- 1Shandong Provincial Key Laboratory of Depositional Mineralization and Sedimentary Minerals, College of Earth Science and Engineering, Shandong University of Science and Technology, Qingdao, China
- 2Shandong Institute of Geophysical and Geochemical Exploration, Jinan, China
- 3No. 7 Geological Brigade, Shandong Provincial Bureau of Geology and Mineral Resources, Linyi, China
- 4Natural Resources Survey Institute of Heilongjiang Province, Harbin, China
Soil organic carbon (SOC) is significant for soil quality and global carbon cycles. SOC was observed to be related to soil geochemistry, and soils originating from different bedrocks have different geochemical properties, but the effect of bedrock on SOC is still undefined. Soils overlying different bedrocks in Zhenxiong County and Weixin County were sampled. Specifically, soils in the mineral horizon, which are less affected by the external environment than surface soils, are focused on to reveal the effect of bedrock on SOC. Al/Ti, Fe/Ti, and Al/Fe indicate a soil–rock successive relationship. SOC contents in the mineral horizon are 0.19–2.74% (1.24% on average), and those in the surface horizon are 1.26–4.01% (2.63% on average). SOC contents in the surface and mineral horizons of the same bedrock are significantly positively correlated, implying that the bedrock is an important factor affecting SOC. SOC in the mineral horizon is related to the first transition metal ions. Significantly, positive correlations of SOC (p < 0.01) with Co, Cu, Ti, V, and Zn, and a positive correlation (p < 0.05) with Ni were observed in the mineral horizon. Organic transition metal complexation seems to play an important role in governing SOC in the mineral horizon. That is, the complexation maintains organic carbon stability, slows down its decomposition rate, and accumulates organic carbon. The Ca–SOC positive correlation in the mineral horizon exits because Ca also can complex with organic carbon. Co, Cu, and V–SOC positive correlations (p < 0.05) were also observed, but there were no significant positive correlations (p < 0.01) in the surface horizon because surface SOC had diversified sources. An SOC evolution model influenced by the bedrock was forwarded. Thus, the different soil geochemistry originating from different bedrocks should be noticed when SOC and global carbon cycles are discussed.
Introduction
Soil organic carbon (SOC) constitutes a large pool within the global carbon cycle. Soil is the third largest carbon reservoir on the Earth’s surface, following the ocean and geologic pools (Lal, 2010; Armas-Herrera et al., 2016). Soil organic carbon storage amounts deeply affect carbon dioxide (CO2) emissions to the atmosphere, and its slight change may have a significant effect on the atmospheric CO2 concentration (Schlesinger and Andrews, 2000). Also, SOC is crucial to maintain the soil ecosystem function and quality (Raiesi, 2021). It not only acts as natural nutrition necessary for plant uptake but also improves soil water and fertilization by altering soil’s physical, chemical, and biological properties (Six et al., 2000). Accordingly, the SOC content and its driving factor have been regarded as important processes in determining the soil quality and regulation of the increasing atmospheric CO2 concentration.
The SOC amount is the result of the equilibrium between gains from organic supplies and decomposition (Lal, 2010). A series of factors affecting the equilibrium is widely mentioned when the SOC mechanisms are discussed, such as land use, environmental factors (temperature, moisture, rainfall, etc.), soil physicochemical properties (bulk density, porosity, cation exchange capacity, etc.), and microbial and human activities (Buyannovsky and Wagner, 1983; Amundson and Davidson, 1990; Bajracharya et al., 1990; Zhang et al., 2016). Notably, soil geochemistry is frequently documented to be related to soil organic carbon. Jiang et al. (2014) argued that more SOC accumulates in calcareous soil which is rich in Ca content. Fe-bearing minerals stabilize SOC by Fe-OM compounds, co-precipitation, and absorption (Mikutta et al., 2014; Wang et al., 2017). The positive relationships between SOC and geochemistry (Al, Fe, Ti, Cu, Zn, etc.) were also widely recorded (Aran et al., 2001; Tonneijck et al., 2010). The soils originating from different bedrocks are characterized by different geochemistry, and thus, SOC must be deeply influenced by the underlying rock. However, there is no detailed information about the effect of underlying rocks on SOC, and the potential influencing mechanisms still remain ambiguous.
Universally, the surface soil has been the main focus when the influential factors of SOC were discussed. However, the surface soil experiences a complicated history and has multiple sources of organics. Surface soil organic carbon is profoundly governed by the external environments, such as climate, vegetation, slope, and elevation. Specifically, the human input of SOC amounts due to agricultural and industrial activities occupies a high ratio for the surface soil. So, it is difficult to clarify the effect of the underlying rocks on SOC by merely comparing SOC contents in surface soils. Contrarily, SOC in the mineral horizon is less affected by the external environment and can be tentatively used to reveal the difference of SOC developing from different bedrocks. However, there is no information detailing SOC in the mineral horizon.
On the basis of the aforementioned details, soils in the mineral horizon (near the interface with the bedrock, within 10 cm) on different bedrocks, together with the surface soils (the upper 10 cm) and bedrocks, were gathered, and SOC and geochemistry were analyzed. The aims of the current article were to 1) evaluate the difference of SOC contents overlying different bedrocks, with the emphasis on SOC difference in the mineral horizon, and 2) discuss the possible influencing mechanism of SOC on different bedrocks by correlating SOC with soil geochemistry.
Study Area and Methods
Study Area
The samples were gathered in Zhenxiong County and Weixin County, Yunnan Province, an area at the junction of Yunnan, Guizhou, and Sichuan provinces. The area has typical karst mountains, dominated by mountainous, semi-mountainous, and frigid alpine areas. The area has an elevation of 480–2,416 m. The area belongs to a warm monsoon climate, characterized by cool and rainy weather. The annual temperature varies between 11 and 20°C, and the annual precipitation is in the range of 600–1,200 mm (Yang and He, 1999).
Zhenxiong County and Weixin County have a total area of 5,088.7 km2, including 1,562.4 km2 farmland, 43.2 km2 garden land, 2,373.1 km2 forest land, 38 km2 grass land, 34 km2 water area, 202.5 km2 construction land, and 835.5 km2 unused land. The main vegetation types in this area include coniferous forest, broad-leaved forest, shrub, and grass (Yang and He, 1999).
The strata in this area mainly include the early and later Paleozoic, early Mesozoic, and Quaternary strata. The widely exposed strata from old to new are the Maokou Formation of early-Permian; the Emeishan, Longtan, and Changxin Formations of mid-Permian; and the Feixianguan and Yongningzheng Formations of early-Trias and the Quaternary. The lithology of the Maokou Formation is bioclastic limestone and chert nodule limestone. The host rock of the Emeishan Formation is vesicular amygdaloidal basalt. The lithology of the Longtan Formation mainly includes shale and sandstone interbedded with coal seam. The Changxin Formation consists of limestone interbedded with shale and fine sandstone. The Feixianguan Formation is characterized by siltite, muddy siltstone, calcareous siltstone, and mudstone. The Yongningzheng Formation is dominated by argillaceous limestone, limestone, and sandy shale. Besides, other strata sporadically occur (Figure 1).
Sampling and Analysis
The samples were gathered for the unit of stratum and bedrock. Totally, 10 types of bedrocks in different stratum were involved (Figure 1). These profiles are silty limestone in the Baota Formation of mid-Ordovician (O2b), argillaceous limestone in the Wufeng Formation of late-Ordovician (O3w), limestone in the Qixia Formation of early-Permian (P1q), bioclastic limestone in the Maokou Formation of early-Permian (P1m), basalt in the Emeishan Formation of mid-Permian (P2β), shale in the Longtan Formation of mid-Permian (P2l), limestone interbedded with shale in the Changxin Formation of mid-Permian (P2c), siltite in the Feixianguan Formation of early-Trias (T1f), dolomite in the Guanling Formation of mid-Trias (T2g), and siltite and sandstone in the Xujiahe Formation of late-Trias (T3x). These profiles cover the main lithology and strata in this area.
SOC is governed by multiple factors, and some other factors affecting SOC were tried to be excluded when sample locations were selected. The soil profiles were away from pollution sources such as industry or agriculture. All the samples were from the shrub land because the shrub land is widely distributed in this area. Moreover, a successive relationship between the bedrock and soil was roughly estimated in the field through the grain size, mineral composition, residual texture, slope, and topography. For every bedrock unit, soils in the surface and mineral horizons, and bedrocks were, respectively, sampled (Figure 2); that is, 3 samples for each bedrock unit were taken. The surface soil was taken in the upper 10 cm layer, and the soil was taken near the interface with the bedrock (within 10 cm) in the mineral horizon. The bedrock should be fresh rock. The samples were taken from 3 to 5 locations in the same horizon and mixed into one. The samples were kept in canvas sampling bags.
The samples were sent to the laboratory and air-dried at room temperature. The samples were ground to less than 100 mesh for analysis. SOC was determined by the wet oxidation method with K2Cr2O7 following the study proposed by Lu et al. (1999). The samples were dissolved by HCl–HNO3, and then the analysis of elements was performed by inductively coupled plasma–atomic emission spectroscopy (ICP-AES). For quality control, standard samples and parallel samples were also measured, and the relative errors were less than 5%. The analytical data are provided in Supplementary Table S1.
For correlation analysis, software SPSS 13.0 and Excel 2019 were used. p < 0.01 indicated significant correlation at 0.01 level, and p < 0.05 indicated correlation at 0.05 level.
Results and Discussion
The Soil–Bedrock Geochemical Characteristics and Successive Relationships
The successive relationship and soil origins are key and important to reveal the SOC characteristics on different bedrocks. Geochemistry acts as an effective tool to trace soil origin, and a series of geochemical indexes was discussed. Fe, Al, and Ti are relatively immobile or weakly mobile during weathering (Hill et al., 2000). Al/Ti, Fe/Ti, and Al/Fe remain roughly equivalent between the bedrock and the in situ soil, and thus can be used to ascertain their succession (Young and Nesbitt, 1998).
The ratios of Al/Ti, Fe/Ti, and Al/Fe are listed in Table 1. The ratios for limestone in the Qixia Formation of early-Permian show obvious deviations between the bedrock and the soil, which indicates that the soil does not originate from the underlying bedrock although the residual texture, slope, and topography had been considered in the field. So, this profile was excluded for this research. For other profiles, the ratios of Al/Ti, Fe/Ti, and Al/Fe between the soil and the bedrock are not absolutely equivalent because of the different active coefficients, illuviation, and soil-forming conditions, but they show roughly equivalent values in the mineral horizon. Additionally, the reason for some greater deviations between the surface soil and the bedrock may possibly be because the surface soil is more affected by the external environments (Sharma and Rajamani, 2000). Significantly, positive correlations between these ratios in the bedrock and in the mineral horizon were observed, with R2Al/Ti = 0.82, R2Fe/Ti = 0.69, and R2Al/Fe = 0.61. The ratios of soils in mineral horizons and in surface horizons on the same bedrock were also significantly positively correlated (R2Al/Ti = 0.66, R2Fe/Ti = 0.83, and R2Al/Fe = 0.56).
Many researchers have discussed the soil origin in this studied area or its neighborhood using mineralogy, trace element geochemistry, particle-size characteristics (Sun et al., 2002; Liu et al., 2004), acid-dissolved extraction experiments (Wang et al., 1999), REE (Wang et al., 1999; Sun et al., 2002), grain size of quartz (Feng et al., 2009), and grain size of soil profiles (Feng et al., 2002). Generally, most researchers confirmed that the soil was the accumulation of insoluble residue from the underlying rock (Isphording, 1978; Danin et al., 1982), while only a few considered that the soil originated from other sources such as clastic materials transported by water or air, volcanic ash, and desert dust (Yaalon, 1997; Wang et al., 2019). Our results also support that the soil is the weathering product of the underlying rock, and the soil geochemistry is succeeded from the bedrock.
Soil Organic Carbon and Its Correlations With Soil Geochemistry
The average SOC contents on different bedrocks are summarized in Table 2. The soils in the mineral horizon had SOC contents of 0.19–2.74% (an average of 1.24%), with the highest of 2.74% on basalt in the Emeishan Formation of mid-Permian and the lowest of 0.19% on dolomite in the Guanling Formation of mid-Trias. The SOC for surface soil showed higher contents of 1.26–4.01% (a mean of 2.63%), with the highest of 4.01% on shale in the Longtan Formation of mid-Permian and the lowest on silty limestone on the Baota Formation of mid-Ordovician. The bedrock organic contents only range between 0.01 and 1.63%.
SOC in the mineral horizon on different bedrocks differs greatly, indicating the effect of underlying rocks on SOC in the mineral horizon. SOC in the mineral horizon is not correlated with organic carbon in rocks (Figure 3), implying the SOC contents in the bedrock itself cannot explain the contents in the mineral horizon. There exists a positive correlation between SOC contents in the mineral horizon and in the surface horizon, indicating SOC in the surface and mineral horizons is affected by similar soil geochemistry since they originate from the same bedrock. However, the correlation coefficient (R2 = 0.58) is low (Figure 3), possibly because the external inputs contribute more to the surface soil than to the soil in the mineral horizon.

FIGURE 3. Correlations between SOC in the surface horizon and the mineral horizon (A), mineral horizon and bedrock (B), and surface horizon and bedrock (C).
The correlations between SOC and soil geochemistry in the mineral horizon are shown in Figure 4. SOC in the mineral horizon is significantly positively correlated (p < 0.01) with Co (R2 = 0.674), Cu (R2 = 0.746), Ti (R2 = 0.81), V (R2 = 0.688), and Zn (R2 = 0.722), and positively correlated (p < 0.05) with Ni (R2 = 0.595). The elements of Co, Cu, Ti, V, Zn, and Ni strongly bind to SOC by forming organic metal complexes because they belong to the first transition metal ions (Goodman and Cheshire, 1973; Cheshire et al., 1977; Xing and Zhu, 2003). Moreover, organic metal complexes have been proven to maintain SOC stability and slow down the SOC decomposition rate (Clough and Skjemstad, 2000; Aran et al., 2001; Tonneijck et al., 2010). Therefore, these elements in the mineral horizon seem to play the most important role in governing SOC. Ca is also documented to enhance SOC stability by Ca–SOC complexing actions (Clough and Skjemstad, 2000; Schmeide and Bernhard, 2009; Saito et al., 2010). The positive Ca–SOC correlation (p < 0.05) was also observed in the mineral horizon, with a low correlation coefficient (R2 = 0.457). Additionally, the significantly positive phosphorus–SOC (p < 0.01) correlation (R2 = 0.73) may result from the fact that SOC and phosphorus are both soil nutrient elements.
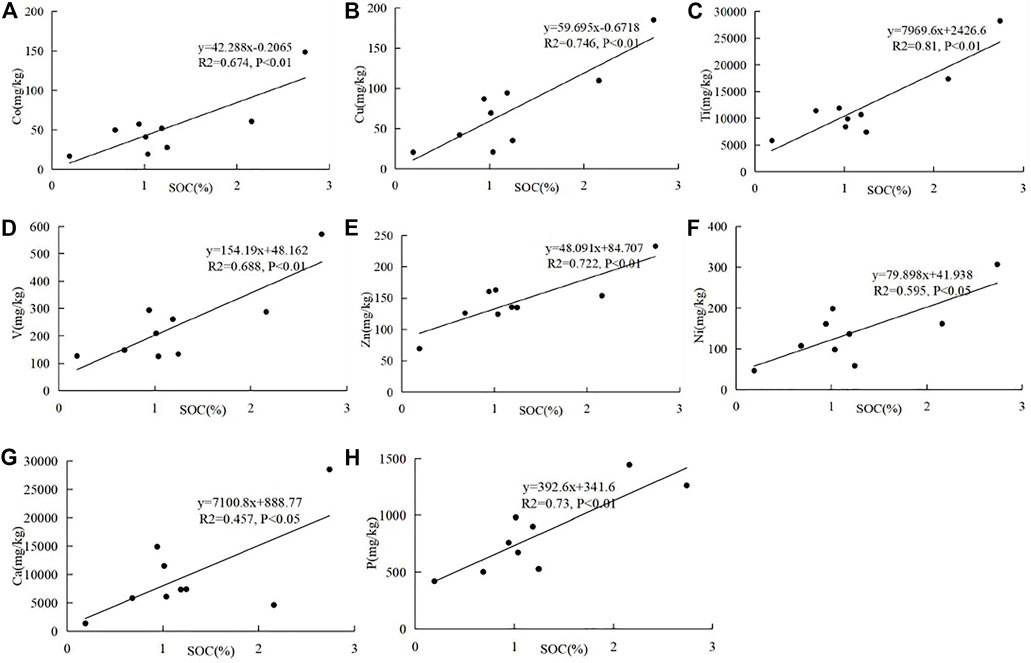
FIGURE 4. Correlations of SOC with Co (A), Cu (B), Ti (C), V (D), Zn (E), Ni (F), Ca (G), and P (H) in the mineral horizon.
The correlations between surface SOC and soil geochemistry are shown in Figure 5. Only Co (R2 = 0.698), Cu (R2 = 0.685), V (R2 = 0.687), and Al (R2 = 0.613) have positive correlations (p < 0.05) with SOC. Compared with the soils in the mineral horizon, SOC is not positively correlated (p < 0.05) with Ti, Zn, and Ni in the surface horizon. Moreover, no significantly positive correlations (p < 0.01) between surface SOC and soil geochemistry were observed. Surface SOC is partly affected by Co, Cu, and V in this study area. Importantly, surface SOC is more affected by the external inputs and external environments, resulting in the difference of its correlations with the first transition metal ions from the SOC in the mineral horizon.
Soil Organic Carbon Evolution Model Influenced by the Bedrock and Its Significance for Global Carbon Cycles
The SOC pool is derived from the by-products of microbial processes and the decomposition of organic matters, and is estimated to be 1,505 Pg to 1 m depth of the soil. The atmosphere only has a carbon pool of 820 Pg (Batjes, 1996). CO2 emission from the soil to the atmosphere is a substantial constituent of greenhouse gas emissions and is a vital part of the carbon cycle. A small emission from the SOC pool may largely affect atmospheric CO2 and aggravate global warming. Also, a small increase in the SOC pool results in a strong drawdown impact on atmospheric CO2. Thus, thoroughly understanding and managing the SOC pool is of a critical importance to limit global warming, and SOC evolution is an important process for the global carbon cycle.
However, the SOC content and its influencing mechanisms on different rocks are difficult to characterize, although a series of factors related to SOC has been widely discussed. Surface soils have wide sources of organic carbon and cannot accurately exemplify the effect of bedrock on SOC. Contrarily, soils in the mineral horizon, which are less affected by the external environments, were focused on in this research.
The variation of SOC content affects the emission amount of CO2 into atmosphere, and carbon fixation in soils is significant for global carbon cycles (Schlesinger and Andrews, 2000; Lal, 2010). The SOC content is the result of the equilibrium between gains from organic supplies and decomposition (Lal, 2010). SOC in the mineral horizon seems to be largely affected by its decomposition since the gained organics are markedly less than those in the surface soil. SOC is rich in oxygen-bearing functional groups such as COOH, OH, and C=O, which can form metal–organic complex. Nitrogen-containing groups such as -NH2 and -N=N- are fewer in SOC, but have a stronger complexing ability with metal ions than oxygen-bearing functional groups (Stevenson, 1991). The metal–organic complexation is also one of the important processes controlling SOC contents. Transition metal ions, especially the first transition metal ions, have priority, high affinity, and strong ligand ability to complex with SOC because of their free electron orbits, preferentially forming stable complexes (Xing and Zhu, 2003).
The mechanisms protecting SOC from decomposition are still the subject of debate (Baldock and Skjemstad, 2000; Tonneijck et al., 2010; Kida and Fujitake, 2020). The metal–organic complex has been proven to maintain SOC stability by restraining against biological decomposition. The formation of metal–organic complexes, which accounted for 40% of the carbon accumulation, was observed to be related to SOC stabilization in Andosols (Tonneijck et al., 2010). Clough and Skjemstad (2000) found organic complexes could not be effectively decomposed even though the soil microbial activity was improved. Complexation with SOC resulted in functional groups becoming more condensed and less susceptible to biological attack (Baldock and Nelson, 2000), and Sollins et al. (2006) stated the average residence time of SOC increased with increasing density. Aran et al. (2001) performed disaggregation tests and incubation experiments, and concluded the complex had strong resistance against disaggregation and biodegradation. Wang et al. (2013) concluded that Fe-o affected by sorption and Fe-p by complexation on SOC preservation, and Fe-p had higher contributions to fix organic carbon than Fe-o. Olatunji and Osibanjo (2014) found the stability of metal–organic carbon complexes depended on the organic carbon type, nature of the complex formed, and the degree of stearic effect. Organo-metallic complexes were widely documented to be responsible for the stabilization of SOM and the high SOC stocks (Blaser et al., 1997; Jiang et al., 2021). Consequently, the SOC decomposition rate is slowed down, and more organic carbon is accumulated in soils with a higher content of transition metal ions.
Also, the relationships between SOC and transition elements have been widely documented. Goodman and Cheshire (1973) added Cu into peaty humic acid and gained the Cu–porphyrin complex compound. Lakato et al (1997) deemed that Cu ligands with heterocyclic nitrogen atoms, not with porphyrin. Senesi et al. (1977) detailed humic acid’s complexation with Fe2+. Goodman and Cheshire (1976) described how VO3− converts into VO2+ when it complexes with SOC. Also, many studies have revealed that SOC is positively correlated with these transition metal ion contents, such as with Cu2+ (Ni et al., 2000; Zhu et al., 2006), Ti2+ (Gao et al., 1994), V2+ (Goodman and Cheshire, 1976), Fe2+ (Wang et al., 2005; He et al., 2006), and Co2+ (Fan et al., 2006).
A series of simulated experiments about the interactions between metal ions and SOC was also recorded. Georg et al. (1994) confirmed that the SOC respiration, as well as SOC solubilization, was reduced by metal–organic complexes. Boudot (1992) concluded that organo-metallic complexes account for the low SOC mineralization rates. The addition of Ca2+ to soil has also been shown to reduce SOC mineralization (Muneer and Oades, 1989). Boudot et al. (1989) observed that the protective effect exerted by metallic ions arose from the formation of metallic hydroxide trapping organic molecules. Cai (2018) found that organic–metal complexes greatly increased under the exogenous addition of Cu and Zn. Zhou (2013) observed the high metal ions decreased the SOC turnover and increased stable organic carbon because of the low mineralization rates.
The rock–soil geochemistry of the first transition metal ions was analyzed to discuss their successive relationship. Ca (Mg) CO3 leaching has been the most important process of soil forming in the carbonate area because carbonate is largely composed of Ca (Mg) CO3, which differs greatly from the non-carbonate area. The trace transition metal ions highly accumulate in soil when Ca (Mg) CO3 leaches. Moreover, Ca (Mg) CO3 leaching is related to the conditions such as pH, Eh, climate, and clay content. Thus, different carbonates have different concentration coefficients of these transition metal ions since they have different Ca (Mg) CO3 contents and Ca (Mg) CO3 leaching abilities. Therefore, no significant correlations of transition metal ions between the bedrock and soil were observed in the carbonate areas. But in the non-carbonate area, Co, Cu, Ti, and V elements have significant positive correlations (p < 0.01), and Zn has positive correlation (p < 0.05) between the bedrock and soil in the mineral horizon (Figure 6). Co, Cu, Ti, and V elements have significant positive correlations (p < 0.01), and Ni has a positive correlation (p < 0.05) between the bedrock and the surface soil (Figure 7). The content of the first transition metal ion in the non-carbonate area largely governs its content in soil.
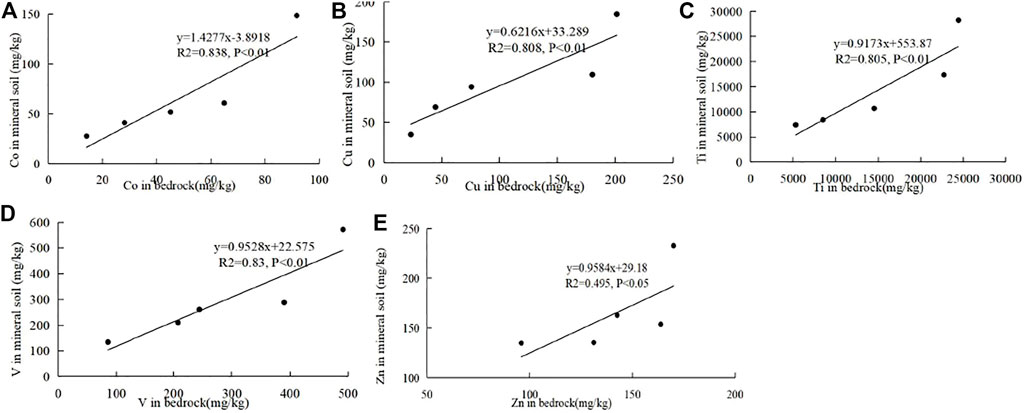
FIGURE 6. Correlations of Co (A), Cu (B), Ti (C), V (D), and Zn (E) between the bedrock and soil in the horizon in the non-carbonate area.
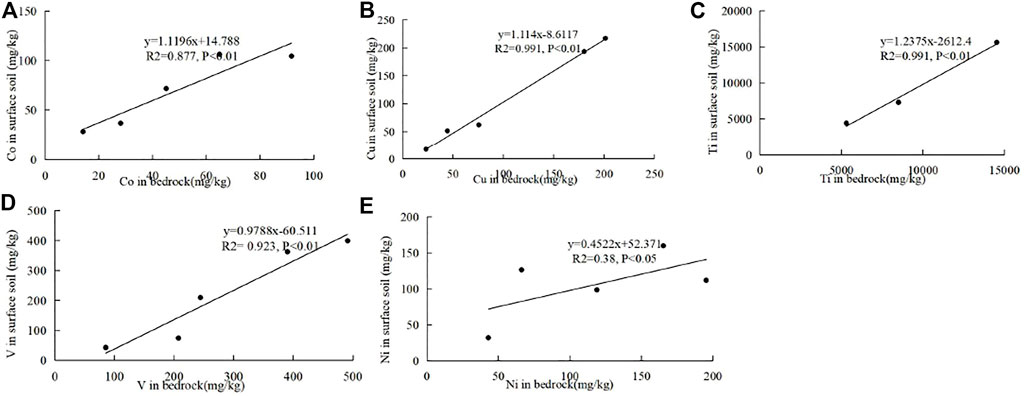
FIGURE 7. Correlations of Co (A), Cu (B), Ti (C), V (D), and Ni (E) between the bedrock and the surface soil in the non-carbonate area.
To summarize, the higher first transition metal ions in the bedrock result in their higher contents in soil during weathering. The SOC stability increases, and its decomposition rate decreases with the increasing of SOC–the first transition metal ion complexes. Consequently, more SOC accumulates in soil with higher first transition metal ions, resulting in higher SOC contents (Figure 8).
Conclusions
The effect of bedrock on SOC was discussed, with emphasis on the soil in the mineral horizon. The followings were gained:
1. The ratios of Al/Ti, Fe/Ti, and Al/Fe show roughly equivalent values between the bedrock and the soil. There exist significant positive correlations between these ratios in the bedrock and in the soil. The soil geochemistry is succeeded from the bedrock in this area.
2. The soils in the mineral horizon have SOC contents of 0.19–2.74%, with an average of 1.24%. SOC for the surface soil shows a higher content range of 1.26–4.01%, with a mean of 2.63%. The bedrock organic content ranges only between 0.01 and 1.63%. The SOC in the mineral horizon on different bedrocks differs greatly. SOC in the surface and mineral horizons are significantly positively correlated because they originate from the same bedrock, implying that the bedrock is an important factor influencing SOC.
3. SOC in the mineral horizon is significantly positively correlated with the first transition metal ions of Co, Cu, Ti, V, and Zn, and positively correlated with Ni. Positive correlations between SOC and Co, Cu, and V in the surface soil also exist. Surface SOC is not positively correlated (P < 0.05) with Ti, Zn, and Ni, and no significant positive correlations (P < 0.01) between SOC and soil geochemistry were observed because surface SOC is more affected by external inputs and external environments. The contents of the first transition metal ions in soil are largely governed by the underlying bedrock.
4. The first transition metal ions can complex with SOC, maintain its stability, slow down the SOC decomposition rate, and result in SOC accumulation. Thus, the first transition metal ion content in soil developing from different bedrocks seems to significantly affect SOC. A SOC evolution model influenced by the bedrock was forwarded, which is significant for the soil quality and global carbon cycle.
Data Availability Statement
The original contributions presented in the study are included in the article/Supplementary Material, further inquiries can be directed to the corresponding author.
Author Contributions
QC and ZH contributed to conceptualization; PZ and SL contributed to data analysis and discussion of the results; QC, PZ, and ZH contributed to writing—original draft preparation; YZ, LH, and LZ contributed to writing—review and editing; BL and XL contributed to drawing. All authors have read and agreed to the published version of the manuscript.
Funding
This work was supported by the Chinese National Key Natural Science Foundation (Grant No. 90202017) and the Natural Science Foundation of Shandong Province (ZR2018MD012).
Conflict of Interest
The authors declare that the research was conducted in the absence of any commercial or financial relationships that could be construed as a potential conflict of interest.
Publisher’s Note
All claims expressed in this article are solely those of the authors and do not necessarily represent those of their affiliated organizations, or those of the publisher, the editors, and the reviewers. Any product that may be evaluated in this article, or claim that may be made by its manufacturer, is not guaranteed or endorsed by the publisher.
Acknowledgments
The authors express the heartfelt thanks to the staff of the Zhaotong Bureau of Sciences and Technology, and the IGSNRR for gathering the samples. The authors also show their appreciation to Shavon Austin for his English correction.
Supplementary Material
The Supplementary Material for this article can be found online at: https://www.frontiersin.org/articles/10.3389/fenvs.2021.784868/full#supplementary-material
References
Amundson, R. G., and Davidson, E. A. (1990). Carbon Dioxide and Nitrogenous Gases in the Soil Atmosphere. J. Geochemical Exploration 38, 13–41. doi:10.1016/0375-6742(90)90091-n
Aran, D., Gury, M., and Jeanroy, E. (2001). Organo-metallic Complexes in an Andosol: a Comparative Study with a Cambisol and Podzol. Geoderma 99, 65–79. doi:10.1016/s0016-7061(00)00064-1
Armas-Herrera, C. M., Mora, J. L., Arbelo, C. D., and Rodriguez-Rodriguez, A. (2016). Factors Affecting CO2 Efflux Rates and the Stability of Soil Organic Carbon Storage in Volcanic Soils of the Canary Islands. Mitig. Adapt. Strat. Gl. 21 (7), 1073–1092. doi:10.1007/s11027-014-9575-2
Bajracharya, R. M., Lal, R., and Kimble, J. M. (2000). Erosion Effects on Carbon Dioxide Concentration and Carbon Flux from an Ohio Alfisol. Soil Sci. Soc. Am. J. 64, 694–700. doi:10.2136/sssaj2000.642694x
Baldock, J. A., and Skjemstad, J. O. (2000). Role of the Soil Matrix and Minerals in Protecting Natural Organic Materials against Biological Attack. Org. Geochem. 31, 697–710. doi:10.1016/s0146-6380(00)00049-8
Batjes, N. H. (1996). Total Carbon and Nitrogen in the Soils of the World. Eur. J. Soil Sci. 47, 151–163. doi:10.1111/j.1365-2389.1996.tb01386.x
Blaser, P., Kernebeek, P., Tebbens, L., Van Breemen, N., and Luster, J. (1997). Cryptopodzolic Soils in Switzerland. Eur. J. Soil Sci. 48, 411–423. doi:10.1111/j.1365-2389.1997.tb00207.x
Boudot, J.-P. (1992). Relative Efficiency of Complexed Aluminum Noncrystalline Al Hydroxide, Allophane and Imogolite in Retarding the Biodegradation of Citric Acid. Geoderma 52, 29–39. doi:10.1016/0016-7061(92)90073-g
Boudot, J. P., Bel Hadj^Brahim, A., Steiman, R., and Seigle-Murandi, F. (1989). Biodegradation of Synthetic Organo-Metallic Complexes of Iron and Aluminium with Selected Metal to Carbon Ratios. Soil Biol. Biochem. 21, 961–966. doi:10.1016/0038-0717(89)90088-6
Buyannovsky, G. A., and Wagner, G. H. (1983). Annual Cycles of Carbon Dioxide Level in Soil Air. Soil Sci. Soc. Am. J. 47, 1139–1145.
Cai, W. C. (2018). Effects of Humic Substances on Heavy Metals Occurrence Mode in Contaminated Soil and the Growth of maize [master’s Thesis]. Kunming, China: Kunming University of Science and Technology.
Cheshire, M. V., Berrow, M. L., Goodman, B. A., and Mundie, C. M. (1977). Metal Distribution and Nature of Some Cu, Mn and V Complexes in Humic and Fulvic Acid Fractions of Soil Organic Matter. Geochimica et Cosmochimica Acta 41, 1131–1138. doi:10.1016/0016-7037(77)90108-9
Clough, A., and Skjemstad, J. O. (2000). Physical and Chemical protection of Soil Organic Carbon in Three Agricultural Soils with Different Contents of Calcium Carbonate. Soil Res. 38 (5), 1005–1016. doi:10.1071/sr99102
Danin, A., Gerson, R., Marton, K., and Garty, J. (1982). Patterns of limestone and Dolomite Weathering by Lichens and Blue-green Algae and Their Palaeoclimatic Significance. Palaeogeogr. Palaeoclimatol. Palaeoecol. 37, 221–233. doi:10.1016/0031-0182(82)90039-6
Fan, W. H., Xi, Y. Z., Yang, L. F., Liu, X. P., Xue, X. G., Wang, R., et al. (2006). Cobalt Contents and its Affecting Factors in Soils of Main Yield Wheat District in Shanxi (In Chinese, English Abstract). J. Soil Water Conservation 20 (3), 40–42. doi:10.1016/S1872-2032(06)60050-4
Feng, Z. G., Wang, S. J., Huang, W., Liu, J., and Liu, X. M. (2009). The Provenance of Weathering Crusts in Karst Terrains, Western Hunan Province: Indication of Grain Size Distribution Characteristics of Quartz (In Chinese, English Abstract). J. Mineral Petrol. 29 (1), 80–85. doi:10.1360/972009-1514
Feng, Z. G., Wang, S. J., Sun, C. X., and Liu, X. M. (2002). Practical index to Distinguish the Origin of Earthy Deposits in Karst Area: Characteristics of Grain Size Distribution. Carsologica Sinica 21 (2), 73–78. doi:10.3969/j.issn.1001-4810.2002.02.001
Gao, B. Z., Zhu, S. T., and Ma, J. J. (1994). Soil Ti Levels and Effects of Fertilizing Ti in Hebei Province (In Chinese, English Abstract). Soil Fertilization 1, 8–12.
Georg, B., Wulff, B. S., and Fahrenkrug, J. (1994). Characterization of the Effects of Retinoic Acid on Vasoactive Intestinal Polypeptide Gene Expression in Neuroblastoma Cells. Endocrinology 135 (4), 1455–1463. doi:10.1210/endo.135.4.7925107
Goodman, B. A., and Cheshire, M. V. (1973). Electron Paramagnetic Resonance Evidence that Copper Is Complexed in Humic Acid by the Nitrogen of Porphyrin Groups. Nat. New Biol. 244, 158–159. doi:10.1038/newbio244158a0
Goodman, B. A., and Cheshire, M. V. (1976). The Occurrence of Copper-Porphyrin Complexes in Soil Humic Acids. J. Soil Sci. 27, 337–347. doi:10.1111/j.1365-2389.1976.tb02005.x
He, R., Zhang, P. F., and Yang, W. (2006). A Study on Humus Composition and Contents of Trace Elements in Soils of Gaoligongshan Natural Reserve (In Chinese, English Abstract). J. West China For. Sci. 35 (2), 48–52.
Hill, I. G., Worden, R. H., and Meighan, I. G. (2000). Geochemical Evolution of a Palaeolaterite: the Interbasaltic Formation, Northern Ireland. Chem. Geology 166, 65–84. doi:10.1016/s0009-2541(99)00179-5
Isphording, W. C. (1978). Mineralogical and Physical Properties of Gulf Coast limestone Soils. Transactions-Gulf Coast Assoc. Geol. Societies 18, 201–214.
Jiang, Z., Lian, Y., and Qin, X. (2014). Rocky Desertification in Southwest China: Impacts, Causes, and Restoration. Earth-Science Rev. 132, 1–12. doi:10.1016/j.earscirev.2014.01.005
Jiang, Z., Liu, Y., Lin, J., Mo, C., Yang, J., and Gunina, A. (2021). Conversion from Double-Rice to Maize-Rice Increases Iron-Bound Organic Carbon by “Iron Gate” and “Enzyme Latch” Mechanisms. Soil Tillage Res. 211, 105014. doi:10.1016/j.still.2021.105014
Kida, M., and Fujitake, N. (2020). Organic Carbon Stabilization Mechanisms in Mangrove Soils: A Review. Forests 11 (9), 981. doi:10.3390/f11090981
Lakatos, B., Tibai, T., and Meisel, J. (1997). ESR Spectra of Humic Acids and Their Metal Complexes. Geoderma 19, 319–338.
Lal, R. (2010). Managing Soils and Ecosystems for Mitigating Anthropogenic Carbon Emissions and Advancing Global Food Security. Bioscience 60, 708–721. doi:10.1525/bio.2010.60.9.8
Liu, X. M., Wang, S. J., Feng, Z. G., and Sun, C. X. (2004). Identification of Origin of limestone Soil: Case Study of Profiles in central and north Guizhou (In Chinese, English Abstract). Soil 36 (1), 30–36. doi:10.13758/j.cnki.tr.2004.01.007
Lu, R. K. (1999). Soil and Agro-Chemical Analytical Methods. Beijing: China Agricultural Science and Technology Press. (In Chinese).
Mikutta, R., Lorenz, D., Guggenberger, G., Haumaier, L., and Freund, A. (2014). Properties and Reactivity of Fe-Organic Matter Associations Formed by Coprecipitation versus Adsorption: Clues from Arsenate Batch Adsorption. Geochimica et Cosmochimica Acta 144, 258–276. doi:10.1016/j.gca.2014.08.026
Muneer, M., and Oades, J. (1989). The Role of Ca-Organic Interactions in Soil Aggregate Stability .II. Field Studies with 14C-Labeled Straw, CaCO3 and CaSO4.2.H2O. Soil Res. 27, 401–409. doi:10.1071/sr9890401
Ni, W., Sun, Z. Q., and Long, X. X. (2000). Copper Situation of Vegetable Garden Soils and its Relation to Soil Organic Carbon (In Chinese, English Abstract). Guangdong Sci. Trace Elem. 7 (3), 53–57.
Olatunji, O. S., and Osibanjo, O. (2014). Investigation of Selected Heavy Metals Level in Top Soils Around Ajaokuta Steel Company, North Central Nigeria. Asian J. Chem. 26 (10), 2982–2986. doi:10.14233/ajchem.2014.16236
Raiesi, F. (2021). The Quantity and Quality of Soil Organic Matter and Humic Substances Following Dry-Farming and Subsequent Restoration in an upland Pasture. Catena 202, 105249. doi:10.1016/j.catena.2021.105249
Saito, T., Sao, H., Ishida, K., Aoyagi, N., Kimura, T., Nagasaki, S., et al. (2010). Application of Parallel Factor Analysis for Time-Resolved Laser Fluorescence Spectroscopy: Implication for Metal Speciation Study. Environ. Sci. Technol. 44, 5055–5060. doi:10.1021/es9036995
Schlesinger, W. H., and Andrews, J. A. (2000). Soil Respiration and the Global Carbon Cycle. Biogeochemistry 48, 7–20. doi:10.1023/a:1006247623877
Schmeide, K., and Bernhard, G. (2009). Redox Stability of Neptunium (V) and Neptunium (Ⅳ) in the Presence of Humic Substances of Varying Functionality. Radiochim. Acta 97, 603–611. doi:10.1524/ract.2009.1661
Senesi, N., Griffith, S. M., Schnitzer, M., and Townsend, M. G. (1977). Binding of Fe3+ by Humic Materials. Geochimica et Cosmochimica Acta 41, 969–976. doi:10.1016/0016-7037(77)90156-9
Sharma, A., and Rajamani, V. (2000). Major Element, REE, and Other Trace Element Behavior in Amphibolite Weathering under Semiarid Conditions in Southern India. J. Geology 108 (4), 487–496. doi:10.1086/314409
Six, J., Paustian, K., Elliott, E. T., and Combrink, C. (2000). Soil Structure and Organic Matter I. Distribution of Aggregate‐Size Classes and Aggregate‐Associated Carbon. Soil Sci. Soc. Am. J. 64, 681–689. doi:10.2136/sssaj2000.642681x
Sollins, P., Swanston, C., Kleber, M., Filley, T., Kramer, M., Crow, S., et al. (2006). Organic C and N Stabilization in a forest Soil: Evidence from Sequential Density Fractionation. Soil Biol. Biochem. 38, 3313–3324. doi:10.1016/j.soilbio.2006.04.014
Stevenson, F. J. (1991). Organic Matter-Micronutrient Reactions in Soil in Micronutrient in Agriculture. 2nd. US: SSSAJ, 145–186.
Sun, C. X., Wang, S. J., and Ji, H. B. (2002). Formation Mechanism of the Superhigh Concentration of REE and the strong Negative Ce Anomalies in the Carbonate Rock Weathering Profiles in Guizhou Province, China (In Chinese, English Abstract). Geochimica 31 (2), 119–128. doi:10.19700/j.0379-1726.2002.02.003
Tonneijck, F. H., Jansen, B., Nierop, K. G. J., Verstraten, J. M., Sevink, J., and De Lange, L. (2010). Towards Understanding of Carbon Stocks and Stabilization in Volcanic Ash Soils in Natural Andean Ecosystems of Northern Ecuador. Eur. J. Soil Sci. 61, 392–405. doi:10.1111/j.1365-2389.2010.01241.x
Wang, C., Li, F., Shi, H., Jin, Z., Sun, X., Zhang, F., et al. (2013). The Significant Role of Inorganic Matters in Preservation and Stability of Soil Organic Carbon in the Baoji and Luochuan Loess/Paleosol Profiles, Central China. Catena 109, 186–194. doi:10.1016/j.catena.2013.04.001
Wang, Q. S., Wei, Q., and Liu, B. F. (2005). Mechanism of Humic and Fulvic Acids Adsorption by Fe2O3, MnO2 or Al2O3 Solid Sorbents (In Chinese, English Abstract). Acta Pedologica Sinica 42 (4), 600–608. doi:10.11766/trxb200408160410
Wang, S., Ji, H., Ouyang, Z., Zhou, D., Zhen, L., and Li, T. (1999). Preliminary Study on Weathering and Pedogenesis of Carbonate Rock. Sci. China Ser. D-earth Sci. 42 (6), 572–581. doi:10.1007/bf02877784
Wang, Y., Wang, H., He, J. S., and Feng, X. (2017). Iron-mediated Soil Carbon Response to Water-Table Decline in an alpine Wetland. Nat. Commun. 8 (1), 15972–15974. doi:10.1038/ncomms15972
Wang, Z. Y., Lin, Q., and Zhao, Y. J. (2019). Problems Related to Weathering and Pedogenesis of Carbonate Rock in Karst Area (In Chinese, English Abstract). J. Guangxi Teach. Education Univ. (Natural Sci. Edition) 36 (1), 94–99.
Xing, G. X., and Zhu, J. G. (2003). Soil Trace Element and Rear Earth Element. (In Chinese) (Beijing: Science Press), 281–287.
Yaalon, D. H. (1997). Soils in the Mediterranean Region: what Makes Them Different? Catena 28 (3), 157–169. doi:10.1016/s0341-8162(96)00035-5
Yang, Z. S., and He, M. (1999). Study on the Strategy of Exploitation and Utilization of Land Resources in the Northeast Mountain Region of Yunnan ProvinceⅡ: The Strategical Guiding Principles, Objective, Scheme and Measures (In Chinese, English Abstract). J. Mountain Sci. 17 (sup), 75–83.
Young, G. M., and Nesbitt, H. W. (1998). Processes Controlling the Distribution of Ti and Al in Weathering Profiles, Siliciclastic Sediments and Sedimentary Rocks. J. Sediment. Res. 68, 449–455. doi:10.2110/jsr.68.448
Zhang, H. Y., Gao, Z. J., Li, W., Shi, M. J., and Mou, K. L. (2016). Analysis of Different Types of Rock-Soil in Soaking experiment (In Chinese, English Abstract). J. Shandong Univ. Sci. Technology (Natural Science) 35 (6), 10–16. doi:10.16452/j.cnki.sdkjzk.2016.06.002
Zhou, T. (2013). Effects of Metal Pollution on Soil Organic Carbon Mineralization, Straw Decomposition and Greenhouse Gases Emission in Paddy Soils (In Chinese). Nanjing: Nanjing Agricultural University.
Keywords: SOC, soil in the mineral horizon, bedrock, organic-transition metal complexes, global carbon cycle
Citation: Chen Q, Zhang P, Hu Z, Li S, Zhang Y, Hu L, Zhou L, Lin B and Li X (2022) Soil Organic Carbon and Geochemical Characteristics on Different Rocks and Their Significance for Carbon Cycles. Front. Environ. Sci. 9:784868. doi: 10.3389/fenvs.2021.784868
Received: 29 September 2021; Accepted: 07 December 2021;
Published: 07 January 2022.
Edited by:
Lu-Jun Li, Northeast Institute of Geography and Agroecology (CAS), ChinaReviewed by:
Adriana Horbe, University of Brasilia, BrazilJeffrey M Dick, Central South University, China
Copyright © 2022 Chen, Zhang, Hu, Li, Zhang, Hu, Zhou, Lin and Li. This is an open-access article distributed under the terms of the Creative Commons Attribution License (CC BY). The use, distribution or reproduction in other forums is permitted, provided the original author(s) and the copyright owner(s) are credited and that the original publication in this journal is cited, in accordance with accepted academic practice. No use, distribution or reproduction is permitted which does not comply with these terms.
*Correspondence: Ziyuan Hu, hzy0618@163.com