- 1School of Resources, Environmental and Chemical Engineering and Key Laboratory of Poyang Lake Environment and Resource Utilization of Ministry of Education, Nanchang University, Nanchang, China
- 2School of Life Science, Nanchang University, Nanchang, China
- 3Institute of Hydrobiology, Chinese Academy of Science, Wuhan, China
- 4Jiangxi Academy of Eco-Environmental Sciences and Planning, Nanchang, China
- 5College of Fisheries, Henan Normal University, Xinxiang, China
A large body of evidence suggests that the physical and chemical characteristics of the sediment in lakes that have undergone eutrophication have been significantly altered. However, the effects of alterations in sediments on submersed macrophytes remain unknown. In this study, we present the results of an outdoor experiment that examined how the growth and anchorage of the widespread submersed macrophyte Myriophyllum spicatum L. responded to the enrichment of organic matter in the sediments and whether water depth affects these responses. We found that low levels of enrichment with organic matter (≤7%) enhanced the growth of M. spicatum. In contrast, high levels of enrichment with organic matter (from 12 to 18%) slightly inhibited its growth. Although the anchorage force of M. spicatum slightly decreased with an increase in the content of organic matter in the sediment, it was much higher than the hydraulic drag force on plants at a relatively high current velocity, indicating that the plants were unlikely to be uprooted in these sediments. The water depth did not alter the responses of growth and anchorage of M. spicatum to enrichment with organic matter. Our results suggest that M. spicatum could be a potential species to restore eutrophic lakes, since it can grow well and anchor stably in sediments with relatively high organic matter and manage low light stress.
Introduction
Submersed macrophytes are highly important for stabilizing the function and structure of freshwater ecosystems, particularly in shallow lakes (Hilt et al., 2017; Lurig et al., 2021). A high coverage and diversity of submersed macrophytes can provide positive feedback for the provision of clear water and enhance the biodiversity of shallow lakes (Scheffer et al., 1993; Law et al., 2019). Recent evidence points toward a progressive loss of submersed macrophytes during the process of lake eutrophication (Sand-Jensen et al., 2000; Zhang et al., 2017). This deterioration has primarily been attributed to an increase in the attenuation of light by phytoplankton and epiphytes following nutrient enrichment in the water column (Phillips et al., 2016). A large body of evidence suggests that the physical and chemical characteristics of the sediment had also been substantially altered as the lakes eutrophied (Van Der Molen et al., 1998; Sand-Jensen et al., 2005; Hobaek et al., 2012), which may have a strong influence on the growth and community structure of submersed macrophytes (Barko et al., 1991; Raun et al., 2010; Bornette and Puijalon, 2011; Xie et al., 2013; Li et al., 2015). However, the effects of alteration of sediments on submersed macrophytes are still largely unknown.
One significant change in sediment with eutrophication is that the content of organic matter increased as a result of the turnover of the thriving macrophytes and sedimentation of organic particles from the production of phytoplankton (Sand-Jensen and Møller, 2014). Previous studies found that submersed macrophytes benefit from the enrichment of organic matter at low levels in the sediment, since the decomposition of organic matter can increase the concentration of nutrients in pore water (Silveira and Thomaz, 2015). However, at high levels, submersed macrophytes may suffer anaerobic and phytotoxic effects induced by organic matter (Wu et al., 2009; Pulido et al., 2011). In addition, an increase in the organic matter in sediment can reduce the strength of submersed macrophytes to anchor, and thus enhance their risk of uprooting (Sand-Jensen and Møller, 2014).
The depth of water has a profound impact on the growth of submersed macrophytes and their allocation and morphology (Fu et al., 2012; He et al., 2019). Previous studies found that water depth can alter the responses of submersed macrophytes to other stressors (Xiao et al., 2007; Xu et al., 2016). For example, Xu et al. (2016) reported that the water depth, substrate type, and wave exposure had extremely significant joint influences on the growth and morphology of Vallisneria natans. The negative influence of high water levels on submersed macrophytes may be aggravated by decreased belowground growth under high water levels, exposure to high waves, and high nutrient conditions (Xu et al., 2016). However, whether the water depth affects the responses of the growth and anchorage of submersed macrophytes to sediment enriched in organic matter remains unclear.
In this study, we aimed to investigate how the submersed macrophyte growth and anchorage responded to the enrichment of organic matter in the sediment and whether water depth affects these responses. To answer these questions, we conducted an outdoor experiment in which we evaluated the response of the widespread submerged macrophyte Myriophyllum spicatum L. to the individual and combined effects of water depth and enrichment of organic matter in the sediment. We hypothesized the following: 1) Low levels of enrichment in organic matter in the sediment would enhance the growth of the plant, while high levels of organic matter would inhibit the plant growth. 2) The anchorage force would decrease with an increase in the content of organic matter in the sediment. 3) The negative effects of high content of organic matter on the plant would be stronger in deep water than shallow ones, since the plant may allocate more resources to the elongation of shoots at deeper sites.
Materials and Methods
Plant Species
M. spicatum is a rooted submersed aquatic plant that forms canopies. It has spread from its native Eurasia to become an invasive plant worldwide (Aiken et al., 1979). This species occurs at a wide range of water and sediment conditions (Sondergaard et al., 2010; Su et al., 2019), and the plant strongly morphologically and physiologically responds to changing environmental conditions, such as variations in temperature, light, nutrients, and dissolved inorganic carbon availability (Strand and Weisner, 2001; Wang et al., 2008; Hussner and Jahns, 2015; Hussner and Heidbuchel, 2021).
Experiment Establishment
A two-by-five factorial design experiment was conducted with two levels of water depth and five levels of the addition of organic matter to the sediment. Each treatment included seven replicates. The experiment was performed using a concrete pond (length 6 m × width 4 m × depth 1.6 m) located outdoors at the Biological Gardens of Nanchang University (Nanchang, China) (28°39′ N, 115°48′ E) and filled with tap water. No further water was added after the beginning of the experiment (except for precipitation). Sediment was collected from Poyang Lake, air-dried, and mixed. The contents of total nitrogen (TN), total phosphorus (TP), and organic matter in the sediment were 0.54 ± 0.02 g kg−1g, 0.25 ± 0.01 g kg−1, and 0.81 ± 0.01% dry weight (dw), respectively. A gradient of 4, 8, 12, and 16% dry weight treatments of organic matter were produced by adding commercial peat soil (Compo, Germany) to the lake sediment. Lake sediment without the addition of organic matter was used as control. Three sediment samples were taken randomly from each gradient to analyze the actual initial organic matter and contents of TN and TP. Three healthy and clean apices of M. spicatum (15 cm in length) were planted in each pot (diameter = 15 cm, height = 10 cm) filled with 8 cm of sediment. Ten plant apices of similar sizes were randomly selected to determine their initial dry weight. Half of the pots were placed at 0.8 m water depth on a steel frame submersed in the pond water. The other pots were placed on the bottom of the pond (without sediment). The pots just covered 5% area of the pond, and the volume of the sediment was only 0.25% of the water; thus, the dissolved organic matter release from the sediment would not strongly influence the plant performance.
Monitoring
Three sites were established to monitor the water parameters in the pond. Two of the sites were next to the middle of the long sides, while one was beside the middle of the short side. The concentration of phytoplankton chlorophyll a (chl-a) and water temperature in the pond were measured weekly using a BBE algae analyzer (AlgeaTorch, Moldaenke, Germany). Dissolved oxygen, pH, and conductivity were measured weekly at 10 cm below the water surface using a multiparameter water quality instrument (HI9829, Hanna, Italy). Photosynthetically active radiation (PAR) was measured weekly at intervals of 0.5 m water depth from the subsurface to 1 m of the water column using a Li-COR UWQ-9525 sensor coupled with a Li-1500 data logger (LI-COR, Lincoln, NE, United States). The attenuation coefficient of light (K) in the water column was calculated based on the PAR at different depths as described by Duarte et al. (1986). Water samples were collected from the pond biweekly to analyze the levels of TN, TP, ammonium nitrogen (NH4+-N), and nitrate nitrogen (NO3−-N). Samples filtered through Whatman GF/C glass fiber filters were used to determine the amount of NH4+-N using Nessler’s reagent colorimetric method and NO3−-N using ultraviolet spectrophotometric methods (State Environmental Protection Administration of China, 2002). TP and TN were analyzed from unfiltered water using the ammonium molybdate spectrophotometric method after digestion with K2S2O8 solution and the ultraviolet spectrophotometric method after digestion with K2S2O8 and the addition of HCl (State Environmental Protection Administration of China, 2002), respectively.
Plant Harvest and Anchorage Force Measurement
The experiment ended when some of the plants at 0.8 m deep reached the water surface. The duration of the experiment was from August 25, 2019, to October 9, 2019, a period of 45 days. The plant height and anchorage force were measured immediately when the pots were removed from the pond. The anchorage force was measured by wrapping a cotton rope around the stem just above the sediment, connecting it via a metal string to a digital force gauge and gradually increasing the pull until the plant was dislodged (Sand-Jensen and Møller, 2014). The maximum pull registered by digital force gauge was registered as the anchorage strength. A few measurements were discarded where the plant broke. The plant shoots and roots of each pot were collected and then dried at 60°C for 48 h for dry weight measurements. The plant relative growth rate (RGR) was calculated as follows:
RGR = ln(Wt/W0)/t,
where W0 is the initial dry weight of M. spicatum (g), Wt is the dry weight (including shoots and roots) of M. spicatum at the end of the experiment (g), and t is the duration of experiment (days).
Calculation of Hydraulic Forces
To evaluate whether the plant will be uprooted in lakes, we calculated the hydraulic force (F) on M. spicatum as described by Schutten and Davy (2000):
F = A×biomass×velocity1.5, where A is a species-specific factor that incorporates the roughness that arises from shoot geometry and surface characteristic. In this study, we used the species-specific factor A = 2564 for M. spicatum delineated by Schutten and Davy (2000) and the velocity = 0.6 m s−1 as described by Schutten et al. (2005). This value of velocity was relatively high compared with that in lakes under normal conditions (Schutten et al., 2005; Qin et al., 2007), but it may occur in extreme conditions, such as during a storm (Schutten et al., 2005).
Sediment and Plant Sample Analyses
The contents of TN, TP, and organic carbon (OC) of the sediment taken at the beginning of the experiment were analyzed using standard methods (Shi, 1994), i.e., the K2Cr2O7–H2SO4 oxidation method for OC, the Kjeldahl acid-digestion method for TN, and the molybdenum blue colorimetric method for TP. The total organic matter of the sediment was determined by multiplying OC with Van Bemmelen’s factor of 1.724. At the end of the experiment, sediment samples were taken from each pot to analyze the water content and dry density analysis as described by Barko and Smart (1986). The contents of C, N, and P of M. spicatum shoots were analyzed after being ground into powder as described above.
Data Analyses
The relationship between TN, TP, water content along with the density, and the content of organic matter in the sediment was examined using linear regression analyses to assess the effects of addition of organic matter on the characteristics of the sediment. The effects of water depth, enrichment in organic matter, and their interaction on the sediment and plant parameters were tested using two-way analyses of variance (ANOVAs). GAMs (generalized additive models) were used to fit the relationship between the content of organic matter and plant parameters at 0.8 and 1.6 m, respectively. GAMs were also used to fit the relationship between shoot N, shoot P, and the plant RGR. The GAMs were constructed with the function “gam” in package “mgcv” using penalized regression splines as the smoothing function, Gaussian error distribution, and automatic calculation of smoothing parameters. All the analyses were performed in R (R Core Team, 2020). The results were considered to be significant at p < 0.05.
Results
Water Characteristics in the Pond
The water conditions were suitable for the growth of submersed macrophytes. There were low concentrations of water nutrients, resulting in a low abundance of phytoplankton and high transparency in the pond (Table 1). The warm temperatures were beneficial to plant growth (Table 1). In addition, warm temperatures can maintain high levels of decomposition of the organic matter in the sediment, which is crucial to properly evaluate the addition of organic matter on the growth of submersed macrophytes.
Effects of the Addition of Organic Matter on the Sediment Characteristics
Both the TN and TP of the sediment increased linearly as the content of organic matter increased (Figures 1A,B; R2 = 0.992, p < 0.001 for N and R2 = 0.973, p = 0.001 for P). The addition of organic matter had strong effects on the water content of the sediment (Table 2, p < 0.001) and sediment density (Table 2, p < 0.001). With the addition of organic matter, the mean water content of sediment increased from 36 to 71% (Table 3, Figure 1C), whereas the mean sediment density decreased from 0.99 g cm−3 to 0.30 g cm−3 (Table 3, Figure 1D).
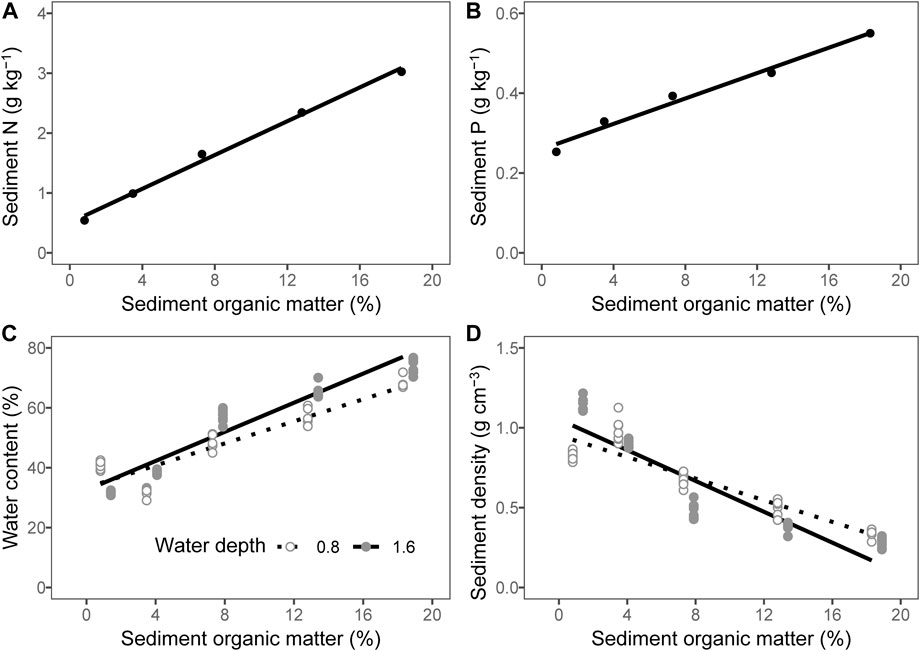
FIGURE 1. Relationship between the sediment organic matter and (A) sediment total nitrogen (N), (B) sediment total phosphorous (P), (C) sediment water content, and (D) sediment density. The lines are predicted by linear models. The points of 1.6 m water depth were moved 0.6% to the right to avoid overlapping.
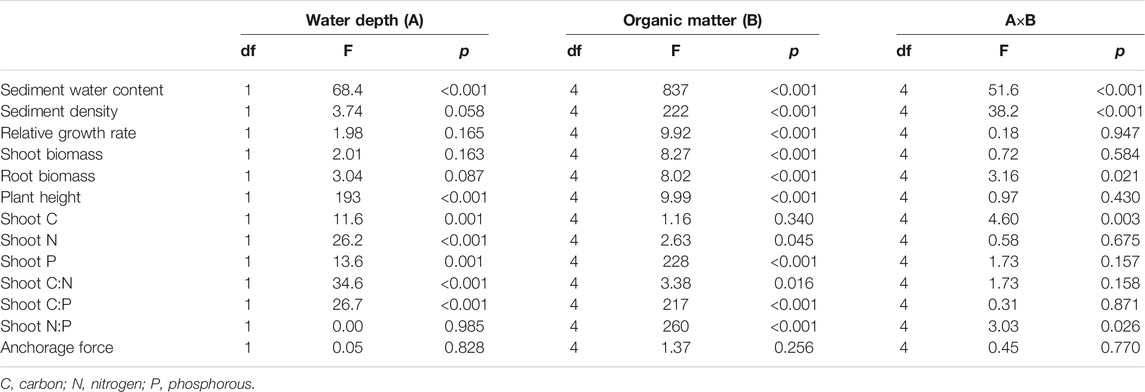
TABLE 2. Results of two-way ANOVAs for water depth and the addition of organic matter on sediment and plant parameters.
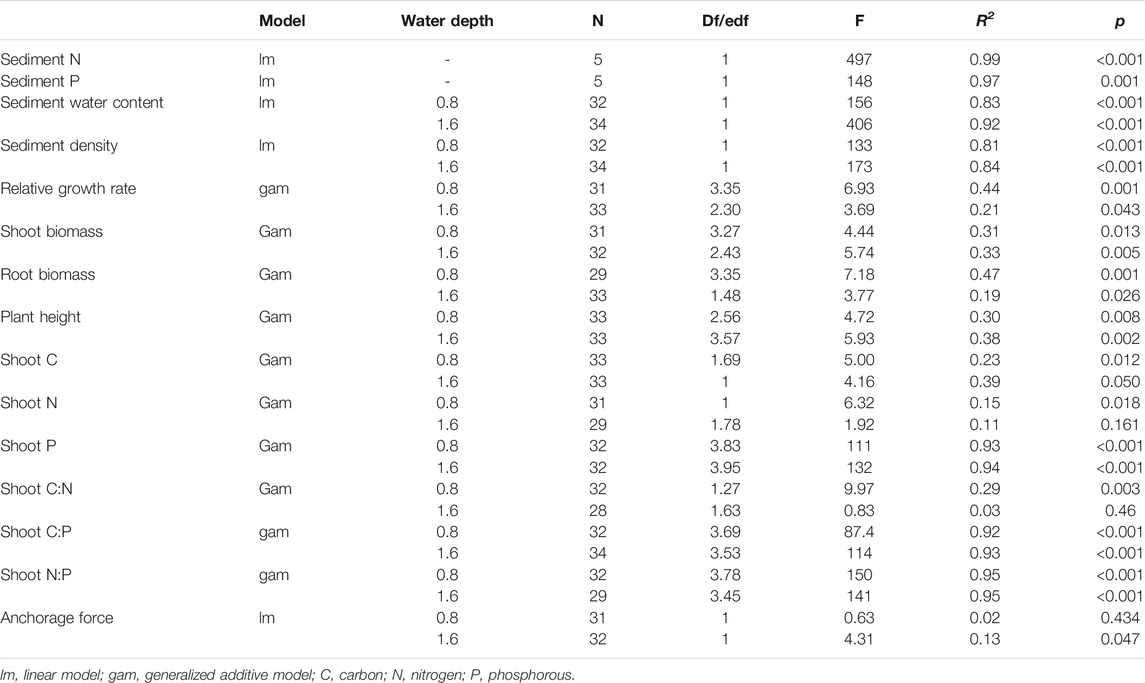
TABLE 3. Relationship between the organic matter in sediments and plant traits and sediment parameters based on linear or generalized additive models.
Effects of Water Depth and the Addition of Organic Matter on Plant Growth
There was no interactive effect of water depth and organic matter addition on the plant growth parameters with the exception of the root biomass (Table 2; p = 0.947 for the relative growth rate, p = 0.584 for shoot biomass, p = 0.430 for plant height and p = 0.021 for root biomass). The water depth had no effect on the plant RGR (Table 2; p = 0.165) and shoot biomass (Table 2; p = 0.163), whereas the effect on plant height was significantly positive with much taller plants at a depth of 1.6 m compared with those at a depth of 0.8 m (Table 2, Figure 2D; p < 0.001). The plant RGR increased in parallel with the content of organic matter of the sediment from 0.81 to 7.29% and tended to slightly decrease when the content of organic matter in the sediment was higher at both water depths (Table 3, Figure 2A; p = 0.001 for 0.8 m and p = 0.043 for 1.6 m). The plant shoot biomass responded similarly to the content of organic matter in the sediment with that of plant RGR (Table 3, Figure 2B; p = 0.013 for 0.8 m and p = 0.015 for 1.6 m). The plant height increased in parallel with that of organic matter in the sediment at the lower end of the gradient at both water depths (Figure 2D, p = 0.008 for 0.8 m and p = 0.002 for 1.6 m). At higher levels of sediment organic matter, the plant height was constant at a water depth of 1.6 m but tended to decrease at a water depth of 0.8 m (Figure 2D, p = 0.008 for 0.8 m and p = 0.002 for 1.6 m).
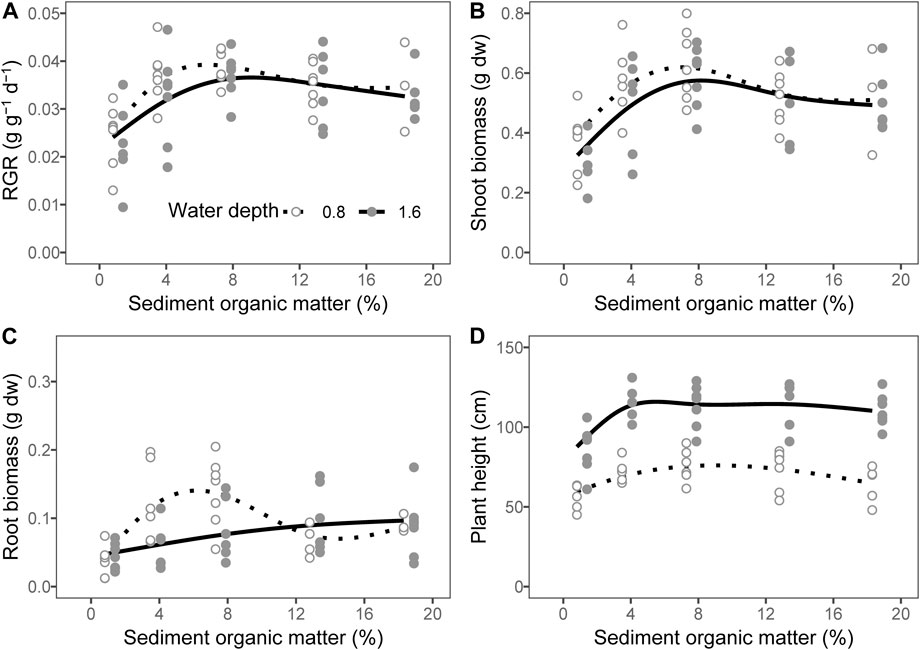
FIGURE 2. Relationship between sediment organic matter and (A) plant relative growth rate (RGR), (B) shoot biomass, (C) root biomass, and (D) plant height. The lines are predicted by generalized additive models. The points of 1.6 m water depth were moved 0.6% to the right to avoid overlapping dw, dry weight.
Effects of Water Depth and the Addition of Organic Matter on the Elemental Content of Plant Shoots
There was no interactive effect between the water depth and the addition of organic matter on the elemental content of the plant shoots with the exception of the shoot C and shoot N:P ratio (Table 2; p = 0.675 for shoot N, p = 0.157 for shoot P, p = 0.158 for shoot C:N, p = 0.871 for shoot C:P, p = 0.003 for shoot C, and p = 0.026 for shoot N:P). The contents of shoot N and P of the plant shoots were slightly higher at a water depth of 1.6 m than that at 0.8 m (Table 2, Figure 3 B, C; p < 0.001 for shoot N and p = 0.001 for shoot P). However, the shoot C, shoot C:N ratio, and shoot C:P ratio were lower at a water depth of 1.6 m than that at 0.8 m (Table 2, Figures 3A,D,E; p = 0.001 for shoot C, p < 0.001 for shoot C:N, and p < 0.001 for shoot C:P). There was no significant effect of water depth on the shoot N:P ratio (Table 2, p = 0.985). The content of shoot C increased in parallel with an increase in the content of organic matter at a water depth of 0.8 m, whereas it showed an inverse trend at a water depth of 1.6 m (Table 3, Figure 3A; p = 0.012 for 0.8 m and p = 0.050 for 1.6 m). The shoot N content decreased with an increase in the sediment organic content at a water depth of 0.8 m, while it remained unchanged at a water depth of 1.6 m (Table 3, Figure 3B; p = 0.018 for 0.8 m and p = 0.161 for 1.6 m). The content of shoot P rapidly increased in parallel with that in the organic matter in the sediment up to 7.29% but remained unchanged at greater values of organic matter at both water depths (Table 3, Figure 3C; p < 0.001 for 0.8 m and p < 0.001 for 1.6 m). The shoot C:N ratio increased as the sediment organic content increased at a water depth of 0.8 m, while it remained constant at a water depth of 1.6 m (Table 3, Figure 3D; p = 0.003 for 0.8 m and p = 0.460 for 1.6 m). The shoot C:P and N:P ratios decreased with an increase in the organic matter in the sediment from 0.81 to 7.29%, but they remained unchanged at greater values of organic content at both water depths (Table 3, Figures 3E,F, p < 0.001).
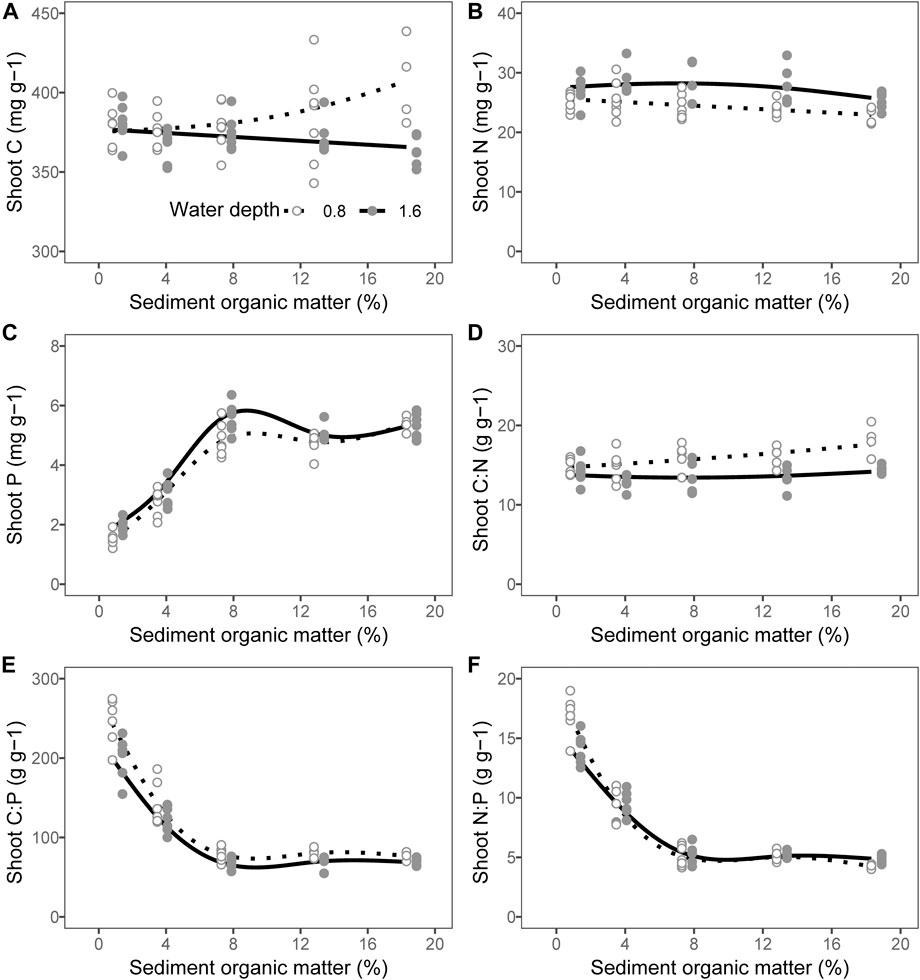
FIGURE 3. Relationship between sediment organic matter and (A) shoot carbon (C), (B) shoot nitrogen (N), (C) shoot phosphorous (P), (D) shoot C:N ratio, (E) shoot C:P ratio, and (F) shoot N:P ratio. The lines are predicted by generalized additive models. The points of 1.6 m water depth were moved 0.6% to the right to avoid overlapping.
Relationship between the Shoot N, Shoot P, and Plant Relative Growth Rate
The plant RGR decreased as the content of shoot N at a water depth of 0.8 m, whereas there was no measurable significant relationship between the plant RGR and the content of shoot N at a water depth of 1.6 m (Table 4, Figure 4A; p = 0.027 for 0.8 m and p = 0.237 for 1.6 m). The plant RGR increased when the content of shoot P was as high as 4 mg g−1, while it remained unchanged with a further increase in the content of P (Table 4, Figure 4B; p = 0.007 for 0.8 m and p = 0.011 for 1.6 m).

TABLE 4. Relationship between shoot nitrogen (N), phosphorous (P), and plant relative growth rate based on generalized additive models.
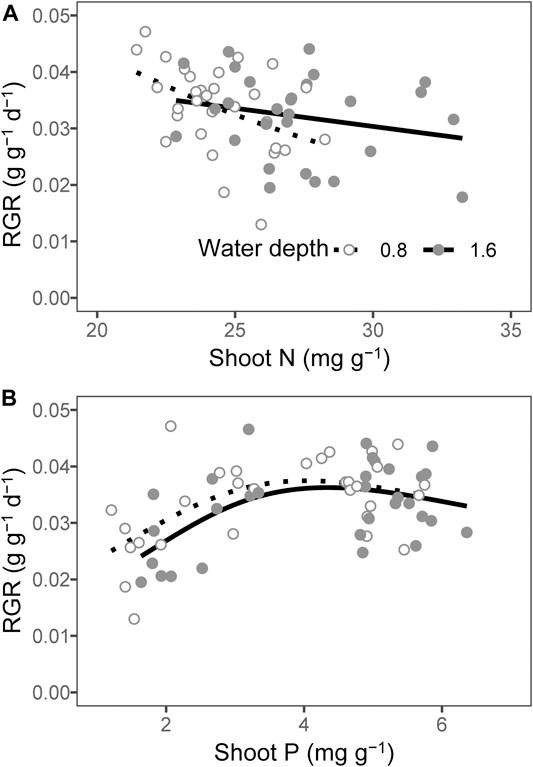
FIGURE 4. Relationship between (A) shoot nitrogen (N), (B) shoot phosphorous (P), and the plant relative growth rate (RGR). The lines were predicted by generalized additive models.
Effects of Water Depth and the Addition of Organic Matter on the Plant Anchorage Force
Neither the water depth nor the addition of organic matter had an effect on the plant anchorage force based on the two-way ANOVA test (Table 2, p = 0.828 for water depth and p = 0.256 for organic matter addition), while the results of GAMs suggested that the plant anchorage force decreased with the content of organic matter in the sediment at a water depth of 1.6 m (Table 3, Figure 5A; p = 0.047). The plant anchorage force was larger than the hydraulic forces in all the treatments. This indicated that there was no risk of M. spicatum becoming uprooted in these sediments when the current velocity was below 0.6 m s−1 (Figure 5B).
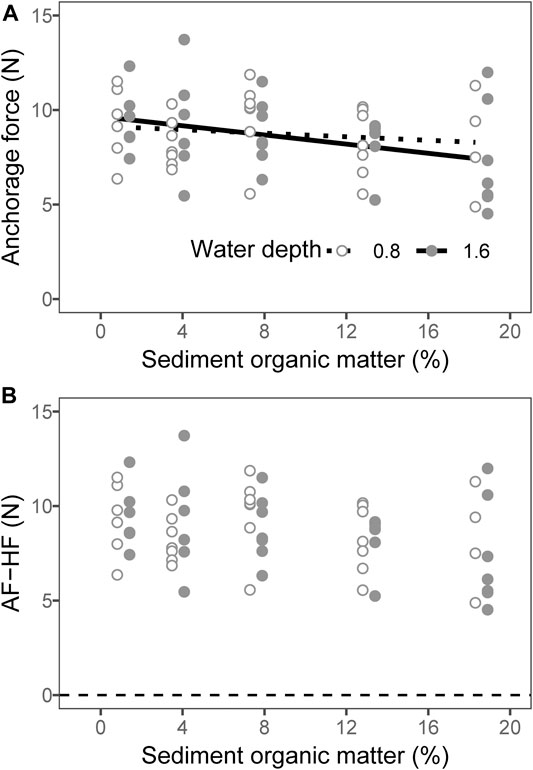
FIGURE 5. Relationship between the sediment organic matter and plant anchorage force (A). The lines are predicted by linear models. Anchorage force minus hydraulic force (AF-HF) for the sediment with different organic matter contents (B). The points of 1.6 m water depth were moved 0.6% to the right to avoid overlapping.
Discussion
Our findings revealed two important aspects with respect to how the enrichment of organic matter in sediment affects the submersed macrophytes. First, low levels of the enrichment of organic matter in the sediment (≤7%) enhanced the growth of M. spicatum by increasing the supply of phosphorus, whereas high levels of organic matter in the sediment inhibited its growth. Second, although the anchorage forces of M. spicatum decreased slightly as the amount of organic matter increased in the sediment, they were much higher than the hydraulic drag force on the plant at a relatively high current velocity. Therefore, our results suggest that the risk of uprooting M. spicatum is very low even in lakes that have high levels of organic matter in their sediment. In contrast to our hypothesis, the water depth did not alter the responses of plant growth and root anchorage of M. spicatum to the enrichment of organic matter.
Effects of the Addition of Organic Matter on Plant Growth
The growth of M. spicatum benefited from the enrichment in low levels of organic matter in the sediment. After a threshold of organic matter was reached, the growth rate of M. spicatum decreased. The optimum level of organic matter (≈7%) for the growth of M. spicatum is consistent with that identified by Barko and Smart (1986) and similar to that of Hydrilla verticillata (Silveira and Thomaz, 2015). The mechanism behind the enhancement of enrichment of organic matter on plant growth could be that the supply of phosphorus increased in parallel with that of the organic matter in sediment. This is confirmed by the results that the contents of shoot P in M. spicatum increased in parallel with that in the content of organic matter (<7%), and the growth rate of M. spicatum increased with the content of shoot P (<4%). Richter and Gross (2013) also found that M. spicatum grows poorly when the supply of phosphorus is low. However, the positive effect of increased phosphorus supply was counteracted by other stressors when the organic matter reached a high level. The stressor was not likely to be the deficiency of nutrients in sediments with a high content of organic matter as described by Barko et al. (1991), since the shoot N and P of M. spicatum growing in sediments with a high content of organic matter were comparable to those growing in moderate ones. Instead, toxicity or the anaerobic conditions induced by the high organic matter may be responsible for the decrease in growth of M. spicatum (Barko et al., 1991; Wu et al., 2009; Silveira and Thomaz, 2015). However, we found that the growth rate of M. spicatum was quite high in our experiment even in the treatment with the highest content of organic matter, indicating that M. spicatum has a high tolerance to the enrichment of organic matter in the sediment. This is consistent with the results of Lemoine et al. (2012), who found that M. spicatum can survive in anaerobic sediments and maintain similar photosynthetic rates with those under aerobic conditions by increasing their root porosity and radial loss of oxygen.
Effects of the Addition of Organic Matter on Plant Anchorage
Anchorage in the sediment is crucial for the survival and growth of submersed macrophytes in lakes (Schutten et al., 2005). The enrichment in organic matter reduced the anchorage of roots of submersed macrophytes by impairing root development and reducing the cohesive strength of the sediment (Sand-Jensen and Møller, 2014). In our study, the force of root anchorage clearly showed a slight tendency to decrease with increasing organic matter in the sediment as predicted at a water depth of 1.6 m. The root anchorage force was primarily determined by the size of plant root and the characteristics of the sediment (Sand-Jensen and Møller, 2014). No significant reduction of the root system in the sediment that contained a high content of organic matter was observed in our experiment. Thus, the reduction of anchorage force in the sediment that had a high content of organic matter was primarily owing to falling mass density and the falling cohesive binding between root surfaces and the surrounding sediment (Sand-Jensen and Møller 2014). Consistent with a previous study (Schutten et al., 2005), we demonstrated that M. spicatum had a very low risk of uprooting even in the sediment with the highest content of organic matter (18%) in our experiment because the anchorage force of the plants was much higher than the hydraulic force that the plant may be exposed in lakes (Schutten et al., 2004).
Effects of Water Depth on the Response of Plant Growth and Root Anchorage to Enriched Organic Matter
Overall, the water depth did not alter the response of plant growth and root anchorage to the enrichment of organic matter in the sediment. M. spicatum elongated its shoots to avoid low light stress and increased the contents of shoot N and P to enhance the photosynthetic efficiency at high water depths, which resulted in a similar biomass at the end of the experiment. Similar responses to low light condition were also identified in M. spicatum (Strand and Weisner, 2001) and other canopy-forming species (He et al., 2018; He et al., 2019). The canopy-forming species elongate their stems as a major strategy to respond to low light conditions, which can help these species to concentrate their leaves closer to the water surface (He et al., 2019). Increased leaf chlorophylls at low light conditions, which resulted in higher N and P contents in leaves (He et al., 2018), could further enhance their ability to absorb light (Strand and Weisner, 2001; Hussner and Heidbuchel, 2021). Interestingly, the plastic responses of M. spicatum to low light stress did not weaken its ability to manage the stresses induced by high organic matter in the sediment. Therefore, we demonstrated that M. spicatum could maintain the ability to assimilate and allocate the resources to both support growth and resist the stress of enrichment of organic matter through morphological and physiological adjustments in deep water. The ability to tolerate high amounts of organic matter in the sediment and effectively manage low light stress could be the reason for the ability of this species to survive eutrophication in lakes (Qiu and Wu, 1996; Cao et al., 2011).
In conclusion, our data showed that M. spicatum had a high tolerance to the enrichment with organic matter and could anchor without the risk of uprooting in sediments with a wide range of organic matter content. In addition, M. spicatum can effectively manage low light stress through morphological and physiological adjustments. Thus, we suggest that M. spicatum could be a potential species for the restoration of eutrophic lakes since it can grow well and anchor stably in sediments with a relatively high amount of organic matter and effectively manage low light stress.
Data Availability Statement
The original contributions presented in the study are included in the article/supplementary material. Further inquiries can be directed to the corresponding authors.
Author Contributions
LH and GG conceived the idea and proposed the method. LH and RW contributed to conduct the experiment. LH, HZ, YL, MZ, GZ, TC, and LN wrote the manuscript. All authors read and approved the final manuscript.
Funding
This work was supported by the National Science Foundation of China (Grant Nos. 31700402, 31860125, and 32071568) and Science and Technology Project of Department of Water Resources of Jiangxi Province (Grant No. 201820YBKT22).
Conflict of Interest
The authors declare that the research was conducted in the absence of any commercial or financial relationships that could be construed as a potential conflict of interest.
Publisher’s Note
All claims expressed in this article are solely those of the authors and do not necessarily represent those of their affiliated organizations, or those of the publisher, the editors, and the reviewers. Any product that may be evaluated in this article, or claim that may be made by its manufacturer, is not guaranteed or endorsed by the publisher.
Acknowledgments
We acknowledge technical assistance by Yueyao Chen, Tongde Yang, Kaipeng Shao, and Zongcheng Yao.
References
Aiken, S. G., Newroth, P. R., and Wile, I. (1979). The Biology of Canadian Weeds. 34 Myriophyllum Spicatum L. Can. J. Plant Sci. 59, 210–215. doi:10.4141/cjps79-028
Barko, J. W., Gunnison, D., and Carpenter, S. R. (1991). Sediment Interactions with Submersed Macrophyte Growth and Community Dynamics. Aquat. Bot. 41 (1), 41–65. doi:10.1016/0304-3770(91)90038-7
Barko, J. W., and Smart, R. M. (1986). Sediment-Related Mechanisms of Growth Limitation in Submersed Macrophytes. Ecology. 67, 1328–1340. doi:10.2307/1938689
Bornette, G., and Puijalon, S. (2011). Response of Aquatic Plants to Abiotic Factors: a Review. Aquat. Sci. 73 (1), 1–14. doi:10.1007/s00027-010-0162-7
Cao, T., Ni, L., Xie, P., Xu, J., and Zhang, M. (2011). Effects of Moderate Ammonium Enrichment on Three Submersed Macrophytes under Contrasting Light Availability. Freshwater Biol. 56 (8), 1620–1629. doi:10.1111/j.1365-2427.2011.02601.x
Duarte, C. M., and Kalff, J. (1986). Littoral Slope as a Predictor of the Maximum Biomass of Submerged Macrophyte Communities. Limnol. Oceanogr. 31, 1072–1080. doi:10.4319/lo.1986.31.5.1072
Fu, H., Yuan, G., Cao, T., Ni, L., Zhang, M., and Wang, S. (2012). An Alternative Mechanism for Shade Adaptation: Implication of Allometric Responses of Three Submersed Macrophytes to Water Depth. Ecol. Res. 27 (6), 1087–1094. doi:10.1007/s11284-012-0991-z
He, L., Zhu, T., Wu, Y., Li, W., Zhang, H., Zhang, X., et al. (2019). Littoral Slope, Water Depth and Alternative Response Strategies to Light Attenuation Shape the Distribution of Submerged Macrophytes in a Mesotrophic Lake. Front. Plant Sci. 10, 169. doi:10.3389/fpls.2019.00169
Hilt, S., Brothers, S., Jeppesen, E., Veraart, A. J., and Kosten, S. (2017). Translating Regime Shifts in Shallow Lakes into Changes in Ecosystem Functions and Services. Bioscience 67 (10), 928–936. doi:10.1093/biosci/bix106
Hobaek, A., Løvik, J. E., Rohrlack, T., Moe, S. J., Grung, M., Bennion, H., et al. (2012). Eutrophication, Recovery and Temperature in Lake Mjøsa: Detecting Trends with Monitoring Data and Sediment Records. Freshw. Biol. 57 (10), 1998–2014. doi:10.1111/j.1365-2427.2012.02832.x
Hussner, A., and Heidbüchel, P. (2021). Sediment-rooting Affects Growth and Biomass Allocation in Myriophyllum Spicatum under Varying Growth Conditions. Aquat. Bot. 170, 103354. doi:10.1016/j.aquabot.2021.103354
Hussner, A., and Jahns, P. (2015). European Native Myriophyllum Spicatum Showed a Higher Use Capacity Than Alien Invasive Myriophyllum Heterophyllum. Hydrobiologia 746 (1), 171–182. doi:10.1007/s10750-014-1976-4
Law, A., Baker, A., Sayer, C., Foster, G., Gunn, I. D. M., Taylor, P., et al. (2019). The Effectiveness of Aquatic Plants as Surrogates for Wider Biodiversity in Standing Fresh Waters. Freshw. Biol. 64 (9), 1664–1675. doi:10.1111/fwb.13369
Lemoine, D. G., Mermillod-Blondin, F., Barrat-Segretain, M.-H., Massé, C., and Malet, E. (2012). The Ability of Aquatic Macrophytes to Increase Root Porosity and Radial Oxygen Loss Determines Their Resistance to Sediment Anoxia. Aquat. Ecol. 46 (2), 191–200. doi:10.1007/s10452-012-9391-2
Li, F., Zhu, L., Xie, Y., Jiang, L., Chen, X., Deng, Z., et al. (2015). Colonization by Fragments of the Submerged Macrophyte Myriophyllum Spicatum under Different Sediment Type and Density Conditions. Sci. Rep. 5, 11821. doi:10.1038/srep11821
Liang, H., Chen, X., Xiaoxi, C., Wei, L., Tianshun, Z., Yao, W., et al. (2018). Leaf Carbon, Nitrogen and Phosphorus Stoichiometric Characteristics and Photosynthetic Pigments Composition of Four Submerged Macrophytes in Response to Various Water Depth in Lake Erhai, China. J. Lake Sci. 30 (5), 1413–1419. doi:10.18307/2018.0522
Lürig, M. D., Narwani, A., Penson, H., Wehrli, B., Spaak, P., and Matthews, B. (2021). Non‐additive Effects of Foundation Species Determine the Response of Aquatic Ecosystems to Nutrient Perturbation. Ecology 102 (7), e03371. doi:10.1002/ecy.3371
Phillips, G., Willby, N., and Moss, B. (2016). Submerged Macrophyte Decline in Shallow Lakes: What Have We Learnt in the Last Forty Years? Aquat. Bot. 135, 37–45. doi:10.1016/j.aquabot.2016.04.004
Pulido, C., Lucassen, E. C. H. E. T., Pedersen, O., and Roelofs, J. G. M. (2011). Influence of Quantity and Lability of Sediment Organic Matter on the Biomass of Two Isoetids, Littorella Uniflora and Echinodorus Repens. Freshw. Biol. 56 (5), 939–951. doi:10.1111/j.1365-2427.2010.02539.x
Qin, B., Xu, P., Wu, Q., Luo, L., and Zhang, Y. (2007). Environmental Issues of Lake Taihu, China. Hydrobiologia 581, 3–14. doi:10.1007/s10750-006-0521-5
Qiu, D., and Wu, Z. (1996). On the Degradation and Restoration of Shallow Eutrophic Chinese Lakes. Resour. Environ. Yangtze Valley 5, 355–361.
R Core Team (2020). R: A Language and Environment for Statistical Computing. Vienna, Austria: R Foundation for Statistical Computing.
Raun, A. L., Borum, J., and Sand-Jensen, K. (2010). Influence of Sediment Organic Enrichment and Water Alkalinity on Growth of Aquatic Isoetid and Elodeid Plants. Freshw. Biol. 55 (9), 1891–1904. doi:10.1111/j.1365-2427.2010.02423.x
Richter, D., and Gross, E. M. (2013). Chara Can Outcompete Myriophyllum under Low Phosphorus Supply. Aquat. Sci. 75 (3), 457–467. doi:10.1007/s00027-013-0292-9
Sand-Jensen, K., Borum, J., and Binzer, T. (2005). Oxygen Stress and Reduced Growth of Lobelia Dortmanna in sandy lake Sediments Subject to Organic Enrichment. Freshw. Biol 50 (6), 1034–1048. doi:10.1111/j.1365-2427.2005.01382.x
Sand-Jensen, K., and Møller, C. L. (2014). Reduced Root anchorage of Freshwater Plants in Sandy Sediments Enriched with fine Organic Matter. Freshw. Biol. 59 (3), 427–437. doi:10.1111/fwb.12275
Sand-Jensen, K., Riis, T., Vestergaard, O., and Larsen, S. E. (2000). Macrophyte Decline in Danish Lakes and Streams over the Past 100 Years. J. Ecol. 88 (6), 1030–1040. doi:10.1046/j.1365-2745.2000.00519.x
Scheffer, M., Hosper, S. H., Meijer, M. L., Moss, B., and Jeppesen, E. (1993). Alternative Equilibria in Shallow Lakes. Trends Ecol. Evol. 8 (8), 275–279. doi:10.1016/0169-5347(93)90254-m
Schutten, J., and Davy, A. J. (2000). Predicting the Hydraulic Forces on Submerged Macrophytes from Current Velocity, Biomass and Morphology. Oecologia 123, 445–452. doi:10.1007/s004420000348
Schutten, J., Dainty, J., and Davy, A. J. (2005). Root anchorage and its Significance for Submerged Plants in Shallow Lakes. J. Ecol. 93 (3), 556–571. doi:10.1111/j.1365-2745.2005.00980.x
Schutten, J., Dainty, J., and Davy, A. J. (2004). Wave-induced Hydraulic Forces on Submerged Aquatic Plants in Shallow Lakes. Ann. Bot. 93 (3), 333–341. doi:10.1093/aob/mch043
Silveira, M. J., and Thomaz, S. M. (2015). Growth of a Native versus an Invasive Submerged Aquatic Macrophyte Differs in Relation to Mud and Organic Matter Concentrations in Sediment. Aquat. Bot. 124, 85–91. doi:10.1016/j.aquabot.2015.03.004
Søndergaard, M., Johansson, L. S., Lauridsen, T. L., Jørgensen, T. B., Liboriussen, L., and Jeppesen, E. (2010). Submerged Macrophytes as Indicators of the Ecological Quality of Lakes. Freshw. Biol. 55 (4), 893–908. doi:10.1111/j.1365-2427.2009.02331.x
State Environmental Protection Administration of China (2002). Monitoring and Analytical Methods of Water and Wastewater. Beijing: China Environmental Science Press.
Strand, J. A., and Weisner, S. E. B. (2001). Morphological Plastic Responses to Water Depth and Wave Exposure in an Aquatic Plant (Myriophyllum Spicatum). J. Ecol. 89 (2), 166–175. doi:10.1046/j.1365-2745.2001.00530.x
Su, H., Wu, Y., Xia, W., Yang, L., Chen, J., Han, W., et al. (2019). Stoichiometric Mechanisms of Regime Shifts in Freshwater Ecosystem. Water Res. 149, 302–310. doi:10.1016/j.watres.2018.11.024
Van Der Molen, D. T., Portielje, R., Boers, P. C. M., and Lijklema, L. (1998). Changes in Sediment Phosphorus as a Result of Eutrophication and Oligotrophication in Lake Veluwe, The Netherlands. Water Res. 32 (11), 3281–3288. doi:10.1016/s0043-1354(98)00117-1
Wang, J., Yu, D., and Wang, Q. (2008). Growth, Biomass Allocation, and Autofragmentation Responses to Root and Shoot Competition in Myriophyllum Spicatum as a Function of Sediment Nutrient Supply. Aquat. Bot. 89 (4), 357–364. doi:10.1016/j.aquabot.2008.04.001
Wu, J., Cheng, S., Liang, W., and Wu, Z. (2009). Effects of Organic-Rich Sediment and Below-Ground Sulfide Exposure on Submerged Macrophyte, Hydrilla Verticillata. Bull. Environ. Contam. Toxicol. 83 (4), 497–501. doi:10.1007/s00128-009-9800-y
Xiao, K., Yu, D., and Wu, Z. (2007). Differential Effects of Water Depth and Sediment Type on Clonal Growth of the Submersed Macrophyte Vallisneria Natans. Hydrobiologia 589, 265–272. doi:10.1007/s10750-007-0740-4
Xie, D., Yu, D., You, W.-H., and Wang, L.-G. (2013). Morphological and Physiological Responses to Sediment Nutrients in the Submerged Macrophyte Myriophyllum Spicatum. Wetlands 33 (6), 1095–1102. doi:10.1007/s13157-013-0465-2
Xu, W., Hu, W., Deng, J., Zhu, J., Zhou, N., and Liu, X. (2016). Impacts of Water Depth and Substrate Type on Vallisneria Natans at Wave-Exposed and Sheltered Sites in a Eutrophic Large Lake. Ecol. Eng. 97, 344–354. doi:10.1016/j.ecoleng.2016.10.029
Keywords: eutrophicaion, submersed macrophyte, organic matter enrichment, sediment phosphorous, lake restoration
Citation: He L, Wang R, Zhang H, Zhang M, Liu Y, Zhu G, Cao T, Ni L and Ge G (2021) Growth and Anchorage of Myriophyllum spicatum L. in Relation to Water Depth and the Content of Organic Matter in Sediment. Front. Environ. Sci. 9:766413. doi: 10.3389/fenvs.2021.766413
Received: 29 August 2021; Accepted: 18 November 2021;
Published: 14 December 2021.
Edited by:
Xiufeng Zhang, Jinan University, ChinaReviewed by:
Jinlei Yu, Nanjing Institute of Geography and Limnology (CAS), ChinaIsabelle Combroux, Université de Strasbourg, France
Copyright © 2021 He, Wang, Zhang, Zhang, Liu, Zhu, Cao, Ni and Ge. This is an open-access article distributed under the terms of the Creative Commons Attribution License (CC BY). The use, distribution or reproduction in other forums is permitted, provided the original author(s) and the copyright owner(s) are credited and that the original publication in this journal is cited, in accordance with accepted academic practice. No use, distribution or reproduction is permitted which does not comply with these terms.
*Correspondence: Gang Ge, Z2dlQG5jdS5lZHUuY24=