- Department of Environmental Engineering, Marwadi University, Rajkot, India
The industrial intervention had a profound impact on our environment and the amount of damage it has bestowed is in the form of polluted water and solid waste accumulation. With upgradation of technologies in the industrial upfront there is also an increase in the robust nature of industrial waste. Keeping in view of the various environmental aspects, advanced techniques were developed by the inculcation of nanotechnology. Among the diverse technologies that have gained prominence is the use of nanoparticles as a medium to monitor and treatment process. One of them is the use of organic techniques, i.e., the use of nano-chitosan. The review article focuses on the new data pertaining to the study and improvement of various nano-scale treatment technologies implemented for wastewater treatment with an environment friendly biodegradation. The eradication of harmful toxicants is an exhaustive process, but this is required to invigorate the sustainable development. Various strategies were promulgated but the inculcation of nanomaterial provided a low-cost, efficient and simple method for removal of contaminants by adsorption. These nanomaterials provide the best adsorbent due to its simple structural properties that provide a better surface area with high absorption capacity. This comprehensive review gives a glimpse of the various green and other nanotechnologies used for wastewater and contaminated soil treatments.
Introduction
The word “nano” is Greek in origin, and it is used to describe one billionth of a meter. The term Nanotechnology was coined by Norio Taniguchi (1974) to describe the manufacturing of materials at the nanometre scale. Nanotechnology is a drastically growing research area with the utilization to implement nanoscale materials in the area of science and technology. It is a multidisciplinary scientific undertaking, involving creation and applications of materials, devices or systems that function at nanoscale. Nano phasic and nanostructured materials are drawing in more consideration because of its distinctness and selectivity related to biological and pharmaceutical applications (G.A. Mansoori, 2002). More than this it also caters to the pollution through various techniques that help in monitoring and assessing the environmental risk. It is likewise put on in cosmetics, electronic and energy associated utilities (Keller et al., 2013).
The synthesis of various Nano structures and particles are mainly categorized into two perspectives. The first is top to down perspective and second is bottom to top up perspective. Both the perspectives consist of various methods to produce nanoparticles. In the top–down perspective, particles are generated from the bulked (grouped) materials and in the bottom–up approach the nucleation sites are first formed then it grows into a nano sized particle. The examples of top-down (physical) approach are ball milling, laser ablation, evaporation–condensation, electromagnetic levitation gas condensation (ELGC), ultra-sonication, lithography, spray pyrolysis, radiolysis, arc discharge, and photo–irradiation. Physical approach is generally applied for high scale production in a minimal time (Sina and Shohreh, 2021). The bottom-up approach, however, relies mostly on the use of reducing and stabilizing agents to produce the various types of nanoparticles and its subcategories in organic and inorganic (it depends upon the use of reactive agents) methods. In this type of synthesis various methods like chemical vapour deposition, sol-gel process, spinning, pyrolysis, etc. are used (Ijaz et al., 2020). The biosynthesis of nanoparticles is done using microorganisms as an alternative for the conventional methods (i.e., physical and chemical methods). Prokaryotes and eukaryotes are applied for production of metallic nanoparticles (viz. silver, gold, platinum, zirconium, palladium, iron, cadmium) and metal oxides (such as titanium oxide, zinc oxide, etc). These microbial organisms incorporate bacteria, fungi, algae and actinomycetes. The synthesis of nanoparticles may be intracellular or extracellular as indicated by the place of nanoparticle development. Biosynthesis processes are environment friendly, effectual, economic, and scalable with desirable controlled features like size and morphology of nanoparticles (K. Rambabu et al., 2021).
Rapid population growth, industrialization, and urbanization have been associated with extensive use of various pollutant-containing products and by-products, these product manufacturing industries are omitting numerous wastes that lead to the accumulations in various ecosystems and environment (Meghdad and Hassan, 2021). World is suffering from the shortage of freshwater and the demand is continuously increasing day by day. It estimated that nearly 3.6 billion people scarce at least 1 month in a year (Syed and Waseem, 2021). Again, with fast populace development and growth of the urban areas, yearly waste production is projected to increase by 70% from 2016 levels to 3.40 billion tons in year 2050.
The most common method implemented for solid waste is through sanitary landfill. They generate large quantities of leachate; the term leachate is elucidated by the liquid that comes out of the landfill and extracted dissolved along with suspended matter. The overproduction of leachate often leads to harmful effects on humans and the environment. As a solution various nanotechnology-based processes are used to treat leachate like nano-filtration (Fadugba et al., 2021).
Wastewater treatment plants are used to remove the water-based pollutants from the industrial, sewage and other wastewater systems. The diverse range of nanoparticles made from noble metals are gold, silver etc. They are initially reproduced and utilized in catalytic reduction processes, which has a high surface to volume ratio and decipher a huge catalytic activity towards the reduction of numerous organic pollutants in wastewater treatment plants (Wenshuai et al., 2020).
In this mini-review, the implementation of green-synthesised nanoparticles used for wastewater and soil treatment are mentioned and their economical way to meet the need of renewable energy goal was also highlighted.
Classification of Nanoparticles
The classification of nanoparticles is based on its size, shape and morphology. Nanoparticles are commonly categorised into organic nanoparticles, inorganic nanoparticles, metal nanoparticles, metal oxides nanoparticles, ceramic nanoparticles and biological nanoparticles (bio nanoparticles) (Cristina and Ivan, 2016).
Organic Nanoparticles
Organic nanoparticles are biodegradable, non-toxic in nature, it includes micelles, dendrimers and ferritin. It may also have hollow spheres like micelles and liposome’s structure that are mostly used for drug delivery. It is also known as polymeric nanoparticles and nano-capsules. They have characteristic heat and light sensitive nature. It is mostly found in nanosphere and capsulated form. In this nanoparticle (matrix particles), the inner matrix part is solid and outer bounds of spherical surface has absorbed compounds, Nanoparticles are encapsulated in a solid mass (Muhammad et al., 2017).
Inorganic Nanoparticles
Inorganic nanoparticles don’t have carbon in their structure, so they are non-toxic as well as hydrophilic and biocompatible in nature. They have more stability compared to organic nanoparticles. Inorganic nanoparticles are categorised into metal and metal-oxide (Muhammad et al., 2017).
Metal Nanoparticles
The nanoparticles made from the metals are known as metal nanoparticles. Metal precursors are used in destructive or constructive manner to produce pure metal nanoparticles. It has unique opto-electrical effects due to plasma resonance property. The reproduction of metal nanoparticles is guided by size, shape and facet. The most common metal nanoparticles are silver, gold, iron, cobalt, zinc, copper, and cadmium nanoparticles. The size and shape control provide the various unique properties to metal nanoparticles like surface area to volume ratio, pore size, structure, surface charge and density, colour and environmental factors (e.g., sunlight, moisture, heat and air) (Erik et al., 2012).
Metal Oxide Nanoparticles
The main reason for the reproduction of metal oxide nanoparticles is to increase the reactivity of metal oxide nanoparticles compared to metal nanoparticles. The synthesis of metal oxide nanoparticles is modified in such a way that increases its reactivity. Most common metal oxide nanoparticles are iron oxide, zinc oxide, aluminium oxide, silicon dioxide, magnetite and titanium oxide (Clifford et al., 2007).
Ceramic Nanoparticles
The synthesis of ceramic nanoparticles is mostly carried out by heating or successive cooling. It is commonly known as nanometallic solid. It has porous, dense, amorphous, polycrystalline and hollow structures. It is generally applied for photocatalysis, catalysis, photodegradation and imaging (Shindu ae al., 2015).
Biological Nanoparticles (Bio-Nanoparticles)
This is an assemblage of nanoparticles fabricated using biological systems. It is naturally occurring nanoparticles. These nanoparticles are produced in extra or intra cellular regions in various organisms and are mainly synthesised by aiming towards particular applications. Bio nanoparticles are commonly found as lipoproteins, ferritin and magneto-some (Irfan et al., 2020).
Nano-Materials
Nano particles are also used in a structural way by constructing Nano-material. It has a structural component (or unit) less than one micro-meter in at least one dimension. Composition blocks 0.2 nm of matter are accounted as nano-material. The nanomaterials have different types e.g., carbon based nano-materials, fullerene, carbon nanotube (single, double and multi walled), carbon black, carbon nano-fiber, graphene etc. Nano-materials can be further arranged on the foundation of their dimensions:
A. One dimensional nano-materials: The thin film size ranges from 1 to 100 nm.
B. Two dimensional nano-materials: The nano-structures have two dimensions, in which outside the size range of nano-metric with peculiar shape and 2D nano-materials are used as building blocks for key components of nano devices, e.g., carbon nanotubes (Jibowu, 2016).
C. Three dimensional nano-materials: the various layers, shapes and morphological nanomaterials are constructed in the defined way to make three dimensional nano-materials. (Sreelakshmy et al., 2016).
Green Synthesis
There are several procedures for green-synthesis of metallic nanoparticles utilizing various solvents, reacting and capping agents. The biomolecules available in the plants such as flavones, terpenoids, ketones, alkaloids, aldehydes, vitamins, proteins, amino acids, tannins, phenolics, saponins, and polysaccharides assume an imperative part in reduction of metals (Vijayaraghavan and Ashokkumar, 2017).
The simple way to produce nanoparticles by various plant’s part is as per below diagram (Figure 1). It may vary from plant to plant and for technique pertaining to synthesis, most of the green synthesis methods are as depicted in Figure 2.
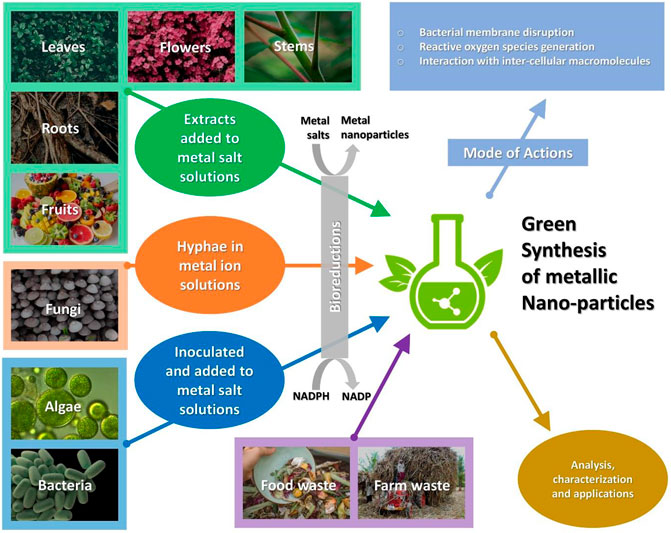
FIGURE 2. Green synthesis of metallic nanoparticles from various sources, mode of actions and further processes.
As mentioned in the above Table 1, the green synthesis process is economical in nature as per the selection of source materials like tea waste, peels of fruits and other organic residues. The economical way to produce the NPs is performed best by the organic waste but it is also important to accept the media and capping agent. The application of these nanoparticles in various fields is an important concern as they are used in traditional medicinal treatment also and in-depth analysis needs to be done as the by-products after treatment will pose risk for the native systems.
Use of Waste Materials as Nanoparticle Production and Application
Waste materials from the different sources like the industries or consumer is the highlighted problem, waste may have value added materials or basic structures that can be utilized to produce the nanoparticles e.g., waste from the rubber/tires, large batteries, wastewater, bio-solids etc. contains the carbon, copper, zinc, lead that can be potential source for the nanoparticles construction. Added to this consumer’s waste may also contain different metallic compounds which can be recovered by various methods and as an end product we can get a good amount of metallic nanoparticles or composite materials (Table 2). The various physical and chemical methods like reduction with sodium borohydride, solvent thermal method with grinding and milling pre-treatment (Sabad et al., 2020).
Carbon Nanotubes (CNT) can be fabricated from plastic wastes after the application of autoclaves, crucible, muffle furnace and quartz tube. These methods disintegrate solid polymers into carbon precursors by pyrolysis. Other than this, polyethylene, polyvinyl alcohol, polypropylene and polyethylene terephthalate can also be utilized for the production of carbon nanotubes (Deng et al., 2016).
Graphene can also be reproduced by numerous plastic waste applying methods like waste polypropylene catalysed by montmorillonite than conversion of carbonized char and dissolved it with HNO3. The amorphous carbon can be obtained and repeated processes of same can give graphene flakes after the centrifugation and isolation process (Gong et al., 2014).
The mode of action approach of various nanoparticles changes with the native environment as the reaction, the medium and the characteristics alters with the availability of resources.
Silver nanoparticles generated from green synthesis using the neem leaf and banana peel is useful for the dye degradation and antimicrobial activity. The potential effects for dye degradation are also effectively applied for the hazardous dye removal (Ahana and Agana, 2021).
Nanoparticles made from gum acacia and copolymer nanocomposite magnetite nanoparticles were used as an adsorbent for the aqueous solution in the wastewater treatment plant and effluent showed the removal of the different magnetic property containing waste materials (Prajwall et al., 2021).
ZnO nanoparticle synthesized from the peel of Passiflora foetida fruit uplifted the removal percentage of methylene blue (93.25%) and Rhodamine B (91.06%) after 70 min of application of nanoparticles (Mujahid et al., 2021). The metallic nanoparticles have potential for removal of the antibiotic resistant bacteria and antibiotic resistant genes from wastewater treatment facilities (Adaora et al., 2021).
Nanoparticles function as a reducing agent and work as an antimicrobial catalyst for the detoxification of pollutants like organic, inorganic, and heavy metals. It’s environment friendly nature, cost effectiveness and potential reaction capability gives an extra edge over the available treatment materials (Zaidi Ab et al., 2015).
Chitosan Nanoparticles: An Exemplary Green Nanomaterial
Chitosan as an Adsorbent
In 1859, Chitosan was initially synthesized by Professor C. Rouget. It is a bioremediable polysaccharide synthesized from chitin deacetylation (with a basic structure of β-1, 4-linked two amino-2-deoxy-d-glucose). Amine group, primary and secondary hydroxyl groups are the three functional groups help in the chemical adsorption of ionic or polar impurities on the outer layer of chitosan. Viscosity, dissolvability in different solvents, polyelectrolytic nature, polyoxysalt development, mucoadhesive behaviour, capacity to frame metal chelates and films are some of the special properties of Chitosan, which make it better for its widespread use in food, agriculture, pharmacy, cosmetics industry etc. The most important factor for using chitosan lies in its properties like biodegradability, biocompatibility and economically feasible which makes it more useful for biomedical and bioremediation (Kaur and Dhillon, 2014). Because of the non-toxic, biocompatible, and biodegradable properties, the use of chitosan has increased. It is used in different aspects like biomedical, food, pesticide industries and also for the treatment of water. The good adsorbent properties help in removing the hazardous contaminations from wastewater like metal ions and organic contaminants such as dyes, pesticides and pharmaceutical residues in water and help in its treatment.
Because of its constructional characteristics and amine presence along with hydroxyl functionalities, the chitosan shows many benefits over different adsorbents. Being economical and disposed as waste it is often found abundantly as natural resources. Since, it is not poisonous or toxic and easily bio-remediable, therefore it is better for the environment. The adsorbent quality is better as it can adsorb different sorts of contaminations existing in wastewater. Though it has several advantages to be used as adsorbents still it is not widely used as it has some drawbacks in the form of low mechanical strength, less adsorption property, substantially low stability in acidic medium and negligible specificity. To improvise and enhance the stability as well as sensitivity the chitosan can be modified physically by changing it into different forms and chemically by crosslinking and grafting (Olivera et al., 2016).
Nanomaterials From Chitosan Used for Removal of Dyes
Chitosan nanoparticles possess relatively larger surface area and higher adsorption potency. These two qualities make it an effective adsorbent material for the decomposition of various dyes in textile wastewater.
The capacity to adsorb and select of the chitosan nanoparticles is further enhanced when surface is modified by some functional groups or by an appropriate grafting compound. The advantage of using magnetic chitosan lies in the simplicity of segregation done via a magnet and have better recyclability.
Tanhaei et al. prepared concoction nanoparticles of chitosan/Al2O3/magnetic iron oxide and applied it for the removal of methyl orange dye. Apart from this the researchers have also prepared simple magnetic chitosan. The magnetic chitosan is good adsorbent for the dye removal, and it was further seen through the experiment results for methyl orange removal.
Glutaraldehyde crosslinked magnetic chitosan nanocomposite prepared by reduction precipitation process are often applied for the elimination of Acid Red 2 and it gives an adsorption potency of 90.06 mg g−1. The production process does not involve use of any hazardous chemicals or extreme heat. The mechanism of adsorption is established on electrostatic interaction of anionic Acid Red 2 dye. This has a positive surface charge for magnetic chitosan nanocomposite and occurs as a consequence of protonation of amine group (Kadam and Lee, 2015).
Nanomaterials Made From Chitosan in Removal of Pesticides
The barriers in the crop productivity are the pests, weeds and harmful substances disposed on the land. In order to eradicate these problems pesticides are used but there is also a problem when these are used as they leach out in the nearby water bodies and promulgates rapid algal growth. Being toxic they often harm the aquatic ecosystem so they need to be removed from the environment with the help of adsorbents. The chitosan nanoparticles are used for the removal of pesticides is the new avenue where very few researchers have worked upon. In a study conducted by Dehaghi et al., 2014, they used the chitosan mixed with zinc oxide nanoparticles to remove the pests.
Chitosan Infused Nanomaterials for the Removal of Other Organic Pollutants
The nanomaterials infused with chitosan have given very good results for the elimination of pollutants and many researchers have applied the nanochitosan for the eradication of polyaromatic hydrocarbons (pH 5, 30°C) (Tahvildari and Mojahedi, 2016).
The magnetic chitosan nanoparticle was prepared by one-step in situ co-precipitation technique. The Quasi-spherical shaped nanoparticles are formed with size 10 nm. The magnetic chitosan nanoparticles prepared removed humic acid from water solution at pH 7 and 25°C. It has a reclaimable property which helps it to be used for a long term (Dong et al., 2014). The capacity to adsorb for the chitosan-based nanoparticles for organic pollution has been widely used due to its effective sorbent properties, economic feasibility and biodegradable nature which is a boon to the environment and its dwellers.
The Use of Nanotechnology for Pesticides and POP Remediation From Soil
The soil pollution is prevalent through the unsurmountable use of pesticides and persistent organic pollutants (POPs) by human activities that lead to the restriction of chemical pollutants use in the Stockholm convention on persistent pollutants (POPs) held in 2004. With the awareness through convention, there was a widespread need to wipe out these contaminants persistent in the soil via proper bioremediation and administration of contaminated soil areas that has been disrupted due to deposition of toxic chemical pollutants. There are eight pesticides out of the total 12 banned POP chemicals and the rest are either from factory-exhaust chemicals and pharmaceuticals (Yang et al., 2008; Loos et al., 2009; Abhilash et al., 2013; Allinson et al., 2016). The biomagnification and bioaccumulation of these toxic compounds pose a greater risk for the cycle of food chain and more persistent in the tertiary consumers so there is a need to remove these pollutants at the initial stage itself. Since these POPs are lipophilic, they tend to deposit as fatty tissues in the living beings and pose an acute and chronic risk. Many pesticides have recently been banned as they pose threats to the health even after it has been abandoned for many years. The problem with POPs arises when they are prevalent by the means of vaporization and are settled at the site far from the origin. The recalcitrant nature of the POPs tends to contaminate the soil for decades and they take several years to degrade from the environment. Photocatalysis is the means for degradation of these pesticide pollutants. The nanoparticles in the process of photolysis acts as catalyst and go through response with chemical contaminants i.e., pesticides and POPs. These nanophotocatalysts transform the hazardous persistent pollutants into simple compounds such as CO2, N2, and H2O. According to Fujishima et al., 2008, the nanoscale titanium dioxide (TiO2) and zinc oxide (ZnO) are better photocatalysts and it was further elucidated by Yu et al., 2007, by his study on the degradation of organochlorine pesticides such as α-, β-, γ-, and δ-hexachlorobenzene; dicofol; and cypermethrin through use of nano-TiO2. The pesticides with nano-TiO2 on its surface attract the peroxide or hydroxyl radicle and helps in electron transfer. The photocatalytic degradation of organophosphorus and carbamate with the use of nano-TiO2 with rhenium (Re+3) have been reported for the leaves of tomato plants (Rui et al., 2010) and around 15–30% photocatalytic degradation was found for organophosphorus and carbamate pesticides. The use of nano-TiO2 for photocatalysis is also found effective in reducing the half-lives of pesticides as defined by Gogos et al., 2012.
The application of carbon nanofibers has expedited as they provide complete mineralization of metolachlor which is used as an herbicide and are capable of giving rise to innumerable by-products in the form of organic acids and aromatic compounds which are harmful and persistent.
The nano zerovalent iron (nZVI) has a better degradative effect on the soil contaminated with Dichlorodiphenyltrichloroethane (DDT). The degradation rate was found around 50% for DDT induced soil and 24% for POP polluted soil.
The application of nZVI has conducted on remediation of soil contaminated with organochlorine (Tilston et al., 2013). The disturbance on soil bacterial community after application of nZVI has been noticed along with the depletion in pursuit of chloroaromatic mineralizing microbial organisms.
The initiation of photodegradation of malathion was done with the incorporation of the semiconductor synthesized nanoparticles attached with various metal core-shell nanocomposites such as TiO2, Au/TiO2, ZnO, and Au/ZnO as they acted as catalyst. The prepared nanocomposite with a coalescence of metal core and semiconductor further improvised the photocatalytic effect and boosted the malathion decomposition in a stipulated time (Fouad and Mohamed, 2011).
The effect of nano-TiO2(anatase) on photocatalytic decomposition of phenanthrene (a polyaromatic hydrocarbon) was studied by Gu, et al., 2012. They found that half-life of phenanthrene was brought down by 14 h (from 46 to 31 h) when TiO2 was loaded at 0 and 4 percentage weight. A significant degradation process was witnessed on the soil surfaces when TiO2 was used. The factors such as the concentration of H2O2, intensity of light and humic acid enhanced the degradation process as depicted in Table 3.
Conclusion
The eco-friendly synthesis through nanoparticles (NPs) offers clean, non-toxic, relatively cost-effective, and environmentally sustainable procedures. Nanotechnology promulgates an upgraded and innovative initiative to fabricate and analyse various novel formulations based on metallic NPs that have tremendous prospect in diverse areas. Various nanoparticles and their prospective applications have been discussed in this review. The increasing threats to human health and the surround emancipated by the NPs are a concern therefore the studies are conducted in order to characterize the toxicity and mechanisms of NPs. The contemporary use of NPs with added advantages has been an important and efficient efforts to improvise the synthesis efficiency. The effective use of chitosan as adsorbent nanomaterial has led to the low-cost treatment for the water apart from the augmentation of antibacterial properties.
The implementation and utilization of these technological approaches for scale up and the commercial applications in the various fields will be upheld in the coming years. In the upcoming years the promulgation of nanotechnology will be useful for the replacement for the present precarious means as it will be eradicated the harmful contaminates from the soil and preserve the environmental sanctity. The remediation with the inculcation of nanotechnology has showed tremendous possibilities for diminishing the total cost and reduction of time allocated for replenishing as well as restoring the contaminated niche on a large scale. The onsite remediation reduces the overall cost for transport, processing and soil dumping afterwards the area has been replenished. The long-term effects due to the use of nanoparticles is still under the cynosure as proper evaluation needs to be done before forging ahead with large scale implementation to curb the future potential environmental effects.
Author Contributions
All authors listed have made a substantial, direct, and intellectual contribution to the work and approved it for publication.
Conflict of Interest
The authors declare that the research was conducted in the absence of any commercial or financial relationships that could be construed as a potential conflict of interest.
Publisher’s Note
All claims expressed in this article are solely those of the authors and do not necessarily represent those of their affiliated organizations, or those of the publisher, the editors and the reviewers. Any product that may be evaluated in this article, or claim that may be made by its manufacturer, is not guaranteed or endorsed by the publisher.
References
Abdelbasir, S. M., McCourt, K. M., Lee, C. M., and Vanegas, D. C. (2020). Waste-Derived Nanoparticles: Synthesis Approaches, Environmental Applications, and Sustainability Considerations. Front. Chem. 8, 782. doi:10.3389/fchem.2020.00782
Abdul Rahim Arifin, A. S., Ismail, I., Abdullah, A. H., Shafiee, F. N., Nazlan, R., and Ibrahim, I. R. (2017). Iron Oxide Nanoparticles Derived from Mill Scale Waste as Potential Scavenging Agent in Dye Wastewater Treatment for Batik Industry. Solid State Phenomena 268, 393–398. doi:10.4028/www.scientific.net/ssp.268.393
Abhilash, P. C., Dubey, R. K., Tripathi, V., Srivastava, P., Verma, J. P., and Singh, H. B. (2013). Remediation and Management of POPs-Contaminated Soils in a Warming Climate: Challenges and Perspectives. Environ. Sci. Pollut. Res. 20 (8), 5879–5885. doi:10.1007/s11356-013-1808-5
Ahmed, S. N., and Haider, W. (20212021). Enhanced Photocatalytic Activity of ZnO-Graphene Oxide Nanocomposite by Electron Scavenging. Catalysts 11, 187. doi:10.3390/catal11020187
Allinson, G., Allinson, M., Bui, A., Zhang, P., Croatto, G., Wightwick, A., et al. (2016). Pesticide and Trace Metals in Surface Waters and Sediments of Rivers Entering the Corner Inlet marine National Park, Victoria, Australia. Environ. Sci. Pollut. Res. 23 (6), 5881–5891. doi:10.1007/s11356-015-5795-6
Argaw, N. (2003). Renewable Energy in Water and Wastewater Treatment Applications; Period of Performance: April 1, 2001--September 1, 2001 (No. NREL/SR-500-30383). Golden, CO (United States): National Renewable Energy Lab.(NREL.
Arora, S., and Saraswat, S. (2021). Vermifiltration as a Natural, Sustainable and green Technology for Environmental Remediation: A New Paradigm for Wastewater Treatment Process. Curr. Res. Green Sust. Chem. 4, 100061. doi:10.1016/j.crgsc.2021.100061
Bakshi, M., and Abhilash, P. C. (2020). Nano-Materials as Photocatalysts for Degradation of Environmental Pollutants. Elsevier, 345–370. doi:10.1016/b978-0-12-818598-8.00017-1Nanotechnology for Soil Remediation: Revitalizing the Tarnished Resource
Benassai, E., Del Bubba, M., Ancillotti, C., Colzi, I., Gonnelli, C., Calisi, N., et al. (2021). Green and Cost-Effective Synthesis of Copper Nanoparticles by Extracts of Non-edible and Waste Plant Materials from Vaccinium Species: Characterization and Antimicrobial Activity. Mater. Sci. Eng. C 119, 111453. doi:10.1016/j.msec.2020.111453
Buzea, C., and Pacheco, I. (2017). EMR/ESR/EPR Spectroscopy for Characterization of Nanomaterials. New Delhi: Springer, 3–45. doi:10.1007/978-81-322-3655-9_1Nanomaterials and Their Classification
Chen, X., Yao, X., Yu, C., Su, X., Shen, C., Chen, C., et al. (2014). Hydrodechlorination of Polychlorinated Biphenyls in Contaminated Soil from an E-Waste Recycling Area, Using Nanoscale Zerovalent Iron and Pd/Fe Bimetallic Nanoparticles. Environ. Sci. Pollut. Res. 21 (7), 5201–5210. doi:10.1007/s11356-013-2089-8
Deng, J., You, Y., Sahajwalla, V., and Joshi, R. K. (2016). Transforming Waste into Carbon-Based Nanomaterials. Carbon 96, 105–115. doi:10.1016/j.carbon.2015.09.033
Devatha, C. P., Thalla, A. K., and Katte, S. Y. (2016). Green Synthesis of Iron Nanoparticles Using Different Leaf Extracts for Treatment of Domestic Waste Water. J. Clean. Prod. 139, 1425–1435. doi:10.1016/j.jclepro.2016.09.019
Dong, C., Chen, W., Liu, C., Liu, Y., and Liu, H. (2014). Synthesis of Magnetic Chitosan Nanoparticle and its Adsorption Property for Humic Acid from Aqueous Solution. Colloids Surf. A: Physicochemical Eng. Aspects 446, 179–189. doi:10.1016/j.colsurfa.2014.01.069
Dreaden, E. C., Alkilany, A. M., Huang, X., Murphy, C. J., and El-Sayed, M. A. (2012). The golden Age: Gold Nanoparticles for Biomedicine. Chem. Soc. Rev. 41 (7), 2740–2779. doi:10.1039/c1cs15237h
El Essawy, N. A., Ali, S. M., Farag, H. A., Konsowa, A. H., Elnouby, M., and Hamad, H. A. (2017). Green Synthesis of Graphene from Recycled PET Bottle Wastes for Use in the Adsorption of Dyes in Aqueous Solution. Ecotoxicology Environ. Saf. 145, 57–68. doi:10.1016/j.ecoenv.2017.07.014
Ezeuko, A. S., Ojemaye, M. O., Okoh, O. O., and Okoh, A. I. (2021). Potentials of Metallic Nanoparticles for the Removal of Antibiotic Resistant Bacteria and Antibiotic Resistance Genes from Wastewater: A Critical Review. J. Water Process Eng. 41, 102041. doi:10.1016/j.jwpe.2021.102041
Fadugba, O. G., Doluyemi-Ayibiowu, B., Suffianyusoff, M., and Iwakin, O. M. (2021). Application Of Nanotechnology In Leachate Treatment (A Critical Review).
Fouad, D. M., and Mohamed, M. B. (2011). Studies on the Photo-Catalytic Activity of Semiconductor Nanostructures and Their Gold Core-Shell on the Photodegradation of Malathion. Nanotechnology 22 (45), 455705. doi:10.1088/0957-4484/22/45/455705
Gogos, A., Knauer, K., and Bucheli, T. D. (2012). Nanomaterials in Plant protection and Fertilization: Current State, Foreseen Applications, and Research Priorities. J. Agric. Food Chem. 60 (39), 9781–9792. doi:10.1021/jf302154y
Gong, J., Liu, J., Jiang, Z., Feng, J., Chen, X., Wang, L., et al. (2014). Striking Influence of Chain Structure of Polyethylene on the Formation of Cup-Stacked Carbon Nanotubes/carbon Nanofibers under the Combined Catalysis of CuBr and NiO. Appl. Catal. B: Environ. 147, 592–601. doi:10.1016/j.apcatb.2013.09.044
Gu, J., Dong, D., Kong, L., Zheng, Y., and Li, X. (2012). Photocatalytic Degradation of Phenanthrene on Soil Surfaces in the Presence of Nanometer Anatase TiO2 under UV-Light. J. Environ. Sci. 24 (12), 2122–2126. doi:10.1016/s1001-0742(11)61063-2
Ijaz, I., Gilani, E., Nazir, A., and Bukhari, A. (2020). Detail Review on Chemical, Physical and green Synthesis, Classification, Characterizations and Applications of Nanoparticles. Green. Chem. Lett. Rev. 13 (3), 223–245. doi:10.1080/17518253.2020.1802517
Jibowu, T. (2016). The Formation of Doxorubicin Loaded Targeted Nanoparticles Using Nanoprecipitation, Double Emulsion and Single Emulsion for Cancer Treatment. J. Nanomed Nanotechnol 7 (379), 2.
Kaabipour, S., and Hemmati, S. (2021). A Review on the green and Sustainable Synthesis of Silver Nanoparticles and One-Dimensional Silver Nanostructures. Beilstein J. Nanotechnol. 12 (1), 102–136. doi:10.3762/bjnano.12.9
Kadam, A. A., and Lee, D. S. (2015). Glutaraldehyde Cross-Linked Magnetic Chitosan Nanocomposites: Reduction Precipitation Synthesis, Characterization, and Application for Removal of Hazardous Textile Dyes. Bioresour. Techn. 193, 563–567. doi:10.1016/j.biortech.2015.06.148
Katata-Seru, L., Moremedi, T., Aremu, O. S., and Bahadur, I. (2018). Green Synthesis of Iron Nanoparticles Using Moringa Oleifera Extracts and Their Applications: Removal of Nitrate from Water and Antibacterial Activity against Escherichia coli. J. Mol. Liquids 256, 296–304. doi:10.1016/j.molliq.2017.11.093
Kaur, S., and Dhillon, G. S. (2014). The Versatile Biopolymer Chitosan: Potential Sources, Evaluation of Extraction Methods and Applications. Crit. Rev. Microbiol. 40, 155–175. doi:10.3109/1040841x.2013.770385
Keller, A. A., McFerran, S., Lazareva, A., and Suh, S. (2013). Global Life Cycle Releases of Engineered Nanomaterials. J. nanoparticle Res. 15 (6), 1–17. doi:10.1007/s11051-013-1692-4
Khan, M., Ware, P., and Shimpi, N. (2021). Synthesis of ZnO Nanoparticles Using Peels of Passiflora Foetida and Study of its Activity as an Efficient Catalyst for the Degradation of Hazardous Organic Dye. SN Appl. Sci. 3 (5), 1–17. doi:10.1007/s42452-021-04436-4
Krishnaswamy, K., Vali, H., and Orsat, V. (2014). Value-adding to Grape Waste: Green Synthesis of Gold Nanoparticles. J. Food Eng. 142, 210–220. doi:10.1016/j.jfoodeng.2014.06.014
Kulal, P., Krishnappa, P. B., and Vishalakshi, B. (2021). Development of Gum acacia Based Magnetic Nanocomposite Adsorbent for Wastewater Treatment.
Kumar, H., Bhardwaj, K., Kuča, K., Kalia, A., Nepovimova, E., Verma, R., et al. (2020). Flower-based green Synthesis of Metallic Nanoparticles: Applications beyond Fragrance. Nanomaterials 10 (4), 766. doi:10.3390/nano10040766
Lee, H., Kim, B. H., Park, Y. K., Kim, S. J., and Jung, S. C. (20152015). Application of Recycled Zero-Valent Iron Nanoparticle to the Treatment of Wastewater Containing Nitrobenzene. J. Nanomater. doi:10.1155/2015/392537
Loos, R., Gawlik, B. M., Locoro, G., Rimaviciute, E., Contini, S., and Bidoglio, G. (2009). EU-wide Survey of Polar Organic Persistent Pollutants in European River Waters. Environ. Pollut. 157 (2), 561–568. doi:10.1016/j.envpol.2008.09.020
Mansha, M., Khan, I., Ullah, N., and Qurashi, A. (2017). Synthesis, Characterization and Visible-Light-Driven Photoelectrochemical Hydrogen Evolution Reaction of Carbazole-Containing Conjugated Polymers. Int. J. Hydrogen Energ. 42 (16), 10952–10961. doi:10.1016/j.ijhydene.2017.02.053
Mansoori, G. A. (2002). Advances in Atomic & Molecular Nanotechnology. UN-APCTT Tech Monitor, 53–59.
Moradi Dehaghi, S., Rahmanifar, B., Moradi, A. M., and Azar, P. A. (2014). Removal of Permethrin Pesticide from Water by Chitosan-Zinc Oxide Nanoparticles Composite as an Adsorbent. J. Saudi Chem. Soc. 18, 348–355. doi:10.1016/j.jscs.2014.01.004
Nayak, P., Kumar, S., Sinha, I., and Singh, K. K. (2019). ZnO/CuO Nanocomposites from Recycled Printed Circuit Board: Preparation and Photocatalytic Properties. Environ. Sci. Pollut. Res. 26 (16), 16279–16288. doi:10.1007/s11356-019-04986-6
Olivera, S., Muralidhara, H. B., Venkatesh, K., Guna, V. K., Gopalakrishna, K., and Kumar K., Y. (2016). Potential Applications of Cellulose and Chitosan Nanoparticles/composites in Wastewater Treatment: A Review. Carbohydr. Polym. 153, 600–618. doi:10.1016/j.carbpol.2016.08.017
Qing, W., Chen, K., Wang, Y., Liu, X., and Lu, M. (2017). Green Synthesis of Silver Nanoparticles by Waste tea Extract and Degradation of Organic Dye in the Absence and Presence of H2O2. Appl. Surf. Sci. 423, 1019–1024. doi:10.1016/j.apsusc.2017.07.007
R. Ali, H., and Hassaan, M. A. (2017). Applications of Bio-Waste Materials as green Synthesis of Nanoparticles and Water Purification. Am 6 (5), 85–101. doi:10.11648/j.am.20170605.16
Rambabu, K., Bharath, G., Banat, F., and Show, P. L. (2021). Green Synthesis of Zinc Oxide Nanoparticles Using Phoenix Dactylifera Waste as Bioreductant for Effective Dye Degradation and Antibacterial Performance in Wastewater Treatment. J. Hazard. Mater. 402, 123560. doi:10.1016/j.jhazmat.2020.123560
Restivo, J., Órfão, J. J. M., Armenise, S., Garcia-Bordejé, E., and Pereira, M. F. R. (2012). Catalytic Ozonation of Metolachlor under Continuous Operation Using Nanocarbon Materials Grown on a Ceramic Monolith. J. Hazard. Mater. 239-240, 249–256. doi:10.1016/j.jhazmat.2012.08.073
Rovani, S., Santos, J. J., Corio, P., and Fungaro, D. A. (2018). Highly Pure Silica Nanoparticles with High Adsorption Capacity Obtained from Sugarcane Waste Ash. ACS omega 3 (3), 2618–2627. doi:10.1021/acsomega.8b00092
Rui, Z., Jingguo, W., Jianyu, C., Lin, H., and Kangguo, M. (2010). Photocatalytic Degradation of Pesticide Residues with RE3+-Doped Nano-TiO2. J. Rare Earths 28, 353–356.
Saratale, R. G., Saratale, G. D., Shin, H. S., Jacob, J. M., Pugazhendhi, A., Bhaisare, M., et al. (2018). New Insights on the green Synthesis of Metallic Nanoparticles Using Plant and Waste Biomaterials: Current Knowledge, Their Agricultural and Environmental Applications. Environ. Sci. Pollut. Res. 25 (11), 10164–10183. doi:10.1007/s11356-017-9912-6
Saxena, R., Saxena, M., and Lochab, A. (2020). Recent Progress in Nanomaterials for Adsorptive Removal of Organic Contaminants from Wastewater. ChemistrySelect 5 (1), 335–353. doi:10.1002/slct.201903542
Sengupta, A., and Sarkar, A. (2021). Synthesis and Characterization of Nanoparticles from Neem Leaves and Banana Peels: a green prospect for Dye Degradation in Wastewater. Ecotoxicology, 1–12. doi:10.1007/s10646-021-02414-5
Sheikhi, M., and Rezaei, H. (2021). Adsorption of Hexavalent Chromium Ions from Aqueous Solutions Using Nano-Chitin: Kinetic, Isotherms and Thermodynamic Studies. Water Pract. Techn. 16 (2), 436–451. doi:10.2166/wpt.2021.007
Sreelakshmy, V., Deepa, M. K., and Mridula, P. (2016). Green Synthesis of Silver Nanoparticles from Glycyrrhiza Glabra Root Extract for the Treatment of Gastric Ulcer. J. Develop Drugs 5 (152), 2.
Tai, C. Y., Tai, C.-T., Chang, M.-H., and Liu, H.-S. (2007). Synthesis of Magnesium Hydroxide and Oxide Nanoparticles Using a Spinning Disk Reactor. Ind. Eng. Chem. Res. 46 (17), 5536–5541. doi:10.1021/ie060869b
Tanhaei, B., Ayati, A., Lahtinen, M., and Sillanpää, M. (2015). Preparation and Characterization of a Novel chitosan/Al2O3/magnetite Nanoparticles Composite Adsorbent for Kinetic, Thermodynamic and Isotherm Studies of Methyl Orange Adsorption. Chem. Eng. J. 259, 1–10. doi:10.1016/j.cej.2014.07.109
Tavker, N., Yadav, V. K., Yadav, K. K., Cabral-Pinto, M. M., Alam, J., Shukla, A. K., et al. (2021). Removal of Cadmium and Chromium by Mixture of Silver Nanoparticles and Nano-Fibrillated Cellulose Isolated from Waste Peels of Citrus Sinensis. Polymers 13 (2), 234. doi:10.3390/polym13020234
Thai, Q. B., Le, D. K., Luu, T. P., Hoang, N., Nguyen, D., and Duong, H. M. (2019). Aerogels from Wastes and Their Applications. JOJ Mater. Sci. 5 (555663), 10–19080.
Thomas, S., Harshita, B. S. P., Mishra, P., and Talegaonkar, S. (2015). Ceramic Nanoparticles: Fabrication Methods and Applications in Drug Delivery. Cpd 21 (42), 6165–6188. doi:10.2174/1381612821666151027153246
Tilston, E. L., Collins, C. D., Mitchell, G. R., Princivalle, J., and Shaw, L. J. (2013). Nanoscale Zerovalent Iron Alters Soil Bacterial Community Structure and Inhibits Chloroaromatic Biodegradation Potential in Aroclor 1242-contaminated Soil. Environ. Pollut. 173, 38–46. doi:10.1016/j.envpol.2012.09.018
Vijayaraghavan, K., and Ashokkumar, T. (2017). Plant-mediated Biosynthesis of Metallic Nanoparticles: a Review of Literature, Factors Affecting Synthesis, Characterization Techniques and Applications. J. Environ. Chem. Eng. 5 (5), 4866–4883. doi:10.1016/j.jece.2017.09.026
Wang, H., Gao, B., Fang, J., Ok, Y. S., Xue, Y., Yang, K., et al. (2018). Engineered Biochar Derived from Eggshell-Treated Biomass for Removal of Aqueous lead. Ecol. Eng. 121, 124–129. doi:10.1016/j.ecoleng.2017.06.029
Yang, W., Hu, W., Zhang, J., Wang, W., Cai, R., Pan, M., et al. (2021). Tannic acid/Fe3+ Functionalized Magnetic Graphene Oxide Nanocomposite with High Loading of Silver Nanoparticles as Ultra-efficient Catalyst and Disinfectant for Wastewater Treatment. Chem. Eng. J. 405, 126629. doi:10.1016/j.cej.2020.126629
Yang, X., Wang, S., Bian, Y., Chen, F., Yu, G., Gu, C., et al. (2008). Dicofol Application Resulted in High DDTs Residue in Cotton fields from Northern Jiangsu Province, China. J. Hazard. Mater. 150 (1), 92–98. doi:10.1016/j.jhazmat.2007.04.076
Yu, B., Zeng, J., Gong, L., Zhang, M., Zhang, L., and Chen, X. (2007). Investigation of the Photocatalytic Degradation of Organochlorine Pesticides on a Nano-TiO2 Coated Film. Talanta 72 (5), 1667–1674. doi:10.1016/j.talanta.2007.03.013
Zaidi Ab, G., Yusoff, M. S., and Andas, J. (2015). Review on Applications of Nanoparticles in Landfill Leachate Treatment. Appl. Mech. Mater. 802, 525–530. doi:10.4028/www.scientific.net/amm.802.525
Keywords: wastewater, nanotechnology, solar, biodegradation, treatment, wastewater (sewage) treatment (WWT)
Citation: Nakum J and Bhattacharya D (2022) Various Green Nanomaterials Used for Wastewater and Soil Treatment: A Mini-Review. Front. Environ. Sci. 9:724814. doi: 10.3389/fenvs.2021.724814
Received: 14 June 2021; Accepted: 08 September 2021;
Published: 28 January 2022.
Edited by:
Abhrajyoti Tarafdar, Korea University, South KoreaReviewed by:
Fathy Elbehiry, Kafrelsheikh University, EgyptRam Naresh Bharagava, Babasaheb Bhimrao Ambedkar University, India
Copyright © 2022 Nakum and Bhattacharya. This is an open-access article distributed under the terms of the Creative Commons Attribution License (CC BY). The use, distribution or reproduction in other forums is permitted, provided the original author(s) and the copyright owner(s) are credited and that the original publication in this journal is cited, in accordance with accepted academic practice. No use, distribution or reproduction is permitted which does not comply with these terms.
*Correspondence: Debleena Bhattacharya, ZGVibGVlbmEuYmhhdHRhY2hhcnlhQGdtYWlsLmNvbQ==