFF Section A and updated functional flow metrics from Section B accounting for altered channel morphology at LOI 1 in the upper reach of the lower Cosumnes River. Values reflect medians and 10th–90th percentiles of functional flow metrics for all water year types combined. Values that were updated are in bold. Magnitude metrics are expressed in cubic feet per second (cfs) and timing metrics are expressed in day of Water Year, where day 1 = October 1).
3.2 Channel Morphology Controls on Streamflow Interactions
The Little Shasta River and Cosumnes River watersheds are less developed than many California watersheds in that they are free of large hydroelectric or water supply dams, leaving natural geomorphic and hydrologic processes largely intact. However, once the rivers reach their downstream valleys, the stream channels are incised to varying degrees throughout their lower reaches potentially affecting streamflow interactions with the floodplain and adjacent groundwater levels. In the Little Shasta River, much of the stream through the bottomlands reach is confined to a single asymmetric channel that constrains baseflow and moderate flows (such as the fall pulse flow) to limited connection with riparian areas. However, analysis of a LIDAR-derived DEM available for the Shasta basin (TerraPoint USA, 2008) suggests that channel incision is modest: peak flows, such as the 2-year flood of 143 cfs, inundate the adjacent floodplain and support riparian recruitment. Thus, the predicted natural range of functional flow metrics from section A for the wet season peak flows and spring recession flow would likely provide expected functionality, and we chose not to adjust the metrics (Table 1). However, as the stream was likely historically a multi-channel system typical of wetlands where lower flows provided greater lateral connectivity and supported GDEs, consideration of channel rehabilitation actions that promote habitat complexity and increase riparian interactions at lower flows is needed.
Conversely, in the middle reach of the lower Cosumnes River between LOI 1 and LOI 2 (Figure 3), the channel was incised such that only flows greater than approximately 8,000 cfs inundated the floodplain (USFWS, 2001). Given the predicted median 2-year flood flow was 7,158 cfs and the predicted wet season median flow was 560 cfs at LOI 1 (Table 2), we expected flows between 1,000 cfs (greater than the wet season median flow) and 7,158 cfs (less than the 2-year flood flow) to at least partially inundate the floodplain during most years. We therefore increased the 2-year flood flow magnitude to 8,000 cfs to ensure more frequent floodplain inundation and support for associated floodplain functions, such as groundwater recharge, riparian recruitment, and extended inundation of GDE habitats (Table 2). Additional results from USFWS (2001) also showed that a minimum of 180 cfs at each LOI in the lower Cosumnes River would be needed to allow salmonid migration and passage during autumn under current physical habitat conditions. This is comparable to the predicted fall pulse flow median magnitude of 212 cfs (range 65–671 cfs) at LOI 1 and 239 cfs at LOI 2 (Table 2). Therefore, we chose to revise the minimum magnitude for the fall pulse flow to reflect the minimum salmonid passage flow of 180 cfs, but retained the remaining range of fall pulse flows to support interannual flow variability.
3.3 Groundwater Effects on Stream Water Quality
Previous studies in the Little Shasta basin have explored the relationship between water quality conditions, including water temperature, and native fish habitat suitability in the foothills and bottomlands reaches, where impacts from grazing and flow diversions result in warm water temperatures, shifts in stream nutrients, and limited riparian cover in some locations (Nichols et al., 2016; Lukk et al., 2019). In the foothills reach under current conditions where spring flows are fully diverted and streamflow is predominantly surface runoff, water temperature and nutrient data from 2016 to 2018 showed that the number of over 20°C days (and thus physiologically stressful for salmonids) increased from upstream to downstream regardless of year, and that nitrogen was the limiting factor on productivity in the reach (Lukk et al., 2019). However, macroinvertebrate data showed water quality conditions were “good” in the upper foothills reach, based on the Hilsenhoff’s Biotic Index (Hilsenhoff, 1987). Below the diversions at the downstream end of the foothills reach, seasonally occurring low-flow and no-flow conditions disconnected the bottomlands from the foothills, and resultingly, macroinvertebrate data indicated “fairly poor” water quality conditions. Thus, while the upper foothills reach currently provides suitable physical, chemical, and biological conditions to support a healthy cold-water ecosystem under predominantly surface runoff conditions, limited productivity from low nutrient content and resulting limited food resources suggest that juvenile salmonids may be less able to tolerate elevated water temperatures in the reach, even for short periods. Increasing groundwater contributions from the springs to the channel will likely have a large impact on keeping water temperatures low and supplementing aquatic productivity. Therefore, we chose to retain the dry season baseflow magnitudes adjusted for spring flow contributions, with no additional adjustments for water quality considerations, presuming that cold nutrient-rich spring flow comprises the additional 10 cfs in dry season baseflow (Table 1).
Previous studies regarding water quality conditions in the lower Cosumnes River have primarily focused on nutrients and pollutants associated with agricultural runoff, point sources, and land uses, rather than water temperatures. While elevated water temperatures during the dry season have been noted as one of many causes of decline in native fishes throughout Central Valley streams (USFWS, 2001), the extensive riparian forest and connected floodplains in the lower reaches help buffer high water temperatures during the warm dry season when sufficient baseflow is available (Robertson-Bryan, 2006). The primary water quality concerns in the lower Cosumnes River are potentially high levels of nitrogen, phosphorus, suspended sediments, and mercury related to agricultural drainage and pesticide use (Robertson-Bryan, 2006). Previous studies on water chemistry showed that the majority of nutrients and suspended sediments in the lower watershed originated from both point sources (e.g. wastewater treatment facilities) and non-point sources (e.g. urban and agricultural runoff), with nutrient concentrations, conductivity, and pH levels generally increasing from upstream to downstream (Ahearn et al., 2005). During the dry season baseflow, in stream reaches that were not dry, nutrient concentrations increased as flows decreased and shallow groundwater contributions from perched aquifers became the dominant source of baseflow. However, sediment and nutrient levels remained within water quality criteria delineated by local regulations (Ahearn et al., 2004). During the early wet season in autumn, the fall pulse flow and early storm events flushed nutrients through the river system, and then in the late wet season as progressive flushing had occurred, conductivity and nutrient concentrations decreased with successive high flows. As a result, in most years, almost the entire annual load of nutrients and sediment moved through the watershed during the wet season (Ahearn et al., 2004). These studies suggest that while water quality may be a concern for aquatic species in the lower Cosumnes River under extreme low flow conditions, when sufficient baseflows occur in the dry season and periodic flushing flows occur during the wet season, water quality conditions likely remain suitable for native aquatic species. We thus chose to retain the natural predicted range of dry season baseflow magnitudes from section A (Table 2) as supportive of suitable water quality conditions throughout the lower reaches, particularly where riparian areas are intact.
4 Discussion
Accounting for groundwater interactions in environmental flow development requires a holistic approach that encompasses evaluation of surface-groundwater interactions and their relationship with channel morphology, local geology, water quality conditions, and aquatic and riparian communities. Application of CEFF to the Little Shasta River and lower Cosumnes River provided a guided but flexible approach to determining ecological flow needs in these groundwater-influenced streams that more accurately reflected hydrologic conditions than other traditional methods that singularly focus on volumetric surface flow conditions or single-species habitat suitability approaches. CEFF provided an initial set of ecological flow needs that were centered around seasonal components of the flow regime that support ecosystem functionality and were derived from predominantly surface runoff characteristics and conditions. However, the flexibility of CEFF allowed for assessment and inclusion of spring flow contributions and groundwater influences, as well as detailed analysis of when and where groundwater influences were most important in each watershed. The focus on ecosystem functions in CEFF, and understanding the importance of groundwater to those functions, provides the opportunity for discussion of management strategies that specifically support groundwater conditions and address the surface-groundwater connectivity that supports groundwater dependent ecosystems.
In California and other semi-arid environments where development of groundwater sources for agricultural use and consumption is common, the loss of groundwater contributions to stream ecosystems can be particularly acute. In the case of the Little Shasta River, depleted streamflows during the summer dry season have impacted both aquatic and riparian communities, including high-profile wild salmon populations. The ecological flow needs analysis completed in CEFF not only provided specific baseflow values needed to improve stream functionality and associated habitat conditions, but also highlighted the critical need for a portion of baseflow to be supplied from nutrient-rich cold spring flow, rather than solely from other warm surface flow sources, in order to provide suitable water quality conditions for benthic invertebrates and native fishes (cf. Lusardi et al., 2016). Similarly, in the lower Cosumnes River, floodplain inundation that promotes groundwater recharge to the shallow aquifer may support seepage of cooler higher quality water to summer baseflow than warmer surface water from agricultural runoff. In both streams, holistic evaluation of the relative importance of groundwater contributions to stream ecosystem dynamics allows for more comprehensive evaluation than traditional species-based approaches and provides additional options for management decisions that support both human uses and sustainability of key refugia for native biota under a rapidly changing climate.
For the Little Shasta River and lower Cosumnes River, stakeholder discussions regarding how to best manage water allocations to provide environmental flow needs and meet agricultural water demands are ongoing. As outlined in section C of CEFF, these discussions include accurate assessments of water use and streamflow alteration, analysis of trade-offs between water use for agriculture and ecological needs, evaluation of management actions that support surface-groundwater interactions and connectivity, and development of monitoring and adaptive management plans. The streamflow alteration analysis in both basins indicated that dry season baseflows are depleted and likely altered (Yarnell and Obester, 2021; Yarnell et al., 2021). Depleted baseflow in the Little Shasta River likely reflects spring flow and surface flow withdrawals (all discrete springs in the basin are currently fully diverted to off-channel uses), small losses to groundwater due to underlying geologic conditions, and, in the bottomlands reach, decreased groundwater elevations associated with groundwater pumping. Similarly, depleted baseflow in the lower Cosumnes River likely reflects small losses to groundwater due to underlying geologic conditions and decreased groundwater elevations associated with groundwater pumping. Information from additional studies quantifying surface-groundwater interactions and contributions from perched aquifers and saturated conditions will help inform the groundwater levels needed to support stream functionality for aquatic species and GDEs during the dry season.
Based on varying hydrogeomorphic conditions throughout each of the reaches in the Little Shasta and lower Cosumnes River, particularly with respect to seasonal connections between surface water and groundwater, several potential management actions could be undertaken in CEFF Section C to improve ecological functionality and robustness. In the foothills reach of the Little Shasta River, where habitat is generally in good condition, actions might include limiting spring water diversions in an effort to support prolonged dry season baseflow with high quality, cold, nutrient-rich water, limiting seasonal groundwater withdrawals to maintain shallow groundwater levels and limit channel seepage losses, and funding support for supplemental water sources for agriculture, such as winter runoff diversions, use of recycled water, and voluntary water use efficiency improvements. In the bottomlands reach of the Little Shasta River, where habitat conditions are poor and a lack of summer baseflow has impacted the aquatic biota in particular, similar actions to those above could be taken as well as actions to reduce channel incision and improve lateral connectivity within riparian areas in an effort to support GDEs. In the lower Cosumnes River, Mount et al. (2001) presented a three-part strategy to improving baseflow conditions that included augmentation of surface flows, management of groundwater pumping, and restoration of natural flood regimes. Building on this strategy for the lower Cosumnes basin, additional management actions that support groundwater contributions may include: managed recharge to primary and perched aquifers, which would benefit surface water-groundwater connections and agricultural water supply; floodplain reconnection, levee relocation/set-backs, and habitat restoration projects to promote riparian and groundwater dependent habitats and improve flood management; and relocation of shallow wells next to the river channel, particularly in perched aquifers, and use of alternative water sources such as stored winter diversions, recycled water, and voluntary water use efficiency improvements. As discussion and evaluation of management actions by stakeholders in each basin continues, consideration of their potential effects on the desired ecological objectives identified in section A should be included. Further study and quantification of the ecological consequences of failing to satisfy ecological flow needs may help in evaluating trade-offs inherent in meeting ecological and non-ecological management objectives. Monitoring plans should, therefore, explicitly measure surface flow conditions, groundwater conditions in both perched and deep aquifers, changes in channel morphology, and the health and suitability of channel and floodplain habitats for aquatic species and GDEs linked to these conditions (Rohde et al., 2017). The ecological conditions and current management practices in the Little Shasta River and lower Cosumnes basin are representative of many groundwater-influenced streams in agricultural landscapes, and thus the management approaches recommended here could apply broadly to similar river systems.
5 Conclusion
Groundwater-influenced streams and their associated GDEs are key climate refugia for arid and semi-arid ecosystems, such as those in California. Under changing climate conditions where extreme hydrologic conditions such as floods and droughts are increasing, water management frameworks that explicitly integrate groundwater and surface water conditions are needed to meet ecological flow needs and determine environmental flows that will support functioning river ecosystems and the aquatic community, improve river health, and sustain the freshwater ecosystem services upon which human societies depend. CEFF provides a flexible framework that is focused on the functionality of flow and incorporates consideration of the interconnections between groundwater, surface runoff, channel morphology, and water quality conditions. We found that application of CEFF to two groundwater-influenced streams in California provided a means to determine ecological flow needs that accounted for groundwater contributions and their interactions with channel morphology and water quality to holistically support ecological functionality. The results will aid ongoing discussions of management actions that support groundwater contributions within each stream and ultimately help to support climate resilient habitats in these watersheds.
Data Availability Statement
Publicly available datasets were analyzed in this study. This data can be found here: Natural functional flow metrics for California: rivers.codefornature.org.
Author Contributions
SY led the preparation of this manuscript. SY, AW, RP, RL, JZ, and TG contributed to completion of the Little Shasta River case study. SY and AO contributed to completion of the Cosumnes River case study. SY, AW, AO, RP, RL, JZ, TG, and ES contributed to the development of the California Environmental Flows Framework, concepts guiding the application of the approach for each case study, and to writing this manuscript. The authors approve this work for publication and declare that the research was conducted in the absence of any commercial or financial relationships that could be construed as a potential conflict of interest.
Funding
Funding for this work was provided by the California Wildlife Conservation Board (Agreement WC-1849AB), American River Conservancy, and The Nature Conservancy. Open access publication fees were provided by University of California, Davis library.
Conflict of Interest
The authors declare that the research was conducted in the absence of any commercial or financial relationships that could be construed as a potential conflict of interest.
Publisher’s Note
All claims expressed in this article are solely those of the authors and do not necessarily represent those of their affiliated organizations, or those of the publisher, the editors and the reviewers. Any product that may be evaluated in this article, or claim that may be made by its manufacturer, is not guaranteed or endorsed by the publisher.
Acknowledgments
We would like to thank members of the Environmental Flows Working Group of the California Water Quality Monitoring Council for valuable feedback on the California Environmental Flows Framework. We would like to thank the Hart Ranch and other private landowners for providing access to their properties in the Little Shasta River. We would like to thank the Cosumnes Coalition for their valuable insights regarding the Cosumnes watershed and for their feedback on the CEFF application to the lower Cosumnes River.
References
Adams, F., Harding, S., Robertson, R., and Tait, C. (1912). Reports on the Irrigation Resources of California, Irrigation Investigations, Office of Experiment Stations. Washington, D.C., United States: U.S. Department of Agriculture.
Ahearn, D. S., Sheibley, R. W., Dahlgren, R. A., Anderson, M., Johnson, J., and Tate, K. W. (2005). Land Use and Land Cover Influence on Water Quality in the Last Free-Flowing River Draining the Western Sierra Nevada, California. J. Hydrol. 313, 234–247. doi:10.1016/j.jhydrol.2005.02.038
Ahearn, D. S., Sheibley, R. W., Dahlgren, R. A., and Keller, K. E. (2004). Temporal Dynamics of Stream Water Chemistry in the Last Free-Flowing River Draining the Western Sierra Nevada, California. J. Hydrol. 295 (1-4), 47–63. doi:10.1016/j.jhydrol.2004.02.016
Barlow, P. M., and Leake, S. A. (2012). Streamflow Depletion by wells— Understanding and Managing the Effects of Groundwater Pumping on Streamflow. Reston, Virginia, U.S: U.S. Geology Survey.
Cooley, S. W., Ryan, J. C., and Smith, L. C. (2021). Human Alteration of Global Surface Water Storage Variability. Nature 591 (7848), 78–81. doi:10.1038/s41586-021-03262-3
Cunjak, R. A. (1988). Physiological Consequences of Overwintering in Streams: The Cost of Acclimitization? Can. J. Fish. Aquat. Sci. 45 (3), 443–452. doi:10.1139/f88-053
CWQMC-EFW (2021). California Environmental Flows Framework. Version 1.0. California: California Water Quality Monitoring Council - Environmental Flows Workgroup. Available at: https://ceff.ucdavis.edu/tech-report.
Davidson, R. S., Letcher, B. H., and Nislow, K. H. (2010). Drivers of Growth Variation in Juvenile Atlantic salmon (Salmo salar): an Elasticity Analysis Approach. J. Anim. Ecol. 79 (5), 1113–1121. doi:10.1111/j.1365-2656.2010.01708.x
Gómez-Baggethun, E., Tudor, M., Doroftei, M., Covaliov, S., Năstase, A., Onără, D.-F., et al. (2019). Changes in Ecosystem Services from Wetland Loss and Restoration: An Ecosystem Assessment of the Danube Delta (1960-2010). Ecosystem Serv. 39, 100965. doi:10.1016/j.ecoser.2019.100965
Grantham, T. E., Carlisle, D. M., Howard, J., Lane, B. A., Lusardi, R. A., Obester, A., et al. Modeling Functional Flows in California’s Rivers. Front. Environ. Science-Freshwater Sci. in review.
Grantham, T. E., Mount, J. F., Stein, E. D., and Yarnell, S. M. (2020). Making the Most of Water for the Environment: A Functional Flows Approach for California Rivers. San Francisco, California, United States: Public Policy Institute of California. Available at: https://www.ppic.org/publication/making-the-most-of-water-for-the-environment/.
Grill, G., Lehner, B., Thieme, M., Geenen, B., Tickner, D., Antonelli, F., et al. (2019). Mapping the World's Free-Flowing Rivers. Nature 569 (7755), 215–221. doi:10.1038/s41586-019-1111-9
Hilsenhoff, W. L. (1987). An Improved Biotic index of Organic Stream Pollution. Great Lakes Entomologist 20, 31–39.
Horne, A., Webb, J. A., Stewardson, M., Richter, B., and Acreman, M. (2017). Water for the Environment: From Policy and Science to Implementation and Management. Cambridge, Massachusetts, United States: Academic Press.
Howard, J., and Merrifield, M. (2010). Mapping Groundwater Dependent Ecosystems in California. Plos One 5 (6), e11249. doi:10.1371/journal.pone.0011249
Isaak, D. J., Luce, C. H., Horan, D. L., Chandler, G. L., Wollrab, S. P., and Nagel, D. E. (2018). Global Warming of Salmon and Trout Rivers in the Northwestern U.S.: Road to Ruin or Path through Purgatory? Trans. Am. Fish. Soc. 147 (3), 566–587. doi:10.1002/tafs.10059
Isaak, D. J., Young, M. K., Nagel, D. E., Horan, D. L., and Groce, M. C. (2015). The Cold-Water Climate Shield: Delineating Refugia for Preserving Salmonid Fishes through the 21st century. Glob. Change Biol. 21 (7), 2540–2553. doi:10.1111/gcb.12879
Jasechko, S., Seybold, H., Perrone, D., Fan, Y., and Kirchner, J. W. (2021). Widespread Potential Loss of Streamflow into Underlying Aquifers across the USA. Nature 591 (7850), 391–395. doi:10.1038/s41586-021-03311-x
Kleinschmidt Associates, (2008). Cosumnes River Preserve Management Plan. Grass Valley. California: The Nature Conservancy.
Lehner, B., Liermann, C. R., Revenga, C., Vörösmarty, C., Fekete, B., Crouzet, P., et al. (2011). High‐resolution Mapping of the World's Reservoirs and Dams for Sustainable River‐flow Management. Front. Ecol. Environ. 9 (9), 494–502. doi:10.1890/100125
Loheide, S. P., and Booth, E. G. (2011). Effects of Changing Channel Morphology on Vegetation, Groundwater, and Soil Moisture Regimes in Groundwater-dependent Ecosystems. Geomorphology 126 (3-4), 364–376. doi:10.1016/j.geomorph.2010.04.016
Lukk, A. K., Vasquez-Housley, P., Lusardi, R. A., and Willis, A. D. (2019). Little Shasta River 2017-2019: Pre-project Assessment of the Proposition 1 Ecosystem Restoration Grant Activities. Sacramento, CA: California Wildlife Conservation Board.
Lusardi, R. A., Bogan, M. T., Moyle, P. B., and Dahlgren, R. A. (2016). Environment Shapes Invertebrate Assemblage Structure Differences between Volcanic spring-fed and Runoff Rivers in Northern California. Freshw. Sci. 35 (3), 1010–1022. doi:10.1086/687114
Lusardi, R. A., Hammock, B. G., Jeffres, C. A., Dahlgren, R. A., and Kiernan, J. D. (2020). Oversummer Growth and Survival of Juvenile Coho salmon (Oncorhynchus kisutch) across a Natural Gradient of Stream Water Temperature and Prey Availability: an In Situ Enclosure experiment. Can. J. Fish. Aquat. Sci. 77 (2), 413–424. doi:10.1139/cjfas-2018-0484
Lusardi, R. A., Nichols, A. L., Willis, A. D., Jeffres, C. A., Kiers, A. H., Van Nieuwenhuyse, E. E., et al. (2021). Not All Rivers Are Created Equal: The Importance of Spring-Fed Rivers under a Changing Climate. Water 13 (12), 1652. doi:10.3390/w13121652
McBain and Trush (2013). Study Plan to Assess Shasta River salmon and Steelhead Recovery Needs. Washington, D.C., United States: US Fish and Wildlife Service.
Milhous, R. T., Updike, M. A., and Schneider, D. M. (1989). Physical Habitat Simulation System (PHABSIM) Reference Manual. Version II. Washington, D.C., United States: U.S. Fish and Wildlife Service.
Mount, J. F., Fogg, G., Kavvas, L., Fleckenstein, J., Anderson, M., Chen, Z. Q., et al. (2001). Linked Surface Water-Groundwater Model for the Cosumnes River Watershed: Hydrologic Evaluation of Management Options to Restore Fall Flows. Washington, D.C., United States: U.S. Fish and Wildlife Service Anadromous Fish Restoration Program.
Moyle, P. B. (2002). Inland Fishes of California: Revised and Expanded. Berkeley, California, United States: University of California Press.
Moyle, P. B., Lusardi, R. A., Samuel, P., and Katz, J. (2017). State of the Salmonids: Status of California's Emblematic Fishes 2017. San Francisco, California: California Trout.
National Marine Fisheries Service (2014). Recovery Plan for the Evolutionarily Significant Units of Sacramento River Winter-run Chinook Salmon and Central Valley Spring-run Chinook Salmon and the Distinct Population Segment of California Central Valley Steelhead. Sacramento, California: National Marine Fisheries Service, California Central Valley Area Office.
National Resources Council (NRC) (2004). Endangered and Threatened Fishes in the Klamath River Basin: Causes of Decline and Strategies for Recovery. Washington, D.C., United States: National Academies Press.
Nichols, A. L., Willis, A. D., Lambert, D., Limanto, E., and Deas, M. L. (2016). Little Shasta River Hydrologic and Water Temperature Assessment: April to December 2015. Elk Grove, California: The Nature Conservancy.
Obester, A. N., Lusardi, R. A., Santos, N. R., Peek, R. A., and Yarnell, S. M. (2021). The Use of Umbrella Fish Species to Provide a More Comprehensive Approach for Freshwater Conservation Management. Aquat. Conserv: Mar. Freshw. Ecosyst., 1–17. doi:10.1002/aqc.3746
Poff, N. L., Allan, J. D., Bain, M. B., Karr, J. R., Prestegaard, K. L., Richter, B. D., et al. (1997). The Natural Flow Regime. Bioscience 47 (11), 769–784. doi:10.2307/1313099
Reid, A. J., Carlson, A. K., Creed, I. F., Eliason, E. J., Gell, P. A., Johnson, P. T. J., et al. (2019). Emerging Threats and Persistent Conservation Challenges for Freshwater Biodiversity. Biol. Rev. 94 (3), 849–873. doi:10.1111/brv.12480
Robertson-Bryan, I. (2006). Lower Cosumnes River Watershed Assessment". Elk Grove, California: The Nature Conservancy.
Rohde, M. M., Froend, R., and Howard, J. (2017). A Global Synthesis of Managing Groundwater Dependent Ecosystems under Sustainable Groundwater Policy. Groundwater 55 (3), 293–301. doi:10.1111/gwat.12511
Spence, R., and Hickley, P. (2000). The Use of PHABSIM in the Management of Water Resources and Fisheries in England and Wales. Ecol. Eng. 16 (1), 153–158. doi:10.1016/s0925-8574(00)00099-9
Stein, E. D., Zimmerman, J., Yarnell, S. M., Stanford, B., Lane, B., Taniguchi-Quan, K. T., et al. (2021). The California Environmental Flows Framework: Meeting the Challenges of Developing a Large-Scale Environmental Flows Program. Front. Environ. Sci. 9, 769943. doi:10.3389/fenvs.2021.769943
TerraPoint Usa, I. (2008). Shasta Valley Airborn LiDAR Survey. Yreka, California: Shasta Valley Resource Conservation District.
Tickner, D., Kaushal, N., Speed, R., and Tharme, R. (2020a). Editorial: Implementing Environmental Flows: Lessons for Policy and Practice. Front. Environ. Sci. 8, 106. doi:10.3389/fenvs.2020.00106
Tickner, D., Opperman, J. J., Abell, R., Acreman, M., Arthington, A. H., Bunn, S. E., et al. (2020b). Bending the Curve of Global Freshwater Biodiversity Loss: An Emergency Recovery Plan. Bioscience 70 (4), 330–342. doi:10.1093/biosci/biaa002
USFWS (2001). Anadromous Fish Restoration Program Final Restoration Plan. Washington, D.C., United States: US Fish and Wildlife Service.
van Rees, C. B., Waylen, K. A., Schmidt‐Kloiber, A., Thackeray, S. J., Kalinkat, G., Martens, K., et al. (2021). Safeguarding Freshwater Life beyond 2020: Recommendations for the New Global Biodiversity Framework from the European Experience. Conservation Lett. 14 (1), e12771. doi:10.1111/conl.12771
Vörösmarty, C. J., McIntyre, P. B., Gessner, M. O., Dudgeon, D., Prusevich, A., Green, P., et al. (2010). Global Threats to Human Water Security and River Biodiversity. Nature 467 (7315), 555–561. doi:10.1038/nature09440
Wiener, J. (2021). Review of Groundwater Recharge and Surface Water-Groundwater Interactions for the Lower Cosumnes River. Coloma, California: American River Conservancy.
Yarnell, S. M., and Obester, A. (2021). An Application of the California Environmental Flows Framework to the Lower Cosumnes River, California. Elk Grove, California: The Nature Conservancy.
Yarnell, S. M., Petts, G. E., Schmidt, J. C., Whipple, A. A., Beller, E. E., Dahm, C. N., et al. (2015). Functional Flows in Modified Riverscapes: Hydrographs, Habitats and Opportunities. BioScience 65 (10), 963–972. doi:10.1093/biosci/biv102
Yarnell, S. M., Stein, E. D., Webb, J. A., Grantham, T., Lusardi, R. A., Zimmerman, J., et al. (2020). A Functional Flows Approach to Selecting Ecologically Relevant Flow Metrics for Environmental Flow Applications. River Res. Applic. 36 (2), 318–324. doi:10.1002/rra.3575
Yarnell, S. M., and Thoms, M. The Role of Geomorphic Processes in Maximizing Environmental Flow Functionality and Creating Resilience. Front. Environ. Science-Freshwater Sci. In Review.
Yarnell, S. M., Willis, A. D., Lusardi, R. A., and Peek, R. A. (2021). Functional Flows in the Little Shasta River: Application of the California Environmental Flows Framework. Sacramento, CA: California Wildlife Conservation Board.
Keywords: environmental flows, groundwater management, groundwater-surface water interactions, holistic method, groundwater dependent ecosystems
Citation: Yarnell SM, Willis A, Obester A, Peek RA, Lusardi RA, Zimmerman J, Grantham TE and Stein ED (2022) Functional Flows in Groundwater-Influenced Streams: Application of the California Environmental Flows Framework to Determine Ecological Flow Needs. Front. Environ. Sci. 9:788295. doi: 10.3389/fenvs.2021.788295
Received: 02 October 2021; Accepted: 27 December 2021;
Published: 20 January 2022.
Edited by:
Sergi Sabater, University of Girona, SpainReviewed by:
Gorazd Urbanič, Urbanzero Institute for Holistic Environmental Management, SloveniaJoan Estrany, University of the Balearic Islands, Spain
Copyright © 2022 Yarnell, Willis, Obester, Peek, Lusardi, Zimmerman, Grantham and Stein. This is an open-access article distributed under the terms of the Creative Commons Attribution License (CC BY). The use, distribution or reproduction in other forums is permitted, provided the original author(s) and the copyright owner(s) are credited and that the original publication in this journal is cited, in accordance with accepted academic practice. No use, distribution or reproduction is permitted which does not comply with these terms.
*Correspondence: Sarah M. Yarnell, c215YXJuZWxsQHVjZGF2aXMuZWR1