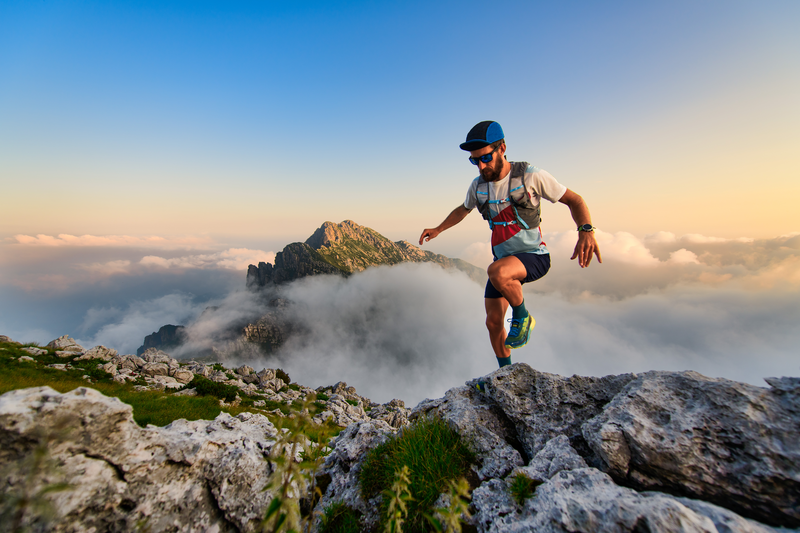
95% of researchers rate our articles as excellent or good
Learn more about the work of our research integrity team to safeguard the quality of each article we publish.
Find out more
ORIGINAL RESEARCH article
Front. Environ. Sci. , 25 November 2021
Sec. Toxicology, Pollution and the Environment
Volume 9 - 2021 | https://doi.org/10.3389/fenvs.2021.764344
This article is part of the Research Topic Fate and toxicity of emerging pollutants in the environment View all 6 articles
Microplastics (MPs) and tetracycline (TC) are severe emerging pollutants in the aquatic environment. But there is a lack of research to investigate the interactive effects of MPs and TC in vivo. This study used Jian carp (Cyprinus carpio var. Jian) as the model organism to explore the bioaccumulation and biochemical status when exposed to MPs and TC, alone and combined. The accumulation of TC and MPs in intestine, variation of enzyme activities in intestine, and expression of immune-related genes in muscle were evaluated. Our results found the bioaccumulation of MPs was not affected by TC, but the presence of MPs would change the content of TC within 48 h. The superoxide dismutase (SOD) and lactate dehydrogenase (LDH) activity showed that TC-MP combined exposure could reduce oxidative damage to Jian carps compared to MP exposure alone. The integrated biomarker response (IBR) index showed that SOD activity was sensitive to TC-MP exposure. In addition, co-exposure to MPs and TC could alleviate the overexpression of interleukin 1 beta (IL-1β), interleukin 10 (IL-10), transforming growth factor beta (TGF-β), and toll like receptor 2 (TLR-2) induced by TC in muscles. TLR-2 gene has the potential to be the candidate gene reflecting the injury of TC exposure. In conclusion, it is inferred that co-exposure may reduce the toxicity of individual exposure in the living organisms. This study provides essential information for the risk assessment of pollution with MPs and TC, individually and combined, as well as a foundation to investigate the interactive effects of MPs and antibiotics on aquatic ecosystems.
Modern life seems to be inextricably linked to the use of plastics. Plastics have become an indispensable solution to meet the ever-changing needs of society. Global production nearly reached 370 million tons (Mts) in 2019 at an average annual rate of 3.1% (Plastics Europe, 2020). However, the extensive use of plastics has given rise to millions of plastic wastes being discarded into the environment (Cózar et al., 2014; Jambeck et al., 2015). Plastic pollution has been an urgent challenge nowadays, which cause severe damage to various ecosystems, both directly and indirectly, especially as the main source of microplastics in aquatic ecosystems and organisms (de Sa et al., 2018; Fu and Wang, 2019; Jacob et al., 2020; Zhou et al., 2021). Microplastics (MPs), originating from both primary and secondary sources, are tiny plastic particles of less than 5 mm in size (Thompson et al., 2004; Cole et al., 2011). They are not only ubiquitously distributed in marine and freshwater ecosystems (Beaumont et al., 2019; Sarijan et al., 2021), atmosphere (Zhang et al., 2020a; Chen et al., 2020), and soil (He et al., 2018; Wang et al., 2020) but also found in the most remote habitats (Morgana et al., 2018). For example, 1,190 synthetic polymers <5 mm from sea ice cores and 125 synthetic polymers from surface water samples were detected in the Arctic Central Basin (Kanhai et al., 2020). In China, MPs are pervasive in surveyed freshwater environments, and the detected MPs mainly show smaller size (<1 mm), fibers, and transparency in freshwater ecosystems (Fu and Wang, 2019). Wright et al. (2020) found that the atmospheric deposition rates of MPs in atmosphere in central London were 771 ± 167 particles/m2/d, and fibers were the overwhelming majority (Wright et al., 2020). To sum up, MP pollution is drawing attention on a global scale.
MPs may be ingested by various organisms because of their small size and/or similar food shape, including fish (Jovanovic, 2017), shellfish (Ding et al., 2020), birds (Carlin et al., 2020), and mammals (Hernandez-Gonzalez et al., 2018). Adverse effects caused by MP ingestion on organisms have been extensively demonstrated. For example, the activities of SOD and catalase (CAT) in zebrafish were significantly increased by polystyrene MP exposure, indicating that oxidative stress could be caused by MPs (Lu et al., 2016). Further, if exposed to MPs at environmentally relevant concentrations, marine medaka (Oryzias melastigma) could delay gonad maturation, decrease fecundity, and negatively regulate female HPG axis in female fish (Wang et al., 2019). What is more, MPs can absorb many other pollutants, potentially altering their environmental fate and ecological impact, and producing multiple forms of toxicity after being ingested (Barboza et al., 2018; Trevisan et al., 2019; Zhou et al., 2020). Many studies have reported that owing to their strong hydrophobicity and large specific surface area, MPs can adsorb heavy metals (Brennecke et al., 2016; Mao et al., 2020), polycyclic aromatic hydrocarbons (PAHs) (Kleinteich et al., 2018; José and Jordao, 2020), polychlorinated biphenyls (PCBs) (Llorca et al., 2020), pharmaceutically active compounds (PHACs) (Li et al., 2018), and so on. For example, the existence of MPs could influence the metabolism of roxithromycin (ROX) in red tilapia (Oreochromis niloticus), but co-exposure mitigated oxidative damage in fish livers (Zhang et al., 2019). Trevisan et al. (2019) indicated that nanoplastics promoted the sorption of PAHs from the exposure medium, increased the agglomeration rate of nanoplastics, and decreased the bioavailability and bioaccumulation of PAHs. Although research on combined MPs and other contaminants is in progress, reports about the interactive effects between MPs and pharmaceutically active compounds (PhACs) is still insufficient, due to the diversity of biological types and the lack of standard dose of pollutants.
Tetracycline (TC) is one of the major categories in PhACs. As an ionizable and polar antibiotic, TC plays an important role preventing and treating diseases in livestock farming, although most of it cannot be absorbed by livestock, thus draining into the environment (Fu et al., 2021; Scaria et al., 2021). Our research team previously found that the contents of tetracyclines were significantly higher than that of sulfonamides in Guangdong coastal areas, ranging from 0.26 to 81.54 ng/L (Xu et al., 2019). Recent studies confirmed that MPs could adsorb TC on the surface mainly through an ion exchange mechanism (Zhao et al., 2021), owing to the surface properties of MPs and chemical characteristics of the aqueous solution (Wan et al., 2019; Yu et al., 2020). The depletion of the bees’ gut microbiota using TC dramatically increased the lethality of MPs (Wang et al., 2021). MPs compound with TC caused gastric cancer cell damage under 24 h exposure (Yan et al., 2020). Oral exposure to MPs and TC resulted in significant bioaccumulation of TC in Enchytraeus crypticus, increased the anti-resistance gene (ARG) diversity and abundances, and significantly perturbed the balance of microbiome (Ma et al., 2020). However, the potential toxicological impact and ecological risk of the combination of MPs and TC, especially on vertebrates, still need further study to be understood.
The present study aims to evaluate interactive effects of MPs and TC on bioaccumulation and biochemical status in fish. In this study, the common freshwater carp (Cyprinus carpio var. Jian) was used as the model organism. Jian carp is the first artificially bred aquatic species in China and has been an important economic fish species cultured nationwide (Gu et al., 2015). The effects of fluorescent polystyrene microplastics (PS-MPs; average diameter: 5 μm; concentration: 700 μg/L) and TC (concentration: 1 μg/L) were investigated. Selected materials and concentrations were based on the results of our previous experiment (unpublished data). The accumulations of TC and MPs in intestine, variation of enzyme activities in intestine, and expression of immune-related genes in muscle were evaluated. Our results provide important information for the risk assessment of pollution caused by MPs and PHACs on fish, as well as form a foundation to investigate the interactive impacts of MPs and antibiotics on aquatic ecosystems.
The green fluorescent polystyrene microspheres (PS-MPs; inspire: 488 nm, launch: 518 nm) with the average size of 5 μm were bought from Da'E Scientific Co., Ltd. (Tianjin, China). Fluorosphere dyes were contained in PS-MPs, rather than adhere to the surface. Accordingly, the dyes of the potential effects on experimental were negligible (Zhang et al., 2019). PS-MPs were stored in the dark at 4°C and treated with ultrasound before application. Tetracycline (TC; analytical grade; purity >98%) was bought from Heowns Biochemical Technology Co., Ltd. (Tianjin, China) and stored in the dark at 4°C. The chemical stock solution was prepared in ultrapure water with concentration of 0.389 mg/ml.
Healthy Jian carp were bought from an aquafarm (Shunde, Guangdong, China) and acclimated in 50 L glass aquariums in College of Marine science, South China Agricultural University for 2 weeks. Fishes were fed twice per day with 3–6% commercial feeds of their body weight until 3 days before the start of the test. During the experiment, MPs and TC were added according to the experiment design in corresponding tanks. We set up the following four experimental groups: the control group (dechlorinated circulating water), TC exposure group (1 μg/L), TC-MP exposure group (700 μg/L PS-MPs + 1 μg/L TC), and MP exposure group (700 μg/L). The selected concentrations were suitable for the detection of the variation of physiological and biochemical indexes in fish and the minimum value for instrument. In addition, our research team previously investigated the contents of tetracyclines and MPs in the field (Xu et al., 2019; Zhang et al., 2020b). The chosen concentrations were based on our field investigation results and laboratory simulation verification (Zhang et al., 2021). Aeration was set in each experimental group to prevent the uneven distribution of MPs and TC. After being starved, 120 carps (3.57 ± 0.25 cm in body length, 0.96 ± 0.25 g in wet weight) were randomly placed in four 50 L glass tanks containing 30 L test solutions (temperature 25.0 ± 2.0°C; pH 7.5 ± 0.3; dissolved oxygen >6.0 mg/L). During the test, water was not changed.
After 48 and 96 h exposure, four fishes were taken from each group and rinsed to remove surficial body particles. Three replicates were performed for each treatment group. The weight and length of sampled fish were measured and recorded, then the fishes were sacrificed to recover the intestine, liver, and muscle. The above processes were carried out while the fish were anesthetized, and animal welfare was considered. The sampled tissues of Jian carp were stored at –80°C for further studies. The enteric samples were used for enzyme activity assay and bioaccumulation measurements of MPs and TC. The sarcous samples were used for the detection of quantitative polymerase chain reaction (qPCR). Due to the light weight of the liver, hepatic samples were synthesized to detect the content of TC in each group.
The concentration of PS-MPs in intestinal samples was analyzed according to Van Cauwenberghe and Janssen (2014) and Van Cauwenberghe et al. (2015) with some slight modifications. To be specific, intestine samples were weighted and digested in 1 ml KOH (10%, v: v) at 60°C for 24 h. After complete digestion, 10 μl digestion solution was added in the hemocytometer XB-K-25 (Shanghai Qiujing Biochemical Reagent Instrument Co., Ltd.) and the number of MPs was counted under a polarizing microscope (Model Eclipse E200; Nikon, Inc., Japan) equipped with the MShot Image Analysis System 1.1.4. MP concentration was expressed as the number of MPs per gram of intestinal wet weight (particles/g).
On the basis of Ding et al. (2016), a slight modification was made to improve the method of sample preparation and extraction. Specifically, each intestine and liver sample was homogenized with 1.5 ml methanol in the high-throughput tissue grinder (Shanghai Jingxin Industrial Development Co., Ltd.) at 4°C. After centrifuging at 4,000 rpm for 15 min at 4°C, the supernatant was transferred to a clean centrifuge tube for analysis of TC concentration using an ultra-high-performance liquid chromatography-tandem mass spectrometer (UPLC/MS/MS, Uplc1290-6470A, Agilent, United States). The TC concentration was expressed as ng/g in wet weight.
In the application of biomarkers, glutathione (GSH) content, SOD, and LDH enzyme activity in the intestine were applied to evaluate oxidative damage at the protein level. The enteric samples in each treatment group were homogenized with ice-cold 0.9% saline solution (1 g: 9 ml) with a high-throughput tissue grinder (Shanghai Jingxin Industrial Development Co., Ltd.). After centrifuging at 3,000 g for 10 min at 4°C, the supernate was transferred to the clean centrifuge tube for the analysis of biomarkers by using microplate test kits following the manufacturer’s instructions (Nanjing Jiancheng Bioengineering Institute, China). All the above indexes were detected by a microplate reader (Synergy™ HTX Multi-Mode Microplate Reader, Biotek, VT., United States) and analyzed by the Gen5 software (Gen5 CH5 3.03., Biotek, VT., United States). Three replicates were performed.
The expression levels of genes related to IL-1β, IL-10, TGF-β, and TLR-2 in muscle were applied to evaluate immune stress at the gene level. The experimental methods of RNA extraction and cDNA synthesis are presented in Supplementary Text S1. The cDNA was stored at −80°C until further analysis. The expression levels of the genes were quantified via RT-PCR assay. Details of the RT-PCR materials and program are presented in Supplementary Text S2. With 18s as the internal standard gene, the selected gene was amplified with specific primers. Specific primer sequences are listed in Table 1 (Meng et al., 2021).
All data were quantified as mean ± standard deviation (SD). The statistical significance between the control group and the experimental group was analyzed by one-way ANOVA with Tukey’s post-hoc test using the SPSS 17.0 (SPSS Inc., United States). Differences were considered significant at p < 0.05 and highly significant at p < 0.01. IBR was the integration of all measured biomarker responses into one general “stress index” (Beliaeff and Burgeot, 2002). The specific method of IBR calculation (Broeg and Lehtonen, 2006) can be found in Supplementary Text S3. The relative gene expression heat map was produced by GraphPad Prism 8 (GraphPad Software, San Diego, CA, United States) software.
During the exposure period, no acute toxicity, such as mortality or abnormalities of the treated fish, was observed. Besides, no MPs were observed in fish intestines of control group or TC exposure group. The average concentration of MPs in water was about 106 particles/L. In the intestine of Jian carp, the average concentration of MPs in TC-MP exposure group in 48 and 96 h was 2.29×104 ± 5.86×103 and 5.93×104 ± 2.88×103 particles/g, respectively. The concentration in MP exposure group was 2.51×104 ± 4.82×103 and 4.55×104 ± 8.92×103 particles/g in 48 and 96 h, respectively (Figure 1A). The concentration of MPs in the treatment groups exposed for 96 h was approximately 2–3 times higher than that of the treatment groups exposed for 48 h (p < 0.05). The above results indicated that MPs in intestine gradually increased with time at both groups with MPs, and TC had barely effect on the accumulation of MPs. MPs are synthetic hydrophobic polymer with high molecular weight, which are difficult to be metabolized or degraded by organisms (Rist et al., 2017). MPs easily accumulate in the gastrointestinal tract of organisms, and the accumulation increases over time until an equilibrium state is reached (Zhang et al., 2019). Huang et al. (2021) also found that the accumulation pattern of MPs in fish did not vary with the presence of antibiotic. The accumulation of MPs in the intestine is most likely related to their physical properties (e.g., size and shape, difficulty in degradation, etc.) (Hirt and Body-Malapel, 2020; Huang et al., 2021).
FIGURE 1. Bar graph presentation of PS-MP concentration (A) and TC concentration (B) variation in intestines of Jian carp treated with various exposure treatments of Control, TC + MP group, and MP group during 48 and 96 h exposure. The values represent mean ± SD of three independent experiments. Different lowercase letters indicate statistically significant difference between treatments (p < 0.05).
The accumulation of TC in intestine and liver of Jian Carp was detected at two exposure times (48 and 96 h). The average concentrations of TC in the intestines were 206.00 ± 54.44, 21.37 ± 7.56, 30.50 ± 3.65, and 16.91 ± 7.99 ng/g, corresponding to TC-48 h, TC-96 h, TC + MPs-48 h, and TC + MPs-96 h treatments, respectively (Figure 1B). The presence of MPs extremely reduced the enteric accumulation of TC in the short-term (p < 0.01). It is reported that the absorption of TC in fish is mainly through gill adsorption and oral administration (Zhang et al., 2019). The basic structure of TC consists of a hydronaphthacene nucleus containing four hexacyclic fused rings, which facilitates the passage of TC through biological membranes into the organism (Dong et al., 2012). Xu et al. (2020) reported that drug absorption occurs mainly in the foregut and midgut, but may also occur to some extent in the hindgut. Normally, TC is attached to the intestine for transmembrane transport, but the presence of MPs may alter the distribution of TC in vivo. MPs have adsorption properties for antibiotics due to the presence of porous polymer, spherical protrusions and micropores on the surface, and internal cross-section (Hirt and Body-Malapel, 2020). The establishment of hydrogen bonds and adsorption of MPs may be related to the fact that TC have multipolar functional groups, such as ketone, hydroxyl, and amino groups (Shen et al., 2018). Feng et al. (2020) revealed that the surface charge of MPs that adsorb TC affected the toxicity of TC to cells. In brief, MPs can act as carriers of TC into fish and may change the fate and toxicological effect of TC.
We also analyzed the concentration of TC in fish liver based on limited samples with tiny weight. The concentration of TC in liver of Jian carp was 41.85, 97.02, 29.63, and 20.61 ng/g, corresponding to TC-48 h, TC-96 h, TC + MPs-48 h, and TC + MPs-96 h treatments, respectively (Supplementary Figure S1). According to the results of TC concentrations between intestine and liver, we assume that TC in intestine might be transferred to the liver in some way (such as intestinal absorption and penetration) after 48 h exposure. Liver is an important storage organ, and its main function in fish is detoxification. The accumulation of TC facilitates detoxification through some defense mechanisms (Nunes et al., 2015). We found that the distribution of TC in vivo was influenced by the presence of MPs within 48 h. During the same exposure time, TC exposure group showed high concentration of TC in the intestine, while TC + MP exposure group showed high concentration of TC in the liver. MPs acted as adsorption carriers and reduced the bioaccumulation of chemical contaminants (Tourinho et al., 2019). We speculated that MPs transported TC to other tissues in the metabolic manner. However, our results only indicated the presence or absence of MPs, corresponding to a high accumulation of TC in the intestine or liver in the short term, and whether there is a substance transfer mechanism between them still needs to be investigated in depth.
It is well known that SOD is considered as the first-line defense against oxidative stress, which contributes to the conversion of reactive oxygen species (ROS) to harmless metabolites (Xie et al., 2016; Neamat-Allah et al., 2019). LDH, which is essential for cellular respiration, is released into the blood by damaged or diseased tissues. Gholamhosseini et al. (2020) found that when exposed to infection or stressed conditions, lower LDH activity might be beneficial to fish. The generation of endogenous antioxidants like SOD and GSH is recognized to neutralize toxic free radicals, as to maintain redox hemostasis and normal cell function (Abdel Mageid et al., 2019; Abdel-Daim et al., 2019). The rising level of GSH is possibly the primary mean of preventing generation of lipid hydroperoxide (Carmo et al., 2019).
SOD and LDH activities, as well as GSH content in the intestines of Jian carp in each group are presented in Figure 2. After 96 h of exposure, the MP group showed significant upregulation in SOD and LDH activity (p < 0.05), indicating that MPs could induce enteric oxidative stress in Jian carp. In contrast to the MP exposure group, the TC-MP and TC exposure groups showed no significant variations. Multiple evidence suggested that imbalance between ROS production and antioxidant defense would lead to oxidative damages, which related to the poisoning of aquatic organisms (Kaya et al., 2015; Giordo et al., 2020). Yonar (2012) found that oxytetracycline could lead to a significant reduction of SOD activity in a rainbow trout study. Nunes et al. (2015) showed that TC caused different toxic phenomena, including oxidative stress and neurotoxicity. Although antibiotics might cause oxidative stress by reducing the antioxidant capacity, our results did not find significant oxidative stress indicators in TC exposure group. It implies that TC is not the main factor causing oxidative stress in 96 h. Combined with the results above and Figure 1, we speculated that the presence of MPs alone caused oxidative stress in aquatic organisms. Our results revealed that the combination of TC and MPs alleviated the oxidative stress in the intestine of Jian carp and protected the fish from oxidative damage to some extent. A potential explanation is that TC could be adsorbed by MPs and then weaken the toxicological effects of MPs. Zhang et al. (2019) also revealed that after 14 d of exposure in red tilapia, co-exposure to MPs and ROX mitigated oxidative damage in fish livers. Therefore, the studied compounds might have a synergic effect in vivo.
FIGURE 2. Biochemical responses in intestines of Jian carp exposure for 96 h to TC and MPs. Key: (A) SOD activity; (B) LDH activity; (C) GSH level. The values represent mean ± SD of three independent experiments. Different lowercase letters indicate statistically significant difference between treatments (p < 0.05).
The IBR index scores the response of multiple biomarkers and summarizes them into a single value for assessing the toxicological impact of contaminants. Huang et al. (2021) compared the contamination stress of red tilapia by the interaction of selected pharmaceuticals with MPs using the IBR index. It is recognized that the IBR index serves as a straightforward and valid tool, which clearly describes the health status of the organism. In this study, IBR was used to visually compare the stress among various treatments of Jian carps by integrating the multi-biomarker responses (SOD, LDH, and GSH) for 96 h of exposure (Figure 3A). The IBR values of SOD in TC-MP combined exposure group were higher than those of MP exposure group, indicating that SOD activity was more sensitive to the co-exposure. All the studied biomarkers of fish were significantly altered due to exposure to MPs, but SOD activity was the most fluctuant biomarker in TC-MP group. The estimated value of SOD remained high level throughout the study, which was a feedback to cope with oxidative stress exerted by MPs efficiently. In summary, seeking for the sensitive biomarker of pollutants should be further studied, and the effort will help to estimate the interaction between MPs and other PhACs on aquatic organisms.
FIGURE 3. (A): Integrated biomarker response (IBR) of selected biomarkers in different treatments after exposure for 96 h; (B): Heat map presentation of the relative expression of immune-related genes in muscle of Jian carp exposure for 96 h.
Cytokines are considered to be an important regulator in the fish immune system (Zou and Secombes, 2016). The regulation of inflammatory response is the integration of stimulating and inhibiting signaling pathways in the immune system, which is a complex response to a variety of stimuli like pathogens and/or tissue damage (Yang et al., 2014). Therefore, the expression level of IL-1β, IL-10, TGF-β, and TLR-2 can reflect the inflammation of the organisms. In this study, sarcous mRNA expression profiles of the above genes in Jian carp after exposure of 96 h are shown in Figure 4. The relative expression levels of all detected genes only in TC exposure group were significantly upregulated (p < 0.01), while genes in TC-MP exposure group and MP exposure group were not.
FIGURE 4. Bar graph presentation of relative expression of immune-related genes (A) IL-1β, (B) IL-10, (C) TGF-β, and (D) TLR-2 in muscle of Jian carp after exposure for 96 h. Values are expressed as mean ± SD from triplicate groups. Different lowercase letters indicate statistically significant difference between treatments (p < 0.05).
The indicators of antibiotic toxicity are morphological and genetic changes. Studies on the genotoxicity caused by antibiotics in fish are generally concerned with the transcriptional regulation of antioxidant enzymes in muscle (Yang et al., 2020). Based on the changes of these indexes in fish tissues induced by antibiotics, it is speculated that antibiotics inhibit the survival, development, and hatchery rate of fish mainly by disrupting the intracellular redox balance and inducing oxidative stress. For example, oxytetracycline regulated the expression of IL-1β and TNF-α in the intestinal tract and liver of Nile tilapia (Limbu et al., 2018). Yu et al. (2019) revealed that chlortetracycline (CTC) or oxytetracycline (OTC) significantly impaired the antioxidant capacity in zebrafish larvae. Our study found that TC induced upregulation of immune-related genes after 96 h exposure in the muscle of Jian carp. Interestingly, the presence of MPs reduced such stress to some extent, possibly by changing the migration, accumulation, and metabolism of TC in fish. We speculated that 5 μm PS-MPs might adhere TC and prevent it from functioning in muscles. Zhang et al. (2019) also found that the presence of MPs affected metabolism of ROX in tilapia according to the variability of cytochrome P450 (CYP) enzyme activities in fish livers.
The heat map visually displayed the sensitive degree of relative expression of the target genes (Figure 3B). Results showed that TLR-2 gene had the potential to be the candidate gene reflecting the injury of TC exposure. TLR-2, as a pattern recognition receptor (PRRS), directly involves in the recognition of specific pathogen-associated molecular patterns (PAMPs) and activates proinflammatory cytokines in fish, which is important in initiating immune immobilization (Noor-Ul et al., 2020). Laboratory studies into the mechanism of combined antibiotic and MP exposure in fish are still in the early stages. More researches are needed to meet the challenge in future environmental toxicity studies.
This study investigated the interactive effects of microplastics and tetracycline on the bioaccumulation and biochemical status in Jian carp. Results revealed that accumulation of MPs was not affected by TC, but the presence of MPs changed the content of TC in vivo within 48 h. Exposure to MPs alone increased SOD and LDH activity in intestine of fish, while co-exposure to TC-MPs mitigated the oxidative damage. The upregulation of IL-1β, IL-10, TGF-β, and TLR-2 induced by TC in muscles was alleviated in the presence of MPs. We speculated that 5 μm PS-MPs might adhere TC and prevent it from functioning in muscles. Overall, this study suggests that the fate and impact of TC and MPs are affected by each other in vivo. Co-exposure may have the synergic effect in Jian carp. Adsorption and desorption rates between TC and MPs need to be focused in the future study. MP physical properties (e.g., size, shape, materials, concentration, exposure time, etc.) and fish feature should be clarified clearly. More studies about biological interaction of MPs and antibiotics are needed to perfect the evidence.
The original contributions presented in the study are included in the article/Supplementary Material. Further inquiries can be directed to the corresponding authors.
The animal study was reviewed and approved by National Institute of Health Guide for the Care and Use of Laboratory Animals of China.
All authors listed have made a substantial, direct, and intellectual contribution to the work and approved it for publication.
This study was funded by the Key Realm R&D Program of Guangdong Province (No. 2020B0202080005) and China Agriculture Research System of MOF and MARA (CARS-45-50).
Author JD was employed by company Guangzhou Liyang Aqua-Technology CO., LTD.
The remaining authors declare that the research was conducted in the absence of any commercial or financial relationships that could be construed as a potential conflict of interest.
All claims expressed in this article are solely those of the authors and do not necessarily represent those of their affiliated organizations, or those of the publisher, the editors, and the reviewers. Any product that may be evaluated in this article, or claim that may be made by its manufacturer, is not guaranteed or endorsed by the publisher.
The Supplementary Material for this article can be found online at: https://www.frontiersin.org/articles/10.3389/fenvs.2021.764344/full#supplementary-material
Abdel Mageid, A. D., Zaki, A. G., El Senosi, Y. A., El Asely, A. M., Fahmy, H. A., El Kassas, S., et al. (2019). The Extent to Which Lipopolysaccharide Modulates Oxidative Stress Response in Mugil cephalus Juveniles. Aquac. Res. 51, 426–431.
Abdel-Daim, M. M., Eissa, I. A. M., Abdeen, A., Abdel-Latif, H. M. R., Ismail, M., Dawood, M. A. O., et al. (2019). Lycopene and Resveratrol Ameliorate Zinc Oxide Nanoparticles-Induced Oxidative Stress in Nile tilapia, Oreochromis niloticus. Environ. Toxicol. Pharmacol. 69, 44–50. doi:10.1016/j.etap.2019.03.016
Barboza, L. G. A., Vieira, L. R., and Guilhermino, L. (2018). Single and Combined Effects of Microplastics and Mercury on Juveniles of the European Seabass (Dicentrarchus labrax): Changes in Behavioural Responses and Reduction of Swimming Velocity and Resistance Time. Environ. Pollut. 236, 1014–1019. doi:10.1016/j.envpol.2017.12.082
Beaumont, N. J., Aanesen, M., Austen, M. C., Börger, T., Clark, J. R., Cole, M., et al. (2019). Global Ecological, Social and Economic Impacts of marine Plastic. Mar. Pollut. Bull. 142, 189–195. doi:10.1016/j.marpolbul.2019.03.022
Beliaeff, B., and Burgeot, T. (2002). Integrated Biomarker Response: a Useful Tool for Ecological Risk Assessment. Environ. Toxicol. Chem. 21, 1316–1322. doi:10.1002/etc.5620210629
Brennecke, D., Duarte, B., Paiva, F., Caçador, I., and Canning-Clode, J. (2016). Microplastics as Vector for Heavy Metal Contamination from the marine Environment. Estuarine, Coastal Shelf Sci. 178, 189–195. doi:10.1016/j.ecss.2015.12.003
Broeg, K., and Lehtonen, K. K. (2006). Indices for the Assessment of Environmental Pollution of the Baltic Sea Coasts: Integrated Assessment of a Multi-Biomarker Approach. Mar. Pollut. Bull. 53, 508–522. doi:10.1016/j.marpolbul.2006.02.004
Carlin, J., Craig, C., Little, S., Donnelly, M., Fox, D., Zhai, L., et al. (2020). Microplastic Accumulation in the Gastrointestinal Tracts in Birds of Prey in central Florida, USA. Environ. Pollut. 264, 114633. doi:10.1016/j.envpol.2020.114633
Carmo, T. L. L., Siqueira, P. R., Azevedo, V. C., Tavares, D., Pesenti, E. C., Cestari, M. M., et al. (2019). Overview of the Toxic Effects of Titanium Dioxide Nanoparticles in Blood, Liver, Muscles, and Brain of a Neotropical Detritivorous Fish. Environ. Toxicol. 34, 457–468. doi:10.1002/tox.22699
Chen, G., Fu, Z., Yang, H., and Wang, J. (2020). An Overview of Analytical Methods for Detecting Microplastics in the Atmosphere. Trac Trends Anal. Chem. 130, 115981. doi:10.1016/j.trac.2020.115981
Cole, M., Lindeque, P., Halsband, C., and Galloway, T. S. (2011). Microplastics as Contaminants in the Marine Environment: A Review. Mar. Pollut. Bull. 62, 2588–97. doi:10.1016/j.marpolbul.2011.09.025
Cózar, A., Echevarría, F., González-Gordillo, J. I., Irigoien, X., Úbeda, B., Hernández-León, S., et al. (2014). Plastic Debris in the Open Ocean. Proc. Natl. Acad. Sci. 111, 10239–10244. doi:10.1073/pnas.1314705111
de Sá, L. C., Oliveira, M., Ribeiro, F., Rocha, T. L., and Futter, M. N. (2018). Studies of the Effects of Microplastics on Aquatic Organisms: What Do We Know and where Should We Focus Our Efforts in the Future. Sci. Total Environ. 645, 1029–1039. doi:10.1016/j.scitotenv.2018.07.207
Ding, J., Li, J., Sun, C., Jiang, F., He, C., Zhang, M., et al. (2020). An Examination of the Occurrence and Potential Risks of Microplastics across Various Shellfish. Sci. Total Environ. 739, 139887. doi:10.1016/j.scitotenv.2020.139887
Ding, J., Lu, G., and Li, Y. (2016). Interactive Effects of Selected Pharmaceutical Mixtures on Bioaccumulation and Biochemical Status in Crucian Carp (Carassius auratus). Chemosphere 148, 21–31. doi:10.1016/j.chemosphere.2016.01.017
Dong, L., Gao, J., Xie, X., and Zhou, Q. (2012). DNA Damage and Biochemical Toxicity of Antibiotics in Soil on the Earthworm Eisenia fetida. Chemosphere 89, 44–51. doi:10.1016/j.chemosphere.2012.04.010
Feng, L.-J., Shi, Y., Li, X.-Y., Sun, X.-D., Xiao, F., Sun, J.-W., et al. (2020). Behavior of Tetracycline and Polystyrene Nanoparticles in Estuaries and Their Joint Toxicity on marine Microalgae Skeletonema Costatum. Environ. Pollut. 263, 114453. doi:10.1016/j.envpol.2020.114453
Fu, Y., Chen, Y., Liu, D., Yang, D., Liu, Z., Wang, Y., et al. (2021). Abundance of Tigecycline Resistance Genes and Association with Antibiotic Residues in Chinese Livestock Farms. J. Hazard. Mater. 409, 124921. doi:10.1016/j.jhazmat.2020.124921
Fu, Z., and Wang, J. (2019). Current Practices and Future Perspectives of Microplastic Pollution in Freshwater Ecosystems in China. Sci. Total Environ. 691, 697–712. doi:10.1016/j.scitotenv.2019.07.167
Gholamhosseini, A., Hosseinzadeh, S., Soltanian, S., Banaee, M., Sureda, A., Rakhshaninejad, M., et al. (2020). Effect of Dietary Supplements of Artemisia Dracunculus Extract on the Haemato‐immunological and Biochemical Response, and Growth Performance of the Rainbow trout (Oncorhynchus mykiss). Aquac. Res.. doi:10.1111/are.15062
Giordo, R., Nasrallah, G. K., Al-Jamal, O., Paliogiannis, P., and Pintus, G. (2020). Resveratrol Inhibits Oxidative Stress and Prevents Mitochondrial Damage Induced by Zinc Oxide Nanoparticles in Zebrafish (Danio rerio). Ijms 21, 3838. doi:10.3390/ijms21113838
Gu, Y., Lu, C., Zhang, X., Li, C., Yu, J., and Sun, X. (2015). Genetic Mapping and QTL Analysis for Body Weight in Jian Carp (Cyprinus carpio Var. Jian) Compared with Mirror Carp (Cyprinus carpio L.). Chin. J. Ocean. Limnol. 33 (3), 636–649. doi:10.1007/s00343-015-4207-6
He, D., Luo, Y., Lu, S., Liu, M., Song, Y., and Lei, L. (2018). Microplastics in Soils: Analytical Methods, Pollution Characteristics and Ecological Risks. Trac Trends Anal. Chem. 109, 163–172. doi:10.1016/j.trac.2018.10.006
Hernandez-Gonzalez, A., Saavedra, C., Gago, J., Covelo, P., Santos, M. B., and Pierce, G. J. (2018). Microplastics in the Stomach Contents of Common Dolphin (Delphinus delphis) Stranded on the Galician Coasts (NW Spain, 2005-2010). Mar. Pollut. Bull. 137, 526–532. doi:10.1016/j.marpolbul.2018.10.026
Hirt, N., and Body-Malapel, M. (2020). Immunotoxicity and Intestinal Effects of Nano- and Microplastics: a Review of the Literature. Part. Fibre Toxicol. 17, 57. doi:10.1186/s12989-020-00387-7
Huang, Y., Ding, J., Zhang, G., Liu, S., Zou, H., Wang, Z., et al. (2021). Interactive Effects of Microplastics and Selected Pharmaceuticals on Red tilapia: Role of Microplastic Aging. Sci. Total Environ. 752, 142256. doi:10.1016/j.scitotenv.2020.142256
Jacob, H., Besson, M., Swarzenski, P. W., Lecchini, D., and Metian, M. (2020). Effects of Virgin Micro- and Nanoplastics on Fish: Trends, Meta-Analysis, and Perspectives. Environ. Sci. Technol. 54, 4733–4745. doi:10.1021/acs.est.9b05995
Jambeck, J. R., Geyer, R., Wilcox, C., Siegler, T. R., Perryman, M., Andrady, A., et al. (2015). Plastic Waste Inputs from Land into the Ocean. Science 347, 768–771. doi:10.1126/science.1260352
José, S., and Jordao, L. (2020). Exploring the Interaction between Microplastics, Polycyclic Aromatic Hydrocarbons and Biofilms in Freshwater. Polycyclic Aromatic Compd., 1–12. doi:10.1080/10406638.2020.1830809
Jovanovic, B. (2017). Ingestion of Microplastics by Fish and its Potential Consequences from a Physical Perspective. Integr. Environ. Assess. Manag. 13, 510–515. doi:10.1002/ieam.v13.1
Kanhai, L. D. K., Gardfeldt, K., Krumpen, T., Thompson, R. C., and O’Connor, I. (2020). Microplastics in Sea Ice and Seawater beneath Ice Floes from the Arctic Ocean. Sci. Rep. 10, 5004. doi:10.1038/s41598-020-61948-6
Kaya, H., Aydın, F., Gürkan, M., Yılmaz, S., Ates, M., Demir, V., et al. (2015). Effects of Zinc Oxide Nanoparticles on Bioaccumulation and Oxidative Stress in Different Organs of tilapia (Oreochromis niloticus). Environ. Toxicol. Pharmacol. 40, 936–947. doi:10.1016/j.etap.2015.10.001
Kleinteich, J., Seidensticker, S., Marggrander, N., and Zarfl, C. (2018). Microplastics Reduce Short-Term Effects of Environmental Contaminants. Part II: Polyethylene Particles Decrease the Effect of Polycyclic Aromatic Hydrocarbons on Microorganisms. Ijerph 15, 287. doi:10.3390/ijerph15020287
Limbu, S. M., Zhou, L., and Sun, S. (2018). Chronic Exposure to Low Environmental Concentrations and Legal Aquaculture Doses of Antibiotics cause Systemic Adverse Effects in Nile tilapia and Provoke Differential Human Health Risk. Environ. Int. 115, 205–19. doi:10.1016/j.envint.2018.03.034
Llorca, M., Ábalos, M., Vega-Herrera, A., Adrados, M. A., Abad, E., and Farré, M. (2020). Adsorption and Desorption Behaviour of Polychlorinated Biphenyls onto Microplastics' Surfaces in Water/Sediment Systems. Toxics 8, 59. doi:10.3390/toxics8030059
Li, J., Zhang, K., and Zhang, H. (2018a). Adsorption of Antibiotics on Microplastics. Environ Pollut. 237, 460–467. doi:10.1016/j.envpol.2018.02.050
Lu, Y., Zhang, Y., Deng, Y., Jiang, W., Zhao, Y., Geng, J., et al. (2016). Uptake and Accumulation of Polystyrene Microplastics in Zebrafish (Danio rerio) and Toxic Effects in Liver. Environ. Sci. Technol. 50, 4054–4060. doi:10.1021/acs.est.6b00183
Ma, J., Sheng, G. D., Chen, Q.-L., and O’Connor, P. (2020). Do combined Nanoscale Polystyrene and Tetracycline Impact on the Incidence of Resistance Genes and Microbial Community Disturbance in Enchytraeus Crypticus. J. Hazard. Mater. 387, 122012. doi:10.1016/j.jhazmat.2019.122012
Mao, R., Lang, M., Yu, X., Wu, R., Yang, X., and Guo, X. (2020). Aging Mechanism of Microplastics with UV Irradiation and its Effects on the Adsorption of Heavy Metals. J. Hazard. Mater. 393, 122515. doi:10.1016/j.jhazmat.2020.122515
Meng, X., Wu, S., Hu, W., Zhu, Z., Yang, G., Zhang, Y., et al. (2021). Clostridium Butyricum Improves Immune Responses and Remodels the Intestinal Microbiota of Common Carp (Cyprinus carpio L.). Aquaculture 530, 735753. doi:10.1016/j.aquaculture.2020.735753
Morgana, S., Ghigliotti, L., Estévez-Calvar, N., Stifanese, R., Wieckzorek, A., Doyle, T., et al. (2018). Microplastics in the Arctic: A Case Study with Sub-surface Water and Fish Samples off Northeast Greenland. Environ. Pollut. 242, 1078–1086. doi:10.1016/j.envpol.2018.08.001
Neamat-Allah, A. N. F., Mahmoud, E. A., and Abd El Hakim, Y. (2019). Efficacy of Dietary Nano-Selenium on Growth, Immune Response, Antioxidant, Transcriptomic Profile and Resistance of Nile tilapia, Oreochromis niloticus against Streptococcus Iniae Infection. Fish Shellfish Immunol. 94, 280–287. doi:10.1016/j.fsi.2019.09.019
Noor-Ul, H., Haokun, L., Junyan, J., Xiaoming, Z., Dong, H., Yunxia, Y., et al. (2020). Dietary Supplementation of Geotrichum Candidum Improves Growth, Gut Microbiota, Immune-Related Gene Expression and Disease Resistance in Gibel Carp CAS Ⅲ (Carassius auratus Gibelio). Fish Shellfish Immunol. 99, 144–153. doi:10.1016/j.fsi.2020.02.001
Nunes, B., Antunes, S. C., Gomes, R., Campos, J. C., Braga, M. R., Ramos, A. S., et al. (2015). Acute Effects of Tetracycline Exposure in the Freshwater Fish Gambusia holbrooki: Antioxidant Effects, Neurotoxicity and Histological Alterations. Arch. Environ. Contam. Toxicol. 68, 371–381. doi:10.1007/s00244-014-0101-z
Plastics Europe (2020). Available at: https://plasticseurope.org/knowledge-hub/plastics-the-facts-2020/
Rist, S., Baun, A., and Hartmann, N. B. (2017). Ingestion of Micro- and Nanoplastics in Daphnia magna - Quantification of Body Burdens and Assessment of Feeding Rates and Reproduction. Environ. Pollut. 228, 398–407. doi:10.1016/j.envpol.2017.05.048
Sarijan, S., Azman, S., Said, M. I. M., and Jamal, M. H. (2021). Microplastics in Freshwater Ecosystems: a Recent Review of Occurrence, Analysis, Potential Impacts, and Research Needs. Environ. Sci. Pollut. Res. 28, 1341–1356. doi:10.1007/s11356-020-11171-7
Scaria, J., Anupama, K. V., and Nidheesh, P. V. (2021). Tetracyclines in the Environment: An Overview on the Occurrence, Fate, Toxicity, Detection, Removal Methods, and Sludge Management. Sci. Total Environ. 771, 145291. doi:10.1016/j.scitotenv.2021.145291
Shen, X.-C., Li, D.-C., Sima, X.-F., Cheng, H.-Y., and Jiang, H. (2018). The Effects of Environmental Conditions on the Enrichment of Antibiotics on Microplastics in Simulated Natural Water Column. Environ. Res. 166, 377–383. doi:10.1016/j.envres.2018.06.034
Thompson, R. C., Olsen, Y., Mitchell, R. P., Davis, A., Rowland, S. J., and John, A. W.G. (2004). Lost at Sea: Where is all the Plastic?. Science 304 (5672), 838. doi:10.1126/science.1094559
Tourinho, P. S., Kočí, V., Loureiro, S., and van Gestel, C. A. M. (2019). Partitioning of Chemical Contaminants to Microplastics: Sorption Mechanisms, Environmental Distribution and Effects on Toxicity and Bioaccumulation. Environ. Pollut. 252, 1246–1256. doi:10.1016/j.envpol.2019.06.030
Trevisan, R., Voy, C., Chen, S., and Di Giulio, R. T. (2019). Nanoplastics Decrease the Toxicity of a Complex PAH Mixture but Impair Mitochondrial Energy Production in Developing Zebrafish. Environ. Sci. Technol. 53, 8405–8415. doi:10.1021/acs.est.9b02003
Van Cauwenberghe, L., Claessens, M., Vandegehuchte, M. B., and Janssen, C. R. (2015). Microplastics Are Taken up by Mussels (Mytilus edulis) and Lugworms (Arenicola marina) Living in Natural Habitats. Environ. Pollut. 199, 10–17. doi:10.1016/j.envpol.2015.01.008
Van Cauwenberghe, L., and Janssen, C. R. (2014). Microplastics in Bivalves Cultured for Human Consumption. Environ. Pollut. 193, 65–70. doi:10.1016/j.envpol.2014.06.010
Wan, T., Lu, S., Cheng, W., Ren, J., Wang, M., Hu, B., et al. (2019). A Spectroscopic and Theoretical Investigation of Interaction Mechanisms of Tetracycline and Polystyrene Nanospheres under Different Conditions. Environ. Pollut. 249, 398–405. doi:10.1016/j.envpol.2019.03.049
Wang, J., Li, Y., Lu, L., Zheng, M., Zhang, X., Tian, H., et al. (2019). Polystyrene Microplastics Cause Tissue Damages, Sex-specific Reproductive Disruption and Transgenerational Effects in marine Medaka (Oryzias Melastigma). Environ. Pollut. 254, 113024. doi:10.1016/j.envpol.2019.113024
Wang, K., Li, J., Zhao, L., Mu, X., Wang, C., Wang, M., et al. (2021). Gut Microbiota Protects Honey Bees (Apis mellifera L.) against Polystyrene Microplastics Exposure Risks. J. Hazard. Mater. 402, 123828. doi:10.1016/j.jhazmat.2020.123828
Wang, W., Ge, J., Yu, X., and Li, H. (2020). Environmental Fate and Impacts of Microplastics in Soil Ecosystems: Progress and Perspective. Sci. Total Environ. 708, 134841. doi:10.1016/j.scitotenv.2019.134841
Wright, S. L., Ulke, J., Font, A., Chan, K. L. A., and Kelly, F. J. (2020). Atmospheric Microplastic Deposition in an Urban Environment and an Evaluation of Transport. Environ. Int. 136, 105411. doi:10.1016/j.envint.2019.105411
Xie, Z., Lu, G., Hou, K., Qin, D., Yan, Z., and Chen, W. (2016). Bioconcentration, Metabolism and Effects of Diphenhydramine on Behavioral and Biochemical Markers in Crucian Carp (Carassius auratus). Sci. Total Environ. 544, 400–409. doi:10.1016/j.scitotenv.2015.11.132
Xu, K., Wang, J., Gong, H., Li, Y., Zhou, L., and Yan, M. (2019). Occurrence of Antibiotics and Their Associations with Antibiotic Resistance Genes and Bacterial Communities in Guangdong Coastal Areas. Ecotoxicology Environ. Saf. 186, 109796. doi:10.1016/j.ecoenv.2019.109796
Xu, N., Li, M., Chou, W.-C., and Lin, Z. (2020). A Physiologically Based Pharmacokinetic Model of Doxycycline for Predicting Tissue Residues and Withdrawal Intervals in Grass Carp (Ctenopharyngodon Idella). Food Chem. Toxicol. 137, 111127. doi:10.1016/j.fct.2020.111127
Yan, X., Zhang, Y., Lu, Y., He, L., Qu, J., Zhou, C., et al. (2020). The Complex Toxicity of Tetracycline with Polystyrene Spheres on Gastric Cancer Cells. Ijerph 17, 2808. doi:10.3390/ijerph17082808
Yang, C., Song, G., and Lim, W. (2020). A review of the Toxicity in Fish Exposed to Antibiotics. Comp. Biochem. Physiol. C. Toxicol. Pharmacol. 237, 108840. doi:10.1016/j.cbpc.2020.108840
Yang, X., Wei, H., Qin, L., Zhang, S., Wang, X., Zhang, A., et al. (2014). Reciprocal Interaction between Fish TGF-Β1 and IL-1β Is Responsible for Restraining IL-1β Signaling Activity in Grass Carp Head Kidney Leukocytes. Dev. Comp. Immunol. 47, 197–204. doi:10.1016/j.dci.2014.07.023
Yonar, M. E. (2012). The Effect of Lycopene on Oxytetracycline-Induced Oxidative Stress and Immunosuppression in Rainbow trout (Oncorhynchus mykiss, W.). Fish Shellfish Immunol. 32, 994–1001. doi:10.1016/j.fsi.2012.02.012
Yu, F., Yang, C., Huang, G., Zhou, T., Zhao, Y., and Ma, J. (2020). Interfacial Interaction between Diverse Microplastics and Tetracycline by Adsorption in an Aqueous Solution. Sci. Total Environ. 721, 137729. doi:10.1016/j.scitotenv.2020.137729
Yu, X., Wu, Y., Deng, M., Liu, Y., Wang, S., He, X., et al. (2019). Tetracycline Antibiotics as PI3K Inhibitors in the Nrf2-Mediated Regulation of Antioxidative Stress in Zebrafish Larvae. Chemosphere 226, 696–703. doi:10.1016/j.chemosphere.2019.04.001
Zhang, C., Wang, J., Zhou, A., Ye, Q., Feng, Y., Wang, Z., et al. (2021). Species-specific Effect of Microplastics on Fish Embryos and Observation of Toxicity Kinetics in Larvae. J. Hazard. Mater. 403, 123948. doi:10.1016/j.jhazmat.2020.123948
Zhang, C., Wang, S., Sun, D., Pan, Z., Zhou, A., Xie, S., et al. (2020a). Microplastic Pollution in Surface Water from East Coastal Areas of Guangdong, South China and Preliminary Study on Microplastics Biomonitoring Using Two marine Fish. Chemosphere 256, 127202. doi:10.1016/j.chemosphere.2020.127202
Zhang, S., Ding, J., Razanajatovo, R. M., Jiang, H., Zou, H., and Zhu, W. (2019). Interactive Effects of Polystyrene Microplastics and Roxithromycin on Bioaccumulation and Biochemical Status in the Freshwater Fish Red tilapia (Oreochromis niloticus). Sci. Total Environ. 648, 1431–1439. doi:10.1016/j.scitotenv.2018.08.266
Zhang, Y., Kang, S., Allen, S., Allen, D., Gao, T., and Sillanpää, M. (2020b). Atmospheric Microplastics: A Review on Current Status and Perspectives. Earth-Science Rev. 203, 103118. doi:10.1016/j.earscirev.2020.103118
Zhao, P., Cui, L., Zhao, W., Tian, Y., Li, M., Wang, Y., et al. (2021). Cotransport and Deposition of Colloidal Polystyrene Microplastic Particles and Tetracycline in Porous media: The Impact of Ionic Strength and Cationic Types. Sci. Total Environ. 753, 142064. doi:10.1016/j.scitotenv.2020.142064
Zhou, A., Zhang, Y., Xie, S., Chen, Y., Li, X., Wang, J., et al. (2021). Microplastics and Their Potential Effects on the Aquaculture Systems: a Critical Review. Rev. Aquacult. 13, 719–733. doi:10.1111/raq.12496
Zhou, R., Lu, G., Yan, Z., Jiang, R., Bao, X., and Lu, P. (2020). A Review of the Influences of Microplastics on Toxicity and Transgenerational Effects of Pharmaceutical and Personal Care Products in Aquatic Environment. Sci. Total Environ. 732, 139222. doi:10.1016/j.scitotenv.2020.139222
Keywords: microplastics, tetracycline, Jian carp, bioaccumulation, antioxidant response
Citation: Zhang C, Wang Q, Zuo Z, Ding J, Xu G and Zou J (2021) Interactive Effects of Microplastics and Tetracycline on Bioaccumulation and Biochemical Status in Jian Carp (Cyprinus carpio var. Jian). Front. Environ. Sci. 9:764344. doi: 10.3389/fenvs.2021.764344
Received: 25 August 2021; Accepted: 11 October 2021;
Published: 25 November 2021.
Edited by:
Anita Mukherjee, University of Calcutta, IndiaCopyright © 2021 Zhang, Wang, Zuo, Ding, Xu and Zou. This is an open-access article distributed under the terms of the Creative Commons Attribution License (CC BY). The use, distribution or reproduction in other forums is permitted, provided the original author(s) and the copyright owner(s) are credited and that the original publication in this journal is cited, in accordance with accepted academic practice. No use, distribution or reproduction is permitted which does not comply with these terms.
*Correspondence: Guohuan Xu, d3N3c2xAZ2lkbS5jbg==; Jixing Zou, em91aml4aW5nQHNjYXUuZWR1LmNu
†These authors have contributed equally to this work
Disclaimer: All claims expressed in this article are solely those of the authors and do not necessarily represent those of their affiliated organizations, or those of the publisher, the editors and the reviewers. Any product that may be evaluated in this article or claim that may be made by its manufacturer is not guaranteed or endorsed by the publisher.
Research integrity at Frontiers
Learn more about the work of our research integrity team to safeguard the quality of each article we publish.