- College of Chemical Engineering, Sichuan University of Science and Engineering, Zigong, China
Methylene blue (MB) is a common pollutant in wastewater of printing and dyeing industry. At present, ozone oxidation is commonly used in the treatment of printing and dyeing wastewater. Ozone is used for oxidation reaction directly and indirectly. However, the reaction rate is not fast enough. In this study, the attapulgite (ATP) was modified by tetrabutyl titanate-ethanol solution. The TiO2/ATP nanocomposite with high catalytic activity was prepared. The synthesized samples were subjected to characterized by X-ray diffraction (XRD) and Fourier Transform infrared spectroscopy (FTIR). The morphology and particle size of the particles were observed by scanning electron microscopy (SEM). The TiO2/ATP nanocomposite was calcined at 300°C for 2 h, and the degradation rate of 80 mg/L methylene blue was 99.8%. The experimental results show that the ozone, temperature and mass transfer factors are excluded, and the effects of temperature, MB concentration and pH on the degradation of methylene blue are investigated. The optimal reaction conditions are as follows: The ozone concentration is 0.067 g/min; the ozone flow rate is 0.15 NL/min; the stirrer rotation speed is 550 r/min; the catalyst is 0.1 g; the temperature is 50°C; Based on the results, the reaction mechanism was derived and the kinetic study of the experiment was carried out.
Introduction
At present, the discharge of dye industry wastewater has reached 2 billion tons per year (Xue 2021). Considered as environmental pollutant, there are over 30,000 kinds of synthetic dyes used worldwide. Among them, the wide application of methylene blue in the printing and dyeing industries has resulted in the alarming pollution of industrial wastewater containing methylene blue to other pure water bodies (Zhang et al., 2008).
The current approaches of wastewater treatment containing organic dye include biological method, coagulation method, oxidation method, adsorption method and membrane separation method. The most commonly used methods for treating dyeing wastewater at home and abroad are biochemical method, flocculation precipitation method and catalytic oxidation with activated as catalyst where later two approaches have been the common practice (Song et al., 2003; Li, 2007; Chen et al., 2009). The activated carbon is expensive and the regeneration process is complicated, which limits its wide application.
Because of its unique crystal structure, large specific surface area, high chemical and mechanical stability, attapulgite is suitable for catalyst carrier. Especially for the low price, attapulgite has practical significance in wastewater treatment. Meanwhile the slag can be reused, where the photocatalyst can be prepared with TiO2. Studies showed that activated attapulgite is resistant to organophosphorus pesticides such as trichlorfon and dichlorvos (Peng et al., 2006; Zhang et al., 2013; Yang et al., 2014). In the wastewater treatment process, the transitional metallic,as a catalytic, can speed up the reaction, and improve the reaction efficiency. The results of previous experiments showed that the homogenous catalysis of Zn, Ti, Mn, Ni. Sometimes, the same element can be not only used as a catalyst in the solution, but also in the solid phase accelerator as an active component (Yin et al., 2003). TiO2 has been widely studied for the degradation of various organic compounds because it is nontoxic, highly efficient, stable, environmentally friendly, and inexpensive (Virkutyte and Varma, 2012; Yin et al., 2013; Fang et al., 2014; Yu et al., 2019; Qiao et al., 2021). So, TiO2 was used as the active component of multiphase catalyst in this paper. Oxygen atoms in ozone have strong electron-philicity or proton-philicity, so they show strong oxidizability. Ozone molecules decompose into highly active oxygen atoms, and part of ozone decompose into hydroxyl radical •OH with strong oxidizing effect in water. Strong oxidation of oxygen atoms and •OH in water can sterilize, destroy the organic structure, degrade the organic matter, make the organic matter mineralization (Masschelein, 1992). The process has the characteristics of non-toxic, low secondary pollution, low selectivity, quick action time and high efficiency. ATP is a very good catalyst carrier, where TiO2 was loaded on as the active component can promote the conversion of ozone oxide into hydroxyl radical which can accelerates the decomposition of MB.
In this paper, TiO2/attapulgite nanocomposite were successfully prepared. The catalytic process, with ozone-assisted oxidation was investigated, including the effects of temperature, MB concentration and pH on the degradation of methylene blue. Moreover the property and microstructure of the catalyst were measured. And the degradation mechanism of MB was derived and the reaction kinetic was proposed.
Experimental
Experimental Set-Up
The experimental set-up used in the experiment are a 4 flask with 250 ml volume, 101-1AB electric blast drying oven (Beijing Zhongxing Weiye Century Instrument Co., Ltd.) for drying sample, a constant temperature magnetic stirrer (Shanghai Lichen Bangxi Instrument Technology Co., Ltd.) for reaction, HY-010 type ozone generator (Chengdu Yifeng Hongyuan Environmental Protection Technology Co., Ltd.), I3 type UV-visible spectrophotometer (Jinan Haineng Instrument Co., Ltd.) for analysis sample concentration and OTL1200 type tube furnace for modifying catalyst with high temperature. The experiment set-up is shown in Figure 1:
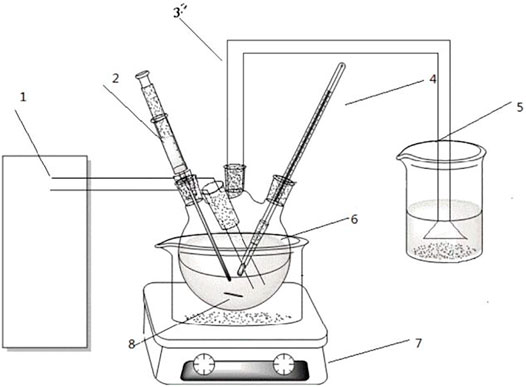
FIGURE 1. Diagram of experimental devices. 1-Ozone generator, 2-colloidal sampler, 3-thermometer, 4-exhaust pipe, 5-tail gas recovery cup, 6-four-necked flask, 7-magnetic stirring heater, 8-stirring rotor.
Experimental Reagents
The chemical reagents used in the experiment are methylene blue C16H18CIN3S·3H2O, attapulgite (ATP) Mg5Si8O20(OH)2(OH2)4·3H2O, tetrabutyl titanate (C16H36O4Ti), anhydrous ethanol (C2H5OH), hydrochloric acid (AR), ammonia (AR), sodium diethyldithio carbamate (AR), hydroxylamine hydro-chloride (AR), ammonium citrate (AR), ethylenediamine (AR), trichlorome -thane (AR).
Preparation of ATP/TiO2
About 40 g of attapulgite with a particle size of about 180 mesh was screened and immersed in 200 ml of 4 mol/L dilute hydrochloric acid for 8 h, and then filtered by suction to neutrality and dried in an oven at a temperature of 80°C for 4 h. A tetrabutyl titanate-ethanol impregnation solution with a volume ratio of 1:4 was placed for use. The water absorption of 31.8 g acid-modified attapulgite was measured to be 24.5 ml, and it was dried in an oven for 3 h. Subsequently, 24.5 ml of tetrabutyl titanate-ethanol impregnation solution was added to 31.8 g of attapulgite, sealed with a plastic wrap, and immersed for 13.5 h. The impregnated attapulgite was hydrolyzed in a water bath for 1 h, hydrolyzed to Ti(OH)4, dried in an oven and oxidized to TiO2, and dried for 3 h. The attapulgite was placed in a tube furnace and calcined at 500°C for 2 h, and sealed in a bag.
Characterization
The X-ray diffractometer (XRD) was used for the physical phase and purity analysis of all samples on the DX-2600 instrument with Cu Kα as the radiation source with an operating voltage of 30 kV and a current of 10 mA. The morphology of the particles was observed by transmission electron microscopy (SEM), and the working voltage was 15 kV. The compostion and functional groups of all catalysts were qualitatively analyzed via infrared spectroscopy (FTIR) with spectral scanning range: 4000-4000 cm−1.
Catalytic Oxidation of Methylene Blue Wastewater
An analytical balance was used to weight 0.1 g of modified attapulgite from section 2.3 for later use. A certain amount of methylene blue stock solution was removed from the 1000 mg/L methylene blue mother liquor to prepare a methylene blue reaction solution of the desired concentration. The prepared MB solution was poured into a dry four-necked flask, with a magnetic stirrer; the four-necked flask containing the solution was placed in a water bath where the MB solution below the water. The speed and temperature of the magnetic stirrer were set; the bottom of the meter and the bottom of the trachea were at the same level as the stirrer; when the reaction temperature of the MB solution reached the specified temperature, it would pass through the four ports. After the modified attapulgite catalyst was added into the flask, the ozone generator switch was turned on and the timer was started. The sampler was used to take 5 ml of sample liquid through the sampling port every time. The residue was filtered through the disposable filter head and then put into the sample tube for testing. The temperature was controlled during the reaction so that the temperature difference was lower than ±1°C. After the reaction was completed, the solution with a constant volume was measured by an ultraviolet spectrophotometer at its maximum wavelength of 665 nm. The degradation rate is calculated according to the following formula:
Where D is the degradation rate (%), and A and A0 are the absorbance before and after degradation, respectively.
Results and Discussion
SEM of the Sample
The SEM is employed to study the morphology of the attapulgite/TiO2 composite materials. The SEM images ot three composite samples, calcined at 300°C, 400°C, and 500°C for 2 hours were shown in Figure 2. One can see that the attapulgite has a loose structure as well as a large surface area and specific surface area, so it has a good adsorption effect. The TiO2 nanoparticles with a particle size of less than 10 nm are more uniformly distributed on the surface of the attapulgite clay, and the morphology and size of the TiO2 and the attapulgite clay change little after loading. Nevertheless, the TiO2 nanoparticles are not fully attached to the surface of the attapulgite clay. With a small amount of TiO2, the crystal form has anatase transformed into rutile type, and a small amount of spherical-like particles appear on the surface. A small portion is dispersed around the TiO2/attapulgite powder, which indirectly increases the specific surface area and surface area. When the temperatures are 300°C and 400°C, the structure changes little, but the crystal structure of TiO2 on the surface will change. At 500°C, the catalyst has a loose structure. Although the surface area has increased, the pore structure of the crystal will collapse, thereby reducing the specific surface area.
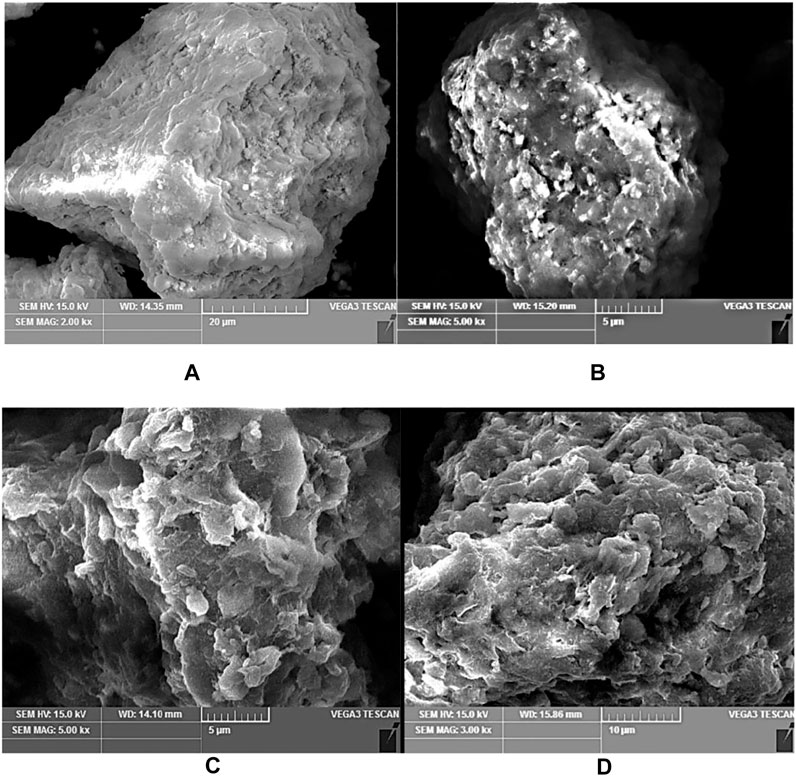
FIGURE 2. (A) SEM image of Original soil, (B) Calcination at 300°C, (C) Calcination at 400°C, (D) Calcination at 500°C.
As shown in Figure 2A, the surface of the original attapulgite without heating has a layered structure with a smooth surface, which has a large surface area and specific surface area.
Figure 2B is the SEM of the modified attapulgite that was calcined at 300°C, one can see that the surface of the heated attapulgite becomes porous, and the internal structure changes. The small white spheres on the surface are TiO2 particles which increase the surface area and the adsorption efficiency.
Figure 2C shows the SEM of attapulgite calcined at 400°C,whchi showed a more fluffy porous structure with a dense layers, the shapes of TiO2 particles become irregular than those in Figure 2B.
When the attapulgite is calcined at 500°C, its surface becomes fluffier and more porous as shown in Figure 2D. Meanwhile the pores collapse, the surface is sintered, and the growth of TiO2 crystals is hindered.
XRD Characterization of the Sample
In order to further investigate the coating condition of the sample, the X-ray diffraction spectrum of sample was collected. The range is 5–90°. The XRD results were analyzed by Jade software to obtain the phase analysis and the results. ATP/TiO2 calcined at 300, 400, and 500°C are shown in Figure 3.
It can be seen from that the XRD patterns of four ATP samples have a strong diffraction peak where the 2θ is at 8.495°. For the ATP/TiO2 sample with a calcination temperature of 300°C, a strong diffraction characteristic peak of TiO2 appears when 2θ is 25.339 and 30.807, respectively. For the ATP/TiO2 sample calcined at 400°C, a strong diffraction characteristic peak of TiO2 appears when 2θ is 27.446, 36.085, and 54.322, respectively. At the calcination temperature of 500°C, a strong diffraction characteristic peak of TiO2 show at 25.27 and 48.049. The higher the temperature, the more TiO2 is loaded in the ATP. In the four graphs, the diffraction peak of ATP decreases with the rising temperature. This is ascribed to the reduction of the ATP diffraction peak intensity caused by the hydroxyl group on the attapulgite clay’s surface as well as the separation of adsorbed water, crystal water and structural water. When the temperature is too high, the internal channel collapses. Other peaks in the graph are caused by the presence of impurities in the sample itself. The overall crystal lattice of the catalyst is intact and not destroyed. The product has obvious diffraction peaks, and the interlayer spacing fluctuates. The diffraction peaks remain orderly after calcination, and the crystal lattice is intact.
In order to further investigate the coating condition of the sample, the FTIR of sample was collected.
Figure 4 was the FTIR patterns of ATP and ATP/TiO2. The absorption peaks at 470 and 1032cm−1 corresponded to the stretching vibration of the Si-O-Si bond in amorphous SiO2. The absorption band of modified attapulgite near 700cm−1 is produced by asymmetric stretching vibration of Ti-O. The absorption peak at 1639cm−1 is produced by N-H. The absorption peak in the range of 3000 to 3800cm−1 is generated by the surface hydroxyl group of SiO2. The absorption peaks of ATP/TiO2 were weakened because other chemical bonds were formed after the modification.
Catalytic Oxidation of Methylene Blue by ATP/TiO2
Effect of Ozone
In 150 ml MB solution, the experimental condition as follows: the concentration is 60 mg/L, the flow rate of ozone is 0.15 NL/min, the concentration of ozone is 0.067 g/L, pH is 5.5, the amount of attapulgite is 0.1 g, the stirring speed is 55 r/min, and the reaction temperature is 50°C. The reaction results are as shown in the figure below:
It can be seen from Figure 5, when the same modified attapulgite is added, the ozone ratio is different, and the degradation rate of methylene blue increases to some extent. In the absence of ozone, the degradation rate can reach 90% or so. After the introduction of ozone, the rate can reach 98%. This indicates that the catalyst has a certain adsorption capacity, but the degradation rate can be further improved under the indirect oxidation of ozone. The addition of catalyst significantly improves the efficiency, indicating that the catalyst plays a critical role in the degradation of methylene blue.
The effect of ozone concentration was also considered, and the results are as shown in Figure 6:
It can be seen from Figure 6 that the degradation rate of MB decreases with the increasing ozone flow rate. When the ozone concentration is above 0.067 g/L, the degradation rate of MB decreases with the increase of ozone concentration. Nevertheless, when the ozone concentration is below 0.067 g/L, the degradation rate of MB decreases as the ozone concentration declines. Thus, it can be concluded that the optimal ozone concentration is 0.067 g/L.
The reason is that during the reaction, the ozone content in the solution is affected by the dissolution, reaction and decomposition of ozone (Chen et al., 2015; Zhang et al., 2015). Within a certain ozone flow range, as the ozone flow rate increases, the solubility of ozone in water increases, and the yield of •OH also increases, resulting a faster degradation of MB. For a certain volume of reaction system, the solubility of ozone in the solution is constant. After he solution is saturated with ozone, the ozone in the reaction system is excessive, in which case, the impact of the amount of ozone on the reaction is negligible. When the ozone flow exceeds a certain value, excessive ozone will participate in the quenching reaction of OH (see Equation 1), which reduces the amount of •OH involved in the reaction and thus impacts the degradation of MB.
The gas-liquid mass transfer factor is eliminated, so that the reaction system is controlled by reaction and non-mass transfer control. In this experiment, in order to make the degradation rate of MB as high as possible, the ozone flow rate was chosen to be 6 L/min. If applied to industrial production, the economic efficiency of unit ozone removal MB should be evaluated to determine the optimal ozone flow.
Effect of Reaction Temperature
The experimental condition as follows: At a rotational speed of 550 r/min, the ozone flux is 0.15 NL/min, the ozone concentration is 0.067 g/L, and the pH is 5.5. The amount of attapulgite is 0.1 g, the initial concentration of 150 ml of MB solution is 60 mg/L. The reaction was carried out under the conditions of 20°C, 30°C, 40°C, 50°C, and 60°C, respectively. The change curve of MB degradation rate over time is shown in Figure 7.
It can be seen from Figure 7 when the reaction temperature is lower than 50°C, the degradation rate of MB gradually increases with the rising reaction temperature; when the reaction temperature is 50°C, the change in reaction temperature has little effect on the degradation of MB. Therefore, with the increase of reaction temperature, the degradation rate of MB first increases and then remains unchanged According to the study by Masschelein (1992), ozone is quite poor in stability and is easy to decompose at even room temperature (see Eq. 2). The half-life of ozone decomposition in water is related to temperature. When the temperature is below 50°C, the decomposition rate will increase accordingly as it rises, and more •OH will be produced in the solution, and increasing temperature will enhance the activation in the reaction system (Li, 2007). The ratio of molecules increases the probability of effective collision and accelerates the degradation rate. Nevertheless, when the temperature rises to a certain value, the solubility of ozone in water will be greatly reduced. Because •OH can be decomposed by ozone in water, the degradation rate is reduced accordingly.
The optimum reaction temperature is 50°C, at which neither the solubility of ozone in water is not too low nor the degradation rate is not too slow, thus the reaction rate can be maintained at a suitable temperature. Furthermore, this is also the temperature that is easier to control, so the temperature of subsequent experiments is set to 50°C as well.
Effect of MB Solution Concentration
The experimental condition as follows: the reaction temperature is 50°C, the rotation speed is 550 r/min, the ozone flux is 0.15 L/min, the ozone concentration is 0.067 g/L, the attapulgite dosage is 0.1 g, pH is 5.5, the initial concentration of 150 ml MB solution is 40 mg/L. The experiment was performed under the conditions of 50 mg/L, 60 mg/L, 70 mg/L and 80 mg/L. The variation curve of MB degradation rate over time is shown in Figure 8.
The gas-liquid-solid heterogeneous reaction is a complex process. As can be seen from Figure 8, under the above optimal experimental conditions, the initial concentration of MB was changed to observe the degradation of MB. The results showed that the degradation rate is faster as the initial concentration of MB is lower. Because of the heterogeneous catalysis, the reactants are adsorbed before the catalytic reaction, and adsorbed in the catalyst pores for catalytic oxidation. Due to the limited the adsorption sites, the higher the MB concentration, the lower the adsorption efficiency. In addition, because the molecular weight of the adsorbed reactants is also limited, the degradation rate declines as the increasing concentration.
Effect of pH
The experimental condition as follows: the reaction temperature is 50°C, the rotational speed is 550 r/min, an ozone flux is 0.15 L/min, the ozone concentration is 0.067 g/L, attapulgite dosage is 0.1 g, an initial concentration of 150 ml of MB solution IS 80 mg/L. The change curve of MB degradation rate over time is shown in Figure 9.
The results showed that the decolorization rate of MB increases along with the pH. Under acidic conditions, the decolorization rate is lower than that under alkaline conditions. By analyzing the principle of ozone oxidation, it is found that the oxidation intensity of ozone enhances with the rising pH. The above experimental phenomenon indicates that the hydroxyl radical generated by ozone decomposition by itself is the main driving force for MB degradation. Under acidic conditions, the direct reaction between ozone and MB molecules is much slower. Therefore, the degradation process of MB can be expressed by a continuous quasi-first-order equation.
Effect of Catalyst Calcination Temperature
The tetrabutyl titanate-ethanol impregnation liquid has a volume ratio of 1:4. After intermediate treatment, it is calcined by a nitrogen atmosphere in a tube furnace, and calcined at 300°C, 400°C, and 500°C, respectively. The effect of calcination temperature on the degradation rate of 80 mg/L MB solution was investigated. The experimental results are as shown in Figure 10.
When the calcination temperature is too high, the catalyst pores collapse and the specific surface area of adsorption is reduced, so the degradation rate will be slowed down, whereas, the temperature increases TiO2 and ATP load better, so the final degradation rate will tend to a close value.
Recycle and Re-Use
Collect the used composite catalyst, wash it several times with deionized water, dry it and grind it. Obtain the relationship between the number of times the composite catalyst is used and the degradation rate, as shown in Figure 11:
From Figure 11, it can be seen that the recovered attapulgite/TiO2 composite photocatalyst still exhibits high catalytic activity, and the degradation effect can reach 89% even if it is used for the fourth time. This shows that attapulgite as a carrier can give full play to its characteristics of strong adsorption and high thermal stability. The composite catalyst solves the problem of secondary pollution while processing methylene blue, and enhances the utilization rate of titanium dioxide.
Ozone Reaction Kinetics
Ozone enters the aqueous solution through aeration, in which the gas-liquid contact mass transfer is involved, and the gas is diffused in the water. According to classical dual-mode theory (Achari et al., 2021; Wu et al., 2021), ozone oxidation of organic matter may exist in two forms: the form of ozone gas diffuses into the liquid, reacts with organic matter at the gas-liquid interface or liquid film before entering the liquid phase, and the selectivity of ozone for such substances Strong results; another portion of unreacted ozone reacts with the organic matter through the liquid membrane into the liquid phase.
Where [O3] and [D] are the concentrations of ozone molecules and organic matter, respectively, and the ozone concentration and OH are deemed to be constant values. In the unbuffered MB solution, a quasi-stable condition for hydroxyl radicals is assumed. The concentration of hydroxyl radicals can be estimated by Benitez and Beltran from Eq. 5:
In the formula, [HO·] and [HOO·] are the concentrations of hydroxyl radicals and hydroxide ions, respectively. [O3] is the dissolved ozone concentration, [D] is the concentration of the MB solution, and kHO· is the second-order rate constant of the [·OH] reaction path. kHOO· is a kinetic constant for the decomposition of ozone to form a free radical HOO· under high pH conditions. At higher pH, the ozone molecules decompose more hydroxyl radicals, resulting in a large amount of hydroxide ions. Substituting [OH] in Eq. 5 into Eq. 6, yields Eq. 7:
Since the concentration of O3 is excessive, the ozone oxidation process of MB molecules is an apparent quasi-first-order kinetic reaction. Therefore, the formulas kO3·[O3] and 3k·OOH·[O3] are assumed to be constant.
Under acidic conditions, the MB solution in deionized water has a PH of 5.5. In weakly acidic solution, because the MB solution is a non-buffered solution, [OH−] is 0 in the solution. The kinetic formula is the apparent first-order kinetics:
With the standard curve of MB solution, the molar concentration at time t at T = 50°C, CMB = 80 mg/L, and pH = 5.5 is obtained. Because ozone is continuously and excessively introduced during the reaction, k·[O3] in the formula can be used as a constant. MATLAB software is used to theoretically model the formula. The kinetic equation is:
The following results are obtained by fitting the experimental data, as shown in Figure 12.
As shown in the figure, the residuals of the experiments are evenly distributed on the upper and lower sides of the zero line, indicating a good fitting degree.
The k values with different temperatures were obtained as shown in Table 1.
According to Arrhenius formula.
Ea was obtain by plotting lnk 1/T.
From Figure 13, the slope is −5610.59, so the Ea is 46.646 kJ/mol.The results show that when the ozone is excessive, the ozone concentration is constant, and the degradation kinetics of the MB solution is the apparent first-order kinetics. Under acidic conditions, MB degradation is carried out by direct ozone oxidation, whereas under alkaline conditions, MB degradation is carried out by indirect ozone oxidation. In terms of the relative degradation rate, the higher the pH, the faster the degradation rate. For the final degradation rate, the weak acidity, neutrality and alkalinity can reach 99% degradation rate. This indicates that the above kinetic equation is suitable for most reactions, except for strongly acidic reactions. The degradation rate of MB in this process is 99.8% greater than 73.8% obtained by some reaserchers (Yin et al., 2003) under the optimum condition.
Conclusion
In this work, the experimental scheme is to impregnate the attapulgite with a volume of tetrabutyl titanate-ethanol solution. After drying, the tetrabutyl titanate was hydrolyzed to TiO2 under the action of water vapor, and calcined at a low temperature of 300°C to obtain a uniform distribution of surface anatase TiO2. The TiO2 attapulgite nanocomposite was loaded, and the material had high catalytic activity.
The attapulgite modified with TiO2 was calcined at 300°C for 2 h, and the degradation rate of 80 mg/L methylene blue was 99.8%. The experimental results show that the ozone, temperature and mass transfer factors are excluded, so the effects of temperature, MB concentration and pH on the degradation of methylene blue are investigated. The optimal reaction conditions are as follows: The ozone concentration is 0.067 g/min; the ozone flow rate is 0.15 NL/min; the stirrer rotation speed is 550 r/min; catalyst is 0.1 g; the temperature is 50°C.
The decolorization process of MB is quasi-first-order kinetics, and the kinetic equation is obtained with Ea 46.646 kJ/mol.
Data Availability Statement
The original contributions presented in the study are included in the article/supplementary material, further inquiries can be directed to the corresponding author.
Author Contributions
JS contributed to the conception of the study, experiment, data analyses and wrote the manuscript; YJ performed the experiment; XQ contributed significantly to analysis and manuscript preparation; BZ performed the data analyses; XL helped perform the analysis with constructive discussions.
Conflict of Interest
The authors declare that the research was conducted in the absence of any commercial or financial relationships that could be construed as a potential conflict of interest.
Publisher’s Note
All claims expressed in this article are solely those of the authors and do not necessarily represent those of their affiliated organizations, or those of the publisher, the editors and the reviewers. Any product that may be evaluated in this article, or claim that may be made by its manufacturer, is not guaranteed or endorsed by the publisher.
Acknowledgments
The authors gratefully acknowledge the Program of Key Laboratories of Fine Chemicals and Surfactants in Sichuan Provincial Universities (No. 2020JXY03), the Scientific Research Fund of Sichuan Provincial Education Department (No. 17ZA0273), and the Talent Introduction Fund of Sichuan University of Science and Engineering (No. 2015RC53).
References
Achari, V. S., Lopez, R. M., Rajalakshmi, A. S., Jayasree, S., Shibin, O. M., John, D., et al. (2021). Microporous Carbon with Highly Dispersed Nano-Lanthanum Oxide (La2O3) for Enhanced Adsorption of Methylene Blue. Separat. Purif. Tech. 279, 119626. doi:10.1016/j.seppur.2021.119626
Chen, R., Zhang, G., and Yi, C. (2015). ExperimentaI Study on the Degradation of oiIfieId Wastewater Containing poIymer by Microwave Enhanced Ozone Oxidation. Ind. Water Treat. 3, 90–92.
Chen, Z., Guo, T., Li, X., Chen, F., Lu, X., and Zhang, Y. (2009). Catalytic Oxidation Treatment of Methylene Blue Dye Wastewater by Attapulgite-Loaded CeO2. Kinetic Energ. Mater. 10 (40), 122–137. doi:10.1016/j.catcom.2008.12.055
Fang, Y., Li, Z., Yang, B., Xu, S., Hu, X., Liu, Q., et al. (2014). Effect of Dye Structure on Optical Properties and Photocatalytic Behaviors of Squaraine-Sensitized TiO2 Nanocomposites. J. Phys. Chem. C 118, 16113–16125. doi:10.1021/jp502208y
Fei Yin, Z., Wu, L., Gui Yang, H., and Hua Su, Y. (2013). Recent Progress in Biomedical Applications of Titanium Dioxide. Phys. Chem. Chem. Phys. 15, 4844–4858. doi:10.1039/c3cp43938k
Li, X. (2007). Application of Attapulgite Clay in Wastewater Treatment. J. Gansu United Univ. 21 (3), 12–18. doi:10.3969/j.issn.1672-691X.2007.03.015
Peng, S., Xie, J., Qing, C., Chen, T., Xu, H., Chen, J., et al. (2006). Treatment of Acid Fuchsin Dye Wastewater by TiO2 Attapulgite Photocatalytic Oxidation Process. J. Chin. Ceram. Soc. 34 (10), 55–57. doi:10.3321/j.issn:0454-5648.2006.10.010
Qiao, X. X., Liu, X. J., Zhang, W. Y., Cai, Y. L., Zhong, Z., Li, Y. F., et al. (2021). Superior Photo-Fenton Activity towards Chlortetracycline Degradation over Novel G-C3n4 Nanosheets/schwertmannite Nanocomposites with Accelerated Fe(III)/Fe(II) Cycling. Separat. Purif. Tech. 279, 119760. doi:10.1016/j.seppur.2021.119760
Song, R., Yang, L., and Sheng, J. (2003). Surface Modification and Characterization of Nano Attapulgite. Bull. Silicate 22 (3), 36–39. doi:10.3969/j.issn.1001-1625.2003.03.009
Virkutyte, J., and Varma, R. S. (2012). Visible Light Activity of Ag-Loaded and Guanidine Nitrate-Doped Nano-TiO2: Degradation of Dichlorophenol and Antibacterial Properties. RSC Adv. 2, 1533–1539. doi:10.1039/c1ra00990g
Wu, H., Liu, Y., Chen, B., Yang, F., Wang, L., Kong, Q., et al. (2021). Enhanced Adsorption of Molybdenum(VI) from Aquatic Solutions by Chitosan-Coated Zirconium-Iron Sulfide compositeEnhanced Adsorption of Molybdenum(VI) from Aquatic Solutions by Chitosan-Coated Zirconium–Iron Sulfide Composite. Separat. Purif. Tech. 279, 119736. doi:10.1016/j.seppur.2021.119736
Xue, G. (2021). TechnoIogy Progress of Dyeing Wastewater Treatment. Ind. Water Treat. 41 (9), 10–17. doi:10.19965/j.cnki.iwt.2021-0433
Yang, J., Wang, G., Guan, Z., Zhang, N., Tong, Y., and Li, J. (2014). Preparation and Characterization of Nano-TiO2 Loaded with Attapulgite Clay and its Adsorption of Ni(II). New Chem. Mater. 7, 24–29.
Yin, L., Lu, X., and Ai, F. (2003). Effect of Ti-Attapulgite Catalyst on Ozonation Degradation of Dye Wastewater. J. Chin. Ceram. Soc. 31 (1), 66–69. doi:10.3321/j.issn:0454-5648.2003.01.014
Yu, C., He, H., Fan, Q., Xie, W., Liu, Z., and Ji, H. (2019). Novel B-Doped BiOCl Nanosheets with Exposed (001) Facets and Photocatalytic Mechanism of Enhanced Degradation Efficiency for Organic Pollutants. Sci. Total Environ. 694, 133727. doi:10.1016/j.scitotenv.2019.133727
Zhang, D., Xu, B., and Zhu, P. (2013). Study on the Mechanism of Photocatalytic Degradation of Methylene Blue by TiO2. J. East China Normal University(Natural Science) (9), 35–42. doi:10.3969/j.issn.1000-5641.2013.05.005
Zhang, L., Liu, H., and Zhang, B. (2015). Mechanism of Enhancement Ozonation by Ultrasound for Degradation of Polyacrylamide in ASP Produced. CIESC Jorunal 6, 2242–2247. doi:10.11949/j.issn.0438-1157.20150085
Keywords: titanium dioxide, attapulgite, methylene blue, catalytic ozonation, kinetics
Citation: Shang J, Jiang Y, Qin X, Zhao B and Li X (2021) Catalytic Oxidation of Methylene Blue by Attapulgite/TiO2. Front. Environ. Sci. 9:783313. doi: 10.3389/fenvs.2021.783313
Received: 26 September 2021; Accepted: 28 October 2021;
Published: 15 November 2021.
Edited by:
Shihai Deng, Xi’an Jiaotong University, ChinaCopyright © 2021 Shang, Jiang, Qin, Zhao and Li. This is an open-access article distributed under the terms of the Creative Commons Attribution License (CC BY). The use, distribution or reproduction in other forums is permitted, provided the original author(s) and the copyright owner(s) are credited and that the original publication in this journal is cited, in accordance with accepted academic practice. No use, distribution or reproduction is permitted which does not comply with these terms.
*Correspondence: Jianping Shang, welsons@126.com