- 1Universidad Nacional de Río Negro, Instituto de Investigación en Paleobiología y Geología, General Roca, Argentina
- 2Consejo Nacional de Investigaciones Científicas y Técnicas (CONICET), Buenos Aires, Argentina
- 3Centro de Estudios Integrales de la Dinámica Exógena, Universidad Nacional de La Plata, La Plata, Argentina
- 4División Zoología Invertebrados, Museo de La Plata, Universidad Nacional de La Plata, La Plata, Argentina
The invasion of the Asiatic clam Corbicula fluminea in South America has already reached Patagonia. Despite the well-known direct and indirect impacts that this species exerts on fresh-water ecosystems, particularly on benthic communities, few studies have been undertaken on its effect on the composition and structure of aquatic macroinvertebrate assemblages. This study evaluated the effects of the invasion of the Asiatic clam C. fluminea on the aquatic macroinvertebrate assemblages of the Limay River, one of the most important rivers of the arid plateau of Argentinian Patagonia. As this river is currently in the process of being invaded, it was possible to compare invaded and non-invaded sites. Invaded sites displayed a certain homogenization of their constituent species and functional composition. Non-metric multidimensional scaling, similarity analysis and similarity percentages showed negative or positive influence of the presence of this species on the abundance of some taxa, due to competition for resources in this low-productivity Patagonian ecosystem. The deposition of the faeces and pseudo-faeces of C. fluminea enriches the organic content of the sediments. However, its pedal feeding can function as a competitive strategy: by consuming its own deposited organic matter when food is scarce, the amount available to other macroinvertebrates is reduced. This paper is the first contribution on the impact of this invasion on northern Patagonia’s aquatic macroinvertebrate assemblages.
Introduction
In the current context of globalization, biological invasions constitute one of the main threats to biodiversity (Bellard et al., 2016; Essl et al., 2020). Invasive bivalves cause biotic and structural changes that influence aquatic macroinvertebrate assemblages (Darrigran et al., 1998; Darrigran and Damborenea, 2011; Wittmann et al., 2012; Werner, 2014). The final impact of each invasion on these organisms is difficult to predict (Lodge, 1993). Invasive species can rapidly replace native taxa (Bachmann et al., 2001; Brown and Barney, 2021), or cause increases in the abundance of favored native taxa (Horvath et al., 1999; Mayer et al., 2002). The scale of the impact may also be related to the size of the invasive population, as well as the susceptibility or resistance of each environment to invasion (Hicks, 2004; Howeth, 2017). Established communities can even facilitate the invasion process of these species (Mörtl and Rothhaupt, 2003).
The invasive Asiatic clam Corbicula fluminea is the most widespread freshwater species in South America (Darrigran et al., 2020). This species causes several direct and indirect impacts on macroinvertebrate assemblages by displacement and/or reduction of available habitat (Vaughn and Hakenkamp, 2001) and upon death their durable valves provide refuge and substrate for other species (Strayer et al., 1999; Gutiérrez et al., 2003).
Assessing the impact of invasion on aquatic macroinvertebrate assemblages is important due to their crucial role in maintaining the structural and functional integrity of freshwater ecosystems (Wittmann et al., 2012; Zhang et al., 2014; Paschoal et al., 2015; Macchi et al., 2018). Macroinvertebrates regulate the geophysical condition of the sediments, participate in the decomposition of debris and the cycling of organic matter and nutrients, and regulate the primary producer communities (Pereira et al., 2012; Batzer and Boix, 2016; Zimmer et al., 2016; Boyero et al., 2021).
The filter-feeding of invasive bivalves can negatively affect the recruitment of some species (i.e., juvenile unionids and spherids) (Yeager et al., 1994; Hakenkamp and Palmer, 1999). In addition, collector-filterers compete for the food resources of other species (Sousa et al., 2005). Some of these effects have led to a decline in the abundance and diversity of native mollusks in freshwater ecosystems in America and Europe (Araujo et al., 1993, Williams et al., 1993; Strayer et al., 1999; Aldridge and Müller, 2001; McMahon, 2002; Sousa et al., 2005; Sousa et al., 2007; Sousa et al., 2008a; Sousa et al., 2014).
One of the more conspicuous effects of invasions is the reconfiguration of biodiversity at a coarse scale through biotic homogenization (loss of β diversity) (Olden et al., 2011), which is considered an overarching process that encompasses either the loss of taxonomic, genetic, or functional distinctiveness over time (Olden et al., 2004). Changes in diversity patterns within a region can occur as a change in species similarity between smaller areas, reducing differentiation diversity between regions. Thus, the establishment of the same invasive species at two separate localities will lead to increases in the similarity of the invaded communities (Olden et al., 2011).
This research aimed to determine if the presence of Corbicula fluminea causes macroinvertebrate assemblage homogenization, by affecting negatively or positively on resource availability through consumption, bioturbation or excretion. Thus, it is predicted that invaded sites will have similar species composition, with an increased density of favored species and a diminished density of vulnerable species.
Materials and Methods
Study Area
The study compares invaded and non-invaded sites in natural lotic and artificial lentic habitats along the Limay River and takes into account the environmental characteristics of each site. The sites are located in Argentina’s Patagonian plateau (Figure 1). Patagonia extends from 35° to 39°W and from 36° to 55°S, with the Colorado and Barrancas rivers at its northern limits. The dominant rock types are basalts (Drago and Quirós, 1995). Increasing aridity is seen along a strong east-west precipitation gradient; annual rainfall levels decrease to around 150 mm/year in the arid plateau region (800 m a.s.l.) causing a continental climate (De Aparicio and Difrieri, 1985). Salinization processes dominate and the soils are typically alkaline with a predominance of mollisols and aridisols (Del Valle, 1998). In addition, the mean annual air temperature fluctuates between 7.5 and 12.5°C (Díaz et al., 2007).
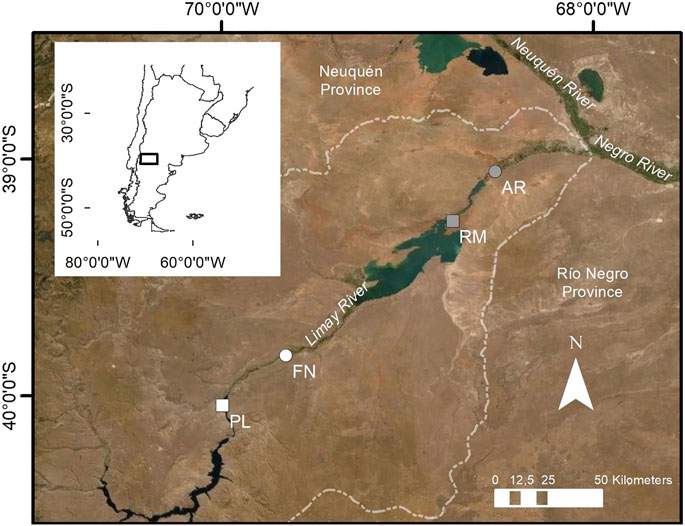
FIGURE 1. Geographic location of sampling river (circles AR, Arroyito; FN, Fortín Nogueira) and reservoir (squares RM, Ezequiel Ramos Mexía; PL, Pichi Picún Leufú) sites of Limay River, Patagonia, Argentina. White panels represent the not invaded sites and gray panels the invaded sites.
The Limay has five dams: Alicurá, Piedra del Águila, Pichi Picún Leufú, Ezequiel Ramos Mexía, and Arroyito, all of which were constructed for the generation of electricity. Across the Limay River basin there are several sources of pollution which include urban sewage, industrial and agrochemical waste discharges (Autoridad Interjurisdiccional de las Cuencas de los ríos Limay, Neuquén y Negro, 2011). However, compared to other regions of the country, this basin is considered to have low levels of nutrients, suspended solids and water mineralization (Daniele et al., 2005). This river has tourist value, with development of sport fishing based on introduced salmonids, and other recreational activities such as rafting and canoeing (Añón Suárez and Albariño, 2020). Sites invaded and not invaded by C. fluminea were selected in two river sections and two reservoirs along the Limay River (Figure 1; Table 1).
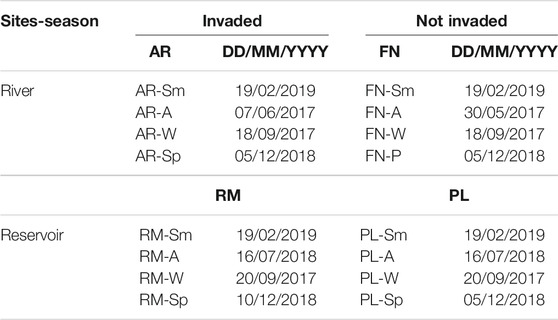
TABLE 1. Sampling labels acording to site and date, with three replicates each one. River (AR, Arroyito; FN, Fortín Nogueira) and reservoir (RM, Ezequiel Ramos Mexía; PL, Pichi Picún Leufú) sites of Limay River, Patagonia. Sm, summer; A, autumn; W, winter and Sp, spring.
Environmental Variables
Biotic homogenization and loss of β diversity during invasion is influenced not only by intrinsic species traits, but also extrinsic environmental characteristics (McKinney and Lockwood, 1999; Wang et al., 2021). Thus, the environmental variables most frequently cited as having an influence on the autecology of the invasive species and or on aquatic macroinvertebrate communities were analyzed (i.e., Darrigran and Damborenea, 2005; Sousa et al., 2008a; Sousa et al., 2008b; Batzer and Boix, 2016; Azis and Abas, 2021). The probe-rod method was used to characterize substrate composition (Bevenger and King, 1995). Substrate composition was separated into five size fractions, reported as % abundance. Sizes included bedrock (large unbroken surface), block (257–500 mm), pebble (65–256 mm), gravel (2–64 mm), and clay + silt + sand (CSS) (<2 mm). Temperature (T) and dissolved oxygen (DO) were measured in situ with a YSI Pro 20 oximeter, and pH and electrical conductivity (EC) were measured with an OAKTON PCS Testr 35 multiparameter probe. A total of 5 L of subsurface water (0.2–0.3 m below the water surface) was collected in plastic bottles at each sampling site for lab analysis.
Total suspended solids in water (TSS) were measured by weighing. The water sample was filtered with a 0.45 µm filter (Whatman filters) and a vacuum filtration system. Filters were washed with distilled water and dried at 103–105°C to a constant weight. The dry weight of the filters was weighed using a Mettler Toledo analytical balance (error = 0.0001 g) and TSS = initial−final weight (APHA 2001).
Total water nitrogen (TN) was determined by basic digestion (Grasshoff et al., 2009) and evaluation of N-NO3− + N-NO2− by reduction in a cadmium column and diazotization (APHA, 2001). Total water phosphorus (TP) was determined colorimetrically (Golterman et al., 1978). Chlorophyll a (Chla) was extracted with 90% acetone and measured using a spectrophotometer (Metrolab M-1700 model) (APHA, 2001).
Sampling and Classification of Aquatic Macroinvertebrates
The aquatic macroinvertebrate assemblages were sampled according to the adapted multi-habitat approach proposed by Barbour et al. (2006). A 30 cm wide D-frame net with a 500 µm mesh was used to collect the macroinvertebrates, along a transect of 150 cm. Thus each replicate had an area of 30 × 150 cm, which consisted of three 50 cm long net trawls, to represent each biotope present at the site. A total area of 1.35 m2 was sampled at each location. The main biotopes were considered according to the multi-habitat approach described by Barbour et al. (1999). Habitats were categorized before each sampling according to substrate type, presence of macrophytes and/or litter, pools and/or runs, through a qualitative evaluation of each site. Three replicates were taken in each of the two river (AR: invaded; FN: not invaded) and two reservoir (RM: invaded; PL: not invaded) sites, during four seasons, giving a total of 48 samples of aquatic macroinvertebrates (Table 1). The replicates were also considered as samples and treated separately.
The samples were preserved in 70% ethanol and identified at the smallest possible taxonomic level, by using a stereoscopic microscope (4X) and an optical microscope (64X–640X). Keys for macroinvertebrates of South America were used, including Bachmann (1981), Wiederholm (1989), Wygodzinsky and Coscarón (1989); Rodríguez-Capítulo (1992); Lopretto and Tell (1995); Archangelsky (1997); Cranston (2000); Epler (2001); Fernández and Domínguez (2001); Oliva et al. (2002); Heckman (2006); Michat and Archangelsky (2007); Heckman (2008); Michat et al. (2008); Alarie et al. (2009); Domínguez and Fernández (2009); Libonatti et al. (2011) and Muzón et al. (2014). Macroinvertebrate density was expressed as ind/m2.
Macroinvertebrate taxa were classified into functional feeding groups (FFG) based on their morphological and behavioral adaptations to acquiring food (Lopretto and Tell, 1995; Merritt and Cummins, 1996; Miserendino, 2007; Ramírez and Gutiérrez-Fonseca, 2014). The FFG were classified as follows: 1) shredders (SH, feed on organic matter in the form of coarse particles >1 mm in size, consisting either of living tissue from aquatic macrophytes or coarse organic matter from terrestrial plants); 2) scrapers (SC, scrape and consume organic matter attached to rocks and other substrates); 3) collector-filterers (CF, sieve fine particles of 1,000–0.45 µm from the flowing water column); 4) collector-gatherers (CG, collect fine particles of organic matter from debris and sediments in stream beds); 5) predators (PD, feed on live animals); and 6) piercer (PC, feed on vascular plants by cutting or perforating the tissue and consume plant liquids). The relative abundance (%) of each FFG was calculated based on its total abundance.
Statistical Analysis
The environmental differences between the invaded and non-invaded sites were evaluated to identify if species similarity occurs in presence of C. fluminea, despite the separate localities involved and the spatial heterogeneity between the sites. An analysis of the differences between the types of environments (reservoir vs. river), was also conducted to examine whether or not abiotic factors are drivers of biotic differentiation and mask the possible homogenization caused by invasive species.
Species richness (S) was determined, and community structure was evaluated, using the Shannon diversity index (H′ =−Σ pi ln pi) (Shannon and Weaver, 1963) and the Pielou evenness (J′ = H′ ln S) (Pielou, 1977) from the abundance of macroinvertebrates (PRIMER 6.1.6). A non-parametric Kruskal-Wallis test was applied to evaluate the differences in abundance between seasons of each sampling site (AR, FN, RM, and PL) (Hollander and Wolfe, 1973). To analyze the differences between aquatic environments (river vs. reservoir) and estimate the impact of C. fluminea (invaded and non-invaded sites) on the abundance of taxa, a non-parametric Wilcoxon test and an Analysis of Variance (ANOVA) were applied using InfoStat (Di Rienzo et al., 2014).
Multivariate ordering by non-Metric Multidimensional Scaling (nMDS) was also used to evaluate the differences between ecosystems (river and reservoir, invaded and non-invaded) (Ludwig and Reynolds, 1988), with respect to the composition of aquatic macroinvertebrates. A Bray-Curtis similarity index matrix of the abundance data was used as a basis, with the log x+1 transformation (Clarke and Warwick, 2001). Spearman correlation indices were calculated between the nMDS coordinates and environmental variables and community attributes (richness and density) with InfoStat (Di Rienzo et al., 2014), to reveal ecological patterns (Ter Braak, 1992; Maloney and Feminella, 2006).
A two-way crossed Analysis of Similarities (ANOSIM) was performed to test differences in macroinvertebrate species composition (abundance similarity matrix) between sites (Clarke and Warwick, 2001). Two comparisons were used in ANOSIM: reservoir vs. river, and invaded sites vs. non-invaded (by C. fluminea). Similarity Percentage analysis (SIMPER) was also used to determine the taxa contributing to the differences between sites (river vs. reservoir and invaded vs. not invaded) (Clarke and Warwick, 2001). The nMDS, ANOSIM and SIMPER analyzes were performed with PRIMER 6.1.6 (Clarke and Gorley, 2006).
Results
Environmental Characteristics of Sites
The sedimentary composition of the river sites was dominated by particles of medium-to-large sizes (65–500 mm), such as pebbles at the AR site (52.5%) and blocks at FN (40.0%). At the reservoir sites, sand-silt-clay (SSC) accounted for more than 50% of the total composition of the sediment particle size (Table 2).
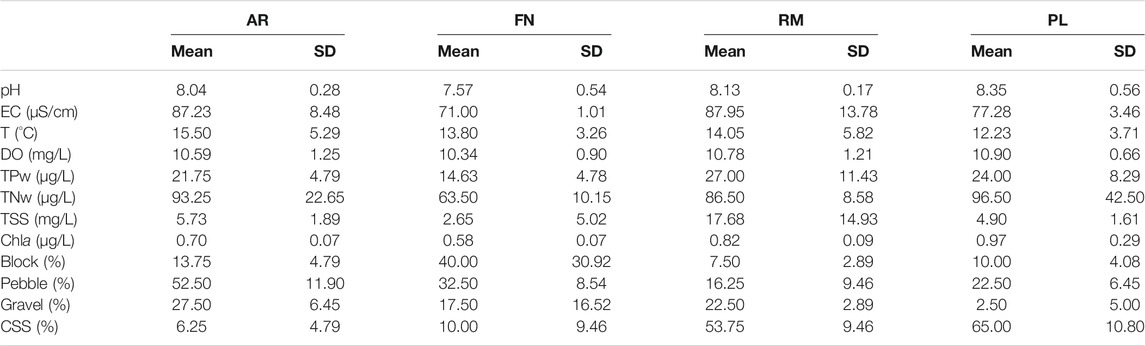
TABLE 2. Physical-chemical variables of sampling river (AR, Arroyito; FN, Fortín Nogueira) and reservoir (RM, Ezequiel Ramos Mexía; PL, Pichi Picún Leufú) sites of Limay River, Patagonia. SD, standard deviation; EC, electrical conductivity; T, Temperature; DO, dissolved oxygen; TP, Total water phosphorus; Total water nitrogen (TN); TSS, Total suspended solids; Chla, chlorophyll a; block (257–500 mm); pebble (65–256 mm); gravel (2–64 mm); clay + silt + sand (CSS) (<2 mm).
The pH values were slightly higher at reservoir sites compared to river sites, with the highest seasonal variations (standard deviation) at non-invaded FN and PL sites (Table 2). Electrical conductivity (EC) was approximately 19 and 12% higher in invaded sites (AR river and RM reservoir respectively) compared to non-invaded homologous sites (FN river and PL reservoir), with a statistically significant difference between river sites (AR vs. FN) (ANOVA F = 14.42 p = 0.009). Dissolved oxygen (DO) concentrations between 10.34 and 10.90 mg/L were recorded.
TP was higher in reservoirs (RM and PL) compared to river sites (AR and FN). The RM reservoir had the highest variability (as standard deviation) of this nutrient between seasons (Table 2). FN site had the lowest nutrient (TP and TN) and chlorophyll-a (Chla) values. On the other hand, PL site had the highest concentrations of TN, and showed a high seasonal variability (SD). Chla values were higher in reservoir sites, and significantly higher in the invaded river site AR (around 17%), compared to its non-invaded counterpart FN (ANOVA; rivers F = 8.42 p = 0.027).
Macroinvertebrate Assemblages and Environmental Relationships
A total richness (S) of 73 taxa (51 and 48 at river and reservoir sites, respectively) from 44 families of Turbellaria, Annelida, Mollusca, Crustacea, Arachnida, and Insecta was recorded. The most abundant families were Dugesiidae (Turbellaria), Cochliopidae, Chilinidae (Mollusca), Hyalellidae (Crustacea), Leptophlebiidae, Baetidae, Caenidae, Chironomidae, Leptoceridae and Hydropsychidae (Insecta). These accounted for almost 90% of the abundance of the Limay River system. Chironomidae was the family with the highest species richness (S = 12), although it had low abundance values and few taxa at the FN river site.
The mean values of richness for the river sites were 21 and 15 (AR and FN respectively), whilst the reservoirs scored 18 and 7 (PL and RM respectively). These totals were calculated taking into account the accumulated richness of the three replicas from each site for the four seasons. The mean of the Shannon diversity indices was higher in the most productive sites AR and PL (higher Chla content), while the Pielou values of evenness were similar at AR, RM and PL (0.63–0.67) and slightly lower at FN (0.54) (Table 3).
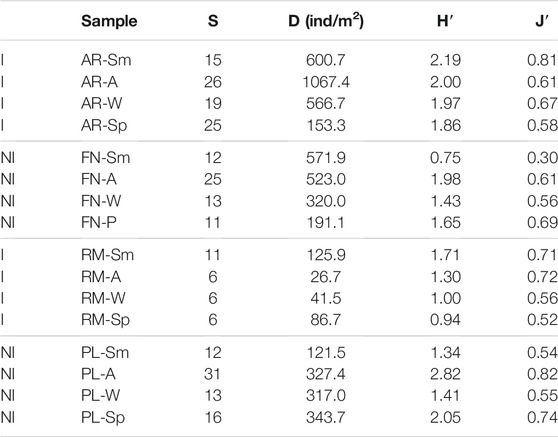
TABLE 3. Diversity index of macroinvertebrate of river (AR, Arroyito; FN, Fortín Nogueira) and reservoir (RM, Ezequiel Ramos Mexía; PL, Pichi Picún Leufú) sites of Limay River, Patagonia. S, species richness; D, density; H′, Shannon index and J′, Pielou evenness index; I, invaded and NI, not invaded sites; Sm, summer; A, autumn; W, winter and Sp, spring.
The total density of macroinvertebrates (D) was higher in the river sites AR (D = 597 ind/m2) and FN (D = 402 ind/m2), compared to the reservoir sites PL (D = 277 ind/m2), and RM (D = 70 ind/m2). There were significant differences in density between the lentic sites (Wilcoxon, W = 93.0; p = 0.001) (Figure 2). The only significant differences in seasonal variation were recorded at the AR river site, where mean densities for spring and autumn were significantly different (H = 8.13; p = 0.043) (Table 3).
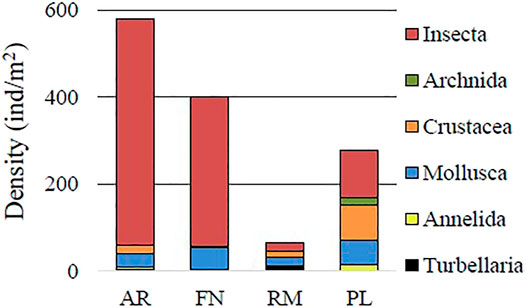
FIGURE 2. Density of macroinvertebrate groups of sampling river (AR, Arroyito; FN, Fortín Nogueira) and reservoir (RM, Ezequiel Ramos Mexía; PL, Pichi Picún Leufú) sites of Limay River, Patagonia.
The taxa of the main groups Turbellaria, Annelida, Mollusca, Crustacea, Arachnida and Insecta are shown in Supplementary Table S1. Insecta, with orders which included Ephemeroptera, Plecoptera, Hemiptera, Odonata, Coleoptera, Diptera, and Trichoptera, was the most abundant group in all sampling sites. This was especially true for rivers (AR and FN) when compared with reservoirs (RM and PL) (W = 378.5; p < 0.001) (Figures 2, 3). Insecta presented density values of 519 and 344 ind/m2 at the AR and FN river sites respectively, and 21 ind/m2 and 108 ind/m2 at the RM and PL reservoirs, respectively. Mollusca was also a relatively abundant group at AR (29 ind/m2) and FN (49 ind/m2) (Figure 2). Mollusca (19 ind/m2), Crustacea (14 ind/m2) and Turbellaria (12 ind/m2) had relatively similar abundance values in RM. At PL, Crustacea (81 ind/m2) and Mollusca (55 ind/m2) were the most abundant groups. Comparing the invaded and non-invaded river sites, Crustacea was the only main group with significantly higher values at AR, compared with FN (W = 158.0; p = 0.005). At the reservoir sites, Mollusca (W = 111.5; p = 0.026), Crustacea (W = 99.0; p = 0.003) and Insecta (W = 100.0; p = 0.004) had significantly higher values of abundance in the non-invaded site (PL) compared to the invaded site (RM).
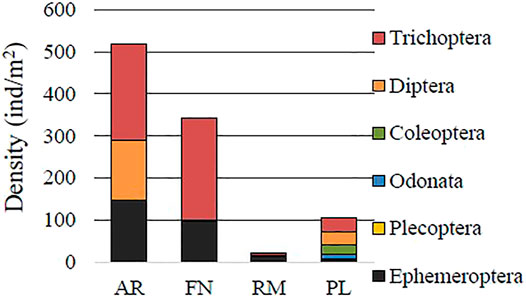
FIGURE 3. Density of Insecta groups of sampling river (AR: Arroyito, FN: Fortín Nogueira) and reservoir (RM, Ezequiel Ramos Mexía; PL, Pichi Picún Leufú) sites of Limay River, Patagonia.
Some species were only registered in river or reservoir sites. Dero sp. (Annelida), Sympetrum villosum (Odonata, Insecta), Dicrotendipes sp., Pentaneura sp. y Molophilus sp. (Diptera, Insecta) were only detected in the RM y PL reservoirs. Furthermore, Brachysetodes sp. (Trichoptera, Insecta) was a relatively abundant species of reservoirs, with relative abundance values of 8.08 and 10.40 % at RM and PL, respectively. In contrast, the species’ values at AR and FN river sites were recorded as 0 and 0.61%, respectively.
Anisancylus obliquus, Heleobia hatcheri (Mollusca), Aegla riolimayana (Crustacea), Camelobaetidus sp., Andesiops torrens (Ephemeroptera, Insecta), Cricotopus sp. (Diptera, Insecta), Smicridea (Rhyacophylax) annulicornis (Trichoptera, Insecta), were species exclusively registered at the AR and FN river sites. Limnodrilus sp., Helobdella sp. (Annelida), Sigara (Tropocorixa) santiagiensis (Hemiptera, Insecta) and Verger sp. (Trichoptera, Insecta) were only registered in non-invaded sites. In contrast, no species which were exclusive to invaded sites (AR and RM) were detected.
The nMDS graph highlights the fact that the sample points do not overlap. (Figure 4). This could be an indication that the composition of macroinvertebrate species is quite specific to each site. Furthermore, the non-invaded ecosystems (FN and PL) show the highest differences while the invaded sites (AR and RM) are near located at graph’s center. A posteriori, it seems that the horizontal axis is positively correlated with macroinvertebrate density (r = 0.57 p = 0.022). TP and TSS are negatively correlated with the horizontal axis (TP r = −0.61 p = 0.012); TSS r = −0.56 p = 0.023). The vertical axis is negatively correlated with pH (r = −0.60 p = 0.014), chlorophyll a (r = −0.57 p = 0.022) and macroinvertebrate richness (r = −0.61 p = 0.011).
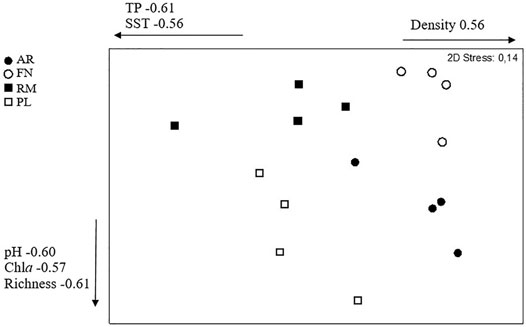
FIGURE 4. Non-metric multidimensional scaling (nMDS) ordination of a Bray–Curtis similarity matrix of macroinvertebrate composition. Sampling river (circles AR, Arroyito; FN, Fortín Nogueira) and reservoir (squares RM, Ezequiel Ramos Mexía; PL, Pichi Picún Leufú) sites of Limay River, Patagonia, Argentina. White panels represent the not invaded sites and black panels the invaded sites. The variables that were significantly correlated with the nMDS axes are displayed.
The ANOSIM test also indicated significant differences between the species composition of invaded and non-invaded sites (ANOSIM Global r = 0.557, p = 0.004) and between rivers and reservoirs (ANOSIM Global R = 0.786, p = 0.005). Invaded (AR and RM) and non-invaded groups (FN and PL), had average similarities of 37.50 and 45.96% respectively, according to the two-way SIMPER. The average dissimilarity between invaded and non-invaded sites was 76.80%. The species with the highest contribution to the discrimination between invaded and non-invaded samples according to the mean dissimilarity/standard deviation ratio were Paratrichocladius sp. (Diptera, Insecta), Hyalella curvispina (Crustacea) and Chilina gibbosa (Mollusca). The taxa in Table 4 contributed up to 56.5% cumulative of the average differences between invaded and non-invaded sites.
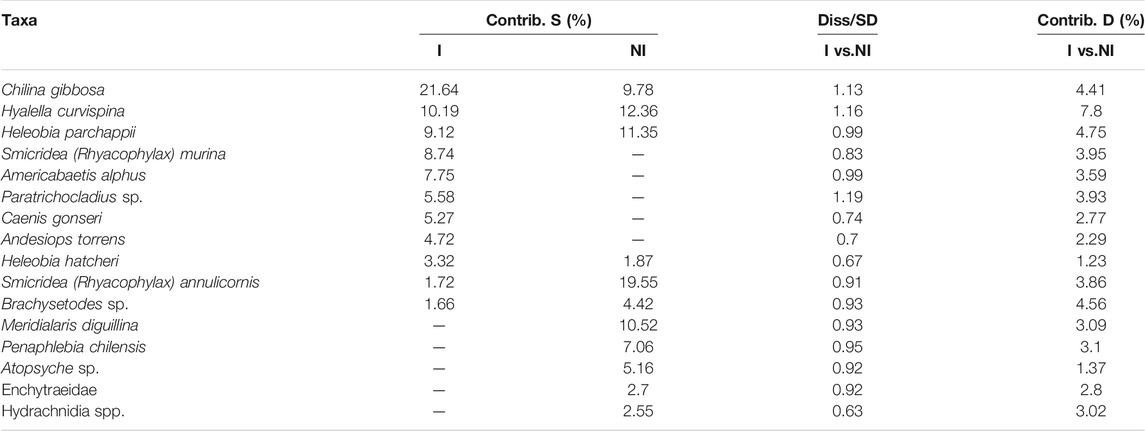
TABLE 4. Summary of Similarity Percentage analysis (SIMPER) of macroinvertebrate, showing the organisms which most contributed to the observed differences among groups (I, invaded and NI, not invaded sites). Contrib.S, contribution to the intragroup simillarity; Diss, mean disimillarity and their SD, standard deviation; Contrib.D, contribution to the intergroup disimillarity.
The two-way SIMPER showed an average similarity of 53.94 and 29.52% within rivers (AR and FN) and reservoirs (RM and PL), respectively (Table 5). The species with the highest contribution to the average similarity within the river group were Smicridea (Rhyacophylax) annulicornis, Chilina gibbosa, Meridialaris diguillina, Caenis gonseri and Penaphlebia chilensis. The average difference between these groups was 86.54%. The species that best discriminated between the river and reservoir samples according to the mean dissimilarity/standard deviation ratio were Aegla riolimayana, Hyalella curvispina (Crustacea), Heleobia parchappii (Mollusca) and Smicridea (Rhyacophylax) annulicornis (Insecta). 19 taxa contributed to 66% of the difference between the river and reservoir macroinvertebrate samples.
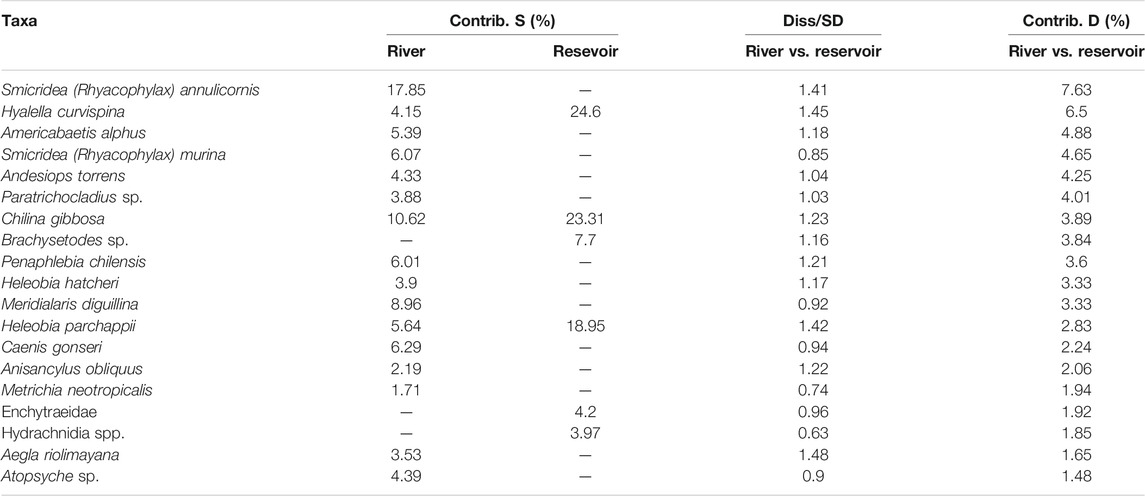
TABLE 5. Summary of Similarity Percentage analysis (SIMPER) of macroinvertebrate, showing the organisms which most contributed to the observed differences among groups (river and reservoir). Contrib.S, contribution to the intragroup simillarity; Diss, mean disimillarity and their SD, standard deviation; Contrib.D, contribution to the intergroup disimillarity.
The invasion’s effect on the decrease in the density of bivalve molluscs is observed (eg. Pisidium sterkianum AR D = 12.6 vs. FN D = 17.78; RM D = 5.6 vs. PL D = 33.7 ind/m2; and Diplodon chilensis AR D = 0 vs. FN D = 1.3; RM D = 0.4 vs. PL D = 3.0 ind/m2) as well as on the decrease in the density of native gastropods (AR D = 29.1 vs. FN D = 48.2; RM D = 18.2 vs. PL D = 50.2 ind/m2) (eg., Heleobia).
The density of Ephemeroptera (Insecta) increased, as a probable consequence of the invasion (AR D = 148.6 vs. FN D = 97.6; RM D = 13.0 vs. PL D = 5.9 ind/m2) (mainly Penaphlebia chilensis, Americabaetis alphus, Camelobaetidus sp., Andesiops torrens, Caenis gonseri). On the contrary, the density of Meridialaris spp., which are also mayflies, decreased. Even so, when all taxa were included in the data, a net increase was observed in Ephemeroptera density. Coleoptera insects (eg., Luchoelmis sp., Stenelmis sp., Austrelmis sp., Lancetes sp., Desmopachria, Tropisternus, Berosus sp. y Curculionidae) also diminished in invades sites (AR D = 0 vs. FN D = 1.48; RM D = 0 vs. PL D = 23.5 ind/m2). For Trichoptera (AR D = 227.4 vs. FN D = 243.7; RM D = 5.4 vs. PL D = 31.3 ind/m2), the differences were lower.
Of the 73 taxa recorded across all sites, 26 were collector-gatherers (CG), 22 predators (PD), 14 scrapers (SC), seven collector-filterers (CF), two piercers (PC) and one was a shredder (SH) (Supplementary Table S1). In terms of total average abundance, the collector-filterers predominated in the river sites, with 460 and 530 ind/m2 at AR and FN, respectively (Figure 5). The dominant functional group in the lentic sites were the collector-gatherers with 77 and 355 ind/m2 at RM and PL reservoirs, respectively. Predators had the following density values AR D = 25, FN D = 25, RM D = 30, PL D = 116 ind/m2. The piercers and shredders were registered only in non-invaded sites FN (river) and PL (reservoir), with density values of between 0 and 13 ind/m2.
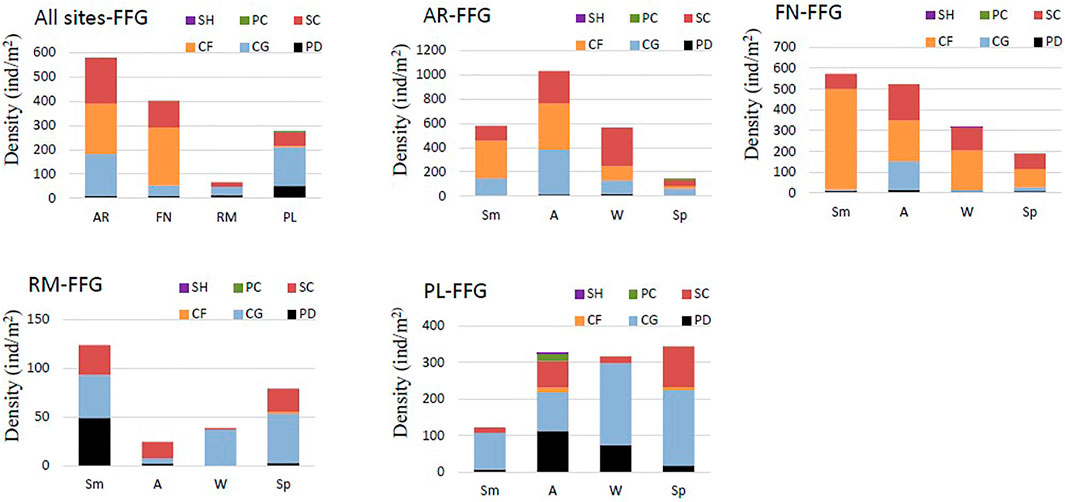
FIGURE 5. Density of functional feeding groups (FFG) of macroinvertebrates in all sites (mean) and in river (AR, Arroyito; FN, Fortín Nogueira) and reservoir (RM, Ezequiel Ramos Mexía; PL, Pichi Picún Leufú) sites of Limay River, Patagonia, SH, shredders; SC, scrapers; CF, collector-filterers; CG, collector-gatherers; PD, predators; PC, piercers; Sm, summer; A, autumn; W, winter and Sp, spring.
The functional groups also showed seasonal variation within each site. At AR in summer and autumn, three groups (CF, CG and SC) had relatively similar abundance values (Figure 5). Predators were recorded with mean abundance values of 0, 15, 20, and 10 ind/m2 in summer, autumn, winter and spring, respectively. Scrapers predominated in winter and collector-filterers in spring.
At the FN river site, collector-filterers dominated in all seasons, especially in summer. In autumn, scrapers, collector-gatherers and predators reached their highest values with 174, 136, and 17 ind/m2, respectively. In autumn and spring, only one herbivorous biting individual was recorded in one of the three replicates for both seasons. In winter and spring, collector-gatherers decreased to approximately 7 and 20 ind/m2 respectively, with total abundance also decreased. In winter, two shredders individuals were recorded in one of the three replicas. Predators had a relatively low abundance (7–17 ind/m2) in all samples (Figure 5).
The RM reservoir had a variable composition with regards to functional groups. The CG, the dominant group of the reservoir, had abundance values of 44, 37, and 51 ind/m2 in summer, winter and spring, but in autumn the values were lower with 5 ind/m2. PD had an abundance of 49 ind/m2 in summer, but 2, 0, and 3 ind/m2 in autumn, winter and spring, respectively. The SC registered values of approximately 30, 17, 2, and 24 ind/m2, and in contrast, the CF were less abundant, with values of 2, 0, 3, and 9 ind/m2 in summer, autumn, winter and spring, respectively (Figure 5).
In the PL reservoir, the CG had density values of 101 and 112 ind/m2 in summer and autumn, respectively, with their values effectively doubling in winter and spring (225 and 207 ind/m2, respectively). PD and SC had similar total and seasonal density values, mainly in summer (PD = 7 ind/m2, SC = 14 ind/m2) and autumn (PD = 107 ind/m2, SC = 72 ind/m2). SH, CF, and FR had their highest abundance values in autumn, with approximately 22, 13, and 3 ind/m2, respectively (Figure 5).
Discussion
The analysis of the impact of C. fluminea invasions is complex (López-Soriano et al., 2018). The invasion of this species has been speculated to have negatively affected the abundance and diversity of native bivalves in North America (Gardner et al., 1976; Taylor and Hughart, 1981; Clarke, 1988). But these results are inconsistent with experimental testing (Strayer at al., 1999) and may even vary according to the different spatial scales at which the data are collected (Vaughn and Spooner, 2006). This study attempted to shed light on the impact of C. fluminea on the homogenization of taxonomic and functional properties of macroinvertebrate assemblages in the Limay River. The two invaded habitats, AR (river) and RM (reservoir) both displayed a certain homogenization of their constituent species and functional composition in comparison to their non-invaded counterparts, FN and PL. These similar effects were considered to be due to the impact of C. fluminea invasion.
For example, no significant relationship was found between the invasion of C. fluminea and the richness and total density of benthic macroinvertebrates in the Limay River. Richness was higher in the AR (invaded) and PL (not invaded) sites, which are also more productive (Chla), and have a higher nitrogen contribution from the basin. The Shannon index and the Pielou evenness index displayed higher values at these invaded and non-invaded sites. This pattern could be due to the fact that nutrient loads can influence the structure of the macroinvertebrate community as a consequence of stimulation of primary production (Zhang et al., 2014).
Thus, either the invasion of C. fluminea’s did not affect α diversity, or its impact is masked by the intrinsic characteristics of the sites of the Limay River, such as productivity. In Patagonian ecosystems, which are usually oligotrophic (Díaz et al., 2007), the competition for nutrients under resource-limitation can limit the number of coexisting species (Hutchinson, 1961). The higher nutrient loads occurring in AR and PL sites compared to their homologous FN and RM could cause intermediate productivity. The intermediate productivity hypothesis predicts that species diversity is maximized at some intermediate level of productivity, in which competition for food is reduced and the coexistence of potentially competing species is promoted (Widdicombe and Austen, 2001).
Furthermore, macroinvertebrate density was higher at the AR and FN river sites, possibly due to the larger size of sediment particles and a more diverse substrate type (which is a surrogate for increasing habitat structural complexity) (Gething et al., 2020), compared with reservoir sites. The highly structured ecosystems of rivers, with their complex physical habitat structures, might support a higher abundance or range of resources or provide refugia (Platt, 2011). Lower density in reservoirs has previously been attributed to the fact that these lentic environments are characterized by the accumulation of fine sediments, which retain fewer coarse particles of organic matter (Larrañaga et al., 2003). They have also been shown to be less colonized by microalgae (Allan and Castillo, 2007), as organic matter and microalgae can be used as food resources by macroinvertebrates (Graça et al., 2004; Jun et al., 2011). A smaller availability of resources can also reduce macroinvertebrate diversity (Larsen et al., 2011; Jones et al., 2012), as observed in the lower richness values of the less productive FN and RM sites, compared with their counterparts AR and PL.
Changes in the abundance of some taxa were detected in presence of C. fluminea, with compositional similarity detected between the invaded sites according to the nMDS, ANOSIM and SIMPER. This suggests a homogenization of the invertebrate fauna, in a process known as biotic homogenization (Olden et al., 2011), in the presence of the invasive species C. fluminea. For example, native bivalves were observed in low density or were absent in invaded environments, compared to their non-invaded counterparts. There are several mechanisms by which invading bivalves can cause a reduction in the abundance of other bivalves. For example, zebra mussels Dreissena polymorpha (Ward and Ricciardi, 2007; Zaiko et al., 2009), being epifaunal and agglutinate on the shells of native bivalves, prevent their host unionids from opening their shells to breathe, feed, move and reproduce (Karatayev et al., 2007). Although this is not the mechanism of the corbiculids, in general, the decrease or absence observed in some taxa can be attributed to competition for space and/or food resources (Strayer et al., 1999; Novais et al., 2016).
C. fluminea’s population densities of between 133–519 ind/m2 in the Limay River were lower than those described for more “successful” populations in northern South America (eg., Rio Grande do Sul, Brazil and Río de La Plata, Argentina), and across the world (Danube, Bulgaria; Mondego, Portugal; New River, United States), which have all recorded values between 900 and 269,000 ind/m2 (Cherry et al., 1986; Dreher-Mansur and Garces, 1988; Darrigran, 1991; Dreher- Mansur et al., 1994; Franco et al., 2012; Hubenov et al., 2013; Modesto et al., 2013; Reshaid et al., 2017). Competition in this species, therefore, seems to be related more to resources than to space.
When resources are limited in the water column, invasive fast-growing bivalves can negatively affect the survival and growth of native bivalves (Crooks, 2001; Ferreira-Rodríguez et al., 2017). Furthermore, invasive species may have a competitive advantage due to their higher trophic plasticity (Novais et al., 2016). The decreased relative abundance of filter feeders in the invaded systems, compared to the non-invaded ones, could therefore be related to competition.
Gastropods in general, and especially the scraper Heleobia parchappii were diminished in both invaded sites (AR and RM), probably also due to a competitive effect for resources at the bottom of the river/reservoir. The clam’s ability to forage (Reid et al., 1992; Hakenkamp and Palmer, 1999) by pedal feeding transports sedimented particles to labial palps through ciliary tracts in the foot (in Way et al., 1990). This type of consumption reduces the amount of benthic and diatom bacteria in the sediment (Hakenkamp et al., 2001). It suggested that, through this mechanism, the clams can also exploit the sediment as a source of nutrients (Hakenkamp and Palmer, 1999; Vaughn et al., 2008), including their own deposited matter. These resources would no longer be available to other benthic taxa (Werner, 2014), and it is a plausible explanation for the decrease in some benthic macroinvertebrate taxa.
As scrapers, gastropods can benefit from the presence of invasive bivalves acting as ecosystem engineers (Sousa et al., 2009; Darrigran and Damborenea, 2011). The bivalves increase the quantity of bottom algae by increasing water clarity through filtration (Uhde et al., 2012), and by the deposition of feces and pseudo feces, which enrich the net organic content of benthic sediments (Vaughn and Hakenkamp, 2001). These changes can provide an additional food resource for benthic invertebrates (Roditi et al., 1997). According to the literature, invasive clams can cause contrasting effects, depending on the initial characteristics of invaded sites, and both increases and decreases in the density of gastropods have been recorded in their presence (Darrigran et al., 1998; Horvath et al., 1999; Mörtl and Rothhaupt, 2003; Marçal and Tasso-Callil, 2008; Zaiko et al., 2009). In the context of the oligotrophic Limay River of northern Patagonia, the present study demonstrated that gastropod populations are reduced in communities invaded by C. fluminea. This supports the hypothesis that the presence of the invasive bivalve causes reduction in gastropod populations in low productivity aquatic environments. However, more evidence might be needed to further examine this idea.
The populations of certain Ephemeroptera species appear to have increased with the invasion of C. fluminea (mainly the collector-gatherers species such as Penaphlebia chilensis, Americabaetis alphus, Camelobaetidus sp., Andesiops torrens, Caenis gonseri). These species use small debris (fine particulate organic matter), as well as coarse particulate organic matter debris, periphyton shed from scrapers, small algae, and debris from upstream organisms (Vannote et al., 1980). This could be favored by the bioturbation activities of C. fluminea which stirs the contents of the sediments. In addition, the collector-gatherers families of mayfly nymphs such as Baetidae (Americabaetis alphus, Camelobaetidus sp., Andesiops torrens) have a hydrodynamic body shape and are good swimmers, which allows better opportunities in the search for food. Furthermore, the empty shells of C. fluminea may act as refuges for some benthic species. For example, an experiment by Werner (2014) found a significantly higher density of ephemeral Caenis spp. in treatments with empty valves of C. fluminea compared to the other treatments.
The influence of C. fluminea on the abundance of coleopterans (Elmidae: Luchoelmis sp. SC, Stenelmis sp. SC Austrelmis sp. SC; Dytiscidae: Lancetes sp. PD and Desmopachria PD; Hidrophilidae: Tropisternus SC, Berosus sp. CG) seems to be a negative one, as these taxa were only present in the non-invaded sites. Elmids, which are scrapers, were only present at the non-invaded river site (FN), while the rest of those with varied functionality such as predators, scrapers and collectors, were only present at the non-invaded reservoir site (PL). The relatively low abundance of some taxa has previously been attributed to the impact of invasive bivalves, when this may actually reflect the different habitat requirements of the two groups (Aldridge et al., 2007). For example, the muddy substrates associated with lentic habitats favor Dytiscidae (Aldridge et al., 2007), while unionids are rarely found in such habitats (Killeen et al., 2004). In this study, both river sites were comprised of fast-flowing waters, and both reservoir sites possessed a sediment composition in which small particles predominate, elmids and dysticid had lower abundance values at invaded river and reservoir sites.
The presence of C. fluminea influences the composition and functionality of macroinvertebrate assemblages, by affecting food availability, especially algal particles or organic matter composed of plankton and sediment. The competition causes a decrease in the density of some taxa. However, as observed, the density of some groups such as scrapers or gatherers, either decreased or indeed increased in the presence of this species, probably as a result of their varying feeding strategies (for example, some gastropod scrapers, whose density decreased, have very little mobility, compared to some ephemeral scrapers, whose density increased). The effect seen on some predators of certain groups may be related to the impact on food chains, and the reduced densities of some primary consumers due to competition.
Despite the potential impact of invasion on the decrease or increase of some major taxa or functional groups compared to non-invaded sites, perhaps the most important finding of this study was the similarity between invaded sites. Under the new conditions created by Corbicula fluminea, similar species prevail, as highlighted by the nMDS, ANOSIM and SIMPER analyses. The rearrangement of macroinvertebrate assemblages across the landscape of Limay River leads to homogenization, with biodiversity (as β diversity) loss. This could be especially harmful in Patagonia’s fragile ecosystems.
Conclusions
The invasive species C. fluminea had a negative influence on the abundance values of some taxa such as gastropods, bivalves, Coleoptera and Trichoptera, due to the competition for the food resource, in these habitually not-very-productive arid Patagonian habitats. However, no influence of the invasive species was found on the total density and α diversity of macroinvertebrates. These variables were mainly driven by the productivity of the sites.
The decreased abundance of collector-filter functional group in the presence of C. fluminea could be related to competition for scarce plankton resources, in these mostly oligotrophic environments. A possible mechanism for the depletion of some benthic macroinvertebrate taxa could be the combination of filter and pedal feeding described for this invasive species. The increase in the density values of Ephemeroptera, which consist of several functional feeding groups, at the invaded sites indicates that the presence of C. fluminea can favor highly mobile taxa with better food-finding capabilities, probably through its bioturbating activities.
In the absence of control, the presence of C. fluminea in the aquatic ecosystems of Patagonia could continue to expand, exerting a homogenizing influence on the benthic assemblages of macroinvertebrates and bringing about a loss of β diversity.
Data Availability Statement
The original contributions presented in the study are included in the article/Supplementary Material, further inquiries can be directed to the corresponding author.
Author Contributions
All authors listed have made a substantial, direct, and intellectual contribution to the work and approved it for publication.
Funding
This work was financed with the project PI UNRN 40 A 549 (2017–2020) of Universidad Nacional de Río Negro “The invasion of Corbicula fluminea (Müller, 1774) in North Patagonia”. CONICET financed the doctoral scholarship awarded to YL.
Conflict of Interest
The authors declare that the research was conducted in the absence of any commercial or financial relationships that could be construed as a potential conflict of interest.
Publisher’s Note
All claims expressed in this article are solely those of the authors and do not necessarily represent those of their affiliated organizations, or those of the publisher, the editors and the reviewers. Any product that may be evaluated in this article, or claim that may be made by its manufacturer, is not guaranteed or endorsed by the publisher.
Supplementary Material
The Supplementary Material for this article can be found online at: https://www.frontiersin.org/articles/10.3389/fenvs.2021.728620/full#supplementary-material
References
Alarie, Y., Michat, M. C., Nilsson, A. N., Archangelsky, M., and Hendrich, L. (2009). Larval Morphology of Rhantus Dejean, 1833 (Coleoptera: Dytiscidae: Colymbetinae): Descriptions of 22 Species and Phylogenetic Considerations. Zootaxa 2317, 1–102. doi:10.11646/zootaxa.2317.1.1
Aldridge, D. C., Fayle, T. M., and Jackson, N. (2007). Freshwater Mussel Abundance Predicts Biodiversity in UK lowland Rivers. Aquat. Conserv: Mar. Freshw. Ecosyst. 17 (6), 554–564. doi:10.1002/aqc.815
Aldridge, D. C., and Müller, S. J. (2001). The Asiatic Clam, Corbicula Fluminea, in Britain: Current Status and Potential Impacts. J. Conchol. 37, 177.
Allan, J. D., and Castillo, M. M. (2007). Stream Ecology: Structure and Function of Running Waters. Dordrecht, The Netherlands: Springer Science and Business Media.
Añón Suárez, D., and Albariño, R. J. (2020). Efecto del establecimiento del alga invasora Didymosphenia geminata sobre la abundancia de macrocrustáceos en el Río Limay superior, Patagonia, Argentina. bacuatica (34), 006–012. doi:10.24215/16684869e006
APHA (2001). American Public Health Association. Standard Methods for the Examination of Water and Wastewater. Hanover, Maryland USA: American Water Works Association.
Araujo, R., Moreno, D., and Ramos, R. A. (1993). The Asiatic Clam Corbicula Fluminea (Müller, 1774) (Bivalvia: Corbiculidae) in Europe. Am. Malacol. Bull. 10, 39–49.
Archangelsky, M. (1997). “Studies on the Biology, Ecology, and Systematics of the Immature Stages of New World Hydrophiloidea (Coleoptera: Staphyliniformia),” in Ohio Biological Survey (Columbus, OH: College of Biological Sciences, the Ohio State University).
Autoridad Interjurisdiccional de las Cuencas de los ríos Limay, Neuquén y Negro (2011). Índices de calidad del agua. Available at: http://www.aic.gov.ar/sitio/archivos/201702/ica%20-%20indices%20de%20calidad%20del%20agua.pdf (Accessed March 2, 2021).
Azis, M. N., and Abas, A. (2021). The Determinant Factors for Macroinvertebrate Assemblages in a Recreational River in Negeri Sembilan, Malaysia. Environ. Monit. Assess. 193 (7), 1–12. doi:10.1007/s10661-021-09196-7
Bachmann, A. O. (1981). Insecta, Hemiptera, Corixidae. En: Ringuelet, A. (dir.), Fauna de Agua Dulce de la República Argentina, FECIC, Bs.As., 35 (2), 1–270.
Bachmann, V., Beisel, J. N., Usseglio-Polatera, P., and Moreteau, J. C. (2001). Decline of Dreissena polymorpha in the River Moselle: Biotic and Abiotic Key Factors Involved in Dynamics of Invasive Species. fal 151, 263–281. doi:10.1127/archiv-hydrobiol/151/2001/263
Barbour, M. T., Gerritsen, J., Snyder, B. D., and Stribling, J. B. (1999). Rapid Bioassessment Protocols for Use in Streams and Wadeable Rivers. Washington: USEPA.
Barbour, M. T., Stribling, J. B., and Verdonschot, P. F. (2006). The Multihabitat Approach of USEPA's Rapid Bioassessment Protocols: Benthic Macroinvertebrates. Limnetica 25 (3), 839–850.
Batzer, D., and Boix, D. (2016). “An Introduction to Freshwater Wetlands and Their Invertebrates,”. Invertebrates in Freshwater Wetlands (Springer International Publishing), 1–23. doi:10.1007/978-3-319-24978-0_1
Bellard, C., Cassey, P., and Blackburn, T. M. (2016). Alien Species as a Driver of Recent Extinctions. Biol. Lett. 12 (2), 20150623. doi:10.1098/rsbl.2015.0623
Bevenger, G. S., and King, R. M. (1995). A Pebble Count Procedure for Assessing Watershed Cumulative Effects, 319. Fort Collins, Colorado: US Department of Agriculture, Forest Service, Rocky Mountain Forest and Range Experiment Station. Research Paper. RM-RP-319.
Boyero, L., López-Rojo, N., Tonin, A. M., Pérez, J., Correa-Araneda, F., Pearson, R. G., et al. (2021). Impacts of Detritivore Diversity Loss on Instream Decomposition Are Greatest in the Tropics. Nat. Commun. 12, 3700. doi:10.1038/s41467-021-23930-2
Brown, B. L., and Barney, J. N. (2021). Rethinking Biological Invasions as a Metacommunity Problem. Front. Ecol. Evol. 8, 584701. doi:10.3389/fevo.2020.584701
Cherry, D. S., Roy, R. L., Lechleitner, R. A., Dunhardt, P. A., Peters, G. T., and Cairns, J. (1986). Corbicula Invasion in the New River with Fouling and Control Measures at the Celco Plant. Second International Corbicula Symposium. Am. Malacol. Bull. 2, 69–81.
Clarke, A. H. (1988). Aspects of Corbiculid-Unionid Sympatry in the United States. Malacology Data Net 2 (3/4), 57–99.
Clarke, K. R., and Gorley, R. N. (2006). PRIMER V6: User Manual/tutorial: Plymouth. Plymouth, UK: Primer-E, Ltd.
Clarke, K. R., and Warwick, R. M. (2001). Change in marine Communities. An Approach to Statistical Analysis and Interpretation. 2nd edition. PRIMER-EPlymouth.
Cranston, P. S. (2000). Electronic Guide to the Chironomidae of Australia. Available at: http://entomology.ucdavis.edu/chiropage/index.html. (Accessed May 15, 2021).
Crooks, J. A. (2001). Assessing Invader Roles within Changing Ecosystems: Historical and Experimental Perspectives on an Exotic Mussel in an Urbanized Lagoon. Biol. Invasions. 3 (1), 23–36. doi:10.1023/A:1011404914338
Daniele, C., Ríos, D., De Paula, M., and Frassetto, A. (2005). Impacto y riesgo de la expansión urbana sobre los valles de inundación en la Región Metropolitana de Buenos Aires. Situación ambiental Argentina, 457–461.
Darrigran, G., Agudo-Padrón, I., Baez, P., Belz, C., Cardoso, F., Carranza, A., et al. (2020). Non-native Mollusks throughout South America: Emergent Patterns in an Understudied Continent. Biol. Invasions. 22 (3), 853–871. doi:10.1007/s10530-019-02178-4
Darrigran, G. (1991). Competencia entre dos especies de Pelecípodos invasores: Corbicula fluminea (Müller 1774) y C. largillierti (Philippi, 1844) en el litoral argentino del estuario del Río de la Plata. Biología Acuática 15 (2).
Darrigran, G., and Damborenea, C. (2005). “La almeja de agua dulce Corbicula fluminea (Müller, 1774),” in Invasores Invertebrados exóticos en el Río de la Plata y región marina aledaña (Buenos Aires: Eudeba), 133–177.
Darrigran, G., and Damborenea, C. (2011). Ecosystem Engineering Impact ofLimnoperna Fortuneiin South America. Zoolog. Sci. 28 (1), 1–7. doi:10.2108/zsj.28.1
Darrigran, G., Martin, S. M., Gullo, B., and Armendariz, L. (1998). Macroinvertebrados associated to Limnoperna fortunei (Dunker, 1857) (Bivalvia, Mytilidae). Río de la Plata, Argentina. Hydrobiologia 367 (1), 223–230. doi:10.1023/a:1003244603854
De Aparicio, F. O., and Difrieri, H. A. (1985). La Argentina. Suma de Geografías. Buenos AiresPeuser: Tomo I.
Di Rienzo, J. A., Casanoves, F., Balzarini, M. G., Gonzalez, L., Tablada, M., and Robledo, Y. C. (2014). InfoStat Versión 2014. Grupo InfoStat, FCA. Argentina: Universidad Nacional de Córdoba. Available at:http://www. infostat. com. ar.
Díaz, M., Pedrozo, F., Reynolds, C., and Temporetti, P. (2007). Chemical Composition and the Nitrogen-Regulated Trophic State of Patagonian Lakes. Limnologica 37 (1), 17–27. doi:10.1016/j.limno.2006.08.006
Domínguez, E., and Fernández, H. R. (2009). Macroinvertebrados Bentónicos Sudamericanos: Sistemática Y Biología. Tucumán, Argentina: Fundación Miguel Lillo, 656.
Drago, E., and Quirós, R. (1995). The Hydrochemistry of the Inland Waters of Argentina: A Review. Int. J. Salt Lake Res. 4, 315–325. doi:10.1007/BF01999115
Dreher-Mansur, M. C., and Garces, L. M. M. P. (1988). Ocorrencia e densidade de Corbicula fluminea (Mueller, 1774) e Neocorbicula limosa (Maton, 1811) na estacao ecologica Do Taim e areas adjacentes, Rio Grande Do Sul, Brasil (Mollusca, Bivalvia, Corbiculidae). Iheringia Ser. Zool. 68, 99–115. doi:10.7213/estud.biol.36.086.AO11
Dreher-Mansur, M. C., Valer, R. M., and Aires, N. C. M. (1994). Distribuição e preferências ambientais dos moluscos bivalves Do açude Do Parque de Proteção Ambiental COPESUL, município de Triunfo, Rio Grande Do Sul, Brasil. Biociências 2 (1), 27–45.
E. C. Lopretto, and G. Tell (Editors) (1995). Ecosistemas de aguas continentales: Metodologías para su estudio (La Plata: Ediciones Sur), 1401.
Epler, J. H. (2001). Identification Manual for the Larval Chironomidae (Diptera) of North and South Carolina. A Guide to the Taxonomy of the Midges of the southeastern United States, Including Florida. Raleigh, NC, and St. Johns River Water Management District, Palatka, FL: North Carolina Department of Environment and Natural Resources, 526. Special Publication SJ2001-SP13.
Essl, F., Lenzner, B., Bacher, S., Bailey, S., Capinha, C., Daehler, C., et al. (2020). Drivers of Future Alien Species Impacts: An Expert‐based Assessment. Glob. Change Biol. 26 (9), 4880–4893. doi:10.1111/gcb.15199
Fernández, H., and Domínguez, E. (2001). Guía para la determinación de los artrópodos bentónicos Sudamericanos. Entomotropica 16 (3), 219.
Ferreira-Rodríguez, N., Fernández, I., Varandas, S., Cortes, R., Cancela, M. L., and Pardo, I. (2017). The Role of Calcium Concentration in the Invasive Capacity of Corbicula Fluminea in Crystalline Basins. Sci. Total Environ. 580, 1363–1370. doi:10.1016/j.scitotenv.2016.12.100
Franco, J. N., Ceia, F. R., Patrício, J., Modesto, V., Thompson, J., Marques, J. C., et al. (2012). Population Dynamics of Corbicula Fluminea (Müller, 1774) in Mesohaline and Oligohaline Habitats: Invasion success in a Southern Europe Estuary. Estuarine, Coastal Shelf Sci. 112, 31–39. doi:10.1016/j.ecss.2011.07.014
Gardner, J. A., Woodall, W. R., Straats, A. A., and Napoli, J. F. (1976). The Invasion of the Asiatic Clam (Corbicula Manilensis Philippi) in the Altamaha River, Georgia. Nautilus 90, 117–125.
Gething, K. J., Ripley, M. C., Mathers, K. L., Chadd, R. P., and Wood, P. J. (2020). The Influence of Substrate Type on Macroinvertebrate Assemblages within Agricultural Drainage Ditches. Hydrobiologia 847 (20), 4273–4284. doi:10.1007/s10750-020-04416-6
Golterman, H. L., Clyno, R. S., and Ohsntad, M. A. M. (1978). Methods for Physical and Chemical Analysis of Freshwater. 2nd ed. Oxford: Blackwell. (JNP Handbook, 8).
Graça, M. A. S., Pinto, P., Cortes, R., Coimbra, N., Oliveira, S., Morais, M., et al. (2004). Factors Affecting Macroinvertebrate Richness and Diversity in Portuguese Streams: a Two-Scale Analysis. Internat. Rev. Hydrobiol. 89 (2), 151–164. doi:10.1002/iroh.200310705
Gutiérrez, J. L., Jones, C. G., Strayer, D. L., and Iribarne, O. O. (2003). Mollusks as Ecosystem Engineers: the Role of Shell Production in Aquatic Habitats. Oikos 101 (1), 79–90. doi:10.1034/j.1600-0706.2003.12322.x
Hakenkamp, C. C., and Palmer, M. A. (1999). Introduced Bivalves in Freshwater Ecosystems: the Impact of Corbicula on Organic Matter Dynamics in a sandy Stream. Oecologia 119 (3), 445–451. doi:10.1007/s004420050806
Hakenkamp, C. C., Ribblett, S. G., Palmer, M. A., Swan, C. M., Reid, J. W., and Goodison, M. R. (2001). The Impact of an Introduced Bivalve (Corbicula Fluminea ) on the Benthos of a sandy Stream. Freshw. Biol. 46 (4), 491–501. doi:10.1046/j.1365-2427.2001.00700.x
Heckman, C. W. (2006). Encyclopedia of South American Aquatic Insects: Odonata-Anisoptera: Illustrated Keys to Known Families, Genera, and Species in South America. Springer Science and Business Media.
Heckman, C. W. (2008). Encyclopedia of South American Aquatic Insects: Odonata-Zygoptera: Illustrated Keys to Known Families, Genera, and Species in South America. Springer Science and Business Media.
Hicks, G. (2004). Turning the Tide: Is Aquatic Bioinvaders Research Heading in the Right Direction? Aquat. Invaders 15, 9–20.
Hollander, M., and Wolfe, D. A. (1973). Nonparametric Statistical Methods. (one-sample). Or second edition (1999), 27–33. New York: John Wiley & Sons, 68–75. (two-sample).
Horvath, T. G., Martin, K. M., and Lamberti, G. A. (1999). Effect of Zebra Mussels, Dreissena polymorpha, on Macroinvertebrates in a lake-outlet Stream. The Am. Midland Naturalist 142 (2), 340–347. doi:10.1674/0003-0031(1999)142[0340:eozmdp]2.0.co;2
Howeth, J. G. (2017). Native Species Dispersal Reduces Community Invasibility by Increasing Species Richness and Biotic Resistance. J. Anim. Ecol. 86, 1380–1393. doi:10.1111/1365-2656.12733
Hubenov, Z., Trichkova, T., Kenderov, L., and Kozuharov, D. (2013). Distribution of Corbicula Fluminea (Mollusca: Corbiculidae) over an Eleven-Year Period of its Invasion in Bulgaria. Acta Zoologica Bulgarica 65 (3), 315–326.
Hutchinson, G. E. (1961). The Paradox of the Plankton. The Am. Naturalist 95, 137–145. doi:10.1086/282171
Jones, J. I., Murphy, J. F., Collins, A. L., Sear, D. A., Naden, P. S., and Armitage, P. D. (2012). The Impact of Fine Sediment on Macro-Invertebrates. River Res. Applic. 28 (8), 1055–1071. doi:10.1002/rra.1516
Jun, Y.-C., Kim, N.-Y., Kwon, S.-J., Han, S.-C., Hwang, I.-C., Park, J.-H., et al. (2011). Effects of Land Use on Benthic Macroinvertebrate Communities: Comparison of Two Mountain Streams in Korea. Ann. Limnol. - Int. J. Lim. 47 (1), S35–S49. doi:10.1051/limn/2011018
Karatayev, A. Y., Boltovskoy, D., Padilla, D. K., and Burlakova, L. E. (2007). The Invasive Bivalves Dreissena polymorpha and Limnoperna Fortunei: Parallels, Contrasts, Potential Spread and Invasion Impacts. J. Shellfish Res. 26, 205–213. doi:10.2983/0730-8000(2007)26[205:TIBDPA]2.0.CO;2
K. Grasshoff, K. Kremling, and M. Ehrhardt (Editors) (2009). Methods of Seawater Analysis (John Wiley & Sons).
Killeen, I. J., Aldridge, D. C., and Oliver, P. G. (2004). Freshwater Bivalves of the British Isles. Shrewsbury, United Kingdom: Occasional Publication, 82.
Larrañaga, S., Díez, J. R., Elosegi, A., and Pozo, J. (2003). Leaf Retention in Streams of the Agüera basin (Northern Spain). Aquat. Sci. 65 (2), 158–166. doi:10.1007/s00027-003-0623-3
Larsen, S., Pace, G., and Ormerod, S. J. (2011). Experimental Effects of Sediment Deposition on the Structure and Function of Macroinvertebrate Assemblages in Temperate Streams. River Res. Applic. 27 (2), 257–267. doi:10.1002/rra.1361
Libonatti, M. L., Michat, M. C., and Torres, P. L. M. (2011). Key to the Subfamilies, Tribes and Genera of Adult Dytiscidae of Argentina (Coleoptera: Adephaga). Revista de la Sociedad Entomológica Argentina 70 (3-4), 317–336.
Lodge, D. M. (1993). Biological Invasions: Lessons for Ecology. Trends Ecol. Evol. 8 (4), 133–137. doi:10.1016/0169-5347(93)90025-k
López-Soriano, J., Quiñonero-Salgado, S., Cappelletti, C., Faccenda, F., and Ciutti, F. (2018). Unraveling the Complexity of Corbicula Clams Invasion in Lake Garda (Italy). Adv. Ocean Limnol 9 (2). doi:10.4081/aiol.2018.7857
Macchi, P., Loewy, R. M., Lares, B., Latini, L., Monza, L., Guiñazú, N., et al. (2018). The Impact of Pesticides on the Macroinvertebrate Community in the Water Channels of the Río Negro and Neuquén Valley, North Patagonia (Argentina). Environ. Sci. Pollut. Res. 25 (11), 10668–10678. doi:10.1007/s11356-018-1330-x
Maloney, K., and Feminella, J. (2006). Evaluation of Single- and Multi-Metric Benthic Macroinvertebrate Indicators of Catchment Disturbance over Time at the Fort Benning Military Installation, Georgia, USA. Ecol. Indicators 6 (3), 469–484. doi:10.1016/j.ecolind.2005.06.003
Marçal, S. F., and Tasso-Callil, C. (2008). Structure of Invertebrates Community Associated with Eichhornia crassipes Mart.(Solms-Laubach) after the Introduction of Limnoperna Fortunei (Dunker, 1857) (Bivalvia, Mytilidae) in the Upper Paraguay River, MT, Brazil. Acta Limnologica Brasiliensia 20 (4), 359–371.
Mayer, C. M., Keats, R. A., Rudstam, L. G., and Mills, E. L. (2002). Scale-dependent Effects of Zebra Mussels on Benthic Invertebrates in a Large Eutrophic lake. J. North Am. Benthological Soc. 21 (4), 616–633. doi:10.2307/1468434
McKinney, M. L., and Lockwood, J. L. (1999). Biotic Homogenization: a Few Winners Replacing many Losers in the Next Mass Extinction. Trends Ecol. Evol. 14, 450–453. doi:10.1016/S0169-5347(99)01679-1
McMahon, R. F. (2002). Evolutionary and Physiological Adaptations of Aquatic Invasive Animals: R Selection versus Resistance. Can. J. Fish. Aquat. Sci. 59 (7), 1235–1244. doi:10.1139/f02-105
Merritt, R. W., and Cummins, K. W. (1996). Methods in Stream Ecology. San Diego: Academic Press, 453–474.Trophic Relations of Macroinvertebrates.
Michat, M. C., Archangelsky, M., and Bachmann, A. O. (2008). Generic Keys for the Identification of Larval Dytiscidae from Argentina (Coleoptera: Adephaga). Rev. Soc. Entomol. Argent 67 (3-4), 17–36.
Michat, M. C., and Archangelsky, M. (2007). Descriptions of Larvae of Desmopachria Babington (Coleoptera: Dytiscidae: Hydroporinae): the D. Vicina Sharp Species Group. Coleopterists Bull. 61 (2), 264–276. doi:10.1649/0010-065X(2007)61[264:DOLODB]2.0.CO;2
Miserendino, M. L. (2007). Macroinvertebrate Functional Organization and Water Quality in a Large Arid River from Patagonia (Argentina). Ann. Limnol. - Int. J. Lim. 43 (3), 133–145. doi:10.1051/limn:2007008
Modesto, V., Franco, J. N., Sousa, R., Patrício, J., Marques, J. C., and Neto, J. M. (2013). Spatial and Temporal Dynamics of Corbicula Fluminea (Muller, 1774) in Relation to Environmental Variables in the Mondego Estuary (Portugal). J. Molluscan Stud. 79 (4), 302–309. doi:10.1093/mollus/eyt026
Mörtl, M., and Rothhaupt, K.-O. (2003). Effects of AdultDreissena Polymorpha on Settling Juveniles and Associated Macroinvertebrates. Internat. Rev. Hydrobiol. 88 (6), 561–569. doi:10.1002/iroh.200310640
Muzón, J., Pessacq, P., and Lozano, F. (2014). The Odonata (Insecta) of Patagonia: A Synopsis of Their Current Status with Illustrated Keys for Their Identification. Zootaxa 3784 (4), 346–388. doi:10.11646/zootaxa.3784.4.2
Novais, A., Dias, E., and Sousa, R. (2016). Inter- and Intraspecific Variation of Carbon and Nitrogen Stable Isotope Ratios in Freshwater Bivalves. Hydrobiologia 765 (1), 149–158. doi:10.1007/s10750-015-2408-9
Olden, J. D., LeRoy Poff, N., Douglas, M. R., Douglas, M. E., and Fausch, K. D. (2004). Ecological and Evolutionary Consequences of Biotic Homogenization. Trends Ecol. Evol. 19 (1), 18–24. doi:10.1016/j.tree.2003.09.010
Olden, J. D., Lockwood, J. L., and Parr, C. L. (2011). Biological Invasions and the Homogenization of Faunas and Floras. Conservation Biogeogr., 224–243. doi:10.1002/9781444390001.ch9
Paschoal, L., Andrade, D., and Darrigran, G. (2015). How the Fluctuations of Water Levels Affect Populations of Invasive Bivalve Corbicula Fluminea (Müller, 1774) in a Neotropical Reservoir? Braz. J. Biol. 75, 135–143. doi:10.1590/1519-6984.09113
Paschoal, L. R. P., Andrade, D. D. P., and Darrigran, G. (2015). How the Fluctuations of Water Levels Affect Populations of Invasive Bivalve Corbicula Fluminea (Müller, 1774) in a Neotropical Reservoir? Braz. J. Biol. 75 (1), 135–143. doi:10.1590/1519-6984.09113
Pereira, D., Arruda, J. A., Bergonci, P. E. A., Oliveira, A. S., Postiglione, R., and Mansur, M. C. D. (2012). Como monitorar moluscos límnicos invasores bentônicos e macroinvertebrados associados. Moluscos límnicos invasores no Brasil. Biologia, prevenção, controle. Porto Alegre: Redes Editora, 155–184.
Platt, J. B. (2011). Habitat Complexity and Species Diversity in Rivers. Doctoral dissertation. Cardiff, United Kingdom: Cardiff University.
Ramírez, A., and Gutiérrez-Fonseca, P. E. (2014). Functional Feeding Groups of Aquatic Insect Families in Latin America: a Critical Analysis and Review of Existing Literature. Rbt 62, 155–167. doi:10.15517/rbt.v62i0.15785
Reid, H. W., McMahon, R. F., Foighil, D. Ó., and Finnigan, R. (1992). The Biology of a Fatal Herpesvirus Infection of Deer (Malignant Catarrhal Fever). The Veliger 35 (2), 93–100. doi:10.1007/978-1-4612-2782-3_26
Reshaid, Y., Cao, L., Brea, F., Blanche, M. O., Torres, S., and Darrigran, G. (2017). Variation in the distribution of Corbicula species (Mollusca: Bivalvia: Corbiculidae) after 25 years of its introduction in the Río de la Plata, Argentina. Zoologia (Curitiba) 34, 1–6. doi:10.3897/zoologia.34.e22181
Roditi, H. A., Strayer, D. L., and Findlay, S. E. G. (1997). Characteristics of Zebra Mussel (Dreissena polymorpha) Biodeposits in a Tidal Freshwater Estuary. fal 140 (2), 207–219. doi:10.1127/archiv-hydrobiol/140/1997/207
Rodrígues-Capítulo, A. (1992). Los Odonata de la República Argentina (Insecta). Fauna de agua dulce de la República Argentina 34 (1), 1–91.
Shannon, C. E., and Weaver, W. (1963). The Mathematical Theory of Communication. Normal, IL: Urban University Illinois Press, 125.
Sousa, R., Antunes, C., and Guilhermino, L. (2007). Species Composition and Monthly Variation of the Mollusca Fauna in the Freshwater Subtidal Area of the River Minho Estuary. Estuar. Coast. Shelf. S. 75, 75–100. doi:10.1016/j.ecss.2007.02.0210.1016/j.ecss.2007.02.020
Sousa, R., Antunes, C., and Guilhermino, L. (2008b). Ecology of the Invasive Asian Clam Corbicula fluminea (Müller, 1774) in Aquatic Ecosystems: An Overview. Ann. Limnol. - Int. J. Lim. 44 (2), 85–94. doi:10.1051/limn:2008017
Sousa, R., Guilhermino, L., and Antunes, C. (2005). Molluscan Fauna in the Freshwater Tidal Area of the River Minho Estuary, NW of Iberian Peninsula. Ann. Limnol. - Int. J. Lim. 41 (2), 141–147. doi:10.1051/limn/2005009
Sousa, R., Gutiérrez, J. L., and Aldridge, D. C. (2009). Non-indigenous Invasive Bivalves as Ecosystem Engineers. Biol. Invasions. 11 (10), 2367–2385. doi:10.1007/s10530-009-9422-7
Sousa, R., Novais, A., Costa, R., and Strayer, D. L. (2014). Invasive Bivalves in Fresh Waters: Impacts from Individuals to Ecosystems and Possible Control Strategies. Hydrobiologia 735, 233–251. doi:10.1007/s10750-012-1409-1
Sousa, R., Rufino, M., Gaspar, M., Antunes, C., and Guilhermino, L. (2008a). Abiotic Impacts on Spatial and Temporal Distribution of Corbicula Fluminea (Müller, 1774) in the River Minho Estuary, Portugalfluminea (Müller, 1774) in the River Minho Estuary, Portugal. Aquat. Conserv: Mar. Freshw. Ecosyst. 18 (1), 98–110. doi:10.1002/aqc.838
Strayer, D. L., Caraco, N. F., Cole, J. J., Findlay, S., and Pace, M. L. (1999). Transformation of Freshwater Ecosystems by Bivalves. BioScience 49 (1), 19–27. doi:10.2307/1313490
Taylor, R. W., and Hughart, R. C. (1981). The Freshwater Naiads of Elk River, West Virginia with a Comparison of Earlier Collections. Nautilus 95 (21), 25.
Ter Braak, C. J. (1992). Multidimensional Scaling and Regression. Stat. Applicata: Ital. J. Appl. Stat. 4, 577–586.
Uhde, V., Massoli, E., and Tasso-Callil, C. (2012). “Efeito Do macrofouling sobre a comunidade de invertebrados aquáticos,”. orgs. Editors Mcd. Mansur, C. P. Santos, D. Pereira, I. C. P. Paz, M. L. L. Zurita, M. T. R. Rodriguezet al. (Porto Alegre: Redes Editora), 235–241.Guia prático: moluscos límnicos invasores do Brasil: biologia, prevenção e controle.
Vannote, R. L., Minshall, G. W., Cummins, K. W., Sedell, J. R., and Cushing, C. E. (1980). The River Continuum Concept. Can. J. Fish. Aquat. Sci. 37 (1), 130–137. doi:10.1139/f80-017
Vaughn, C. C., and Hakenkamp, C. C. (2001). The Functional Role of Burrowing Bivalves in Freshwater Ecosystems. Freshw. Biol. 46 (11), 1431–1446. doi:10.1046/j.1365-2427.2001.00771.x
Vaughn, C. C., Nichols, S. J., and Spooner, D. E. (2008). Community and Foodweb Ecology of Freshwater Mussels. J. North Am. Benthological Soc. 27 (2), 409–423. doi:10.1899/07-058.1
Vaughn, C. C., and Spooner, D. E. (2006). Scale-dependent Associations between Native Freshwater Mussels and Invasive Corbicula. Hydrobiologia 568 (1), 331–339. doi:10.1007/s10750-006-0210-4
Wang, S., Loreau, M., de Mazancourt, C., Isbell, F., Beierkuhnlein, C., Connolly, J., et al. (2021). Biotic Homogenization Destabilizes Ecosystem Functioning by Decreasing Spatial Asynchrony. Ecology 102 (6), e03332. doi:10.1002/ecy.3332
Ward, J. M., and Ricciardi, A. (2007). Impacts of Dreissena Invasions on Benthic Macroinvertebrate Communities: a Meta‐analysis. Divers. Distrib. 13 (2), 155–165. doi:10.1111/j.1472-4642.2007.00336.x
Werner, S. (2014). Effects of the Invasive Asian Clam Corbicula Fluminea on the Littoral Communities of Lake Constance Dissertation. February. doi:10.1127/1863-9135/2008/0173-0145
Widdicombe, S., and Austen, M. C. (2001). The Interaction between Physical Disturbance and Organic Enrichment: an Important Element in Structuring Benthic Communities. Limnol. Oceanogr 46 (7), 1720–1733. doi:10.4319/lo.2001.46.7.1720
Wiederholm, T. (1989). Chironomidae of the Holarctic Region. Keys and Diagnoses. Part 3. Adult Males, Supplement. Lund, Sweden: Entomologica Scandinavica.34.
Williams, J. D., Warren, M. L., Cummings, K. S., Harris, J. L., and Neves, R. J. (1993). Conservation Status of Freshwater Mussels of the United States and Canada. Fisheries 18 (9), 6–22. doi:10.1577/1548-8446(1993)018<0006:csofmo>2.0.co;2
Wittmann, M. E., Chandra, S., Reuter, J. E., Caires, A., Schladow, S. G., and Denton, M. (2012). Harvesting an Invasive Bivalve in a Large Natural lake: Species Recovery and Impacts on Native Benthic Macroinvertebrate Community Structure in Lake Tahoe, USA. Aquat. Conserv: Mar. Freshw. Ecosyst. 22 (5), 588–597. doi:10.1002/aqc.2251
Wygodzinsky, P. W., and Coscarón, S. (1989). Revision of the Black Fly Genus Gigantodax (Diptera, Simuliidae). Bull. Am. Mus. Nat. Hist. 189, 269.
Yeager, M. M., Cherry, D. S., and Neves, R. J. (1994). Feeding and Burrowing Behaviors of Juvenile Rainbow Mussels, Villosa Iris (Bivalvia:Unionidae). J. North Am. Benthological Soc. 13 (2), 217–222. doi:10.2307/1467240
Zaiko, A., Daunys, D., and Olenin, S. (2009). Habitat Engineering by the Invasive Zebra Mussel Dreissena polymorpha (Pallas) in a Boreal Coastal Lagoon: Impact on Biodiversity. Helgol. Mar. Res. 63 (1), 85–94. doi:10.1007/s10152-008-0135-6
Zhang, Y., Liu, L., Cheng, L., Cai, Y., Yin, H., Gao, J., et al. (2014). Macroinvertebrate Assemblages in Streams and Rivers of a Highly Developed Region (Lake Taihu Basin, China). Aquat. Biol. 23 (1), 15–28. doi:10.3354/ab00600
Keywords: Corbicula fluminea, macroinvertebrate assemblages, homogenization, invasion, productivity, Patagonia
Citation: Labaut Y, Macchi PA, Archuby FM and Darrigran G (2021) Homogenization of Macroinvertebrate Assemblages and Asiatic Clam Corbicula fluminea Invasion in a River of the Arid Patagonian Plateau, Argentina. Front. Environ. Sci. 9:728620. doi: 10.3389/fenvs.2021.728620
Received: 21 June 2021; Accepted: 17 September 2021;
Published: 01 October 2021.
Edited by:
Eugenia López-López, Instituto Politécnico Nacional de México (IPN), MexicoReviewed by:
Marcos Callisto, Federal University of Minas Gerais, BrazilPieter Boets, Ghent University, Belgium
Copyright © 2021 Labaut, Macchi, Archuby and Darrigran. This is an open-access article distributed under the terms of the Creative Commons Attribution License (CC BY). The use, distribution or reproduction in other forums is permitted, provided the original author(s) and the copyright owner(s) are credited and that the original publication in this journal is cited, in accordance with accepted academic practice. No use, distribution or reproduction is permitted which does not comply with these terms.
*Correspondence: Yeny Labaut, eWxhYmF1dGJldGFuY291cnRAdW5ybi5lZHUuYXI=