- 1Beijing Key Lab for Source Control Technology of Water Pollution, College of Environmental Sciences and Engineering, Beijing Forestry University, Beijing, China
- 2Appraisal Center for Environment and Engineering, Ministry of Ecology and Environment, Beijing, China
- 3State Key Laboratory of Environmental Criteria and Risk Assessment, Chinese Research Academy of Environmental Sciences, Beijing, China
Study of the occurrence and fate of thyroid hormones in aquatic environments requires a highly sensitive method that is capable of detecting such compounds at sub-ng/L concentrations. By using isotope-dilution UPLC-MS/MS, we developed a sensitive method for quantifying thyroxine (T4), 3,3ʹ,5-triiodothyronine (T3), 3,3ʹ,5ʹ-triiodothyronine (rT3), iodotyrosine (MIT), and 3,5-diiodotyrosine (DIT) in lake water and sediment samples. MIT and DIT were first reported in aquatic environments in this study and were detected in all sediment and water samples with concentrations of 0.01–1.1 ng/g dw and 1.9–9.9 ng/L, respectively. T4 was only observed in sediment, with the measured concentrations and frequency of <LOQ-0.07 ng/L and 87%, respectively. T3 and rT3 were not found in this study. It was observed that the MIT/DIT ratios in sediment (2.4 ± 0.92) were significantly higher than those in water (0.84 ± 0.18), and the MIT/DIT ratios significantly correlated with DOC values in water samples, indicating that sorption to organic C could be of important mechanism. In addition, aquaculture, livestock and poultry farms probably were the important sources for the pollution of MIT, DIT, and T4 in Taihu Lake.
Introduction
The presence of endocrine disrupting compounds (EDCs) in the environment has attracted increasing attention due to their potential hazards (Crisp et al., 1998; Chang et al., 2007; 2009; Shen et al., 2018; 2020). So far most of work has focused on the disruption of chemicals with the estrogen or androgen systems (Zhou et al., 2016). However, it has been reported unusual thyroid gland development and ratios of circulating thyroid hormones in wild animal populations (Colborn, 2002). Also, in recent years studies have reported thyroidal activities in environmental samples. For example, Jugan et al. (2009) reported thyroidal activities in rivers and wastewaters in France. Quesada-García et al. (2012) detected thyroidal activities in fish feed extracts. Valdehita et al. (2014) found thyroidal activities in broiler and poultry manure applied as agricultural amendment. All this evidences the necessity of studying the environmental occurrences and risks of chemicals interacting with thyroidal systems known as thyroid disrupting chemicals.
Thyroid hormones including thyroxine (T4) and 3,3ʹ,5-triiodothyronine (T3) exist in all vertebrates and play a critical role in a wide variety of physiological functions such as embryonic development, cell differentiation, metabolism, and the regulation of cell proliferation (Yamanaka et al., 2007). Several studies have reported the potential ecotoxicological effects of these compounds on fish. It has been reported that the addition of T3/T4 to feed in aquaculture could cause the abnormal bone development for larva fish, such as the caudal fin and skull abnormalities (Faustino and Power, 1998; 1999; 2001). For amphibians, the morphological changes are directly regulated by thyroid hormones. It is noteworthy that there is the absence of endogenous circulating thyroid hormones before the morphological changes, and thus environmental exposure of T4/T3 may induce the early occurrence of metamorphosis (Tata, 2006; Brown and Cai, 2007; Maher et al., 2016).
T4 and T3 are secreted about 90 and 8 µg/day, respectively, in normal euthyroid individuals (Gnocchi et al., 2016). Different formulations of medicine T4 are also widely applied for hypothyroidism with non-trivial amounts worldwide (Ledeţi et al., 2016). In the period of 2007–2011, the aggregate sum of the various brands of T4 is the third most prescribed pharmaceutical in Canada (Rotermann et al., 2015). Thus, both natural T4/T3 and synthetic medicines contribute to the occurrence of T4/T3 in the environment. Besides T4 and T3, their precursor compounds, e.g., iodotyrosine (MIT) and 3,5-diiodotyrosine (DIT), are necessary to be simultaneously quantified. MIT and DIT are also naturally secreted by humans and animals and enter the receiving waters along with T4 and T3. In addition, MIT and DIT are the characteristic hydrolysis products of cascoiodine (Wang et al., 2008), which is widely used in aquaculture and livestock and poultry breeding to regulate growth and development of animals. MIT and DIT are recently discovered to be the substrates of a specific iodotyrosine dehalogenase enzyme (DEHAL1), and that DEHAL1 deficiency induces iodine wasting. Several genetic defects of this enzyme, as well as its inhibition by xenobiotics, are known to be associated with primary hypothyroidism (Moreno et al., 2008). MIT and DIT assay is under consideration as a clinical test in neonatal screening and/or in the evaluation of potential endocrine disruptors (Borsò et al., 2020). These observations raised interest in the quantitative detection of the wider spectrum of thyroid hormones (including MIT and DIT) in serum, tissues and various environmental matrices. Furthermore, T4 is generated by coupling two DIT residues while T3 is the generated by coupling one DIT with one MIT in organisms. Some phenolic pollutants such as chlorophenols and estradiol were reported to produce cross-coupled or polymerization products in both organisms and the environment (Pang et al., 2014; Ashrap et al., 2017; Zhong et al., 2019). It is possible that MIT and DIT are combined to generate T4 or T3 in the environment, and further research is necessary.
In this study, we developed an isotope-dilution UPLC-MS/MS method that allows the simultaneous analysis of MIT, DIT, T4, T3 and its isomer its isomer 3,3ʹ,5ʹ-triiodothyronine (rT3) (Figure 1). We then applied this method to investigate the occurrence of each thyroid hormones in sediment and water samples from Taihu Lake of China. This study provided the first analytical method that included MIT and DIT in lake water and sediment samples and provided the first evidence for the occurrence of MIT and DIT in aquatic environments.
Materials and Methods
Chemicals and Reagents
MIT (purity >95%), DIT (purity >95%), rT3 (purity >95%), T4 (purity >95%), 13C6-MIT (purity >98%), 13C6-T3 (purity >98%), and 13C6-T4 (purity >98%) were obtained from Toronto Research Chemicals (Downsview, ON, Canada). T3 (purity >97%) was obtained from Sigma Aldrich (Oakville, ON, Canada). LC-MS grade methanol and ethyl acetate (EtOAc) were obtained from Merck (Darmstadt, Germany). Ultrapure water was produced through the Milli-Q purification system (Millipore, Billerica, United States) at conductivity of 18.2 Ω·cm−1. Sodium hydroxide and hydrochloric acid were purchased from Xilong Scientific Co., Ltd. (Chengdu, China).
Sample Collection
Thirty pairs of sediment and water samples were collected in October 2019 from Taihu Lake of China. The sampling sites are shown in Figure 2. Sediment samples (upper 20–30 cm) were collected at the lake bottom with a stainless-steel grab sampler, and water samples (upper 50 cm) were collected with a stainless-steel vertical sampler. All water samples were collected in precleaned 4-L amber glass bottles. The samples were sealed without a headspace and transported to the laboratory within 6 h of collection. Water samples were filtered and extracted immediately, and sediment samples were stored at −20°C until further analyses.
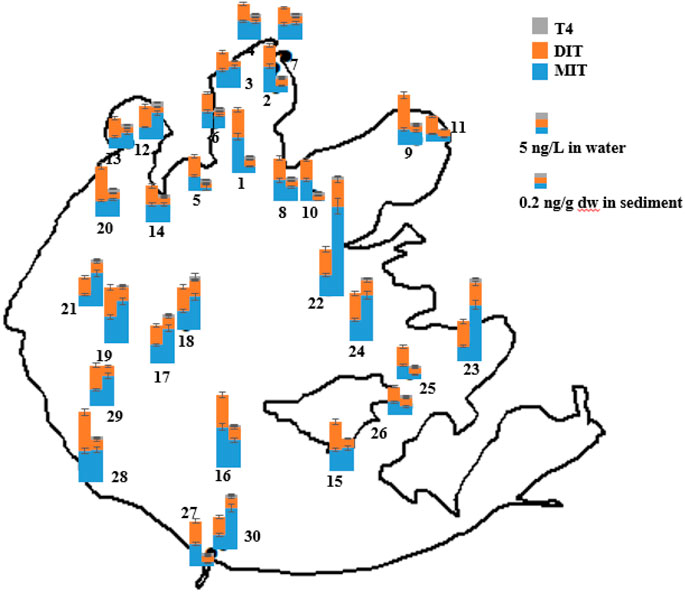
FIGURE 2. Occurrence and distribution of the thyroid hormones detected in 30 pairs of water (left) and sediment samples (right) from Taihu Lake.
Sample Preparation
Glass fiber pads (GF/F, 0.7 μm, Whatman, Maidstone, United Kingdom) were used for filtering water samples to remove suspended materials. The target thyroid hormones were extracted from water samples with Waters Oasis HLB extraction cartridges (500 mg, 6 cc). Prior to extraction, isotope-labelled standards (13C6-MIT, 13C6-T3, and 13C6-T4) were added into water samples at concentrations of 100 ng/L as surrogates to compensate for losses and matrix effects, and then water samples were acidified to pH∼2.5. The HLB cartridges were preconditioned with 6 ml of EtOAc, 6 ml of methanol, and 6 ml of distilled water (pH 2.5), and then water samples were loaded at a flow rate of 5–10 ml/min. After rinsed with 10 ml of acidified water, the cartridges were dried and eluted with 6 ml of EtOAc. The extracts were evaporated under a gentle stream of nitrogen to dryness and reconstituted with 200 μl of methanol for UPLC-ESI-MS/MS analysis.
For sediment samples, they were freeze-dried and grinded to pass through 100 mesh sieve. Prior to extraction, sediment samples were spiked with 20 ng of each surrogates (13C6-MIT, 13C6-T3, and 13C6-T4) and were ultrasonically extracted with 20 ml of mixture of methanol and EtOAc (1:1, v:v). The eluent was evaporated to dryness and reconstituted with 200 ml of distilled water. The subsequent purified steps followed the same procedure as for the water samples described above.
UPLC-MS/MS Analysis
The LC apparatus was an ACQUITY Ultra Performance LC system (Waters, Milford, MA, United States). Separation was carried out on a Waters BEH C18 column (100 mm × 2.1 mm, 1.7 μm particle size). The column was maintained at 35°C, and the flow rate and injection volume were 0.3 ml/min and 2 μl, respectively. Aqueous (0.1%) acetic acid (A) and 0.1% acetic acid in methanol (B) were used as the mobile phases. The initial gradient of 10% B was held for 3 min, and then increased to 50% at 6 min, 90% at 10 min, and then to 100% at 10.5 min. After that the column was washed with 100% B for 2 min, then the column was re-equilibrated with the initial mobile phase composition for 4 min before the next injection.
Mass spectrometry was performed using a Waters Xevo TQ-XS Triple Quadrupole Mass Spectrometer equipped with an electrospray ionization source in positive ion mode. A flow injection of a standard solution of each compound was used to find the optimum conditions in the ESI source and the average parameters selected were: source temperature 150°C; desolvation temperature 500°C; capillary voltage 3.2 kV; desolvation gas flow 1000 L/h and cone gas flow 150 L/h. Quantitative analysis of the target thyroid hormones was performed in multi-selected reaction monitoring (MRM). In the MRM transitions, the dwell times were automatically selected. Data acquisition was performed with MassLynx 4.2 (Micromass, Manchester, United Kingdom). The MRM transitions, cone and collision energy of target thyroid hormones were listed in Table 1.
Results and Discussion
Analytical Procedure and Method Performance
The five thyroid hormones were simultaneously analyzed by MS/MS in the MRM mode. The two abundant MRM transitions, cone voltage and collision energies, were optimized for each analyte by infusing the standard solutions into the mass spectrometer (Table 1). All of the precursor ions were protonated molecular ions [(M + H)+]. Under MS/MS conditions, the protonated molecular ions lost water and carbon monoxide, corresponding to a mass loss of 46 Da, yielding [M + H-H2O-CO]+ ions for all target thyroid hormones. At high collision energy, T4, T3 and rT3 showed losses of 127 Da, corresponding to the loss of HI. No loss of HI was observed in the spectrum of MIT and DIT, suggesting that the losses of HI in T4, T3 and rT3 spectrums were more likely to occur on the phenolic rings. For MIT, the other abundant product ion was the result of loss of water from the protonated molecule at low collision energy. In the case of DIT, the other abundant ion at m/z 289.9 was produced by the cleavage of benzene ring. Chromatographic separation was performed on a BEH C18 column. Methanol and the addition of acetic acid in the mobile phases increased the retention of target thyroid hormones to the column, improving the chromatographic behavior of the analytes (Figure 3).
This study used the isotope dilution method for quantifying the target analytes. The use of such method allowed to correct the losses of analytes during sample pretreatment, and to compensate for matrix effects from different samples and variations in instrumental signals between injections. The recovery experiments were conducted using pooled water and sediment samples from Taihu Lake. Analyte addition was made with the criterion of at least three times the original concentration that was determined prior to the fortification experiment. As shown in Table 2, the recoveries of all target analytes were 84–102% in sediment and 87–101% in water. The relative standard deviation (RSD) values were less than 19% in all of the spiked samples. The extent of signal suppression and enhancement in the UPLC-ESI/MS/MS detection was evaluated by spiking extracts of the pooled water and sediment samples with a standard solution. The matrix effect observed with each analyte was calculated using the percentage signal intensity in the sample matrix versus the signal for the same concentration in methanol. The response ratios for all target analytes in pooled water and sediment samples were 25–30% and 40–51%, respectively (Table 2). Based on a signal-to-noise ratio of 10, the limit of quantifications (LOQs) for target thyroid hormones in water and sediment samples were 0.08–0.1 ng/L and 0.008–0.01 ng/g dw, respectively. To our knowledge, only one study has previously reported the detection of T4 and T3 in surface water, and the LOQs of T4 (0.6 ng/L), T3 (0.9 ng/L) and rT3 (3.2 ng/L) (Svanfelt et al., 2010) are lower than the present study.

TABLE 2. Recoveries (%), matrix effects and limits of quantitation (LOQ) of the target thyroid hormones from sediment and water samples (n = 5).
Concentrations of Target Thyroid Hormones
As shown in Figure 2 and Table 3, MIT and DIT were detected in all sediment and water samples. MIT was detected with 0.01–1.1 ng/g dw in sediment and 1.9–9.9 ng/L in water, and DIT was 0.06–0.33 ng/g dw and 4.1–9.6 ng/L, respectively. T4 was only observed in sediment, with the measured concentrations and frequency of <LOQ-0.07 ng/L and 87%, respectively. T3 and rT3 were not found in this study. To our knowledge, the present study is the first one that reports such a contamination of surface water by MIT and DIT which are important for sustaining thyroid hormone synthesis in organisms and humans. One study reported 0.8–1.7 ng/ml of urinary MIT and DIT in euthyroid people, but much higher of MIT (316 ng/ml) and DIT (129 ng/ml) were measured in DEHAL1 deficient humans. It has been reported that some algae species can form MIT and DIT which are probably bound to proteins (Shah et al., 2005). Thus, in addition to human/animal excretion, algae may be a potential source for MIT and DIT contamination. It requires further research on whether algae species from Taihu Lake are able to metabolize MIT and DIT. Besides, MIT and DIT are the characteristic hydrolysis products of cascoiodine (Wang et al., 2008), which is widely used in aquaculture and livestock and poultry breeding to regulate the growth and development of animals (Yu, 2004), and thus the addition of cascoiodine in the feed maybe another source of MIT and DIT found in Taihu Lake. In the present study, T4 was found in sediment but not water. This is on one hand due to the different sediment/water partition [the log Kow of T4: 4.12 (Li et al., 2014)]. On the other hand, combination of T4 with protein compounds would probably make it prone to sorption onto sediment. It has been reported that the mean daily urinary excretion of free T4 and T3 by healthy humans are 1.4 and 0.63 μg (Faber et al., 1981), respectively, while that for fecal T4 (probably in the sorbed form to proteins) amounts to 20 μg (Svanfelt et al., 2010). Protein compounds not only exist in organisms but also are ubiquitous in aquatic environment. Xia et al. (2013) reported that both bovine albumin from animal and soy peptone from plant could combine with perfluoroalkyl substances and affect their bioaccumulation by Daphnia magna in water. Thus, the protein compounds in sediment from Taihu Lake possibly help T4 sorb onto sediment, which needs further researches. T3 is naturally less produced compared with T4 (Faber et al., 1981). T4 is generated by coupling two DIT residues while T3 is generated by coupling one DIT with one MIT in organisms. But thyroid peroxidase is much more efficient at combining of two DIT residues and thus generation of T4 occurs much more readily, explaining why T4 excretion is much higher than that of T3. Also, T4 is a widely used medicine with more than 400 different formulations worldwide, but synthetic T3 is relatively less applied (Brown and Wong, 2017). These can partly explain the “non-detection” of T3 in this study.
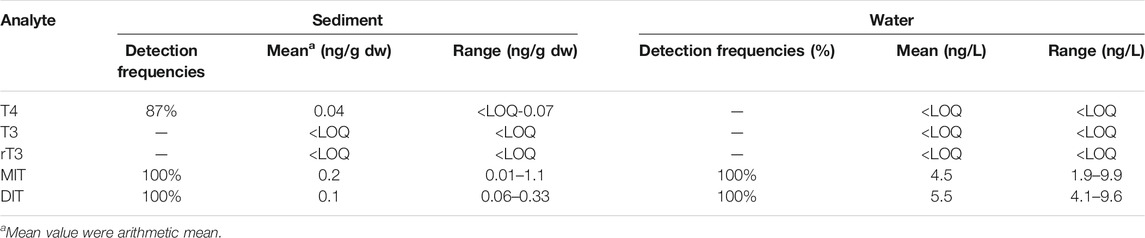
TABLE 3. Detection frequencies and concentrations of thyroid hormones in water and sediment samples from Taihu Lake.
The concentration ratios of MIT/DIT in water and sediment samples from Taihu Lake are given in Figure 4. It is interesting to find that the MIT/DIT ratios in sediment (2.4 ± 0.92) is significantly higher than those in water (0.84 ± 0.18) (p < 0.001), and the different sorption behavior between them should be the major reason. The pH, clay content, and organic C content in the environment can affect their sorption onto suspended particles and sediment. In this study, MIT and DIT would be in neutral or anionic forms in these samples at the measured pH values of 7.59–9.26, indicating that sorption to organic C could be the dominant sorption mechanism. Similar phenomenon has been reported for the sorption of hydrophilic sulfonamide antibiotics in freshwater-sediment microcosms (Carstens et al., 2013). Furthermore, as one of important components of organic C, protein compounds readily combined with DIT compared to MIT, which possibly increased sorption and binding of DIT residues to sediment by producing nonextractable sediment-bound residue or transformation products (such as T4). Figure 5 showed a significant correlation between the MIT/DIT ratios and DOC values in water samples (R2 = 0.3684, p < 0.001), also indicating that sorption to organic C could be of important mechanism for their sorption to the solid phases.
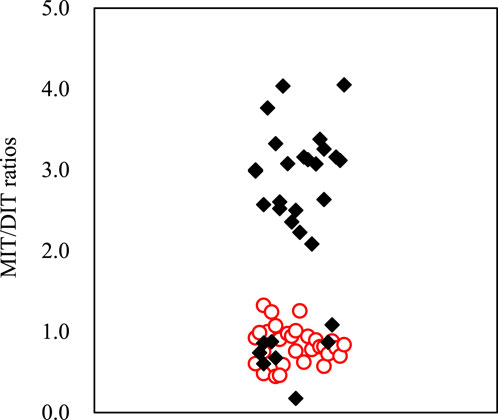
FIGURE 4. Concentration ratio of MIT and DIT in water (red circle) and sediment samples (black diamond shape) from Taihu Lake.
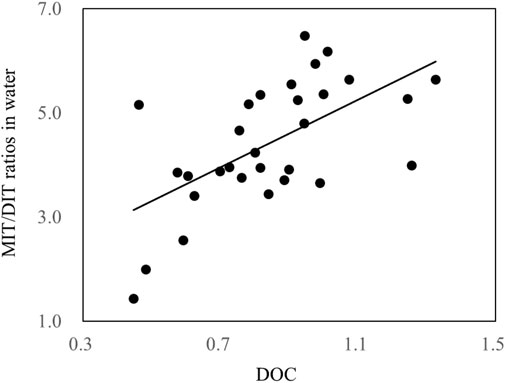
FIGURE 5. Correlations between the concentration ratios of MIT and DIT and DOC values (mg/L) in water samples of Taihu Lake in 2019.
As shown in Figure 2, the total concentrations of detected thyroid hormones were 6.2–18 ng/L in water and 0.15–1.5 ng/g dw in sediment. The total concentrations of detected thyroid hormones in the eastern area (sites 22, 23 and 24) were relatively higher than those in other areas of Taihu Lake. This was probably due to the usage of cascoiodine in the feedstuff in the aquaculture and livestock and poultry breeding in the eastern area which used to have lots of crab, fish, livestock and poultry farms. Cascoiodine is widely used as synthetic thyroxine additives to regulate the growth and development of animals (Yu, 2004), and it can be hydrolyzed into MIT, DIT, and T4 under alkaline conditions (Wang et al., 2007). In comparison, relatively low concentrations of thyroid hormones were found in the northwestern area of Taihu Lake (including Zhushan Bay, Meiliang Bay, and Gonghu Bay) where relatively heavy contamination has been reported (Zhao et al., 2019). Thus, MIT and DIT may be a possible indicator of wastewater input from aquaculture and livestock and poultry breeding, and deserve more future study.
Conclusion
We developed a sensitive method for monitoring MIT, DIT, rT3, T3, and T4 in sediment and water using UPLC-MS/MS, and then 30 pairs of water and sediment samples were analyzed to reveal their occurrence and distributions in Taihu Lake. MIT and DIT, which are important for sustaining thyroid hormone synthesis in organisms and humans, were first reported in surface water. T4 was only observed in sediment. T3 and rT3 were not found in this study. Aquaculture, livestock and poultry farms probably were the important sources for the ubiquitous pollution of MIT, DIT, and T4. Sorption to organic C could be of important mechanism based on the observations of higher MIT/DIT ratios in sediment than in water and a significant correlation between the MIT/DIT ratios and DOC values in water samples.
Data Availability Statement
The original contributions presented in the study are included in the article/Supplementary Material, further inquiries can be directed to the corresponding authors.
Author Contributions
HC was involved in writing the manuscript with the help of SZ, JZ, and FS. JZ and FS helped in field sample collection and reviewing the manuscript. SZ was involved in method development and data analysis. All authors read and approved the final manuscript.
Funding
The authors are grateful for financial support by the Major Science and Technology Program for Water Pollution Control and Treatment (2018ZX07208001), the National Natural Science Foundation of China (42077306), and the Fundamental Research Funds for the Central Universities (JC 2015-02). The authors wish to acknowledge the support of a grant from the State Key Laboratory of Environmental Criteria and Risk Assessment (SKLECRA2019OFP07).
Conflict of Interest
The authors declare that the research was conducted in the absence of any commercial or financial relationships that could be construed as a potential conflict of interest.
Publisher’s Note
All claims expressed in this article are solely those of the authors and do not necessarily represent those of their affiliated organizations, or those of the publisher, the editors and the reviewers. Any product that may be evaluated in this article, or claim that may be made by its manufacturer, is not guaranteed or endorsed by the publisher.
Acknowledgments
The authors also thank the Beijing Municipal Education Commission for its financial support through Innovative Transdisciplinary Program “Ecological Restoration Engineering.”
References
Ashrap, P., Zheng, G., Wan, Y., Li, T., Hu, W., Li, W., et al. (2017). Discovery of a Widespread Metabolic Pathway Within and Among Phenolic Xenobiotics. Proc. Natl. Acad. Sci. USA. 114, 6062–6067. doi:10.1073/pnas.1700558114
Borsò, M., Agretti, P., Zucchi, R., and Saba, A. (2020). Mass Spectrometry in the Diagnosis of Thyroid Disease and in the Study of Thyroid Hormone Metabolism. Mass. Spectrom. Rev. 2020, 1–26. doi:10.1002/mas.21673
Brown, A. K., and Wong, C. S. (2017). Measurement of Thyroxine and its Glucuronide in Municipal Wastewater and Solids Using Weak Anion Exchange Solid Phase Extraction and Ultrahigh Performance Liquid Chromatography-Tandem Mass Spectrometry. J. Chromatogr. A. 1525, 71–78. doi:10.1016/j.chroma.2017.10.010
Brown, D. D., and Cai, L. (2007). Amphibian Metamorphosis. Developmental Biol. 306 (1), 20–33. doi:10.1016/j.ydbio.2007.03.021
Carstens, K. L., Gross, A. D., Moorman, T. B., and Coats, J. R. (2013). Sorption and Photodegradation Processes Govern Distribution and Fate of Sulfamethazine in Freshwater-Sediment Microcosms. Environ. Sci. Technol. 47 (19), 10877–10883. doi:10.1021/es402100g
Chang, H., Hu, J., and Shao, B. (2007). Occurrence of Natural and Synthetic Glucocorticoids in Sewage Treatment Plants and Receiving River Waters. Environ. Sci. Technol. 41, 3462–3468. doi:10.1021/es062746o
Chang, H., Wan, Y., and Hu, J. (2009). Determination and Source Apportionment of Five Classes of Steroid Hormones in Urban Rivers. Environ. Sci. Technol. 43, 7691–7698. doi:10.1021/es803653j
Colborn, T. (2002). Clues From Wildlife to Create an Assay for Thyroid System Disruption. Environ. Health Perspect. 110 Suppl 3 (Suppl. 3), 363–367. doi:10.1289/ehp.02110s3363
Crisp, T. M., Clegg, E. D., Cooper, R. L., Wood, W. P., Anderson, D. G., Baetcke, K. P., et al. (1998). Environmental Endocrine Disruption: an Effects Assessment and Analysis. Environ. Health Perspect. 106 Suppl 1, 11–56. doi:10.1289/ehp.98106s111
Faber, J., Busch-sørensen, M., Rogowski, P., Kirkegaard, C., Siersbæenielsen, K., and Friis, T. (1981). Urinary Excretion of Free and Conjugated 3′,5′-Diiodothyronine and 3,3′-Diiodothyronine. J. Clin. Endocrinol. Metab. 53 (3), 587–593. doi:10.1210/jcem-53-3-587
Faustino, M., and Power, D. M. (1998). Development of Osteological Structures in the Sea Bream: Vertebral Column and Caudal Fin Complex. J. Fish Biol. 52 (1), 11–22. doi:10.1111/j.1095-8649.1998.tb01548.x
Faustino, M., and Power, D. M. (1999). Development of the Pectoral, Pelvic, Dorsal and Anal Fins in Cultured Sea Bream. J. Fish Biol. 54 (5), 1094–1110. doi:10.1111/j.1095-8649.1999.tb00860.x
Faustino, M., and Power, D. M. (2001). Osteologic Development of the Viscerocranial Skeleton in Sea Bream: Alternative Ossification Strategies in Teleost Fish. J. Fish Biol. 58 (2), 537–572. doi:10.1111/j.1095-8649.2001.tb02272.x
Gnocchi, D., Steffensen, K. R., Bruscalupi, G., and Parini, P. (2016). Emerging Role of Thyroid Hormone Metabolites. Acta Physiol. 217 (3), 184–216. doi:10.1111/apha.12648
Jugan, M. L., Oziol, L., Oziol, L., Huteau, V., Tamisier-Karolak, S., Blondeau, J. P., et al. (2009). In Vitro assessment of Thyroid and Estrogenic Endocrine Disruptors in Wastewater Treatment Plants, Rivers and Drinking Water Supplies in the Greater Paris Area (France). Sci. Total Environ. 407 (11), 3579–3587. doi:10.1016/j.scitotenv.2009.01.027
Ledeţi, I., Ledeţi, A., Vlase, G., Vlase, T., Matusz, P., Bercean, V., et al. (2016). Thermal Stability of Synthetic Thyroid Hormone L-Thyroxine and L-Thyroxine Sodium Salt Hydrate Both Pure and in Pharmaceutical Formulations. J. Pharm. Biomed. Anal. 125, 33–40.
Li, P., Hu, B., He, M., and Chen, B. (2014). Ion Pair Hollow Fiber Liquid-Liquid-Liquid Microextraction Combined With Capillary Electrophoresis-Ultraviolet Detection for the Determination of Thyroid Hormones in Human Serum. J. Chromatogr. A. 1356, 23–31. doi:10.1016/j.chroma.2014.06.046
Maher, S. K., Wojnarowicz, P., Ichu, T.-A., Veldhoen, N., Lu, L., Lesperance, M., et al. (2016). Rethinking the Biological Relationships of the Thyroid Hormones, L-Thyroxine and 3,5,3′-Triiodothyronine. Comp. Biochem. Physiol. D: Genomics Proteomics. 18, 44–53. doi:10.1016/j.cbd.2016.04.002
Moreno, J. C., Klootwijk, W., van Toor, H., Pinto, G., D'Alessandro, M., Lèger, A., et al. (2008). Mutations in the Iodotyrosine Deiodinase Gene and Hypothyroidism. N. Engl. J. Med. 358, 1811–1818. doi:10.1056/nejmoa0706819
Pang, S.-Y., Jiang, J., Gao, Y., Zhou, Y., Huangfu, X., Liu, Y., et al. (2014). Oxidation of Flame Retardant Tetrabromobisphenol A by Aqueous Permanganate: Reaction Kinetics, Brominated Products, and Pathways. Environ. Sci. Technol. 48 (1), 615–623. doi:10.1021/es4041094
Quesada-García, A., Valdehita, A., Fernández-Cruz, M. L., Leal, E., Sánchez, E., Martín-Belinchón, M., et al. (2012). Assessment of Estrogenic and Thyrogenic Activities in Fish Feeds. Aquaculture. 338-341, 172–180. doi:10.1016/j.aquaculture.2012.02.010
Rotermann, M., Sanmartin, C., Hennessy, D., and Arthur, M. (2015). Prescription Medication Use by Canadians Aged 6 to 79. Health Rep. 25 (6), 3–9.
Shah, M., Wuilloud, R. G., Kannamkumarath, S. S., and Caruso, J. A. (2005). Iodine Speciation Studies in Commercially Available Seaweed by Coupling Different Chromatographic Techniques With UV and ICP-MS Detection. J. Anal. Spectrom. 20 (3), 176–182. doi:10.1039/b415756g
Shen, X., Chang, H., Sun, D., Wang, L., and Wu, F. (2018). Trace Analysis of 61 Natural and Synthetic Progestins in River Water and Sewage Effluents by Ultra-high Performance Liquid Chromatography-Tandem Mass Spectrometry. Water Res. 133, 142–152. doi:10.1016/j.watres.2018.01.030
Shen, X., Chang, H., Sun, Y., and Wan, Y. (2020). Determination and Occurrence of Natural and Synthetic Glucocorticoids in Surface Waters. Environ. Int. 134, 105278. doi:10.1016/j.envint.2019.105278
Svanfelt, J., Eriksson, J., and Kronberg, L. (2010). Analysis of Thyroid Hormones in Raw and Treated Waste Water. J. Chromatogr. A. 1217 (42), 6469–6474. doi:10.1016/j.chroma.2010.08.032
Tata, J. R. (2006). Amphibian Metamorphosis as a Model for the Developmental Actions of Thyroid Hormone. Mol. Cell Endocrinol. 246, 10–20. doi:10.1016/j.mce.2005.11.024
Valdehita, A., Quesada-García, A., Delgado, M. M., Martín, J. V., García-González, M. C., Fernández-Cruz, M. L., et al. (2014). In Vitro Assessment of Thyroidal and Estrogenic Activities in Poultry and Broiler Manure. Sci. Total Environ. 472, 630–641. doi:10.1016/j.scitotenv.2013.11.098
Wang, Z. Y., Li, D. F., Zhou, Z. Q., Zhang, L. Y., Li, B. Y., Yang, W. J., et al. (2007). Determination of MIT, DIT and T4 in Cascoiodine by NaOH Hydrolysis by HPLC. Feed Res. 12, 21–23. in Chinese. doi:10.3969/j.issn.1002-2813.2007.12.006
Wang, Z. Y., Yang, W. J., Zhang, L. Y., and He, P. L. (2008). Determination of DIT and MIT in Hydrolysate of Premixed Feed by Liquid Chromatography-Mass Spectrometry. J. Instrument Anal. 27, 227–230. in Chinese.
Xia, X., Rabearisoa, A. H., Rabearisoa, A. H., Jiang, X., and Dai, Z. (2013). Bioaccumulation of Perfluoroalkyl Substances byDaphnia Magnain Water With Different Types and Concentrations of Protein. Environ. Sci. Technol. 47 (19), 10955–10963. doi:10.1021/es401442y
Yamanaka, H., Nakajima, M., Katoh, M., and Yokoi, T. (2007). Glucuronidation of Thyroxine in Human Liver, Jejunum, and Kidney Microsomes. Drug Metab. Dispos. 35 (9), 1642–1648. in Chinese. doi:10.1124/dmd.107.016097
Yu, D. R. (2004). Mechanism of Action of Cascoiodine and its Application in Feed. Jiangxi Feed. 6, 17–19. doi:10.3969/j.issn.1008-6137.2004.06.007
Zhao, H., Liu, L., Li, Y., Zhao, F., Zhang, S., Mu, D., et al. (2019). Occurrence, Bioaccumulation, and Trophic Transfer of Oligomeric Organophosphorus Flame Retardants in an Aquatic Environment. Environ. Sci. Technol. Lett. 6, 323–328. doi:10.1021/acs.estlett.9b00262
Zhong, C., Zhao, H., Cao, H., and Huang, Q. (2019). Polymerization of Micropollutants in Natural Aquatic Environments: A Review. Sci. Total Environ. 693, 133751. doi:10.1016/j.scitotenv.2019.133751
Keywords: thyroid hormones, water, sediment, taihu lake, UPLC-MS/MS, occurrence, source
Citation: Zhang S, Zhang J, Chang H and Sun F (2021) Thyroid Hormones in Sediment and Water: Method Validation and Detection in Taihu Lake of China. Front. Environ. Sci. 9:733243. doi: 10.3389/fenvs.2021.733243
Received: 30 June 2021; Accepted: 12 August 2021;
Published: 24 August 2021.
Edited by:
Shuwen Yan, Fudan University, ChinaReviewed by:
Huajun Zhen, East China University of Science and Technology, ChinaShuying Li, Zhejiang University, China
Haifeng Zhang, Research Center for Eco-environmental Sciences (CAS), China
Copyright © 2021 Zhang, Zhang, Chang and Sun. This is an open-access article distributed under the terms of the Creative Commons Attribution License (CC BY). The use, distribution or reproduction in other forums is permitted, provided the original author(s) and the copyright owner(s) are credited and that the original publication in this journal is cited, in accordance with accepted academic practice. No use, distribution or reproduction is permitted which does not comply with these terms.
*Correspondence: Hong Chang, Y2hhbmdoQGJqZnUuZWR1LmNu; Fuhong Sun, c3VuZmhpYWVAMTI2LmNvbQ==